DOI:
10.1039/D0RA10548A
(Paper)
RSC Adv., 2021,
11, 13666-13673
A facile and efficient route to one-pot synthesis of new cyclophanes using vinamidinium salts†
Received
15th December 2020
, Accepted 26th March 2021
First published on 13th April 2021
Abstract
In this study, an efficient method for the synthesis of new cyclophanes (5a–f, 6a–g) through the condensation of 1,4-phenylenedimethanamine (3) or 2,3,5,6-tetramethylbenzene-1,4-diamine (4) with 2-substituted vinamidiniums (2a–g) is described. The cyclophane derivatives are obtained in good to excellent yields in the presence of acetic acid in refluxing acetonitrile after 15 h. The structure of new compounds was validated based on their spectral data (1H NMR, 13C NMR, IR) and elemental analysis.
Introduction
The structure of a vast range of macromolecules1 confirmed that the design and construction of macrocyclic compounds has been one of the most important reasons for improvement in supramolecular science. A well-known group of macrocyclic compounds are cyclophanes and their particular chemistry has attracted the attention of researchers, recently2 and has been broadly discussed within the field of modern supra-molecular chemistry.3,4 Cyclophanes5–42 are constrained organic molecules consisting of aromatic ring(s) as well as aliphatic unit(s). The aromatic rings support the rigidity of their structure, while the aliphatic unit(s) create bridge(s) between the aromatic rings and cause the flexibility of the whole structure.
Due to their special structure, they are considered as an important class of compounds in “host–guest” chemistry43–47 and supramolecular assembly.48–51 On the other hand, the clear structure and high strain of cyclophanes has contributed in a large number of applications such as pharmaceuticals,52,53 asymmetric catalysis,54 insulating plastics,55 organic electronics,56 metal capture57,58 and supramolecular chemistry.59 Besides, since cyclophane structure is the main foundation unit in many biologically active natural products (Fig. 1),60–62 their design and application is a special interest of groups working in fields including biological, medicinal and organic chemistry. Moreover, cyclophanes are attractive in the area of theoretical chemistry due to their particular topology and intra-molecular interactions.
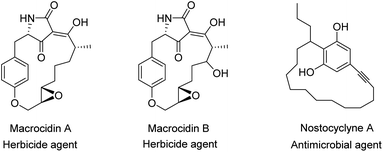 |
| Fig. 1 Natural products containing the cyclophane skeleton. | |
So far, several methods have been reported for the synthesis of cyclophanes compounds63–66 (Scheme 1). In some instances, cyclophanes are formed in low yields and also with side products. Some procedures require many steps or harsh reaction conditions such as high-pressure, and difficult separation methods. Therefore, designing better routes and improving conditions to achieve cyclophanes is still needed.
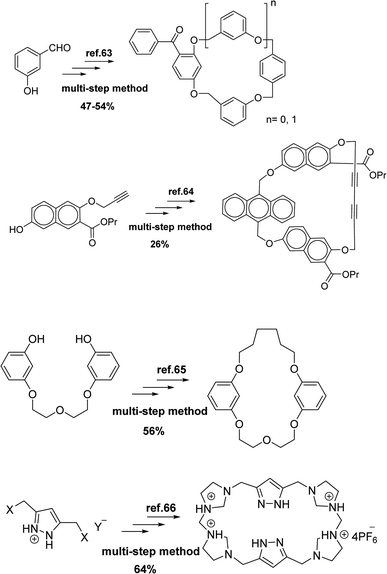 |
| Scheme 1 Reported methods for the synthesis of cyclophanes. | |
Vinamidinium salts are examples of stabilized alkenes by “push–pull” influences between the electron-donating amino group and the electron-withdrawing ammonium group. They can easily undergo condensation reaction with bifunctional nucleophiles to form heterocycles. During past years, our group has been investigated the utilization of vinamidinium salts for the synthesis of heterocyclic compounds67–76 (Scheme 2).
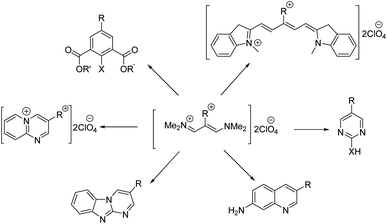 |
| Scheme 2 Application of vinamidinium salts in organic synthetic chemistry. | |
Results and discussion
In continuation of these studies and due to the importance of cyclophanes in different branches of sciences, we decided to investigate the one-pot synthesis of cyclophanes from vinamidinium salts (Scheme 3). To the best of our knowledge, vinamidinium salts have not yet been applied for preparing cyclophanes.
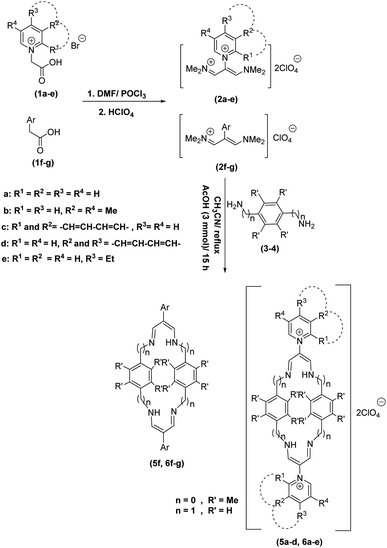 |
| Scheme 3 Synthesis of cyclophanes. | |
As shown in Scheme 3, the procedure is done in two-step: (i) synthesis of the 2-substituted vinamidinium salts (2a–g) by the Vilsmeier–Arnold formylation of the substituted acetic acids (1a–g) as explained in authors previous work;67–76 and (ii) synthesis of cyclophane derivatives (5a–f, 6a–g) by the reaction of 2-substituted vinamidinium salts (2a–g) with 1,4-phenylenedimethanamine (3) or 2,3,5,6-tetramethylbenzene-1,4-diamine (4) by applying acetic acid in acetonitrile as solvent.
In the first step, vinamidiniums were isolated as the perchlorate salts and used directly without additional purification for the synthesis of cyclophanes.
As illustrated in Scheme 3, the symmetrical vinamidinium salts (2a–g) were able to react with 1,4-phenylenedimethanamine (3) or 2,3,5,6-tetramethylbenzene-1,4-diamine (4) in refluxing acetonitrile in the presence of acetic acid for 15 h to manage the cyclophane derivatives (5a–f, 6a–g). To provide the best reaction conditions in second step, the reaction of vinamidinium salt 2f with 1,4-phenylenedimethanamine (3) was chosen as model reaction and the impacts of solvents and catalysts were investigated. The obtained results are summarized in Table 1. When EtOH and MeOH were applied as the solvent and the mixture was subjected to reflux in the presence of AcOH, the desired product, 5f, was achieved in low yields (43% and 36%, respectively) after 24 h (Table 1, entries 1 and 2). In a modified protocol, the reaction was carried out in refluxing acetonitrile. In this case, remarkable improvement of yield was observed (Table 1, entry 3). No better results were obtained when the reaction was carried out in DMF, CHCl3, CH2Cl2 or toluene (Table 1, entries 4–7). Therefore, the subsequent reactions were carried out in CH3CN.
Table 1 Effect of the different reaction parameters on the reaction of 1,4-phenylenedimethanamine (3) with ((E)-N-(3-(dimethylamino)-2-(naphthalen-1-yl)allylidene)-N-methylmethanaminium perchlorate (2f))
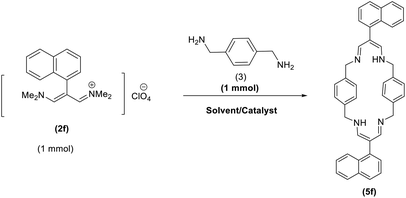
|
Entry |
Condition |
Solvent |
Time (h) |
Yielda (%) |
Isolated yield. |
1 |
AcOH (3 mmol) |
EtOH/reflux |
24 |
43 |
2 |
AcOH (3 mmol) |
MeOH/reflux |
24 |
36 |
3 |
AcOH (3 mmol) |
CH3CN/reflux |
15 |
90 |
4 |
AcOH (3 mmol) |
CHCl3/reflux |
24 |
35 |
5 |
AcOH (3 mmol) |
CH2Cl2/reflux |
24 |
Trace |
6 |
AcOH (3 mmol) |
Toluene/reflux |
24 |
— |
7 |
AcOH (3 mmol) |
DMF/100 °C |
24 |
45 |
8 |
Et3N (3 mmol) |
CH3CN/reflux |
24 |
— |
9 |
i-Pr2NEt (3 mmol) |
CH3CN/reflux |
24 |
— |
10 |
— |
CH3CN/reflux |
24 |
— |
11 |
AcOH (4 mmol) |
CH3CN/reflux |
15 |
90 |
12 |
AcOH (2 mmol) |
CH3CN/reflux |
24 |
64 |
13 |
AcOH (1 mmol) |
CH3CN/reflux |
24 |
40 |
After choosing the solvent, the model reaction was conducted under neutral and basic conditions. As shown in entries 8 and 9 of Table 1, in the presence of basic catalysts such as triethylamine and ethyldiisopropylamine, the desired product 5f, was not achieved. In neutral conditions also, no product was formed (Table 1, entry 10). Increasing the amount of AcOH did not affect the reaction appreciably, while, decreasing the amount of AcOH, resulted the product in lower yield (Table 1, entries 11–13). So, acidic media is critical to the success of the reaction.
We then applied the obtained optimized conditions for the reaction of different vinamidinium salts with 1,4-phenylenedimethanamine (3). The results are listed in Table 2.
Table 2 The synthesis of cyclophane derivatives from the reaction of 2-substituted vinamidinium salts (2a–g) (1.0 mmol), 1,4-phenylenedimethanamine (3) (1.0 mmol) in the presence of AcOH (3.0 mmol) in CH3CN (8.0 mL) at reflux conditions
The results demonstrated that the variety of vinamidinium salts were successfully employed in this process, affording novel cyclophanes in high yields in suitable reaction times.
After successful application of 1,4-phenylenedimethanamine (3) in cyclophane synthesis, to enhance the generality of the system, we applied 2,3,5,6-tetramethylbenzene-1,4-diamine (4) as bifunctional nucleophile and investigated the formation of new cyclophanes.
2,3,5,6-Tetramethylbenzene-1,4-diamine (4) was also tolerated well in this procedure to give the desired products (Table 3).
Table 3 The synthesis of cyclophane derivatives from the reaction of 2-substituted vinamidinium salts (2a–g) (1.0 mmol), 2,3,5,6-tetramethylbenzene-1,4-diamine (4) (1.0 mmol) in the presence of AcOH (3.0 mmol) in CH3CN (8.0 mL) at reflux conditions
According to our previous works and above results, a reasonable mechanism for the synthesis of cyclophane compounds (5a–f, 6a–g) in the presence of AcOH is illustrated in Scheme 4.
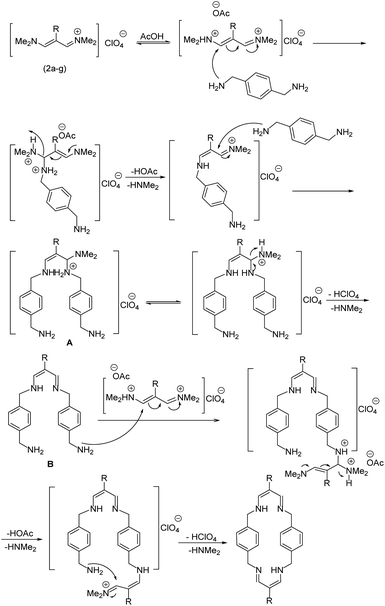 |
| Scheme 4 The proposed mechanism for the synthesis of cyclophanes in the presence of AcOH. | |
The reaction proposed by the initial attack of the amino group in 1,4-phenylenedimethanamine (3) or 2,3,5,6-tetramethylbenzene-1,4-diamine (4) to protonated vinamidinium salt. Then, removal of dimethylamine occurs, followed by the nucleophilic attack of second molecule of amine on the obtained iminium salt to produce intermediate A. The loss of the second dimethylamine molecule produces intermediate B. The reaction of this intermediate with the second molecule of vinamidinium salt, followed by the loss of two dimethylamine molecules and intramolecular nucleophilic cyclization, yields the desired cyclophane.
Conclusion
In this study, an efficient and applicable protocol has been developed for the synthesis of novel cyclophanes by the reaction of 2-substituted vinamidinium salts with 1,4-phenylenedimethanamine or 2,3,5,6-tetramethylbenzene-1,4-diamine in the presence of acetic acid.
This protocol has several advantages such as: simple and one-step procedure, absence of by-products, inexpensive catalyst, normal atmospheric conditions, high to excellent yields and easy purification of the products. Furthermore, the products are well-known, stable solids and have a long shelf-life when stored in an anhydrous environment.
Experimental section
General procedure for the synthesis of cyclophane derivatives (5a–f, 6a–g)
To a flame dried one-necked round-bottomed flask equipped with magnetic stirring and reflux condenser, 2-substituted vinamidinium salts (2a–g) (1.0 mmol), 1,4-phenylenedimethanamine (3) or 2,3,5,6-tetramethylbenzene-1,4-diamine (4) (1.0 mmol), AcOH (3.0 mmol) and CH3CN (8.0 mL) were added. The mixture was allowed to reflux for 15 h in an oil bath. After completion of the reaction, distilled H2O (20 mL) was added to the mixture. The resulting precipitate was gathered, washed with Et2O (3 × 3 mL). Finally, the precipitate was washed with 2-propanol (3 × 3 mL) and dried under vacuum at 80 °C to afford the corresponding cyclophanes.
Conflicts of interest
There are no conflicts of interest to declare.
Acknowledgements
The authors thank Persian Gulf University Research Councils for the financial support of this worK.
Notes and references
- Encyclopedia of supramolecular chemistry, ed. J. L. Atwood and J. W. Steed, Marcel Dekker, New York, 2004 Search PubMed.
- Modern cyclophane chemistry, ed. R. Gleiter and H. Hopf, Wiley-VCH, Weinheim, 2004 Search PubMed.
- J. W. Steed and J. L. Atwood, Supramolecular chemistry, John Wiley & Sons Ltd, Chichester, England, 2000 Search PubMed.
- F. Diederich, Cyclophanes. Monographs in Supramolecular Chemistry, ed. J. F. Stoddart, The Royal Society of Chemistry, Cambridge, United Kingdom, 1989 Search PubMed.
- C. Li, Pillararene-based supramolecular polymers: from molecular recognition to polymeric aggregates, Chem. Commun., 2014, 50, 12420 RSC.
- J. N. Kim and K. Y. Lee, Synthesis of cyclic compounds from the Baylis-Hillman adducts, Curr. Org. Chem., 2002, 6, 627 CrossRef CAS.
- Ś. W. liwa and T. Zujewska, Interlocked molecules containing quaternary azaaromatic moieties, Heterocycles, 2005, 65, 1713 CrossRef.
- G. J. Bodwell and P. R. Nandaluru, Olefination reactions in the synthesis of cyclophanes, Isr. J. Chem., 2012, 52, 105 CrossRef CAS.
- Y. Okada and J. Nishimura, The design of cone-fixed calix [4]arene analogs by taking syn-[2.n]metacyclophanes as a building block, J. Inclusion Phenom. Mol. Recognit. Chem., 1994, 19, 41 CrossRef CAS.
- M. H. Schwartz, Charge-transfer interactions in cyclophanes, J. Inclusion Phenom. Mol. Recognit. Chem., 1990, 9, 1 CrossRef CAS.
- M. Ángeles Herranz, J. A. Rivera, R. J. Alvarado, N. Martn, C. Thilgen, F. Diederich and L. Echegoyen, Chemical retro-cyclopropanation reactions in methanofullerenes: effect of the 18-crown-6 moiety, J. Supramol. Chem., 2001, 1, 299 CrossRef.
- T. Gulder and P. S. Baran, Strained cyclophane natural products: macrocyclization at its limits, Nat. Prod. Rep., 2012, 29, 899 RSC.
- M. J. McGlinchey and S. Milosevic, From [10] paracyclophane to ferrocenophanones, Isr. J. Chem., 2012, 52, 30 CrossRef CAS.
- D. Ramaiah, P. P. Neelakandan, A. K. Nair and R. R. Avirah, Functional cyclophanes: promising hosts for optical biomolecular recognition, Chem. Soc. Rev., 2010, 39, 4158 RSC.
- T. Gaich and P. S. Baran, Aiming for the ideal synthesis, J. Org. Chem., 2010, 75, 4657 CrossRef CAS PubMed.
- R.-Y. Tang, G. Li and J.-Q. Yu, Conformation-induced remote meta-C–H activation of amines, Nature, 2014, 507, 215 CrossRef CAS PubMed.
- S. R. Naini, S. Ranganathan, J. S. Yadav, K. V. S. Ramakrishna, G. Gayatri, G. N. Sastry, K. B. Roy and N. Shamala, The exploration of Kemp's triacid (KTA) as the core for the synthesis of 3-fold symmetric 23-cyclophane, 22-cyclophane and novel linker directed designs, RSC Adv., 2014, 4, 5322 RSC.
- M. Juriček, N. L. Strutt, J. C. Barnes, A. M. Butterfield, E. J. Dale, K. K. Baldridge, F. Stoddart and J. S. Siegel, Induced-fit catalysis of corannulene bowl-to-bowl inversion, Nat. Chem., 2014, 6, 222 CrossRef PubMed.
- S. Gago, J. González, S. Blasco, A. J. Parola, M. T. Albelda, E. Garcia-España and F. Pina, Protonation, coordination chemistry, cyanometallate “supercomplex” formation and fluorescence chemosensing properties of a bis(2,2′-bipyridino)cyclophane receptor, Dalton Trans., 2014, 43, 2437 RSC.
- M. Wang, Clicking cyclophane to boron doped diamond surfaces, Chin. Sci. Bull., 2013, 58, 2898 CrossRef CAS.
- P. Rajakumar, R. Padmanabhan and N. Rajesh, Synthesis, study on anti-arthritic, anti-inflammatory activity and toxicity of some novel bis-oxy cyclophane diamides, Bioorg. Med. Chem. Lett., 2012, 22, 3770 CrossRef CAS PubMed.
- K. Kanda, R. Hamanaka, K. Endo and T. Shibata, Asymmetric ortho-lithiation of 1,n-dioxa[n]paracyclophane derivatives for the generation of planar chirality, Tetrahedron, 2012, 68, 1407 CrossRef CAS.
- O. Hayashida and K. Ichimura, Synthesis and characterization of reduction-responsive cyclophane dimer based on disulfide linkage, Chem. Lett., 2012, 41, 1650 CrossRef CAS.
- R. J. Vermeij, D. O. Miller, L. N. Dawe, I. Aprahamian, T. Sheradsky, M. Rabinovitz and G. J. Bodwell, Mixed [2.2]cyclophanes of pyrene and benzene, Aust. J. Chem., 2010, 63, 1703 CrossRef CAS.
- G. J. Bodwell, R. Frim, H. Hopf and M. Rabinovitz, Cyclophanes, XXXVIII. [2]metacyclo[2]indenophanes: synthesis, anions and iron complexes, Chem. Ber., 1993, 126, 167 CrossRef CAS.
- V. A. D, yakonov, O. A. Trapeznikova, A. de Meijere and U. M. Dzhemilev, Metal complex catalysis in the synthesis of spirocarbocycles, Chem. Rev., 2014, 114, 5775 CrossRef PubMed.
- S. Sankararaman, M. Srinivasan, V. Narayanan and B. Varghese, Synthesis of cyclophanes bearing 1,4-dioxabut-2-yne and 1,6-dioxahexa-2,4-diyne bridges and nanoscale cavities, Indian J. Chem., Sect. B: Org. Chem. Incl. Med. Chem., 2004, 43, 1499 Search PubMed.
- Y. Yang, M. R. Mannion, L. N. Dawe, C. M. Kraml, R. A. Pascal Jr and G. J. Bodwell, Synthesis, crystal structure, and resolution of [10](1,6)pyrenophane: an inherently chiral [N]cyclophane, J. Org. Chem., 2012, 77, 57 CrossRef CAS PubMed.
- G. J. Bodwell, D. O. Miller and R. J. Vermeij, Nonplanar aromatic compounds. 6. [2] paracyclo[2](2,7)pyrenophane. A novel strained cyclophane and a first step on the road to a “Vögtle” belt, Org. Lett., 2001, 3, 2093 CrossRef CAS.
- G. J. Bodwell, T. J. Houghton and D. Miller, Synthesis, structure and AM1 conformational study of 1,12-dioxa-2,11-dioxo[3.3]orthocyclophane, Tetrahedron Lett., 1997, 38, 1469 CrossRef CAS.
- G. A. Boyle, T. Govender, H. G. Kruger and G. E. M. Maguire, Synthesis of novel 3-hydroxy-3-pyridylcamphor derivatives, Tetrahedron: Asymmetry, 2004, 15, 3775 CrossRef CAS.
- P. R. Ashton, V. Balzani, A. Credi, O. Kocian, D. Pasini, L. Prodi, N. Spencer, J. F. Stoddart, M. S. Tolley, M. Venturi, A. J. P. White and D. J. Williams, Cyclophanes and [2]catenanes as ligands for transition metal complexes: synthesis, structure, absorption spectra, and excited state and electrochemical properties, Chem.–Eur. J., 1998, 4, 590 CrossRef CAS.
- J. B. Bremner, P. A. Keller, S. G. Pyne, A. D. Robertson, B. W. Skelton, A. H. White and H. M. Witchard, The synthesis of new dibenzothiophene amino acid and cyclophane derivatives, Aust. J. Chem., 2000, 53, 535 CrossRef CAS.
- M. V. Baker, D. H. Brown, R. A. Haque, B. W. Skelton and A. H. White, A new binding geometry for an ortho-xylylene-linked bis(NHC)cyclophane: a ruthenium(II) complex with a chelating (η1-NHC)2:η6-arene ligand, Dalton Trans., 2010, 39, 70 RSC.
- J. R. Davy and J. A. Reiss, Cyclophanes. IV. Synthetic approaches to cyclophane dienes. The preparation of [2,2](2,7)naphthalenophane-1,11-diene, Aust. J. Chem., 1976, 29, 163 CrossRef CAS.
- C. Davies, L. Ren, R. Gustafson, T. Buthelezi, R. A. Bartsch and M. Surowiec, Molecular association of benzene with a new cyclophane receptor, J. Inclusion Phenom. Macrocyclic Chem., 2008, 61, 347 CrossRef CAS.
- R. A. Bartsch, P. Kus, N. K. Dalley and X. Kou, A novel cyclophane-anthracene complex, Tetrahedron Lett., 2002, 43, 5017 CrossRef CAS.
- R. T. Swann and V. Boekelheide, Multilayered iron complexes of metacyclophanes, J. Organomet. Chem., 1982, 231, 143 CrossRef CAS.
- F. Gerson, J. Lopez and V. Boekelheide, The radical anion of [24](1,2,4,5)cyclophane, J. Chem. Soc., Perkin Trans. 2, 1981, 1298 RSC.
- Y. Morisaki, R. Hifumi, L. Lin, K. Inoshita and Y. Chujo, Practical optical resolution of planar chiral pseudo-ortho-disubstituted [2.2]paracyclophane, Chem. Lett., 2012, 41, 990 CrossRef CAS.
- Y. Morisaki and Y. Chujo, Synthesis of novel alternating π-conjugated copolymers having [2.2]paracyclophane and fluorene units in the main chain leading to the blue light-emitting materials, Chem. Lett., 2002, 31, 194 CrossRef.
- H. Takemura, S. Nakata, A. Inoue and A. Mishima, Synthesis of a hexacationic cyclophane and formation of EDA-type host–guest complexes, J. Inclusion Phenom. Macrocyclic Chem., 2013, 77, 483 CrossRef CAS.
- J. C. Barnes, M. Juriček, N. L. Strutt, M. Frasconi, S. Sampath, M. A. Giesener, P. L. McGrier, C. J. Bruns, C. L. Stern, A. A. Sarjeant and J. F. Stoddart, A polycyclic aromatic hydrocarbon scavenger, J. Am. Chem. Soc., 2013, 135, 183 CrossRef CAS.
- P. Wald and H.-J. Schneider, Reinvestigation of supramolecular complexes with cyclophanes of the Stetter and Koga type: agreement and disagreement with solid-state structures, Eur. J. Org. Chem., 2009, 3450 CrossRef CAS.
- H. Takemura, [1n]Paracyclophanes, Curr. Org. Chem., 2009, 13, 1633 CrossRef CAS.
- B. Masci, S. Pasquale and P. Thuéry, Supramolecular control of a fast and reversible Diels–Alder reaction, Org. Lett., 2008, 10, 4835 CrossRef CAS PubMed.
- A. Schmitt, O. Perraud, E. Payet, B. Chatelet, B. Bousquet, M. Valls, D. Padula, L. Di Bari, J.-P. Dutasta and A. Martinez, Improved hemicryptophane hosts for the stereoselective recognition of glucopyranosides, Org. Biomol. Chem., 2014, 12, 4211 RSC.
- H. Abe, K. Ohtani, D. Suzuki, Y. Chida, Y. Shimada, S. Matsumoto and M. Inouye, Pyridine-acetylene macrocycles: π-stacking self-assemblies enhanced by intermolecular dipole–dipole interaction, Org. Lett., 2014, 16, 828 CrossRef CAS PubMed.
- M. Juriček, J. C. Barnes, E. J. Dale, W.-G. Liu, N. L. Strutt, C. J. Bruns, N. A. Vermeulen, K. C. Ghooray, A. A. Sarjeant, C. L. Stern, Y. Y. Botros, W. A. Goddard and J. F. Stoddart, Interdependent modes of binding in a two-nanometer-long synthetic receptor, J. Am. Chem. Soc., 2013, 135, 12736 CrossRef PubMed.
- E. Elacqua, T. Friščić and L. R. MacGillivray, Paracyclophane as a target of the organic solid state: emergent properties via supramolecular construction, Isr. J. Chem., 2012, 52, 53 CrossRef CAS.
- P. Hu, S. Yang and G. Feng, Discrimination of adenine nucleotides and pyrophosphate in water by a zinc complex of an anthracene-based cyclophane, Org. Biomol. Chem., 2014, 12, 3701 RSC.
- C. J. Bruns, M. Frasconi, J. Iehl, K. J. Hartlieb, S. T. Schneebeli, C. Cheng, S. I. Stupp and J. F. Stoddart, Redox switchable daisy chain rotaxanes driven by radical-radical interactions, J. Am. Chem. Soc., 2014, 136, 4714 CrossRef CAS PubMed.
- E. M. Driggers, S. P. Hale, J. Lee and N. K. Terrett, The exploration of macrocycles for drug discovery—an underexploited structural class, Nat. Rev. Drug Discovery, 2008, 7, 608 CrossRef CAS PubMed.
- L. Jimenez and F. Diederich, Catalytic cyclophanes: a highly efficient model for pyruvate oxidase, Tetrahedron Lett., 1989, 30, 2759 CrossRef CAS.
- P. J. Pye, P. J. Pye, K. Rossen, R. A. Reamer, N. N. Tsou, R. P. Volante and P. J. Reider, A new planar chiral bisphosphine ligand for asymmetric catalysis: highly enantioselective hydrogenations under mild conditions, J. Am. Chem. Soc., 1997, 119, 6207 CrossRef CAS.
- W. F. Gorham, A new, general synthetic method for the preparation of linear poly-p-xylylenes, J. Polym. Sci., Part A: Polym. Chem., 1966, 4, 3027 CrossRef CAS.
- C.-Y. Yu and M. L. Turner, Soluble poly(p-phenylenevinylenes) through ring-opening metathesis polymerization, Angew. Chem., Int. Ed., 2006, 45, 7797 CrossRef CAS PubMed.
- J. Gross, G. Harder, A. Siepen, J. Harren, F. Vögtle, H. Stephan, K. Gloe, B. Ahlers, K. Cammann and K. Rissanen, Concave hydrocarbons, Chem.–Eur. J., 1996, 2, 1585 CrossRef CAS.
- K. Cammann, Das arbeiten mit ionenselektiven elektroden, Springer, 1996 Search PubMed.
- S. Kotha and M. K. Dipak, Strategies and tactics in olefin metathesis, Tetrahedron, 2012, 68, 397 CrossRef CAS.
- D. D. Ridley, E. Ritchie and W. C. Taylor, Chemical studies of the proteaceae. IV. The structures of the major phenols of grevillea striata; a group of novel cyclophanes, Aust. J. Chem., 1970, 23, 147 CrossRef CAS.
- D. J. Newman, G. M. Cragg and K. M. Snader, The influence of natural products upon drug discovery, Nat. Prod. Rep., 2000, 17, 215 RSC.
- Y. Vida and E. Perez-Inestrosa, Cyclophane size drives the photochemical behaviour of benzophenone, Photochem. Photobiol. Sci., 2012, 11, 1645 CrossRef CAS.
- M. J. Cloninger and H. W. Whitlock, A synthetic receptor which uses multiple edge–face interactions to bind aromatic guests, J. Org. Chem., 1998, 63, 6153 CrossRef CAS PubMed.
- S. Kotha and G. T. Waghule, New approach to cyclophanes containing ethyleneoxy bridge by Glaser-Eglinton coupling, Heterocycles, 2015, 90, 1289 CrossRef CAS.
- J. Altmann, C. Jandl and A. Pöthig, Introducing a pyrazole/imidazole based hybrid cyclophane: a hydrogen bond sensor and binucleating ligand precursor, RSC Adv., 2015, 44, 11278 Search PubMed.
- Z. Rafiee Samani, A. M. Mehranpour and A. Hasaninejad, Preparation of 2,5-disubstituted pyrimidines from vinamidinium salts and synthesis of novel disulfane derivatives, J. Heterocycl. Chem., 2020, 57, 2150 CrossRef CAS.
- Z. Rafiee Samani and A. M. Mehranpour, Synthesis of novel 5-substituted isophthalates from vinamidinium salts, Tetrahedron Lett., 2019, 60, 151002 CrossRef CAS.
- E. Bahman Jahromi and A. M. Mehranpour, A novel synthesis of new 1,8-naphthyridine derivatives using the reaction of vinamidinium salts with 2,6-diaminopyridine, Org. Prep. Proced. Int., 2016, 48, 468 CrossRef.
- E. Bahman Jahromi, A. M. Mehranpour and N. Nowrouzi, Facile synthesis of novel 3-substituted pyrido[1,2-a]pyrimidinium salts using vinamidinium salts, Synth. Commun., 2016, 46, 1833 CrossRef.
- A. M. Mehranpour, S. Hashemnia and J. Ameri Rad, Synthesis of new metal-free 1,4,8,11-tetraaza[14]annulene derivatives using 2-heteroaryl-substituted trimethinium salts, J. Heterocycl. Chem., 2013, 50, 821 CrossRef CAS.
- A. M. Mehranpour, S. Hashemnia and R. Maghamifar, Synthesis and characterization of new γ-substituted pentamethine cyanine dyes, Synth. Commun., 2010, 40, 3594 CrossRef CAS.
- A. M. Mehranpour, S. Hashemnia and F. Azamifar, Synthesis of new malonaldehyde derivatives using 2-heteroaryl-substituted trimethinium salts, Tetrahedron Lett., 2013, 54, 321 CrossRef CAS.
- A. M. Mehranpour and M. Zahiri, Synthesis and characterization of new benzimidazole derivatives using 2-substituted 1,3-bis(dimethylamino)-trimethinium salts, Tetrahedron Lett., 2014, 55, 3969 CrossRef CAS.
- A. M. Mehranpour, S. Hashemnia and E. Bashiri, Synthesis of new dibenzo-tetraaza and dibenzo-dioxadiaza[14]annulene derivatives using 3-bromo-substituted vinamidinium salt, Synth. Commun., 2013, 43, 1931 CrossRef CAS.
- A. M. Mehranpour, Synthesis of new derivatives of 1,5,9,13-tetraaza[16]annulene using 2-substituted vinamidinium salts, Tetrahedron Lett., 2014, 55, 5229 CrossRef CAS.
Footnote |
† Electronic supplementary information (ESI) available. See DOI: 10.1039/d0ra10548a |
|
This journal is © The Royal Society of Chemistry 2021 |