DOI:
10.1039/D0RA10766B
(Paper)
RSC Adv., 2021,
11, 10922-10928
Preparation of N/S doped carbon dots and their application in nitrite detection†
Received
23rd December 2020
, Accepted 5th March 2021
First published on 15th March 2021
Abstract
Detection of carcinogens is generally recognized to be meaningful, especially for nitrites (NO2−). Here blue-green fluorescent carbon dots (CDs) were successfully synthesized by using p-aminobenzenesulfonic acid, and their surfaces were identified to be abundant in the functional groups of amino, hydroxyl, and sulfuric acid. Importantly, the sulfuric acid group and aromatic primary ammonia groups on the surfaces of CDs showed the interactions with the nitrites to cause fluorescence quenching. The novel CDs showed high sensitivity and selectivity for NO2− detection with a low detection limit of 0.03 mM in water due to the fluorescence quenching effect of the CDs. Consequently, the proposed CDs here may provide a new way of monitoring NO2− in the target samples.
Introduction
Carbon dots (CDs) have attracted more and more attention due to their excellent optical properties, and have been widely applied and developed in the fields of cell imaging,1 photoelectric devices,2 drug delivery,3 analysis and detection,4 and fluorescent ink.5 Accordingly, multifarious synthetic methods, such as hydrothermal or solvothermal,6,7 microwave-assisted,8 electrochemical,9 and laser ablation,10 have been proposed to prepare fluorescent CDs. In addition to a variety of preparation methods, the raw materials used to prepare CDs are becoming more and more diversified. Many research groups use hydrothermal or solvo-thermal methods to explore green, non-toxic and environmentally friendly carbon sources. In addition to the classic citric acid series, other biomass materials are also used to prepare CDs. At present, proteins,11 chitosan,12 fruits and vegetables and biological derivatives13 have all become carbon sources for the preparation of CDs, and these CDs generally have good biocompatibility and low preparation cost. The types of effective sensing sites on the surface of non-functionalized CDs are limited. Therefore, to obtain CDs with excellent performance, functionalized CDs are often considered. The functionalization of CDs can be divided into doping and surface modification. Doping can not only realize the optical modification of CDs but also form action sites on the surface of CDs for sensing. Surface modification can expand the selectivity and improve the sensitivity of CDs. Under their superior fluorescence and various effective sensing sites on its surface, diverse CDs have been accordingly utilized to sensing the targets towards various purposes, mainly including ion detection, food sub-detection, pesticide contamination detection, etc.
Nitrite, as a natural component of the nitrogen cycle in the ecosystem, widely exists in natural water bodies and is a key indicator to determine whether natural water bodies are polluted. It will cause direct or indirect harm to human bodies, animals and plants, and is a potentially dangerous substance in the environment. High intake of nitrite in the human body can cause methemoglobin disease, leading to hypoxia of human tissues.14 Besides, nitrite will be converted into nitrosamines under the action of gastric acid and other factors, and nitrosamines have strong carcinogenicity, will cause harm to the human body, infants and children are particularly sensitive to nitrite.15 Nitrate and nitrite are often found in some food systems such as pickles, dairy products, and specially formulated foods. Nitrate can be converted to nitrite by nitrifying bacteria, so they are at risk of ingestion. Hence, the World Health Organization and US Environmental Protection Agency recommend the limits of NO2− as being below 3 mg L−1 and 1 mg L−1 for drinking water.16 Likewise, monitoring the allowable level of nitrite in both food and the environment is of great importance. There are many methods to detect nitrite: high performance liquid chromatography,17 ion chromatography,18 spectrophotometry,19 colorimetry20 and capillary electrophoresis21 and more. Among these, fluorescence-based nanosensors exhibit the advantages of simplicity, sensitivity, and high efficiency. As been reported, the hybrid system UiO-66-NH2-Cit is achieved by the covalently post-synthetic modification of UiO-66-NH2 with citric acid through their condensation reaction. It can be utilized as a probe to detect NO2− by its luminescence quenching.22 Also, citric acid and 3-aminoboric acid as the precursors were utilized to synthesize carbon dots through the hydrothermal way for detecting nitrite.23 Therefore, it is very significant to prepare CDs as NO2− detection probes facilely and controllable for food safety, pollution detection, and other applications.
Herein, sulfanilic acid was originally utilized as the precursors to synthesize fluorescent CDs through a one-step solvothermal method,24 and their fluorescence of CDs showed the response to varied pH values. Significantly, the as-prepared CDs were proved to be equipped with the functional groups of –NH2, –COOH and –HSO3, especially aromatic primary, and the fluorescence quenching of CDs is the result of the comprehensive action of various factors. In particular, adding nitrite solution into CDs caused their fluorescence quenching due to the static quenching effect. At the same time, the CDs are combined with methylcellulose to prepare a fluorescent film, which still has fluorescence after redissolved in water and can be quenched by sodium nitrous acid, which can be used for portable sensors.
Experimental section
Materials
All experimental materials were purchased from commercial suppliers and used without further purification; all experiments were performed in deionized water. The reagents used in the synthesis were obtained from Sinopharm Chemical Reagent Co., Ltd. Methylcellulose, p-aminobenzenesulfonic acid, dimethylformamide, tetrahydrofuran, acetone, dimethyl sulfoxide, methanol, ethanol, dichloromethane, isopropanol and ethyl acetate are all analytical reagents. Complex anion solutions (0.01 mol L−1) are prepared with various salts ((NH4)2S2O8, NaHCO3, K2CO3, Na2HPO4, NaH2PO4, Na2SO4, NaNO3, NaNO2, NaCl, CH3COONH4, K2SO3) and deionized water. pH buffer solution (citrate phosphate buffer concentrate) was used to adjust the pH of the solution.
Preparation of N, S-CDs
CDs were synthesized from p-aminobenzenesulfonic acid by a one-step solvothermal method (Fig. 1). In brief, 0.6 g of p-aminobenzenesulfonic acid and 25 ml of deionized water and ethanol were treated by stirring by magnetic force at 60 °C for 1 hour. Then, the entire reaction mixture was transferred into a Teflon-lined stainless steel autoclave and kept in the oven at 200 °C for 4 hours. Then cooled to room temperature and diluted for later use.
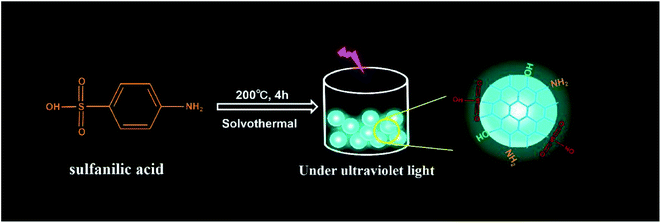 |
| Fig. 1 Scheme of synthesizing CDs. | |
Preparation of water-soluble fluorescent films
The methylcellulose powder and CDs solution were stirred for more than 12 hours (the volume mass fraction of methylcellulose was 3%) to obtain the methylcellulose/CDs colloid, which was dried at 60 °C to obtain the fluorescent film.
Characterization
To study morphology and the average size of the synthesized N, S-CDs, transmission electron microscopy (TEM) analysis was conducted (TEM, JEM-2100 (HR)) at an accelerating voltage of 200 kV. UV-visible spectra were recorded on a UV-3600 spectrophotometer. The luminescence spectra were recorded on a Hitachi F-4600 5J2-0004 spectrophotometer (both excitation and emission slit widths were 5 nm). Similarly, ESCALAB 250 X-ray photoelectron spectrometer (XPS) and Fourier transform infrared (FTIR) spectrometer (Nicolet 5700) were utilized to analyze the elements and functional groups. REX pH S-3E digital pH meter (Shanghai, China) for measuring the pH values of various solutions. Besides, we introduced the Thermal analyzer (TGA2/DSC3) to study the thermal stability of CDs. DF-101S thermostat water bath is used for stirring and keeping warm. Ultraviolet lamps are used to irradiate CDs.
Results and discussion
Characterization of CDs
To identify the as-synthesized CDs, we first acquired their fluorescence spectra. Briefly, the maximum emission of CDs under 400 nm excitation is about 480 nm (Fig. 2A), and the blue-green fluorescence of CDs with UV light was observed as well as being transparent under daylight (Fig. 2A, photograph in the upper right corner). Simultaneously, there appeared an obvious peak of around 240 nm for UV-vis spectrum of CDs (Fig. 2A), which is attributed to the carbon core itself, mainly due to the sp2 hybrid aromatic π–π* system electronic transition.25 Meanwhile, within the excitation range of 360 nm to 420 nm, the fluorescence intensity showed obvious changes, but the emission wavelength was basically unchanged, which was around 480 nm, indicating that their fluorescence emission was not affected by the excitation wavelength (Fig. 2B). And also found that the strongest emission wavelength can be obtained when the excitation wavelength is about 400 nm. Furthermore, the morphology and structure of as-synthesized N, S-CDs were investigated via transmission electron microscopy (TEM) analysis; the results revealed the spherical morphology and have good dispersion (Fig. 2C), and its size is mainly distributed at about 1 nm (Fig. 2D).
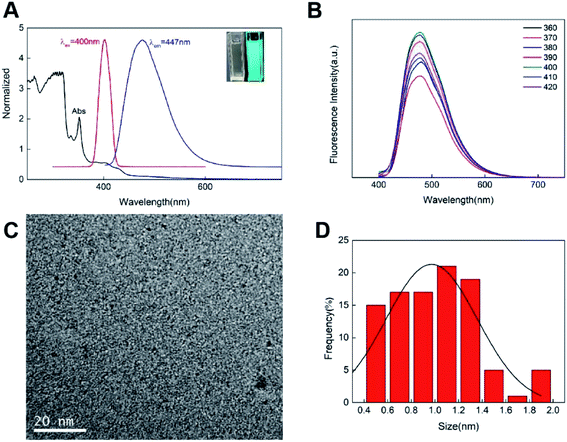 |
| Fig. 2 (A) Fluorescence and UV-vis spectra of CDs; (B) fluorescence emission spectra of CDs with different excitations; (C) TEM images of CDs; (D) diameter distribution of CDs. | |
To further elucidate the components and surface functional groups of CDs, their corresponding FTIR and XPS spectra were subsequently performed. In particular, the FTIR have C–S and SO3− telescopic vibrations, C–O stretching vibration, C–N stretch vibration and C
C stretch vibration in aromatic compounds, O–H and N–H stretching vibration, the above results indicate that amino, sulfonic and hydroxyl groups exist on the surface of the CDs (Fig. 3A). And the bending vibration of N–H indicates the presence of primary amine on the surface of the CDs. Furthermore, their XPS survey of CDs indicated that the elements of C, N, O and S were the major components, and the contents of C, N, O and S were separately determined to be 52.85%, 7.51%, 31.14% and 8.49% (Fig. 3B). To be more specific, C1s peak could be deconvoluted into three peaks of 285.69, 284.82, 284.12 eV, which were originated from C–S/C–O, C–N and C
C, respectively. Also, the deconvolution of O1s spectrum described that there existed four types of carbon bonds including C–O (532.17 eV), S
O (531.44 eV). Again, N1s spectrum exhibited the signals of C–N/N–H at 401.90 eV, C
N–C at 401.05 eV. Again, S1s spectrum exhibited the signals of S
O at 169.28 eV and C–S at 167.95 eV. These findings suggested that the results of FTIR and XPS spectra were consistent, revealing that there existed groups of hydroxyl, carboxyl and sulfuric acid groups on their surfaces of CDs.
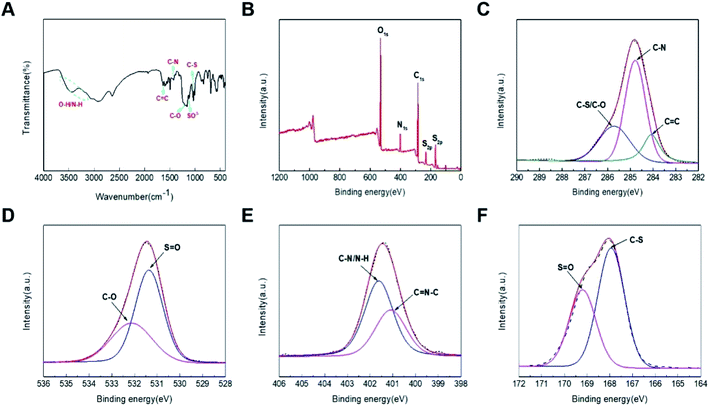 |
| Fig. 3 (A) FT-IR diagrams of N/S-CDs; (B) XPS survey of CDs; XPS spectra of C1s (C), O1s (D), N1s (E), S2p (F). | |
Optimization of synthesizing CDs
A series of experiments were designed and carried out to find out the best reaction conditions for the synthesized CDs. As revealed in Fig. S1,† the fluorescence intensity of CDs varied accompanied by the ratio of reactive solvents and the mass of the precursor, demonstrating that preparing CDs was regulated by these selected conditions. Consequently, the mass of the precursor is 0.6 g, the volume ratio of 1
:
1 for water and ethanol, 200 °C and 4 h were identified as the optimal conditions for preparing CDs.
Stability of CDs
To explore the stability of CDs, the potential effect on CDs by temperature, time, irradiation time and concentrations of NaCl, pH, organic solvents were therefore investigated in detail. As revealed in Fig. S2,† the CDs described here were generally stable with the time passing, varied temperature and different concentrations of NaCl. With the increase of ambient temperature, the fluorescence intensity of CDs will decrease slightly. The fluorescence intensity of the CDs solution decreased slightly after 6 months, indicating that the CDs were stable. With the increase of the irradiation time (400 nm), the fluorescence intensity of CDs solution decreased significantly, indicating that its anti-photobleaching was not good and it needs to be kept out of light. At the same time, with the increase of NaCl concentration, the fluorescence intensity of CDs decreased significantly, which may be because after the addition of NaCl, the interaction between the hydrophilic groups of CDs and NaCl weakened the non-radiation decay, increased the CDs aggregation degree of the system, and weakened the fluorescence intensity.26
The influence of concentration, pH value and solvent polarity on CDs fluorescence performance is shown in Fig. 4. As shown in Fig. 4A, with the increase of water, the fluorescence intensity of the CDs solution first increases and then decreases. When the volume ratio of CDs solution to water is 1
:
5, the fluorescence intensity is the strongest, which is the best concentration of CDs used for the detection experiment. The pH value of the solution not only affects the fluorescence intensity of CDs, but also affects the emission wavelength of CDs. To put it simply, the fluorescence intensity of CDs in acidic solution is stronger than that in alkaline solution, and the emission wavelength of CDs blue shifts with the pH changing from acidic to alkaline (Fig. 4B). It was due to the protonation and deprotonation of the functional groups on their surface of CDs such as amino and carboxyl groups, thus resulting in the response of CDs towards various pH.27 In addition, the fluorescence properties of CDs in organic solvents of different polarities were very diverse (Fig. 4C and D). The CDs show obvious solvent dependence, which is probably related to the functional groups on its surface. The fluorescence of CDs in an aqueous solution is very good, which is suitable for the detection of the ionic solution. In contrast, the fluorescence of CDs showed a response to diverse organic solvents and pH values, which revealed that these factors could affect the fluorescent property of CDs.
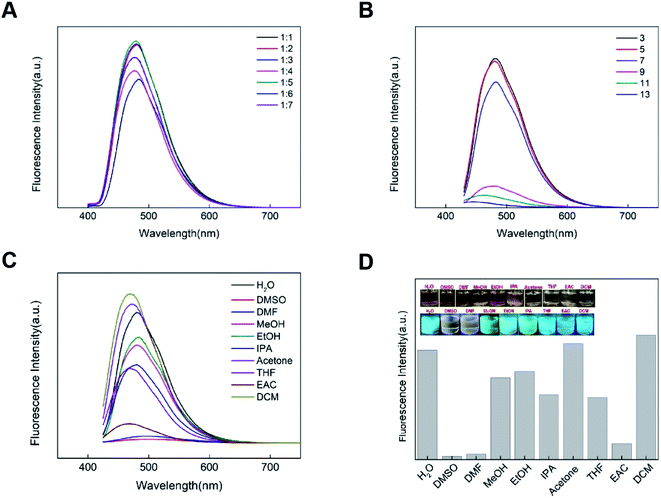 |
| Fig. 4 (A) Fluorescence intensity of CDs solution and water diluted by different volume ratio; (B) fluorescence spectra of CDs with various pH; (C) fluorescence spectra of CDs with organic solvents; (D) fluorescence intensity histogram of CDs under the action of organic solvent and the pictures in daylight and ultraviolet light. | |
The thermal stability of powdered CDs is characterized by a thermal analyzer. As revealed in Fig. S3,† with the increase of temperature, the mass of CDs decreases continuously. When the temperature is about 120 °C, the mass loss rate of CDs is stable. At this time, water and crystal water are mainly lost, and the CDs at this temperature is relatively stable. After further heating to 300 °C, CDs began to carbonize and decompose, and after heating to 600 °C, CDs almost completely decomposed.
Detection of NO2− using CDs
The obtained CDs were then investigated for the detection of nitrite. Different concentrations of nitrite ranging from 0 to 0.5 mM were added to an aqueous solution of CDs, respectively. Then the liquid was excited by the excitation wavelength of 400 nm, and the fluorescence emission spectrum was obtained. As shown in Fig. 5A, the fluorescence intensity of CDs at 480 nm decreases with the increasing nitrite concentration. This result suggests that nitrite can quench the fluorescence of the CDs. Fig. 5B shows the relationship of the fluorescence quenching value F/F0 with the concentration of nitrite (F0 and F are the fluorescence intensity of CDs at 480 nm in the absence and the presence of nitrite). Briefly, various concentrations of nitrite (0 to 0.5 mmol L−1) were separately added into CDs under the optimized conditions, and their fluorescence of CDs regularly decreased with the increasing amount of nitrite (R2 = 0.994), and the corresponding detection limit was 30 μM. In the detection method of nitrite ion, the detection effect is good.28 Therefore, the current CDs provided a new way of detecting NO2−, demonstrating its generally satisfactory detection limit and linear range.29
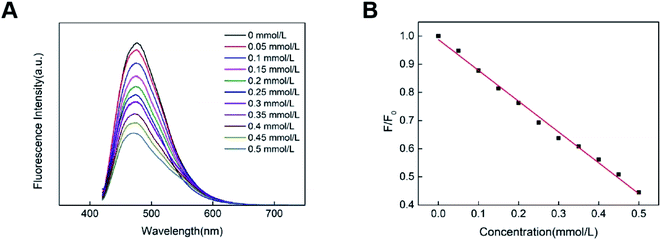 |
| Fig. 5 (A) Fluorescence spectra of CDs with various concentrations of NO2−; (B) F/F0 versus varied concentrations of NO2−. | |
Selectivity of CDs
To elucidate the selectivity of CDs detecting S2O82−, HCO3−, CO32−, HPO42−, H2PO4−, SO42−, NO3−, NO2−, Cl−, COONH4−, SO32− were investigated. Particularly, the results described that these negative ions exhibited a scarce effect on the fluorescence of CDs (Fig. S4†), indicating the acceptable selectivity of CDs towards NO2−.
The mechanism for the fluorescence response of CDs to NO2−
NO2− can quench the fluorescence of CDs prepared in this experiment, which may be related to the sulfonic groups and amino groups on the surface of CDs. To further explore the reasons for the quenching of CDs by nitrite ions, UV-vis and FTIR spectroscopy were performed on CDs after NO2− was added. As shown in Fig. 5A, in CDs solution after adding NO2−, a solution of the change in the absorption spectrum, but in the 400 nm excitation light emission wavelength of the basic no change (Fig. 6A), suggests that the addition of NO2− is only changed the absorption spectra of CDs, did not change the emission spectrum of CDs, fluorescence quenching of CDs, therefore, it is impossible to be dynamic quenching, because dynamic quenching affects only the fluorescent molecular excited states, and does not alter the absorption spectra of the fluorescent substance.30 The absorption peak of PA-CDs after participating in the coupling reaction with NO2− exhibited a red shift, which was attributed to the formation of an azo group (N
N) via a diazo coupling reaction.28 As shown in Fig. 6B, after the addition of NO2−, the absorption peak of the functional group of the CDs changes significantly, and the peak strength of the functional group changes significantly. The absorption peak of sulfur group is significantly reduced, which is probably due to the oxidation of sulfonyl group to sulfuric acid group by nitrite ion. The oxidation of sulfonic groups by nitrites to sulfuric acid is natural because the oxidation of NO2− under acidic conditions is stronger than that of NO3−. And of course, there's also hydrogen bonding, which changes the stretching vibrations of the bonds. These factors together lead to the fluorescence quenching of CDs.
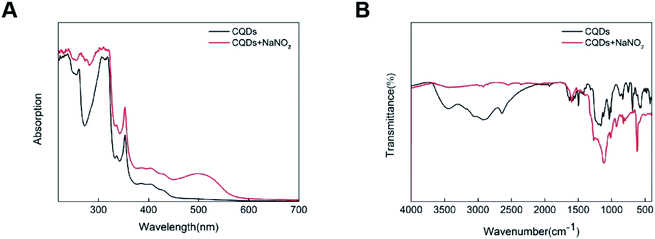 |
| Fig. 6 (A) UV-vis absorption spectra of N/S-CDs without and with two concentrations of NO2−; (B) FTIR spectroscopy of N/S-CDs without and with two concentrations of NO2−. | |
The prospect of easy detection
Methylcellulose/CDs fluorescence film was placed in deionized water, and it was found that it could be completely dissolved, and the solution had fluorescence, as shown in Fig. S5.† Then, NO2− was added to the solution, and it was found that it could be quenched, which made portable ion detection possible.
Conclusions
In summary, we have successfully prepared novel N/S-CDs by using a one-step solvothermal method with p-aminobenzenesulfonic acid as the precursor, which is green and effective and does not require any posttreatment. Meanwhile, the proposed CDs exhibited the generally favorable blue-green fluorescence and were elucidated to be equipped with the functional groups of amino, hydroxyl and sulfuric acid groups. This increases the number of effective induction sites on the surface of the CDs, giving it better selectivity and sensitivity. Besides, it is sensitive to PH, organic solvents and irradiation time. Significantly, the introduction of NO2− into CDs resulted in their reduced fluorescence. Besides, the obtained CDs show high selectivity and sensitivity for the detection of NO2− in aqueous solution with a low detection limit of 0.03 mM owing to the fluorescence quenching effect of the CDs. Again, our findings show that the fluorescence quenching of CDs caused by nitrite is the result of the combined action including diazo coupling reaction and oxidation–reduction reaction. At the same time, the methylcellulose/CDs complex is soluble in water and can maintain the fluorescence of CDs, which provides an idea for an environment-friendly portable fluorescence sensor.
Conflicts of interest
There are no conflicts to declare.
Acknowledgements
Thanks to the School of Printing and Packaging, Wuhan University for its instrument support; this paper is supported by Lab of Green Platemaking and Standardization for Flexographic Printing (the project number is ZBKT202002).
References
- H. Ding, S. B. Yu and J. S. Wei, et al. Full-color light-emitting carbon dots with a surface-state-controlled luminescence mechanism, ACS Nano, 2016, 10(1), 484–491 CrossRef CAS PubMed.
- Y. Li, Y. Hu and Y. Zhao, et al. An electrochemical avenue to green-luminescent graphene quantum dots as potential electron-acceptors for photovoltaics, Adv. Mater., 2011, 23(6), 776–780 CrossRef CAS PubMed.
- H. Ding, F. Du and P. Liu, et al. DNA-carbon dots function as fluorescent vehicles for drug delivery, ACS Appl. Mater. Interfaces, 2015, 7(12), 6889–6897 CrossRef CAS PubMed.
- Y. Dong, R. Wang and H. Li, et al. Polyamine-functionalized carbon quantum dots for chemical sensing, Carbon, 2012, 50(8), 2810–2815 CrossRef CAS.
- S. Zhu, Q. Meng and L. Wang, et al. Highly photoluminescent carbon dots for multicolor patterning, sensors, and bioimaging, Angew. Chem., Int. Ed., 2013, 52(14), 3953–3957 CrossRef CAS PubMed.
- M. X. Gao, C. F. Liu and Z. L. Wu, et al. A surfactant-assisted redox hydrothermal route to prepare highly photoluminescent carbon quantum dots with aggregation-induced emission enhancement properties, Chem. Commun., 2013, 49(73), 8015–8017 RSC.
- K. Jiang, S. Sun and L. Zhang, et al. Red, Green, and Blue Luminescence by Carbon Dots: Full-Color Emission Tuning and Multicolor Cellular Imaging, Angew. Chem., 2015, 54(18), 5360–5363 CrossRef CAS PubMed.
- S. Qu, X. Wang and Q. Lu, et al. A Biocompatible Fluorescent Ink Based on Water-Soluble Luminescent Carbon Nanodots, Angew. Chem., 2012, 124(49), 12381–12384 CrossRef.
- Q. L. Zhao, Z. L. Zhang, B. H. Huang, J. Peng, M. Zhang and D. W. Pang, Facile preparation of low cytotoxicity fluorescent carbon nanocrystals by electrooxidation of graphite, Chem. Commun., 2008, 5116–5118 RSC.
- B. Zhu, S. Sun and Y. Wang, et al. Preparation of carbon nanodots from single chain polymeric nanoparticles and theoretical investigation of the photoluminescence mechanism, J. Mater. Chem. C, 2012, 1(3), 580–586 RSC.
- V. B. Kumar, J. Sheinberger and Z. Porat, et al. A hydrothermal reaction of an aqueous solution of BSA yields highly fluorescent N doped C-dots used for imaging of live mammalian cells, J. Mater. Chem. B, 2016, 4(17), 2913–2920 RSC.
- L. Wang, B. Li and F. Xu, et al. High-yield synthesis of strong photoluminescent N-doped carbon nanodots derived from hydrosoluble chitosan for mercury ion sensing via smartphone APP, Biosens. Bioelectron., 2015, 79, 1 CrossRef PubMed.
- V. Sharma, P. Tiwari and S. M. Mobin, Sustainable carbon-dots: recent advances in green carbon dots for sensing and bioimaging, J. Mater. Chem. B, 2017, 5(45), 8904–8924 RSC.
- Y. Zhang, J. Nie and H. Wei, et al. Electrochemical detection of nitrite ions using Ag/Cu/MWNT nanoclusters electrodeposited on a glassy carbon electrode, Sens. Actuators, B, 2017, 258, 1107–1116 CrossRef.
- H. Wu, X. Shen and D. Huo, et al. Fluorescent and colorimetric dual-readout sensor based on Griess assay for nitrite detection, Spectrochim. Acta, Part A, 2020, 225, 117470 CrossRef CAS PubMed.
- B. M. Jayawardane, S. Wei and I. D. Mckelvie, et al. Microfluidic Paper-Based Analytical Device for the Determination of Nitrite and Nitrate, Anal. Chem., 2014, 86(15), 7274 CrossRef CAS PubMed.
- X. Ding, J. Yang and Y. Dong, Advancements in the preparation of high-performance liquid chromatographic organic polymer monoliths for the separation of small-molecule drugs, J. Pharm. Anal., 2018, 8(002), 75–85 CrossRef PubMed.
- Y. Zhong, P. Chen and X. Yu, et al. Determination of nitrite and nitrate in dairy products by improved ion chromatography, Chin. J. Chromatogr., 2012, 30(6), 635 CrossRef CAS PubMed.
- N. Altunay, R. Gürkan and E. Olgaç, Development of a New Methodology for Indirect Determination of Nitrite, Nitrate, and Total Nitrite in the Selected Two Groups of Foods by Spectrophotometry, Food Anal. Methods, 2017, 10(7), 1–13 Search PubMed.
- J. Zhu, C. Li and S. Liu, et al. A non-diazotization-coupling reaction-based colorimetric determination of nitrite in tap water and milk, Eur. Food Res. Technol., 2014, 238(5), 889–894 CrossRef CAS.
- Y. Xiong, C. J. Wang and T. Tao, et al. A miniaturized fiber-optic colorimetric sensor for nitrite determination by coupling with a microfluidic capillary waveguide, Anal. Bioanal. Chem., 2016, 408(13), 3413–3423 CrossRef CAS PubMed.
- S. Zhu, L. Zhao and B. Yan, A novel spectroscopic probe for detecting food preservative NO2: citric acid functionalized metal–organic framework and luminescence sensing, Microchem. J., 2020, 155, 104768 CrossRef CAS.
- J. Jana, H. J. Lee and J. S. Chung, et al. Blue emitting nitrogen-doped carbon dots as a fluorescent probe for nitrite ion sensing and cell-imaging, Anal. Chim. Acta, 2019, 1079, 212–219 CrossRef CAS PubMed.
- Y. Deng, J. Qian and Y. Zhou, et al. Regulatory Preparation of N/S Doped Carbon Quantum Dots and Their Applications as Fe(III) Ion Sensors, ChemistrySelect, 2020, 5(17), 5306–5311 CrossRef CAS.
- R. Wang, X. Wang and Y. Sun, One-step synthesis of self-doped carbon dots with highly photoluminescence as multifunctional biosensors for detection of iron ions and pH, Sens. Actuators, B, 2017, 241, 73–79 CrossRef CAS.
- X. U. Jin-Gou and W. Zun-Ben, Fluorometric Analysis Method, Beijing, Science Press, 2006, pp. 64–70 Search PubMed.
- D. Gao, X. Liu and D. Jiang, et al. Exploring of multicolor emissive carbon dots with novel double emission mechanism, Sens. Actuators, B., 2018, 277, 373–380 CrossRef CAS.
- W. Li, S. Huang and H. Wen, et al. Fluorescent recognition and selective detection of nitrite ions with carbon quantum dots, Anal. Bioanal. Chem., 2020, 412(4), 993–1002 CrossRef CAS PubMed.
- L. Gan, Q. Su and Z. Chen, et al. Exploration of pH-responsive carbon dots for detecting nitrite and ascorbic acid, Appl. Surf. Sci., 2020, 530, 147269 CrossRef CAS.
- T. G. Chatzimitakos and C. D. Stalikas, Carbon nanodots from natural (re)sources: a new perspective on analytical chemistry, Handb. Nanomater. Anal. Chem., 2020, 3–28 CAS.
Footnote |
† Electronic supplementary information (ESI) available. See DOI: 10.1039/d0ra10766b |
|
This journal is © The Royal Society of Chemistry 2021 |