DOI:
10.1039/D0RA10771A
(Paper)
RSC Adv., 2021,
11, 8255-8263
On-line separation/analysis of Rhodamine B dye based on a solid-phase extraction high performance liquid chromatography self-designed device†
Received
23rd December 2020
, Accepted 15th February 2021
First published on 22nd February 2021
Abstract
A special self-designed device based on poly-1-vinyl-3-pentylimidazole hexafluorophosphate (PILs-C5) solid-phase extraction and high performance liquid chromatography (HPLC) is proposed as a novel method for the on-line separation and analysis of Rhodamine B (RhB) dye. Single factor experiment design and orthogonal experiment design were used to optimize the experimental parameters, such as pH, the amount of PILs-C5, sample volume, flow rate, eluent type, eluent concentration, eluent volume, and the flow rate of eluent. Under the optimized conditions, the linear range was 0.02–2.4 μg mL−1, with the correlation coefficients (R2) of 0.997. The limit of detection (LOD) and limit of quantification (LOQ) were 0.004 μg mL−1 and 0.02 μg mL−1, respectively. The extraction capacity was 6.22 mg g−1, and enrichment ratio was 15. The extraction mechanism and the post-treatment method of PILs-C5 were also studied. This method was applied to analyze RhB in a wide variety of real samples with satisfactory results.
Introduction
Rhodamine B (RhB) as a kind of red synthetic dye, has been used as a colorant in plastics, leather, printing, dyeing, paper, textile industries and other industries. The wastewater containing RhB should be analysed and treated carefully.1 It has been regarded as a notable carcinogenic dye which is capable of causing severe genetic disorders, organ damage, anaemia and several other destructive disorders in the human body and poses great challenges to the environment.2 Therefore, it is necessary to develop simpler, faster, cheaper, and more efficient methods to detect and remove RhB.
The detection methods of RhB are as follows: high-performance liquid chromatography (HPLC),3,4 electrochemical detection (EC),5 fluorescence spectrometry (FL),6 and ultraviolet visible spectrometry (UV),7 etc. Because of the low content or complex matrix, separation/enrichment techniques such as liquid–liquid extraction8 and solid-phase extraction (SPE)9,10 are usually carried out before determination. Among them, SPE is the better choice because it has the advantages of less organic reagents, faster speed, and lower cost.11,12
SPE includes off-line13–15 and on-line methods.16–18 The use of on-line techniques has made the development of faster methods possible by reducing the sample preparation time and thus increasing the sample throughput. It can decrease the risk of contamination of the sample or sample extract, eliminate the analyse losses by evaporation or by degradation during sample preconcentration, and improve precision and accuracy. On-line methods are SPE-HPLC,16 SPE-atomic fluorescence spectroscopy (AFS),17 and SPE-UV19 etc. On-line SPE was finished with manually control the injection valve.20,21 However, to the best of our knowledge, there are few reports on on-line analysis RhB with SPE-HPLC.
Poly(ionic liquid)s (PILs) exhibit the characteristics of an ionic liquid on each repetitive monomer,22 and they have numerous potential applications.23–27 In our previous publication, Fe3O4@ionic liquids-β-cyclodextrin cross-linked polymer SPE reported coupled with FL to assay RhB.28 PILs are easily separated and recovered in SPE, which expands the application of ionic liquids in SPE. This manuscript reports the first application of poly-1-vinyl-3-pentylimidazole hexafluorophosphate (PILs-C5) for the extraction of RhB.
In this paper, PILs-C5 was synthesized and characterized. It was used to fill a microcolumn that was linked to a special designed device for the separation/analysis of RhB. The device could be easily used in combine with other analytical instruments, such as UV, FL, EC, and so on. The extraction and elution parameters were optimized by single factor experiment design (SFED) and orthogonal experiment design (OED). The extraction mechanism was studied by Gaussian simulation. From the point of view of green chemistry, the post-treatment method of the PILs was proposed. The optimized method was used to analyze RhB in a variety of foods and daily chemicals with satisfactory results.
Experimental
Instrumentation, reagents and materials
Instrumentation. A 1200 high performance liquid chromatography (Agilent, USA) was used to analyse RhB. A Tensor 27 Fourier transform infrared spectrometer (FTIR) (Bruke, Germany) was used to characterize organic matter. All weight-average molecular weights were determined by a PL-GPC50 gel permeation chromatography system (Agilent, USA). Thermal correlation analyses were performed with a TGA8000/CLARUS thermogravimetric-infrared detector (PerkinElmer, USA) and TG 209 F3 Tarsus thermogravimetric analyzer (Netzsch, Germany). Liquid samples were transported through a 3110 flow injector (Beijing Jitian, China). A DZF vacuum drying oven (Shanghai Kuntian, China) was used to dry products. A DF-101S thermostatic water-bath (Shanghai Licheng, China) was used to control temperature. Deionized water was produced by a Milli-Q ultra-pure water generator (Millipore, USA)Self-designed device: the scheme of the device was displayed in Fig. 1 which included a microcolumn, two peristaltic pumps, a glass tube with lid, a HPLC cup and two valves. Details could be found in the experimental process.
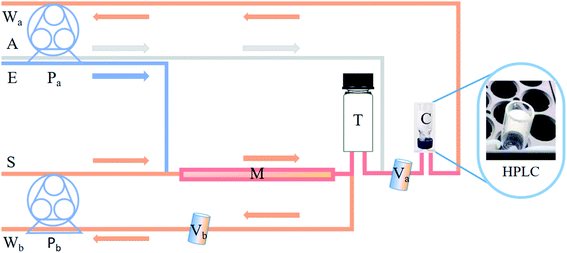 |
| Fig. 1 Self-designed SPE-HPLC system. Air stream (A), HPLC cup (C), eluent stream (E), extraction microcolumn (M), peristaltic pump a (Pa), peristaltic pump b (Pb), sample stream (S), tube (T), valve a (Va), valve b (Vb), liquid waste (Wa), liquid waste (Wb). | |
Reagents and materials. The RhB (>99.0%), Rh6G (>99.0%), Rh123 (>99.0%), sunset yellow (>95.0%), Congo red (>98.0%), fuchsin (>90.0%), neutral red (>90.0%), and 2,2′-azobis-1-vinylimidazole (>99.0%) were obtained from Shanghai Aladdin Bio-Chem Technology Co., Ltd (China). The RhB standard (DC16813550) was purchased from national standard network. All other chemicals and reagents (methyl-n-butyl-bromide, n-amyl-bromide, n-hexane bromide alcohol, ethyl ethanol, acetone, ethyl acetate, hydrochloric acid, sodium hydroxide, benzene, acetonitrile, chloroform, n-hexane, ethyl ether, potassium hexafluorophosphate, sodium chloride, magnesium sulfate, zinc chloride, iron sulfate) were of analytical grade and purchased from Sinopharm Chemical Reagent Co., Ltd (China). Aqueous solutions were prepared by using ultra-pure water obtained from a Milli-Q ultra-pure water generator.
Experimental method
Synthesis of PILs-C5
The synthesis of PILs-C5 was as follows. 9.44 g (0.1 mol) 1-vinylimidazole were added into a three-necked flask (250 mL), followed by the addition of 15.2 g (0.1 mol) n-amyl bromide under agitation. The mixture was stirred for 120 min at 70 °C in a water bath until the formation of the viscous and yellowish 1-vinyl-3-pentylimidazole bromide ([VPIM]Br). The obtained product was washed three times by ethyl acetate and dried in a vacuum drying oven at 65 °C. Then 100 mL water and 18.8 g (0.1 mol) KPF6 were added into the [VPIM]Br, and the mixture was stirred at room temperature for 5 h. The ionic liquid phase was separated and washed with water (10 mL) three times. Then, it was baked at 100 °C for 4 h to obtain yellowish viscous ionic liquid 1-vinyl-3-pentylimidazolium hexafluorophosphate (ILs-C5).
Proper amount of ILs-C5 and acetone was mixed in a 100 mL three-neck flask. Appropriate amount of 2,2′-azobis was added slowly under nitrogen protection and agitation. The reaction was kept at 80 °C for 1 h, yielding a yellow brittle solid. The solid was ground, filtered through 80 mesh screen, washed with deionized water three times, and stored after drying.
Experimental process
As shown in Fig. 1, the tube (T) and HPLC cup (C) were both specially designed with two holes opened on the opposite of the bottom, so solution could be flowed through T into C. A proper amount of PILs was added to a tetrafluoroethylene microcolumn (length 6 cm, inner diameter 0.35 cm). Sample stream (S), elution stream (E), air stream (A) and 2 peristaltic pumps (Pa, Pb) in parallel, run by a program, were used to control the extraction and elution of samples.
Extraction: turn off Pa and Va and let the sample flow into the M from the S, and out from the Vb. Elution: only turn on Pa, and then let the elution flow into T from E. At the same time, A was pumped into T to mix the eluent evenly by bubbling. Determination: T was capped, the eluent flowed into C and was taken by HPLC automatic sampler to be analysed at 554 nm.
Sample preparation
Shampoo: 10.0 g of red shampoo was dissolved in 30.0 mL water. The mixture was transferred to a 50 mL bottle, volume it to scale with deionized water, and shook it evenly.
Red pencil core: 20.0 mg crushed red pencil core was mixed with 5.0 mL n-hexane and extracted by ultrasonication for 5 min. After filtration, 5.0 mL n-hexane was added to the filtration slag, followed by ultrasonic extraction for 5 min. The two filtrates were combined together and was evaporated using a vacuum drying oven at 40 °C to remove n-hexane residual. The extracted RhB was dissolved with 10.0 mL distilled water. Finally, the solution was transferred to a 50 mL bottle, diluted with deionized water to the calibration line, and shook thoroughly.
Match head: 30 mg of the crushed red match head was took, and was treated as those of the pencil core treatment.
Eye shadow: 200.0 mg of eye shadow was mixed with 5 mL n-hexane and extracted by ultrasonication for 5 min. After filtration, 5 mL n-hexane was added to the filtration slag, followed by ultrasonic extraction for 5 min. Then, two times of filtrate were merged together. n-Hexane was evaporated by a vacuum drying oven at 40 °C, and the extracted RhB was dissolved with 5 mL deionized water. Finally, the solution was transferred into 10 mL capacity bottle, diluted with deionized water to the calibration line, shook well, and set aside.
Lipstick: 500.0 mg Lipstick was mixed with 5 mL carbon tetrachloride and extracted by ultrasonication for 5 min. After filtration, 5 mL carbon tetrachloride was added to the filtration slag, followed by ultrasonic extraction for 5 min. Then, two times of filtrate were merged together. Carbon tetrachloride was evaporated by a vacuum drying oven at 40 °C, and the extracted RhB was dissolved with 5 mL deionized water. Finally, the solution was transferred into 10 mL capacity bottle, diluted with deionized water to the calibration line, shook well, and set aside.
Chili powder: Take the crushed samples 1.00 g, the other treatment procedures were the same as those of eye shadow.
Lao Gan Ma spice sauce: Take the crushed samples 1.00 g, the other treatment procedures were the same as those of eye shadow.
Chromatographic conditions
All the measurements were analyzed by an HPLC with a UV detector at 554 nm and a injector with a 10.0 μL loop. Chromatography was performed C18 reversed-phase column (150 mm × 4.6 mm I.D., 5 μm, Shimadzu Corporation, Japan) at 35 °C. The mobile phase was consisted of methanol and water (70
:
30, v/v). The flow rate was set at 1.0 mL min−1.
Results and discussion
Optimization of PILs synthesis
The monomer, ILs-C5, is a yellowish viscous liquid at room temperature. After polymerization, it is transformed into a yellowish solid. Fig. 2 shows the dw/dlog
M curve of gel chromatography (GPC) analysis of PILs-C5. It shows that the molecular weight (MW) of the PILs-C5 is mainly distributed in the range of 8000–15
500. Therefore, the formation of PILs-C5 was confirmed.
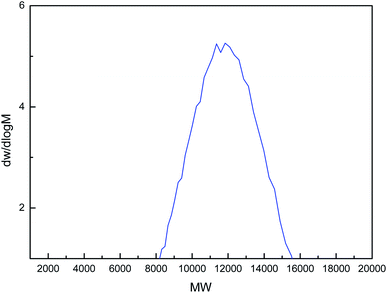 |
| Fig. 2 GPC analysis of PILs-C5. | |
The side chain length of the ionic liquid monomer and the amount of initiator greatly influence the extraction performance of PILs. So, taking the extraction ratio (ER) of RhB as an index, the synthesis of PILs was optimized. The results were presented in Fig. 3. Fig. 3 showed that the highest ER was obtained when the side chain length of the ionic liquid monomer was 5 (C5) (Fig. 3a) and when Rm (the molar ratio of initiator and monomer) was 3.0
:
20 (Fig. 3b).Therefore, PILs-C5 and Rm 3.0
:
20 were selected for the following experiment.
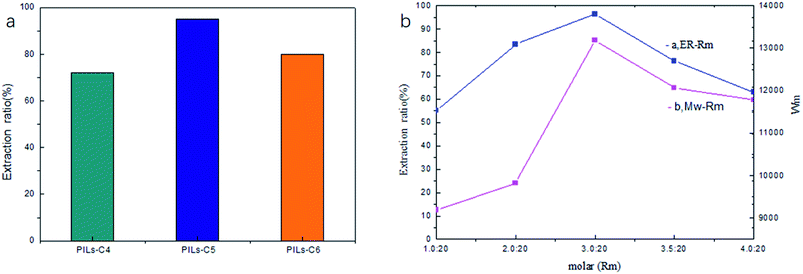 |
| Fig. 3 Optimization of PILs synthesis. | |
Optimization of extraction conditions
In the experiment, the parameters were optimized by SFED first, and then by OED on the basis of SFED optimization.
Optimization of extraction parameters by SFED. SFED means that although there are many factors affecting the index, under the assumption that there is no interaction between these factors, change only one of the factors, and the other conditions remain unchanged.The optimization of pH, amount of PILs-C5, RhB volume, and pump speed using SFED are shown in Fig. 4. The optimum extraction conditions are as follows: pH 4.0, 0.30 g PILs-C5, 30 mL sample volume, and pump speed of 30 rpm. Under the optimized conditions, the ER was greater than 90%.
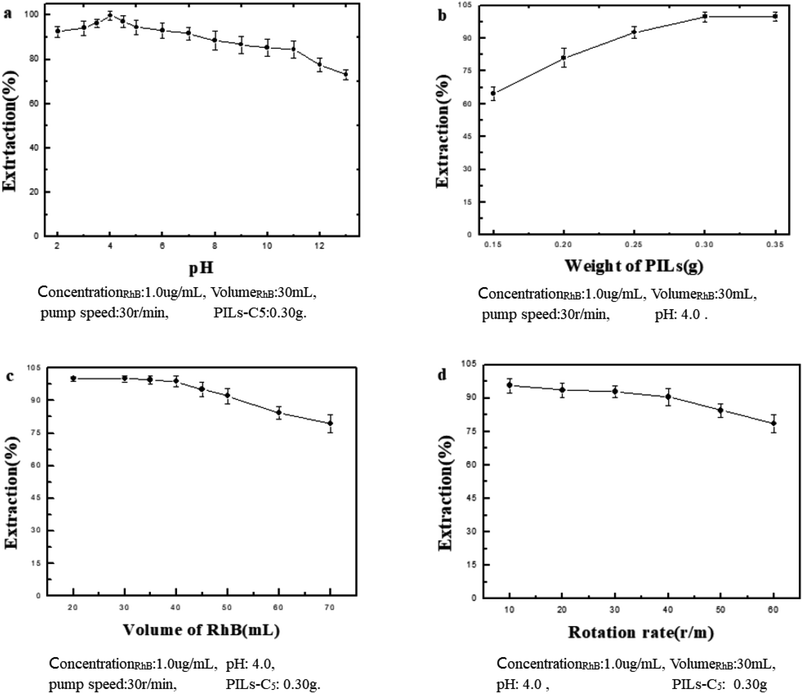 |
| Fig. 4 Optimization of extraction parameters by SFED. | |
Optimization of extraction parameters by OED. It is simple to use the SFED method when optimizing the experimental conditions. However, incorrect results may be obtained if there is a cross-effect between different factors. OED was utilized for further optimization. OED is commonly employed in studying behaviors of parameters, which involve multivariate and multilevel factors. Based on orthogonality, representative samples of test points were selected from comprehensive test variables. These representative test points were uniformly dispersed and neatly comparable. This statistical analysis technique has been demonstrated to be a highly efficient, accurate, fast, and economical in experimental design methods.29–32 In general, if the optimization factors do not influence each other, the results of SFED and OED may be consistent with each other.In this paper, on the basis of the results obtained by SFED, factors such as pH, amount of PILs-C5, RhB volume, and pump speed were optimized by OED, and the details are shown in Table 1.
Table 1 Optimization of extraction parameters by OED
Level |
pH |
Pump speed (rpm) |
Volume of RhB (mL) |
Amount of PILs-C5 (g) |
Empty column |
ER (%) |
1 |
3.0 |
10 |
30 |
0.20 |
1 |
79.8 |
2 |
3.0 |
20 |
40 |
0.25 |
2 |
83.2 |
3 |
3.0 |
30 |
50 |
0.30 |
3 |
85.9 |
4 |
3.0 |
40 |
60 |
0.35 |
4 |
92.5 |
5 |
3.5 |
10 |
40 |
0.30 |
4 |
94.7 |
6 |
3.5 |
20 |
30 |
0.35 |
3 |
96.3 |
7 |
3.5 |
30 |
60 |
0.20 |
2 |
60.4 |
8 |
3.5 |
40 |
50 |
0.25 |
1 |
73.6 |
9 |
4.0 |
10 |
50 |
0.35 |
2 |
92.4 |
10 |
4.0 |
20 |
60 |
0.30 |
1 |
86.8 |
11 |
4.0 |
30 |
30 |
0.25 |
4 |
91.4 |
12 |
4.0 |
40 |
40 |
0.20 |
3 |
72.5 |
13 |
4.5 |
10 |
60 |
0.25 |
3 |
79.7 |
14 |
4.5 |
20 |
50 |
0.20 |
4 |
70.8 |
15 |
4.5 |
30 |
40 |
0.35 |
1 |
99.3 |
16 |
4.5 |
40 |
30 |
0.30 |
2 |
92.5 |
K1 |
85.350 |
86.650 |
90.000 |
70.875 |
84.725 |
— |
K2 |
81.250 |
84.275 |
87.275 |
81.975 |
82.125 |
— |
K3 |
85.775 |
84.100 |
80.675 |
89.975 |
83.600 |
— |
K4 |
85.425 |
82.775 |
79.850 |
95.125 |
87.350 |
— |
Range |
4.525 |
3.875 |
10.150 |
24.250 |
5.225 |
— |
The optimum conditions obtained by OED are as follows: pH 4.0, 0.35 g PILs-C5, 30 mL sample volume, and pump speed of 10 rpm. Compared with the SFED results, the pump speeds are different (30 rpm (SFED) and 10 rpm (OED)). From Table 1, the order of factors affecting ER is as follows: amount of PILs-C5 > volume of RhB > pH > pump speed. Thus, the pump speed has the least effect on ER. Although reducing the pump speed is beneficial to improve ER, it has little effect on the increase of ER. Therefore, to decrease analysis time, the pump speed of 30 rpm was selected.
Optimization of elution conditions
Selection of eluent types. In this experiment, methanol, ethanol, acetone, benzene, hydrochloric acid, and sodium hydroxide were used to elute the extracted RhB. It was found that hydrochloric acid, sodium hydroxide, and benzene could not elute RhB. Acetone could elute RhB, but it damaged PILs-C5 and affected the reusability of the material. The elution effect of methanol was the same as ethanol. In consideration of green chemistry methods, ethanol was chosen as the eluent.
Optimization of elution parameters by SFED. The optimization of the elution parameters with SFED is shown in Fig. 5. The optimized elution is as follows: eluent concentration 35%, volume of eluent 2.0 mL, and rotation of pump 40 rpm.
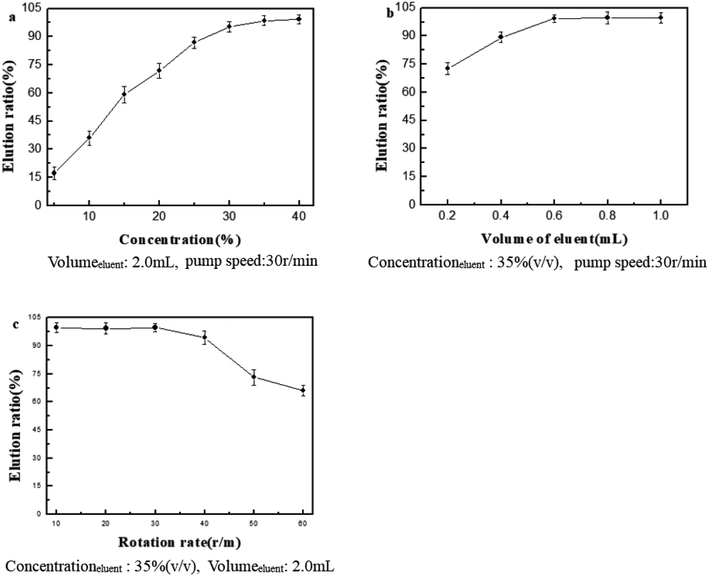 |
| Fig. 5 Optimization of elution parameters by SFED. | |
Optimization of elution parameters by OED. Similar to the optimization of extraction parameters, factors such as eluent concentration, volume of eluent, and rotation of the pump were optimized by OED, and the elution condition was consistent with the optimal experimental scheme obtained by the SFED. The results showed that there was no cross-effect among these factors. Under the optimized conditions, the elution rate was reached to 99.4%.
Reusability of PILs-C5
The reusability of PILs-C5 was studied. The experimental results are shown as Fig. 6.
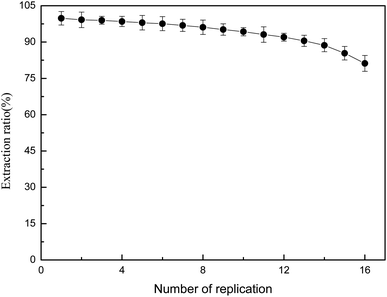 |
| Fig. 6 Reusability of PILs-C5. | |
Fig. 6 shows that the ER was greater than 85% after 15 consecutive uses, indicating PILs-C5 had good reusability.
Effect of interferences
Under the condition that the relative error is not more than ±5%, the potential interference of Rhodamine 6G, Rhodamine 123, and other dyes was studied. The results in Table 2 show that when the concentration of these dyes were 100 times that of RhB, there was no interference in the extraction and detection of RhB.
Table 2 The effects of potential interference from other dyes
Foreign substances |
Tolerance limits (interferences/RhB) |
Foreign substances |
Tolerance limits (interferences/RhB) |
Rh6G |
100 |
SO42− |
500 |
Rh123 |
100 |
Na+ |
1000 |
Sunset yellow |
100 |
K+ |
1000 |
Neutral red |
100 |
Cu2+ |
1000 |
Fuchsin |
100 |
Mg2+ |
1000 |
Congo red |
100 |
Zn2+ |
1000 |
Cl− |
500 |
Fe3+ |
1000 |
Analytical figures of merit
The proposed method was evaluated with particular focus on linear range, detection limit, extraction capacity, and enrichment ratio. The experimental results showed that under the optimized conditions, the linear range was 0.02–2.4 μg mL−1, and the correlation coefficients (R2) was 0.997. The LOD was 0.004 μg mL−1 (signal-to-noise ratio (S/N) was 3) and LOQ was 0.02 μg mL−1 (S/N was 10), respectively. The extraction capacity was 6.22 mg g−1, and the enrichment ratio is 15.
The analysis of real samples
Under the optimal experimental conditions, the chromatograms of the lipstick samples before (curve e) and after (curve c) SPE were measured (Fig. 7). It could be found that (1) the retention time of RhB was about 7.2 min (curve a); (2) the peak in the real samples were significantly enhanced after the SPE (curve b and c); (3) the peak at 9.2 min of curve c disappeared after extraction. The results of chromatographic experiments show that PILs-C5 has strong enrichment ability and good selectivity.
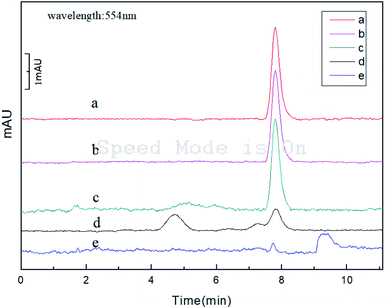 |
| Fig. 7 Analysis of real samples. (a) RhB (0.6 μg mL−1); (b) shampoo (after extraction); (c) lipstick (after extraction); (d) shampoo (before extraction); (e) lipstick (before extraction). | |
Under the optimized conditions, this method was applied to the detection of RhB in a variety of practical samples, such as foods and daily chemical products using the standard addition method. The results were shown in Table 3. It was found that the recovery was between 91.0% and 110.0%.
Table 3 Determination of RhB in real samples
Sample |
RhB added (μg L−1) |
RhB found ± SD (μg L−1) |
Recovery (%) |
RSD (%) |
RhB standard |
0 |
0.81 ± 0.01 |
— |
1.3 |
1.00 |
1.83 ± 0.03 |
103.0 |
2.2 |
2.00 |
2.92 ± 0.03 |
105.5 |
1.8 |
3.00 |
3.77 ± 0.07 |
98.0 |
1.9 |
Lipstick |
0.00 |
0.22 ± 0.01 |
— |
4.5 |
0.20 |
0.44 ± 0.02 |
110.0 |
5.1 |
0.40 |
0.60 ± 0.02 |
95.0 |
3.8 |
0.60 |
0.81 ± 0.03 |
98.3 |
4.1 |
Shampoo |
0.00 |
2.78 ± 0.09 |
— |
3.3 |
2.00 |
4.79 ± 0.19 |
100.5 |
4.2 |
3.00 |
5.84 ± 0.22 |
102.0 |
3.7 |
5.00 |
7.92 ± 0.34 |
102.8 |
4.6 |
Red pencil core |
0.00 |
2.73 ± 0.07 |
— |
2.5 |
2.00 |
4.58 ± 0.15 |
92.5 |
3.2 |
3.00 |
5.80 ± 0.31 |
102.3 |
5.3 |
5.00 |
7.85 ± 0.23 |
102.4 |
2.8 |
Match head |
0.00 |
1.81 ± 0.08 |
— |
4.4 |
2.00 |
3.63 ± 0.15 |
91.0 |
4.2 |
3.00 |
4.92 ± 0.15 |
103.7 |
3.0 |
5.00 |
6.70 ± 0.18 |
97.8 |
2.7 |
Eye shadow |
0.00 |
0.25 ± 0.01 |
— |
4.7 |
2.00 |
2.19 ± 0.09 |
97.0 |
4.1 |
3.00 |
3.22 ± 0.11 |
99.0 |
3.3 |
5.00 |
5.23 ± 0.16 |
99.6 |
3.0 |
Chili powder |
0.00 |
0.00 |
— |
— |
2.00 |
1.95 ± 0.09 |
97.5 |
4.7 |
3.00 |
3.10 ± 0.13 |
103.3 |
4.3 |
5.00 |
5.17 ± 0.27 |
103.4 |
5.4 |
Lao Gan Ma spice sauce |
0.00 |
0.00 |
— |
— |
2.00 |
2.08 ± 0.14 |
104.0 |
5.3 |
3.00 |
2.97 ± 0.12 |
99.0 |
3.8 |
5.00 |
4.85 ± 0.20 |
97.0 |
4.1 |
Comparison of the present method with others
Table 4 lists the comparison of this method with those reported in the literature. It can be seen that the recovery ratio and linear range of this method are better than or on the same order of magnitude of the references. It is necessary to point out that this method achieves automated on-line separation and analysis in all processes with less than 3 minutes determination time. All in all, this method has the advantages of simple operation process, short analysis time, and labor-saving.
Table 4 Comparison of the present method with other reported methodsa
Method |
LR (μg L−1) |
LOD (μg L−1) |
Recovery (%) |
Reference |
LR: line range; LOD: limit of detection. |
SPE-UV |
250–3000 |
3.14 |
96.0–118.0 |
10 |
SPE-HPLC |
100–8000 |
3.4 |
78.47–101.6 |
33 |
SPE-HPLC |
200–5000 |
107 |
81.9–97.5 |
34 |
MSPE-FL |
100–9000 |
5.2 |
97.0–100.7 |
28 |
SPE-HPLC |
20–2400 |
4.0 |
91.0–110.0 |
Present work |
Mechanism discussion
During extraction optimization, it was found that the ER in acidic environment was significantly higher than in alkaline environment, and the highest ER was found at pH 4.0 (see Fig. 4a). This was due to the protonation of PILs-C5 under acidic conditions. A hydrogen bond is formed with two oxygen atoms of carboxylic acid on RhB. This leads to an increase in ER in acidic environment.
The conclusions obtained by Gaussian simulation show that hydrogen (on PILs-C5) and oxygen (on RhB) may form hydrogen bonds. Fig. 8 shows the calculated bond lengths are O1–H2: 2.136 Å, O1–H3: 2.703 Å, and O2–H1: 2.164. Since these bond lengths are less than 3 Å, it proves that two oxygen atoms on RhB have formed hydrogen bonds with hydrogen on PILs-C5.
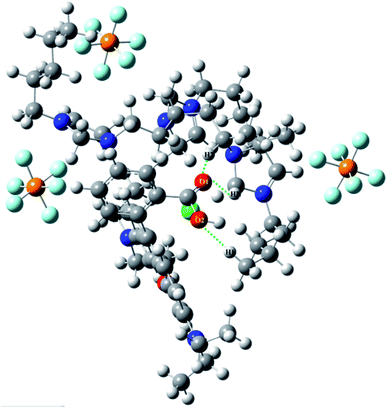 |
| Fig. 8 Gaussian simulation. | |
The post-treatment of PILs-C5
In the study of PILs, it is rarely shown how to treat used PILs. However, PILs may bring harm to the environment if discarded casually. Therefore, the post-treatment of PILs is a problem worthy of consideration. In order to explore the post-treatment of PILs-C5 synthesized in this paper, proper amounts of PILs-C5 and PILs-C5 extracted from RhB were transferred to a platinum crucible. After slowly heating to 800 °C and maintaining for 10 min, PILs-C5 had decomposed completely, and there was no residue in the crucible. The thermal decomposition was analyzed by TG-FTIR at 10 °C min−1, and the infrared stacking curve (Fig. 9) showed that a number of gaseous products were produced. According to the molecular structure and comparison with an infrared analysis spectrum database, these gaseous substances were identified as hydrogen fluoride (peak a), phosphorus pentafluoride (peak d, g), phosphorous trifluoride (peak g), fluorohydrocarbon (peak b, e, f), nitrile (d), and carbon dioxide (peak c).35–37 The TG diagram (Fig. S2†) also confirms the complete decomposition of PILs-C5 at temperatures higher than 800 °C. Therefore, the PILs-C5 used in this study can be post treated by burning. The waste gas from combustion can be washed by lye and organic solvent.
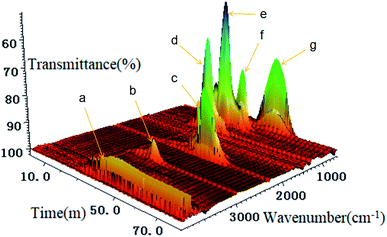 |
| Fig. 9 TG-FTIR analysis of PILs-C5. | |
Conclusion
In this work, PILs-C5 with the advantages of excellent selectivity and simple separation/enrichment was synthesized and characterized. The polymer was used as a solid-phase extraction material in a novel self-designed device for the automated on-line determination of trace RhB. The method has the advantages of high sensitivity, low cost, simple operation, and minimal interference. It can be applied to the analysis of RhB in real samples. The PILs, used in the experiment, could be treated by burn, realizing the post-treatment. This novel and small device could be easily used in combination with other analytical instruments, such as HPLC, UV, FL, EC, and so on. It provides a simple way for the on-line sample pretreatment and analysis.
Conflicts of interest
There are no conflicts to declare.
Acknowledgements
The authors acknowledge the financial support from the National Natural Science Foundation of China (21375117) and a project funded by the Priority Academic Program Development of Jiangsu Higher Education Institutions and Postgraduate Research/Practice Innovation Program of Jiangsu province.
References
- X. Y. Zheng, H. L. Zheng, Z. K. Xiong, R. Zhao, Y. Z. Liu, C. Zhao and C. F Zheng, Chem. Eng. J., 2019, 392, 123706 CrossRef.
- G. J. Janet, P. K. Senthil, M. Govarthanan, P. N. Tsopbou and A. Abilarasu, Environ. Pollut., 2021, 269, 116173 CrossRef.
- M. Arabi, A. Ostovan, A. R. Bagheri, X. T. Guo, J. H. Li, J. P. Ma and L. X. Chen, Talanta, 2020, 215, 120933 CrossRef CAS.
- M. Arabi, A. Ostovan, A. R. Bagheri, X. T. Guo, L. Y. Wang, J. H. Li, X. Y. Wang, B. W. Li and L. X. Chen, Trends Anal. Chem., 2020, 128, 115923 CrossRef CAS.
- X. L. Zhu, G. L. Wu, C. Z. Wang, D. M. Zhang and X. Yuan, Measurement, 2018, 120, 206–212 CrossRef.
- Y. Y. Chang, H. L. Wu, H. Fang, T. Wang, Z. Liu, Y. Z. Ouyang, Y. J. Ding and R. Q. Yu, Spectrochim. Acta, Part A, 2018, 204, 141–149 CrossRef CAS.
- E. Ghasemi and M. Kaykhaii, Spectrochim. Acta, Part A, 2016, 164, 93–97 CrossRef CAS.
- N. Xiao, J. Deng, K. H. Huang, S. Q. Ju, C. H. Hu and J. Liang, Spectrochim. Acta, Part A, 2014, 128, 312–318 CrossRef CAS.
- A. R. Bagheri and M. Ghaedi, Int. J. Biol. Macromol., 2019, 139, 40–48 CrossRef CAS.
- T. Tomića, S. Babić, M. Biošić, N. U Nasipak and A. M. Čižmek, Dyes Pigments, 2018, 150, 216–222 CrossRef.
- L. L. Xu, H. B. Suo, R. M. Liu, H. M. Liu and H. D. Qiu, RSC Adv., 2019, 9, 30125–30133 RSC.
- A. A. Dudkina, T. N. Volgina, N. V. Saranchina, N. A. Gavrilenko and M. A. Gavrilenko, Talanta, 2019, 202, 186–189 CrossRef CAS.
- K. Madej, U. S. Garmendia, A. Weber and W. Piekoszewski, J. Liq. Chromatogr. Relat. Technol., 2016, 39, 128–133 CrossRef CAS.
- D. F. Zhang, L. Zhang and T. Liu, Analyst, 2018, 143, 3100–3106 RSC.
- M. X. Zhang, J. H. Yang, X. X. Geng, Y. Li, Z. X. Zha, S. H. Cu and J. Yang, J. Chromatogr. A, 2019, 1598, 20–29 CrossRef CAS.
- K. Fikarová, B. Horstkotte, D. Machián, H. Sklenářová and P. Solich, Talanta, 2021, 221, 121427 CrossRef.
- Q. Tan, Y. Pan, L. Liu, S. B. Shu and Y. Liu, Microchem. J., 2019, 144, 495–499 CrossRef CAS.
- H. J. Gan and H. Xu, Anal. Chim. Acta, 2018, 1008, 48–56 CrossRef CAS.
- V. Abrahamsson, F. Jumaah and C. Turner, J. Supercrit. Fluids, 2018, 131, 157–165 CrossRef CAS.
- J. H. Xiao, B. C. Ni, Y. Tao and J. B. Wang, J. Chromatogr., B, 2020, 1158, 122342 CrossRef CAS.
- M. Lohse, S. R. G. A. Blaser, D. Vetterlein, S. Schlueter, E. Oburger, T. Reemtsma and O. J. Lechtenfeld, Anal. Chem., 2020, 92, 10442–10449 CrossRef CAS.
- J. Y. Yuan, D. Mecerreyes and M. Antonietti, Prog. Polym. Sci., 2013, 38, 1009–1036 CrossRef CAS.
- S. F. Cheng, B. H. Chen, L. Qin and Y. Y. Zhang, RSC Adv., 2019, 9, 8137–8145 RSC.
- F. X. Liu, S. Wang, H. Chen, J. S. Li, X. Wang, T. J. Mao and Z. Wang, Renewable Energy, 2021, 163, 1692–1700 CrossRef CAS.
- E. M. Maya, E. Verde-Sesto, M. Daniele, I. Marta and M. David, Eur. Polym. J., 2019, 110, 107–113 CrossRef CAS.
- B. Y. Yin, W. Q. Xu, C. J. Liu, M. Q. Kong, Y. D. Lv, Y. J. Huang, Q. Yang and G. X. Li, RSC Adv., 2020, 10, 2085–2095 RSC.
- P. Maksym, M. Tarnacka, R. Bielas, B. Hachuła, A. Zajac, A. Szpecht, M. Smiglak, K. Kaminski and M. Paluch, Polymer, 2020, 192, 122262 CrossRef.
- A. A. Ahmed Bakheet and X. S. Zhu, J. Fluoresc., 2017, 27, 1087–1094 CrossRef CAS.
- S. Hinislioglu and O. U. Bayrak, Civ. Eng. Environ. Syst., 2004, 21, 79–90 CrossRef.
- Y. Zhou, P. Feng, P. J. Li, H. L. Hu and C. J. Shuai, Adv. Electr. Electron. Eng., 2011, 2, 657–661 Search PubMed.
- A. R. Bagheri, M. Arabi, M. Ghaedi, A. Ostovan, X. Y. Wang, J. H. Li and L. X. Chen, Talanta, 2019, 195, 390–400 CrossRef CAS.
- M. Ghaedi, M. Arabi, Q. Yang, J. Li and L. Chen, ACS Appl. Mater. Interfaces, 2018, 10, 4140–4150 CrossRef.
- X. M. Su, X. Y. Li, J. J. Li, M. Liu, F. H. Lei, X. C. Tan, P. F. Li and W. Q. Luo, Food Chem., 2015, 171, 292–297 CrossRef CAS.
- S. Dixit, S. K. Khanna and M. Das, J. AOAC Int., 2011, 94, 1874–1881 CrossRef CAS.
- M. Golets, M. R. Shimpi, Y. L. Wang, O. N. Antzutkin, S. Glavatskih and A. Laaksonen, Phys. Chem. Chem. Phys., 2016, 18, 22458–22466 RSC.
- W. Al-Sallami, P. Parsaeian and A. Neville, Lubric. Sci., 2019, 31, 229–238 CrossRef CAS.
- S. D. Chambreau, J. A. Boatz, G. L. Vaghjiani, C. Koh, O. Kostko, A. Golan and S. R. Leone, J. Phys. Chem. A, 2012, 116, 5867–5876 CrossRef CAS.
Footnote |
† Electronic supplementary information (ESI) available. See DOI: 10.1039/d0ra10771a |
|
This journal is © The Royal Society of Chemistry 2021 |
Click here to see how this site uses Cookies. View our privacy policy here.