DOI:
10.1039/D1RA00341K
(Review Article)
RSC Adv., 2021,
11, 15213-15230
Piperazine based antimicrobial polymers: a review
Received
14th January 2021
, Accepted 2nd April 2021
First published on 23rd April 2021
Abstract
Microbial infections are a life threatening concern in several areas, which include the biomedical sector, healthcare products, water purification systems, and food packaging. Polymers with low molecular weight bioactive agents or disinfectants help the scientific community to reduce the lethality rate caused by pathogenic microbes. Antimicrobial polymeric approach is one of the advanced approaches made by researchers in concern with the problems associated with small molecules that restrict their applications in broad spectrum. History reveals the synthesis of numerous antimicrobial polymers using various antimicrobial agents but lacks the use of piperazine molecule, which is of pharmaceutical importance. This review gives an insight into the current and future perspective for the development of piperazine-based antimicrobial polymers.
1. Introduction
The piperazine molecule has been classified as a privileged structural motif in drug discovery.1 It is a six-membered heterocyclic compound with the chemical formula C4H10N2 and is also called hexahydropyrazine. Hexahydropyrazine was named as piperazine because of its chemical similarity with piperidine, which is a part of the piperine structure isolated from the black pepper plant (Piper nigrum), and it contains two reactive secondary amine groups at the first and fourth positions.2,3 Piperazine was first used in the treatment of gout disease in 19th century, and later on molecules obtained by modifying the piperazine moiety were used in the treatment of intestinal infections.4–6 In the early 20th century, numerous researchers synthesized piperazine and substituted piperazine molecules, which were important pharmacophores found in numerous marketed drugs such as antibiotics, anticancer, antimicrobial, antipsychotics, and antidepressants.7 Piperazine was majorly found in the second generation antibiotic drugs and was extended up to sixth generation antibiotics.8,9 A recent statistical analysis of the substructure has shown that piperazine is the third most frequently used N-heterocycle (ranked right behind piperidine and pyridine) in pharmaceutical small molecule drugs. Several drugs containing the piperazine moiety are amongst the top 100 best-selling pharmaceutical products and are listed in Table 1.1,8–10
Table 1 List of commercialized drugs containing the piperazine moiety in pharmaceutical applications
S. no. |
Drug |
Activity |
Product name |
Company |
Ref. |
1 |
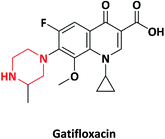 |
Antibiotic |
Gatiflo, Tequin and Zymar |
Kyorin Pharmaceut-icals, Japan |
11–13 |
2 |
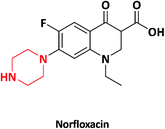 |
Noroxin |
Merck Co., USA |
14–16 |
3 |
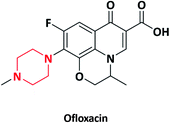 |
Floxin, Ocuflox |
Daiichi Sankyo, Japan |
17–19 |
4 |
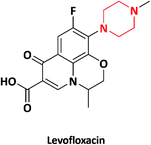 |
Antibiotic |
Levaquin |
Sanofi-Aventis, France |
20 and 21 |
5 |
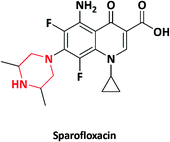 |
Spacin, Zagam |
Torrent pharmaceuticals, India |
22–24 |
6 |
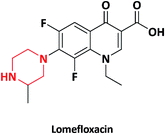 |
Maxaquin, Okacyn, Uniquin |
Wockhardt, India |
25 and 26 |
7 |
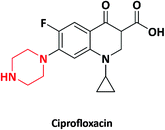 |
Antibacterial |
Cipro, Ciproxifan |
Bayer AG, Germany |
27–31 |
8 |
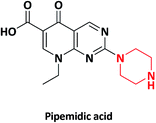 |
Pipemidic acid |
Yoshindo, Japan |
32–34 |
9 |
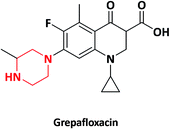 |
Raxar, Glaxo Wellcome |
GlaxoSmithKline, UK |
35–37 |
10 |
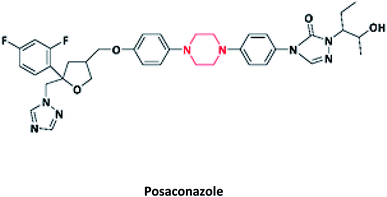 |
Antifungal |
Noxafil |
Schering-Plough, USA |
38–42 |
11 |
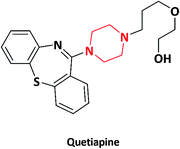 |
Antipsychotic |
Seroquel |
Biovail Corporation, Canada |
43–45 |
12 |
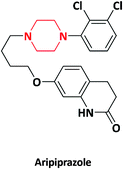 |
Abilify |
Otsuka pharmaceuticals, Japan |
46–48 |
13 |
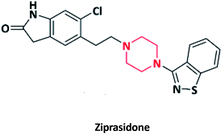 |
Geodon |
Pfizer, USA |
49–51 |
14 |
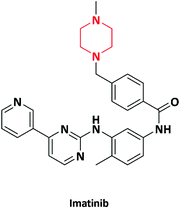 |
Anticancer |
Gleevec |
Ciba-Geigy (Navartis), Switzerland |
52–54 |
15 |
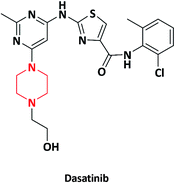 |
Sprycel |
Bristol-Myers Squibb, USA |
55–57 |
16 |
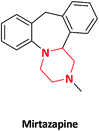 |
Antidepressant |
Remeron |
Adcock Ingram pharmaceuticals, South Africa |
58 |
17 |
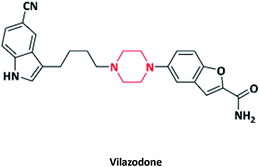 |
Viibryd |
Merck co., USA |
59–61 |
18 |
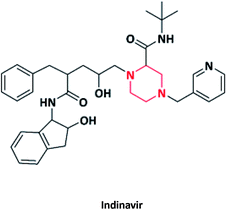 |
Antiretroviral |
Crixivan |
Merck co., USA |
62–64 |
19 |
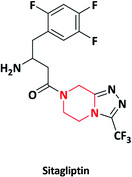 |
Antidiabetic |
Januvia, Tesavel, Xelevia |
Merck co., USA |
65–67 |
20 |
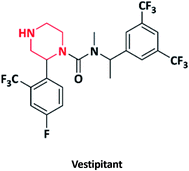 |
Anxiolytic |
Vestipitant |
GlaxoSmithKline, UK |
68–70 |
21 |
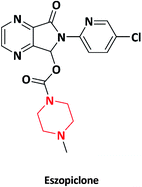 |
To treat insomnia |
Lunesta |
GlaxoSmithKline, UK |
71–73 |
22 |
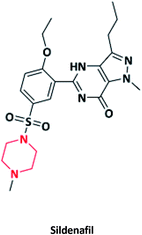 |
To treat erectile dysfunction |
Viagra |
Pfizer, USA |
74–76 |
Small-molecule drugs containing the piperazine moiety have substitutes on either both or single nitrogen atoms and are predominantly used as a linker for different molecules/macromolecules to adjust the physico-chemical properties of a macromolecule. Piperazine, a six-membered heterocyclic ring with two opposing nitrogen atoms, provides a large polar surface area, structural rigidity, and hydrogen-bond acceptors and donors, which often lead to enhanced target affinity, specificity, water solubility, oral bioavailability, and ADME (i.e., absorption, distribution, metabolism, and excretion) properties.2,77–82 The absence of vinyl groups and multifunctional groups in the piperazine molecule restricts their use in the synthesis of polymers though piperazine and substituted piperazine derivatives are extensively used as antibiotic, anticancer, antimicrobial, antipsychotic, antidepressant drugs and so forth.
Polymeric materials are one among those materials used in combating pathogenic microorganisms to prevent infectious diseases. The growth of pathogenic microorganisms in the surroundings can be inhibited or killed by the macromolecules with the antimicrobial property. The polymer molecules provide promise for escalating the efficacy of some existing low-molecular-weight antimicrobial agents and minimize environmental issues by reducing their residual toxicity. The applications of antimicrobial polymers can be found in water purification systems, fibers, food packaging, surfactants, detergents, surgical, pharmaceutical industries, and so forth.83–89 Some of the disadvantages of antimicrobial polymers are as follows: (i) macromolecules are very large and thus may not act as fast as small molecule agents, (ii) short half-life, and (iii) degradation issues.90,91 This review article gives an insight into the recent advancements in the synthesis, applications and development of novel piperazine-based antimicrobial monomers and polymers and is classified into two sub-sections based on their composition, i.e., homo-/co-/tripolymers and grafted polymers.
1.1 Piperazine-based homo-/co-/tripolymers
In 2003, norfloxacin-containing quinolone moieties were converted to monomer (Scheme 1) and polymers and further blended with numerous synthetic polymers and were tested for their antimicrobial activity, thermal stability and mechanical properties by Moon et al. The as-synthesized monomer, polymer and polymer blends exhibited potential activity against Escherichia coli (E. coli), Staphylococcus aureus (S. aureus), Bacillus subtilis (B. subtilis) and Micrococcus luteus (M. luteus). Quinolones were chosen for the synthesis of monomer, polymer and polymer blends in food packaging due to their high thermal stability. The moulding of the quinolone polymer was practically impossible because the polymer was brittle and rigid in nature. To reduce the rigidity, the quinolone polymer was blended with numerous synthetic polymers such as low-density polyethylene (LDPE), poly(methyl methacrylate) (PMMA), poly(butylene succinate) (PBS), polycaprolactone (PCL) and maleated polypropylene (PPMA) with varying concentrations of polymer quinolone from 1 wt% to 5 wt%. The quinolone polymer exhibited a potent antimicrobial activity even at the concentration of 1 wt% in the polymer blends. Due to the increase in the concentration of quinolone polymers to the polymer blends, there was a decrease in the tensile property. The decrease in the tensile property was due to the incompatibility between polymer quinolone and synthetic polymers. The decrease in the tensile properties due to compounding with polymer quinolone was less significant in the PCL/PQ blend compared to those in LDPE/PQ, PMMA/PQ, PBS/PQ, and PMMA/PQ blends. This indicated that PQ is more compatible with PCL compared to other polymers. Henceforth, the PCL/PQ blend was expected to have application in food packaging materials due to their antimicrobial activity, high thermal and mechanical properties.92
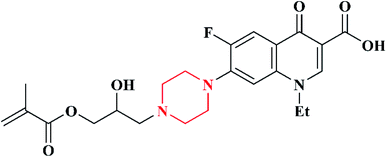 |
| Scheme 1 Acrylic monomer-containing quinolone moiety. | |
An efficient non-leaching contact-killing polymer was developed by Supriya et al. in 2006. First, piperazine was reacted with ethylene glycol dimethacrylate (EGDMA), followed by its quaternization using an alkyl iodide (1-iodooctane) to yield a quaternary monomer (Scheme 2). Further, the quaternized monomer was copolymerized with 2-hydroxyethyl methacrylate (HEMA) using redox initiators (ammonium per sulphate (APS) and N,N,N′,N′-tetramethyl ethylenediamine (TEMED)). The copolymers with varying quaternized monomer (QAMA) percentages (0 to 40%) were synthesized. Decline in the thermal properties was observed as the concentration of the monomer increased in the copolymer system, which was due to increase in the hydrocarbon chain length of alkyl iodide. The copolymer with a high monomer ratio exhibited increased activity against E. coli and S. aureus compared to other copolymers with a lower monomer concentration. The copolymer with 40% of the monomer concentration displayed 100% contact-killing activity in a time span of just 10 min.93
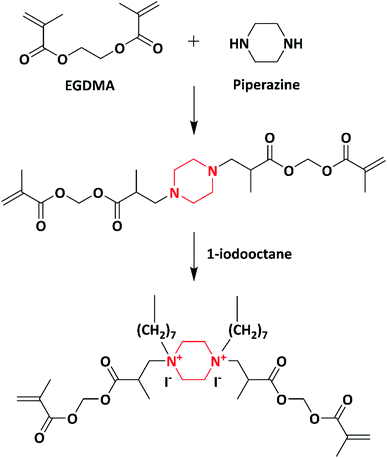 |
| Scheme 2 Synthesis of the quaternary amine methacrylate monomer. | |
Based on their previous study, the same authors in 2007 made an attempt to develop piperazine copolymers with non-leaching, contact-killing properties. Initially the methacrylate monomer, trimethylolpropane trimethacrylate–piperazine–ethyleneglycol dimethacrylate (TMPTMA–PPZ–EGDMA) (Scheme 3), was synthesized by the amination of trimethylolpropane trimethacrylate (TMPTMA) with piperazine (PPZ), followed by its reaction with ethyleneglycol dimethacrylate (EGDMA). The methacrylate monomer (TMPTMA-PPZ-EGDMA) was further copolymerized with 2-hydroxyethyl methacrylate (HEMA) using redox initiators (ammonium persulfate (APS) and N,N,N′,N′-tetramethyl ethylenediamine (TEMED)). The copolymers with varying monomer (TMPTMA–PPZ–EGDMA) percentages (0 to 100%) were synthesized, but after varying monomer (TMPTMA–PPZ–EGDMA) beyond 60% incomplete in the polymerization was observed. Further the obtained copolymers with different monomer ratios were quaternized with 1-iodooctane. Due to increase in the hydrocarbon chain length of the alkyl iodide, depletion in the decomposition temperature was observed as the concentration of the monomer (TMPTMA–PPZ–EGDMA) increased from 0 to 60% in the copolymer system. The quaternized copolymer with variable percentages of TMPTMA–PPZ–EGDMA from 5% to 60% was tested against E. coli and S. aureus. The site of quaternization increases as the monomer ratio increases; hence, the bacterial growth percentage decreases. The contact-killing microbiocidal role of the copolymer can be attributed to the hydrophobic interaction of the alkyl chain with the bilipid layer of the cell wall and the amphiphilic nature of the copolymer, which disrupts the cell membrane and leads to the leakage of K+ ions and cytoplasmic fluid. This mechanism was studied via scanning electron microscopy (SEM). The copolymer showed broad spectrum contact-killing antimicrobial properties without releasing any bioactive agents. Hence, the synthesized copolymer is used as contact-killing antimicrobial polymer for numerous biomedical applications.94
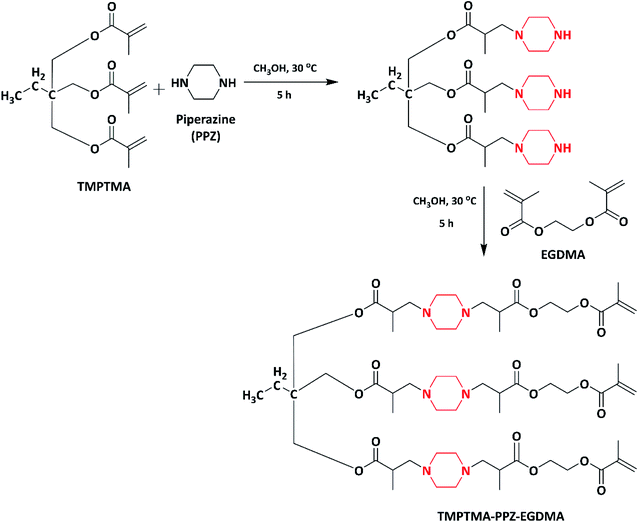 |
| Scheme 3 Synthesis of the trimethylolpropane trimethacrylate–piperazine–ethyleneglycol dimethacrylate monomer. | |
In 2012, a well-known second generation antibiotic drug, ciprofloxacin containing piperazine moiety, was converted to a novel methacrylate monomer and cationic tripolymers by Xue et al. The macromonomer (GCM) was synthesized by reacting ciprofloxacin (CPF) with glycidyl methacrylate. Further, the copolymerization of macromonomer (GCM) with acrylamide and diallyl dimethyl ammonium chloride (DADMAC) at different molar ratios was carried out via free radical polymerization using KPS as the initiator, which resulted in the formation of a cationic tripolymer (Scheme 4). The antimicrobial activity of the synthesized cationic tripolymers was screened against E. coli. As the macromonomer (GCM) concentration increased, the antimicrobial activity of the tripolymers also increased. The authors designed these CPF pendant cationic tripolymers for the purpose of antimicrobial paper products (such as tissues, paper towels, kitchen paper, food wrapper and bank notes) and for numerous hygiene products including cellulose based fibres.95
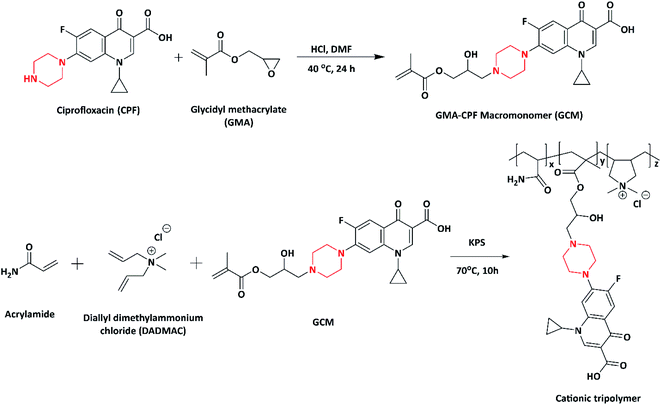 |
| Scheme 4 Synthesis of the ciprofloxacin pendant polymer (cationic tripolymer). | |
In 2018, Ahmed et al. designed cationic poly(guanylurea)s (PGUs) using piperazine and ethylenediamine for the development of biocompatible, specific, and selective antimicrobial polymers. Poly(guanylurea)–piperazine (PGU–P) and poly(guanylurea)–ethylenediamines (PGU–E) (Scheme 5) were synthesized by reacting piperazine and ethylenediamine with a monomer containing tert-butyloxycarbonyl (Boc)-protected guanidine groups at the end of short ethylene oxide side chains. The as-synthesized PGU–P exhibited broader antimicrobial activity compared to PGU–E with high selectivity to target against Mycobacterium smegmatis (M. smegmatis), S. aureus, methicillin resistant Staphylococcus aureus (MRSA), and Shigella flexneri (S. flexneri). The PGU–P polymer has key functional characteristics i.e., piperazine moiety, positive charge, H-bonding, and the lipophilicity, which help kill the bacteria via interacting with the cell membrane, followed by its disruption; the mechanism was studied via transmission electron microscopy (TEM).96
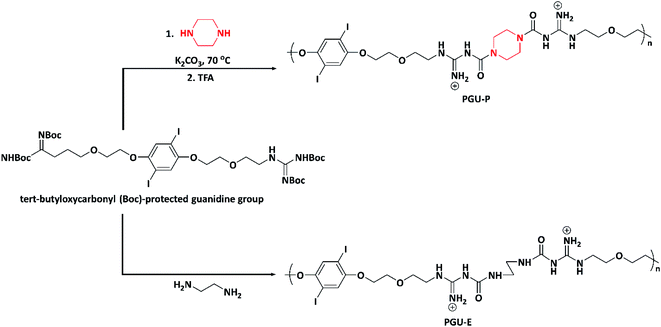 |
| Scheme 5 Synthesis of poly(guanylurea)–piperazine (PGU–P) and poly(guanylurea)–ethylenediamines (PGU–E). | |
In 2019, a novel piperazine–methacrylate monomer and its homopolymer were synthesized by Jalageri et al. Initially, piperazine and epichlorohydrin were used to react with each other to prepare a bifunctional coupler (piperazine-bearing aminochlorohydrin and azetidinium group). To increase the functionalities, the bifunctional coupler was quaternized with bromohexadecane and N,N′-dimethylaminoethyl methacrylate (DMAEMA) to yield a piperazine–methacrylate monomer. Further, the quaternized piperazine–methacrylate monomer was homopolymerized using an azobis(2-methylpropionitrile) (AIBN) initiator (thermal) yielding a multifunctionalized piperazine polymer (Scheme 6). The multifunctionalized piperazine polymer exhibited efficient antimicrobial activity against E. coli, M. smegmatis, S. aureus and Candida albicans (C. albicans) in comparison with standard drugs ciprofloxacin (antibacterial) and fluconazole (antifungal). The mode of action is as follows: the electrostatic interaction occurs between a negatively charged microbial cell wall and positively charged quaternary ammonium group that leads to cell lysis. The authors concluded that the as-synthesized piperazine-based polymer could be applicable in wound dressing, textile industry, biomedical field, water purification system, and so forth.74
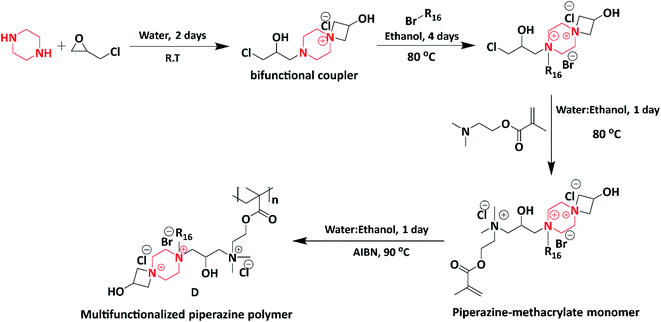 |
| Scheme 6 Synthesis of the piperazine–methacrylate monomer and its homopolymer. | |
In 2019, novel biocompatible piperazine polymer (PE) (Scheme 7) for bacterial repellence on biomedical materials was designed and synthesized via green synthetic route by Zhang et al. The piperazine polymer was prepared by the straight-forward reaction of piperazine with ethylenediaminetetraacetic dianhydride (EDTAD). The piperazine polymer exhibited significant antimicrobial activity against E. coli and S. aureus in comparison with standard antibacterial ciprofloxacin. Piperazine targets the cytoplasmic membrane of the bacteria, resulting in the leakage of intercellular components leading to cell death. Based on their antibacterial activity and biocompatibility, the authors reported that the polymer could be applicable in biomedical field.97,98
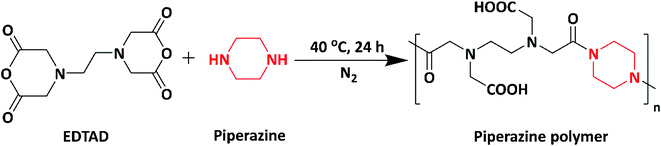 |
| Scheme 7 Synthesis of the biocompatible piperazine polymer. | |
Mechano-growth factor and its 24 amino acid peptide (MGF-Ct24E)-modified piperazine polymer (PEM) for biomedical applications was designed and synthesized by the same author in 2019. First, the piperazine polymer (PE) was synthesized by reacting piperazine with ethylenediaminetetraacetic dianhydride (EDTAD) via the condensation polymerization method. Further, the MGF-Ct24E peptide polymer was grafted to the as-synthesized piperazine polymer (PE) yielding a piperazine-modified polymer (PEM) (Scheme 8). The antimicrobial activity of PE and PEM was tested against E. coli and S. aureus, in which PE showed better antibacterial activity against E. coli compared to PEM. In case of S. aureus, both PE and PEM showed similar activity. After the insertion of MGF-Ct24E to PE, the as-synthesized polymeric material not only maintained the physical and chemical properties but also reduced the biological toxicity of PE and produced the balance between the antibacterial and biological toxicity. Piperazine and amino peptides target the cell wall and cytoplasmic membrane of the bacteria, eventually leading to the leakage of cytoplasmic fluid and cell death; the mechanism was studied via scanning electron microscopy (SEM). The authors stated that the as-synthesized polymer could be applicable in biomedical field for the repair of bone, muscle and neuronal tissues.97,99 Numerous piperazine-based homo/co/tripolymers are listed in Table 2.
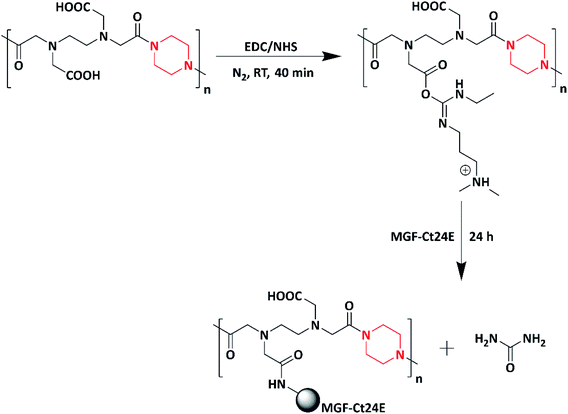 |
| Scheme 8 Synthesis of the MGF-Ct24E-modified piperazine polymer. | |
Table 2 List of piperazine-based homo/co/tripolymers synthesized by researchers against numerous pathogenic microorganisms
S. no. |
Year |
Author |
Monomer/Polymer |
Antimicrobial activity |
Applications |
Ref. |
1 |
2003 |
Woong sig moon et al. |
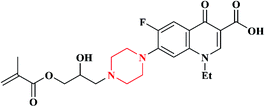 |
E. coli, S. aureus, B. subtilis, and M. luteus |
Food packaging |
92 |
2 |
2006 |
Supriya et al. |
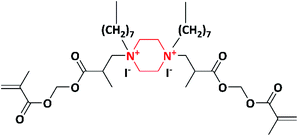 |
E. coli and S. aureus |
Biomedical |
93 |
3 |
2007 |
Supriya et al. |
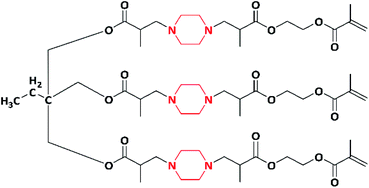 |
E. coli and S. aureus |
Biomedical |
94 |
4 |
2012 |
Xue et al. |
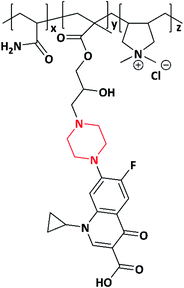 |
E. coli |
Paper products |
95 |
5 |
2018 |
Ahmed et al. |
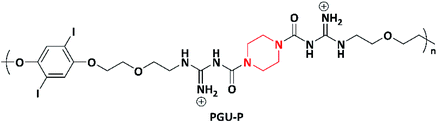 |
M. smegmatis, S. aureus, MRSA, and S. flexneri |
Biomedical |
96 |
6 |
2019 |
Jalageri et al. |
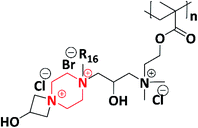 |
E. coli, S. aureus, M. smegmatis and C. albicans |
Biomedical, water purification and textile |
74 |
7 |
2019 |
Zhang et al. |
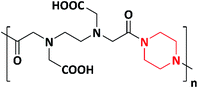 |
E. coli and S. aureus |
Biomedical |
98 |
8 |
2019 |
Zhang et al. |
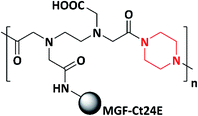 |
E. coli and S. aureus |
Biomedical |
97 and 99 |
1.2 Piperazine grafted polymers
In 2014, Subrata et al. developed non-leaching antimicrobial surfaces in a solution by synthesizing azetidinium-functionalized polytetrahydrofurans. Initially, they prepared a bifunctional coupler (piperazine-bearing amino chlorohydrin and azetidinium group) using piperazine and epichlorohydrin. The as-synthesized bifunctional coupler was further post-polymerized with synthetic polymer aminotelechelic polytetrahydrofuran (PTHF) [XTJ-548], resulting in the formation of azetidinium-functionalized polytetrahydrofuran polymers (Scheme 9). The authors concluded that the as-synthesized functional polymer could be applicable to solve the problems associated with hospital environment because of its excellent activity exhibited (>9.99–100%) in both solution and on the surfaces of textiles against E. coli and S. aureus. Azetidinium-functionalized polymers possess cationic nature, ionic interaction and covalent linkage, which helps to inhibit the bacterial growth via interacting with cell wall, followed by its death.100,101
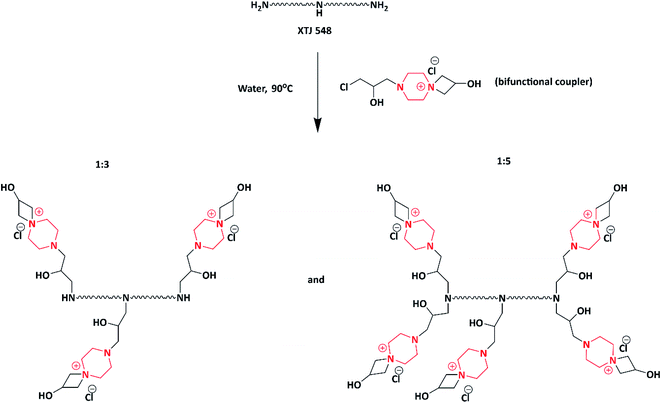 |
| Scheme 9 Synthesis of functionalized polytetrahydrofurans. | |
The same author in 2014 fabricated antimicrobial fabrics by coating synthesized multifunctional polymers induced with piperazine on to cotton fibre. A one pot synthesis method was adopted to synthesize multifunctional polymers. Piperazine was reacted with epichlorohydrin to produce a bifunctional coupler, which helped in producing polymers. Further to induce hydrophobicity, the bifunctional coupler was reacted with decylamine (C-10 amine) and hexylamine (C-6 amine) to produce two different hydrophobic couplers. Hydrophobic couplers and bifunctional couplers were introduced to the side chain of Poly(vinyl amine)s (PVAms) using the post-polymerization method to produce multifunctional azetidinium-functionalized Poly(vinyl amine)s (PVAms) (Scheme 10), which mimic the natural antimicrobial peptides. Further, these polymers were coated onto cotton fabrics and tested for their antimicrobial activity against E. coli and S. aureus. Due to the presence of alkyl chains and cationic moieties (hydrophobic and hydrophilic modifications), the polymers exhibited efficient activity against tested microbes.102
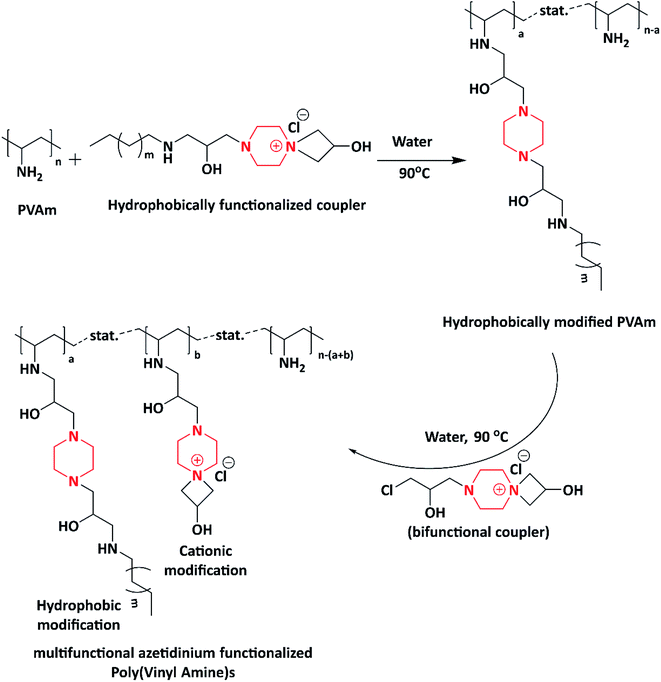 |
| Scheme 10 Step-wise synthesis of multifunctional azetidinium-functionalized Poly(vinyl amine) by reacting PVAm with the functionalized coupler and the bifunctional coupler. | |
Jalageri et al. in 2019 synthesized an eco-friendly functionalized Jeffamine polymer having the piperazine moiety for surface coating applications. The functionalized Jeffamine polymer (Scheme 11) was synthesized by the post-polymerization of Jeffamine ED-2003 (having molecular weight of 2000) with a bifunctional coupler (piperazine-bearing aminochlorohydrin and azetidinium group) via a one-pot synthesis method. Because of the presence of the piperazine moiety, counter ions (Cl−), hydroxyl (–OH), and quaternary ammonium groups, the functionalized Jeffamine polymer exhibited antimicrobial activity against E. coli, M. smegmatis, S. aureus and C. albicans. The antimicrobial action of the functionalized Jeffamine polymer is ascribed to the quaternary ammonium group, which leads to cell lysis where electrostatic interaction takes place between the positively charged Jeffamine polymer and negatively charged microbial cell wall. In addition, it also contains –OH groups associated with azetidinium and amino-chlorohydrin, which are known for targeting the cytoplasmic membrane of microorganisms. This leads to leakage in intercellular components, followed by the death of microbes; the mechanism was studied via scanning electron microscopy (SEM). The author conveyed that the as-synthesized functionalized Jeffamine polymer having the piperazine moiety could be applicable in antimicrobial coatings, biomedical applications, textile industries, water purification system, and so forth.2 Numerous piperazine-grafted polymers are listed in Table 3.
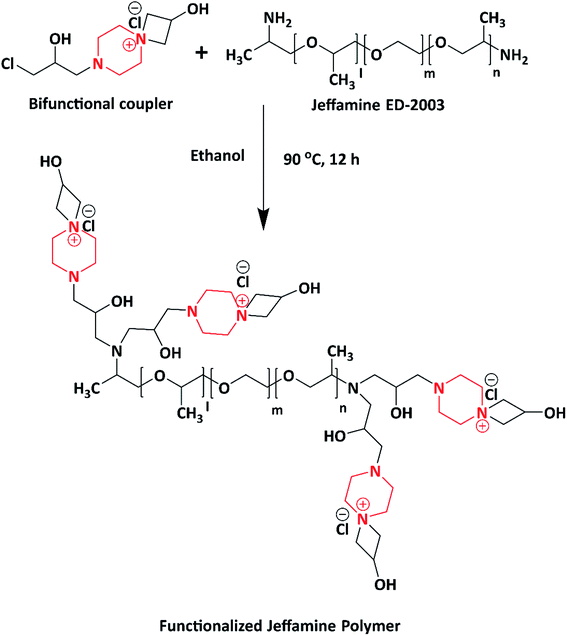 |
| Scheme 11 Synthesis of the functionalized Jeffamine polymer. | |
Table 3 List of piperazine grafted polymers synthesized by researchers against numerous pathogenic microorganisms
S. no. |
Year |
Author |
Polymer |
Antimicrobial activity |
Applications |
Ref. |
1 |
2012 |
Subrata et al. |
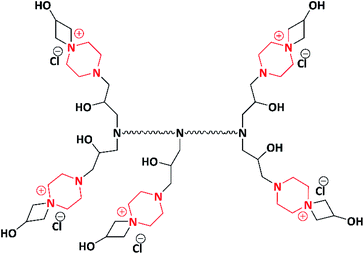 |
E. coli and S. aureus |
Textile |
100 and 101 |
2 |
2013 |
Subrata et al. |
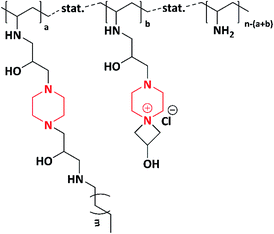 |
E. coli and S. aureus |
Textile |
102 |
3 |
2019 |
Jalageri et al. |
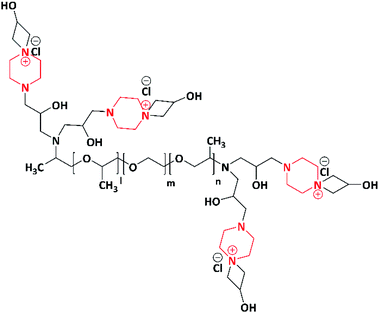 |
E. coli, S. aureus, M. smegmatis and C. albicans |
Biomedical, coatings, and textile |
2 |
2. Conclusion
In summary, this review highlights the use of piperazine in the synthesis of antimicrobial polymers due to their wide range of pharmacological activities, which lead to the development of new therapeutic agents. In this aspect, piperazine-based antimicrobial polymers have gained interest from both academic and industrial researchers. The probable drawbacks and challenges encountered in the synthesis of piperazine polymers are (i) difficultly in handling due to its hygroscopic nature that liquidifies by absorbing moisture from air, therefore interrupting in the polymerization process, (ii) it contains only two reactive sites: secondary amines that restrict the adaptability of conversion to monomer or limits itself in incorporating into polymer structures, (iii) synthesized polymers may encounter difficulty in maintaining physical stability due to the hygroscopic nature of piperazine, (iv) polymer synthesis involves a multi-step synthetic procedure, which makes the purification of the polymer laborious. By considering the future perspectives of piperazine, a highly potential pharmacological interest can be used for the synthesis of antimicrobial polymers, which may have significant importance in sectors such as biomedical, food packaging and storage, textile, drug carriers, wound dressing, water purification system, and health care products.
Conflicts of interest
There are no conflicts to declare.
References
- K. E. Gettys, Z. Ye and M. Dai, Recent Advances in Piperazine Synthesis, Synthesis, 2017, 49, 2589–2604 CrossRef CAS.
- M. D. Jalageri, Y. M. Puttaiahgowda, A. M. Parambil and T. Varadavenkatesan, Synthesis and fabrication of highly functionalized Jeffamine antimicrobial polymeric coating, Polym. Adv. Technol., 2019, 30, 1616–1627 CrossRef CAS.
- M. D. Jalageri, Y. Malgar Puttaiahgowda, A. M. Parambil and A. Kulal, Design of multifunctionalized piperazine polymer and its activity toward pathogenic microorganisms, J. Appl. Polym. Sci., 2019, 136, 47521 CrossRef.
- W. C. Campbell, Serendipity and New Drugs for Infectious Disease, ILAR J., 2005, 46, 352–356 CrossRef CAS PubMed.
- J. Gordon, A Contribution to the Study of Piperazine, BMJ, 1894, 1, 1291–1294 CrossRef CAS PubMed.
- M. Bonelli, Citrosalicylate of piperazine in therapy of uric acid diathesis, Minerva Med., 1954, 45, 221 CAS.
- M. Shaquiquzzaman, G. Verma, A. Marella, M. Akhter, W. Akhtar, M. F. Khan, S. Tasneem and M. M. Alam, Piperazine scaffold: A remarkable tool in generation of diverse pharmacological agents, Eur. J. Med. Chem., 2015, 102, 487–529 CrossRef CAS PubMed.
- C.-C. Guo, H.-P. Li and X.-B. Zhang, Study on synthesis, characterization and biological activity of some new nitrogen heterocycle porphyrins, Bioorg. Med. Chem., 2003, 11, 1745–1751 CrossRef CAS PubMed.
- C.-C. Guo, R.-B. Tong and K.-L. Li, Chloroalkyl piperazine and nitrogen mustard porphyrins: synthesis and anticancer activity, Bioorg. Med. Chem., 2004, 12, 2469–2475 CrossRef CAS PubMed.
- E. Vitaku, D. T. Smith and J. T. Njardarson, Analysis of the Structural Diversity, Substitution Patterns, and Frequency of Nitrogen Heterocycles among U.S. FDA Approved Pharmaceuticals, J. Med. Chem., 2014, 57, 10257–10274 CrossRef CAS PubMed.
- R. S. Howard and A. Laboratories, The Effect of Fourth-Generation Fluoroquinolones Gatifloxacin and Moxifloxacin on Epithelial Healing Following Photorefractive Keratectomy, Am. J. Ophthalmol., 2005, 140, 83–87 CrossRef PubMed.
- L. J. Cervantes and F. S. Mah, Clinical use of gatifloxacin ophthalmic solution for treatment of bacterial conjunctivitis, Clin. Ophthalmol., 2011, 5, 495–502 CAS.
- C. Schultz, Ophthalmology and Eye Diseases Gatifloxacin Ophthalmic Solution for Treatment of Bacterial Conjunctivitis: Safety , Efficacy and Patient Perspective, Ophthalmol. Eye Dis., 2012, 4, 65–70 CAS.
- B. Holmes, R. N. Brogden and D. M. Richards, Norfloxacin A Review of Its Antibacterial Activity , Pharmacokinetic Properties and Therapeutic Use, Drug Eval., 1985, 30, 482–513 CrossRef CAS PubMed.
- P. N. R. Heseltine, Compassionate Use of Norfloxacin, Am. J. Med., 1987, 82, 88–92 CrossRef CAS PubMed.
- H. Aizawa, L. L. Gan and C. Prakash, Handbook of Metabolic Pathways of Xenobiotics, 2014, pp. 55–56 Search PubMed.
- D. El, J. P. Monk, D. M. Campoli-richards and C. Chemioterapia, Ofloxacin: A Review of its Antibacterial Activity , Pharmacokinetic Properties and Therapeutic Use, Drugs, 1987, 33, 346–391 CrossRef PubMed.
- J. Michael, FORMULARY FORUM, Ann. Pharmacother., 1989, 23, 839–846 Search PubMed.
- P. A. Todd and D. Faulds, A Reappraisal of its Antimicrobial Activity, Pharmacology and Therapeutic Use, Drugs, 1991, 42, 825–876 CrossRef CAS PubMed.
- P. Ball, Efficacy and Safety of Levofloxacin in the Context of Other Contemporary Fluoroquinolones: A Review, Curr. Ther. Res., 2003, 64, 646–661 CrossRef CAS PubMed.
- G. J. Noel, A Review of Levo fl oxacin for the Treatment of Bacterial Infections, Clin. Med.: Ther., 2009, 1, 433–458 CAS.
- Y. I. J Shimada and T. Nogita, Clinical Pharmacokinetics of Sparfloxacin, Clin. Pharmacokinet., 1993, 25, 358–369 CrossRef PubMed.
- H. Lode, M. Aubier, H. Portier, A. Ovtqvist and S. Group, Sparfloxacin as alternative treatment to standard therapy for community-acquired bacterernic pneumococcal pneumonia, Clin. Microbiol. Infect., 1998, 4, 135–143 CrossRef CAS PubMed.
- J. J. Schentag, Sparfloxacin: A Review, Clin. Ther., 2000, 22, 372–387 CrossRef CAS PubMed.
- E. Rubinstein, History of quinolones and their side effects, Chemotherapy, 2001, 47, 3–8 CrossRef CAS PubMed.
- R. I. Al-Wabli, Profiles of Drug Substances, Excipients and Related Methodology, 2017, vol. 42, pp. 193–240 Search PubMed.
- R. Wise, J. M. Andrews and L. J. Edwards, Vitro Activity of Bay 09867 , a New Quinoline Derivative , Compared with Those of Other Antimicrobial Agents, Am. Soc. Microbiol., 1983, 23, 559–564 CAS.
- L. M. Fisher, J. M. Lawrence, I. C. Josty, R. Hopewell and E. E. Margerrison, Ciprofloxacin and the Fluoroquinolones New Concepts on the Mechanism of Action and Resistance, Am. J. Med., 1989, 87, 2–8 CrossRef.
- B. Fantin, X. Duval, L. Massias, L. Alavoine, S. Retout, A. Andremont and F. Mentre, Ciprofloxaci Dosage and Emergence of Resistance in Human Commensal Bacteria, J. Infect. Dis., 2009, 200, 390–398 CrossRef CAS PubMed.
- P. C. Sharma, A. Jain, S. Jain, R. Pahwa and M. S. Yar, Ciprofloxacin: review on developments in synthetic, analytical, and medicinal aspects, J. Enzyme Inhib. Med. Chem., 2010, 25, 577–589 CrossRef CAS PubMed.
- D. Sharma, R. P. Patel, S. T. R. Zaidi, G. M. Camerino, B. Aldo and L. A. Moraes, Interplay of the Quality of Ciprofloxacin and Antibiotic Resistance in Developing Countries, Front. Pharmacol., 2017, 8, 1–7 Search PubMed.
- M. Shimizu, Y. Takase, S. Nakamura, H. Katae, A. Minami, K. Nakata and N. Kurobe, Pipemidic Acid: Its Activities Against Various Experimental Infections, Antimicrob. Agents Chemother., 1976, 9, 569–574 CrossRef CAS PubMed.
- M. Sugiura, R. Hayakawa and T. Osada, Fixed drug eruption due to amlexanox, Contact Dermatitis, 1998, 38, 65–67 CrossRef CAS PubMed.
- J. M. Brogard, F. Comte and J. Lavillaureix, Comparative pharmacokinetic profiles of Cinoxacin and pipemidic acid in humans, Eur. J. Drug Metab. Pharmacokinet., 1983, 8, 251–259 CrossRef CAS PubMed.
- J. Child, J. M. Andrews and R. Wise, Pharmacokinetics and tissue penetration of the new fluoroquinolone grepafloxacin, Antimicrob. Agents Chemother., 1995, 39, 513–515 CrossRef CAS PubMed.
- C. E. Langan, P. Zuck, F. Vogel, A. McIvor, W. Pierzchala, M. Smakal, H. Staley and C. Marr, Randomized, double-blind study of short-course (5 day) grepafloxacin versus 10 day clarithromycin in patients with acute bacterial exacerbations of chronic bronchitis, J. Antimicrob. Chemother., 1999, 44, 515–523 CrossRef CAS PubMed.
- H. Lode, F. Vogel and W. Elies, Grepafloxacin: A review of its safety profile based on clinical trials and postmarketing surveillance, Clin. Ther., 1999, 21, 61–74 CrossRef CAS PubMed.
- I. I. Raad, J. R. Graybill, A. B. Bustamante, O. A. Cornely, V. Gaona-flores, C. Afif, D. R. Graham, R. N. Greenberg, S. Hadley, A. Langston, R. Negroni, J. R. Perfect, P. Pitisuttithum, A. Restrepo, G. Schiller, L. Pedicone and A. J. Ullmann, Safety of Long-Term Oral Posaconazole Use in the Treatment of Refractory Invasive Fungal Infections, Clin. Infect. Dis., 2006, 42, 1726–1734 CrossRef CAS PubMed.
- N. D. Greer, Posaconazole (Noxafil): a new triazole antifungal agent, Baylor Univ. Med. Cent. Proc., 2007, 20, 188–196 CrossRef PubMed.
- V. Nagappan and S. Deresinski, Posaconazole: A Broad-Spectrum Triazole Antifungal Agent, Clin. Infect. Dis., 2007, 45, 1610–1617 CrossRef CAS PubMed.
- S. Antonio, Posaconazole Therapeutic Drug Monitoring: a Reference Laboratory Experience, Antimicrob. Agents Chemother., 2009, 53, 2223–2224 CrossRef PubMed.
- J. N. Moore, J. R. Healy, W. K. Kraft, E. Therapeutics, S. T. Street and M. Building, HHS Public Access, Expert Rev. Clin. Pharmacol., 2016, 8, 321–334 CrossRef PubMed.
- N. S. Gunasekara, C. M. Spencer, A. G. Awad, R. L. Borison, B. Park, G. D. Burrows, P. Meats, M. Unit, K. Mill and J. Peuskens, Quetiapine: A Review of its Use in Schizophrenia, CNS Drugs, 1998, 9, 325–340 CrossRef CAS PubMed.
- E. J. Daly, A review of quetiapine in combination with antidepressant therapy in patients with depression, Neuropsychiatr. Dis. Treat., 2007, 3, 855–867 CrossRef CAS PubMed.
- K. Komossa, D. Am, A. Gaudchau, W. Kissling and S. Leucht, Second-generation antipsychotics for major depressive disorder and dysthymia (Review), Cochrane database Syst. Rev., 2010, 8, 1–230 Search PubMed.
- M. A. Grady, T. L. Gasperoni and P. Kirkpatrick, Aripiprazole, Nat. Rev. Drug Discovery, 2003, 2, 427–428 CrossRef CAS PubMed.
- L. Citrome, Review of aripiprazole in the treatment of patients with schizophrenia or bipolar I disorder, Neuropsychiatr. Dis. Treat., 2006, 2, 427–443 CrossRef CAS PubMed.
- A. De Bartolomeis, C. Tomasetti and F. Iasevoli, Update on the Mechanism of Action of Aripiprazole: Translational Insights into Antipsychotic Strategies Beyond Dopamine Receptor Antagonism, CNS Drugs, 2015, 29, 773–799 CrossRef CAS PubMed.
- L. Citrome, Using oral ziprasidone effectively: the food effect and dose-response, Adv. Ther., 2009, 26, 739–748 CrossRef CAS PubMed.
- C. Mattei, M. P. Rapagnani and S. M. Stahl, Ziprasidone Hydrocloride: What Role in the Management of Schizophrenia?, J. Cent. Nerv. Syst. Dis., 2011, 3, S4138 CrossRef PubMed.
- D. M. Gardner, A. L. Murphy, S. Kutcher, S. Beaulieu, C. Carandang, A. Labelle, P. Lalonde, A. Malla, H. Milliken, C. O'Donovan, A. Schaffer, J. Soni, V. H. Taylor and R. Williams, Evidence review and clinical guidance for the use of ziprasidone in Canada, Ann. Gen. Psychiatry, 2013, 12, 1 CrossRef PubMed.
- R. Capdeville, S. Silberman and S. Dimitrijevic, Imatinib: the first 3 years, Eur. J. Cancer, 2002, 38, S77–S82 CrossRef PubMed.
- N. Iqbal and N. Iqbal, Imatinib: A Breakthrough of Targeted Therapy in Cancer, Chemother. Res. Pract., 2014, 1–9 Search PubMed.
- T. Sacha, Imatinib in Chronic Myeloid Leukemia: an Overview, Mediterr. J. Hematol. Infect. Dis., 2014, 6, 1–9 Search PubMed.
- A. Hochhaus and H. Kantarjian, The development of dasatinib as a treatment for chronic myeloid leukemia (CML): from initial studies to application in newly diagnosed patients, J. Cancer Res. Clin. Oncol., 2013, 139, 1971–1984 CrossRef CAS PubMed.
- C. Garbe and T. K. Eigentler, Small Molecules in Oncology, Springer Berlin Heidelberg, Berlin, Heidelberg, 2014, vol. 201 Search PubMed.
- D. Keskin, S. Sadri and A. E. Eskazan, Dasatinib for the treatment of chronic myeloid leukemia: patient selection and special considerations, Drug Des., Dev. Ther., 2016, 10, 3355–3361 CrossRef CAS PubMed.
- K. F. Croom, C. M. Perry and G. L. Plosker, Mirtazapine: A Review of its Use in Major Depression and Other, CNS Drugs, 2009, 23, 427–452 CrossRef CAS PubMed.
- M. P. Cruz, Vilazodone HCL (Viibryd): A serotonin partial agonist and reuptake inhibitor for the treatment of major depressive disorder, P T, 2012, 37, 28–31 Search PubMed.
- S.-M. Wang, C. Han, S.-J. Lee, A. A. Patkar, P. S. Masand and C.-U. Pae, Vilazodone for the Treatment of Depression: An Update, Chonnam Med. J., 2016, 52, 91 CrossRef CAS PubMed.
- Z. T. Sahli, P. Banerjee and F. I. Tarazi, The Preclinical and Clinical Effects of Vilazodone for the Treatment of Major Depressive Disorder, Expert Opin. Drug Discovery, 2016, 11, 515–523 CrossRef CAS PubMed.
- G. L. Plosker, S. Noble, H. S. Limited, B. G. Gazzard, H. I. V Gum, W. Hospital, R. M. Gulick, C. Clinical, T. Unit, N. York, N. York, M. Harris, J. Ruedy, I. Deficiency, S. Paul, B. Columbia, I. Tropicales, H. Rothschild, U. Wintergerst and C. Hospital, A Review of its Use in the Management of HIV Infection, Drugs, 1999, 58, 1165–1203 CrossRef CAS PubMed.
- D. S. Wu and M. L. Stoller, Indinavir urolithiasis, Curr. Opin. Neurol.Curr. Opin. Urol., 2000, 10, 558–561 Search PubMed.
- A. F. Giorgio, M. Rouquayrol, J. Greiner, A. Aubertin, P. Vierling and R. Guedj, Synthesis and anti-HIV activity of prodrugs derived from saquinavir and indinavir, Antiviral Chem. Chemother., 2000, 11, 97–110 CrossRef CAS.
- B. Gallwitz, Review of sitagliptin phosphate: a novel treatment for type 2 diabetes, Vasc. Health Risk Manage., 2007, 3, 203–210 CrossRef CAS PubMed.
- E. L. S. Onge, S. Miller and E. Clements, Sitagliptin/Metformin (Janumet) as Combination Therapy In the Treatment of Type-2 Diabetes Mellitus, P T, 2012, 37, 4135–4141 Search PubMed.
- L. J. Scott, Sitagliptin: A Review in Type 2 Diabetes, Drugs, 2017, 209–224 CrossRef CAS PubMed.
- G. K. Reddy, R. J. Gralla and P. J. Hesketh, mini- review, Supportive Cancer Ther., 2006, 3, 140–142 CrossRef CAS PubMed.
- M. Brocco, A. Dekeyne, C. Mannoury, M. Touzard, S. Girardon, S. Veiga, G. De Nanteuil, R. Trynke, B. Olivier and M. J. Millan, Cellular and behavioural profile of the novel , selective neurokinin 1 receptor antagonist , vestipitant: A comparison to other agents, Eur. Neuropsychopharmacol., 2008, 18, 729–750 CrossRef CAS PubMed.
- E. Ratti, D. J. Carpenter, S. Zamuner, S. Fernandes, L. Squassante and H. Danker-hopfe, Efficacy of Vestipitant, A Neurokinin-1 Receptor Antagonist, Primary Insomnia, Sleep, 2013,vol. 3 6, pp. 1823–1830 Search PubMed.
- B. D. Brielmaier, Eszopiclone (Lunesta): A New Nonbenzodiazepine Hypnotic Agent, Proc. (Bayer Univ. Med. Content), 2006, 19, 54–59 Search PubMed.
- A. N. Siriwardena, Effectiveness of non-benzodiazepine hypnotics in treatment of adult insomnia: meta-analysis of data, BMJ, 2012, 1–13 Search PubMed.
- S. Rösner, C. Englbrecht, R. Wehrle, G. Hajak and M. Soyka, Eszopiclone for insomnia (Review), Cochrane Database Syst. Rev., 2018, 1–129 Search PubMed.
- I. P. Levinson, I. M. Khalaf and K. Z. M. Shaeer, Efficacy and safety of sildenafil citrate (Viagra s) for the treatment of erectile dysfunction in men in Egypt and South Africa, Int. J. Impotence Res., 2003, 15, S25–S29 CrossRef CAS PubMed.
- K. Hatzimouratidis, Sildenafi l in the treatment of erectile dysfunction: an overview of the clinical evidence, Clin. Interventions Aging, 2006, 1, 403–414 CrossRef CAS PubMed.
- B. Gong, M. Ma, W. Xie, X. Yang and Y. Huang, Direct comparison of tadalafil with sildenafil for the treatment of erectile dysfunction: a systematic review and meta - analysis, Int. Urol. Nephrol., 2017, 49, 1731–1740 CrossRef CAS PubMed.
- M. A. Walker, Novel tactics for designing water-soluble molecules in drug discovery, Expert Opin. Drug Discov., 2014, 9, 1421–1433 CrossRef CAS PubMed.
- C.-V. T. Vo and J. W. Bode, Synthesis of Saturated N-Heterocycles, J. Org. Chem., 2014, 79, 2809–2815 CrossRef CAS PubMed.
- M. D. Jalageri, Y. Malgar Puttaiahgowda, A. M. Parambil and A. Kulal, Design of multifunctionalized piperazine polymer and its activity toward pathogenic microorganisms, J. Appl. Polym. Sci., 2019, 47521 CrossRef.
- S. Kanth, A. Nagaraja and Y. M. Puttaiahgowda, Polymeric approach to combat drug-resistant methicillin-resistant Staphylococcus
aureus, J. Mater. Sci., 2021, 56, 7265–7285 CrossRef CAS PubMed.
- M. D. Jalageri, Y. M. Puttaiahgowda and Hariprasad, Design and antimicrobial activity of piperazine polymer nanocomposite, Mater. Today: Proc., 2019, 15, 262–267 CAS.
- N. Akshatha and M. P. Yashoda, Synthesis of environmental-friendly polymer nanocomposite against pathogenic microorganism, Mater. Today: Proc., 2019, 15, 273–276 Search PubMed.
- A. Nagaraja, M. D. Jalageri, Y. M. Puttaiahgowda, K. Raghava Reddy and A. V Raghu, A review on various maleic anhydride antimicrobial polymers, J. Microbiol. Methods, 2019, 163, 105650 CrossRef CAS.
- A. Nagaraja, Y. M. Puttaiahgowda and D. Devadiga, Synthesis and fabrication of high-potent antimicrobial polymeric ultrathin coatings, J. Appl. Polym. Sci., 2019, 136, 47893 CrossRef.
- A. Nagaraja, Y. M. Puttaiahgowda, A. Kulal, A. M. Parambil and T. Varadavenkatesan, Synthesis, Characterization, and Fabrication of Hydrophilic Antimicrobial Polymer Thin Film Coatings, Macromol. Res., 2019, 27, 301–309 CrossRef CAS.
- A. Nagaraja, M. D. Jalageri and Y. M. Puttaiahgowda, Engineered Antimicrobial Surfaces, 2020, pp. 123–134 Search PubMed.
- M. P. Ajithkumar, M. P. Yashoda, S. Prasannakumar, T. V. Sruth and V. B. Sameer Kumar, Poly(N-vinyl-2-pyrrolidone-maleic anhydride-styrene) grafted terpolymer: Synthesis, characterization, and bactericidal property evaluation against E. coli and S. epidermidis, J. Macromol. Sci., Part A: Pure Appl.Chem., 2017, 54, 480–488 CrossRef CAS.
- M. P. Ajithkumar, M. P. Yashoda, S. Prasannakumar, T. V. Sruthi and V. B. Sameer Kumar, Synthesis, characterization, microstructure determination, thermal studies of poly (N-vinyl pyrrolidone-maleic anhydride-methyl methacrylate), J. Macromol. Sci., Part A: Pure Appl.Chem., 2018, 55, 362–368 CrossRef CAS.
- N. Venugopal, G. Krishnamurthy, H. S. Bhojya Naik and J. D. Manohara, DNA Binding, Molecular Docking and Antimicrobial Evaluation of Novel Azo Dye Ligand and Their Metal Complexes, J. Inorg. Organomet. Polym. Mater., 2020, 30, 2608–2625 CrossRef CAS.
- E.-R. Kenawy, S. D. Worley and R. Broughton, The Chemistry and Applications of Antimicrobial Polymers: A State-of-the-Art Review, Biomacromolecules, 2007, 8, 1359–1384 CrossRef CAS PubMed.
- N. F. Kamaruzzaman, L. P. Tan, R. H. Hamdan, S. S. Choong, W. K. Wong, A. J. Gibson, A. Chivu and M. De Fatima Pina, Antimicrobial polymers: The potential replacement of existing antibiotics?, Int. J. Mol. Sci., 2019, 20(11), 2747 CrossRef CAS PubMed.
- W. S. Moon, J. C. Kim, K. H. Chung, E. S. Park, M. N. Kim and J. S. Yoon, Antimicrobial activity of a monomer and its polymer based on quinolone, J. Appl. Polym. Sci., 2003, 90, 1797–1801 CrossRef CAS.
- S. Punyani and H. Singh, Preparation of iodine containing quaternary amine methacrylate copolymers and their contact killing antimicrobial properties, J. Appl. Polym. Sci., 2006, 102, 1038–1044 CrossRef CAS.
- S. Punyani and H. Singh, Synthesis, characterization, and antimicrobial properties of novel quaternary amine methacrylate copolymers, J. Appl. Polym. Sci., 2008, 107, 2861–2870 CrossRef CAS.
- Y. Xue, Y. Guan, A. Zheng, H. Wang and H. Xiao, Synthesis and Characterization of Ciprofloxacin Pendant Antibacterial Cationic Polymers, J. Biomater. Sci., Polym. Ed., 2012, 23, 1115–1128 CrossRef CAS PubMed.
- M. S. Ahmed, T. Annamalai, X. Li, A. Seddek, P. Teng, Y.-C. Tse-Dinh and J. H. Moon, Synthesis of Antimicrobial Poly(guanylurea)s, Bioconjugate Chem., 2018, 29, 1006–1009 CrossRef CAS PubMed.
- M. Zhang, Y. Wang, J. Sun, J. Wu, W. Yan and Y. Zheng, Design and Synthesis of Novel Piperazine Derivatives with High Antibacterial Activity, Chem. Lett., 2013, 42, 227–228 CrossRef CAS.
- M. Zhang, G. Zeng, X. Liao and Y. Wang, An antibacterial and biocompatible piperazine polymer, RSC Adv., 2019, 9, 10135–10147 RSC.
- M. Zhang, G. Zeng, Y. Wang and Z. Zhao, MGF-Ct24E-modified piperazine polymer: A balance of antimicrobial activity and cytotoxicity, J. Appl. Polym. Sci., 2019, 136, 1–8 Search PubMed.
- S. Chattopadhyay, H. Keul and M. Moeller, Synthesis of azetidinium-functionalized polymers using a piperazine based coupler, Macromolecules, 2013, 46, 638–646 CrossRef CAS.
- S. Chattopadhyay, E. Heine, H. Keul and M. Moeller, Azetidinium Functionalized Polytetrahydrofurans: Antimicrobial Properties in Solution and Application to Prepare Non Leaching Antimicrobial Surfaces, Polymers, 2014, 6, 1618–1630 CrossRef.
- S. Chattopadhyay, E. T. Heine, H. Keul and M. Möller, Multifunctional Poly(Vinyl Amine)s Bearing Azetidinium Groups: One Pot Preparation in Water and Antimicrobial Properties, Macromol. Biosci., 2014, 14, 1116–1124 CrossRef CAS.
|
This journal is © The Royal Society of Chemistry 2021 |
Click here to see how this site uses Cookies. View our privacy policy here.