DOI:
10.1039/D0RA10475B
(Paper)
RSC Adv., 2021,
11, 3003-3011
An efficient and practical aerobic oxidation of benzylic methylenes by recyclable N-hydroxyimide†
Received
13th December 2020
, Accepted 4th January 2021
First published on 14th January 2021
Abstract
An efficient and practical benzylic aerobic oxidation catalyzed by cheap and simple N-hydroxyimide organocatalyst has been achieved with high yields and broad substrate scope. The organocatalyst used can be recycled and reused by simple workup and only minute amount (1 mol% in most cases) of simple iron salt is used as promoter. Phenyl substrates with mild and strong electron-withdrawing group could also be oxygenated in high yields as well as other benzylic methylenes. Influence of substituents, gram-scale application, catalysts decay and general mechanism of this methodology has also been discussed.
1. Introduction
Phenyl ketones are widely used as active ingredients of pharmaceuticals, pesticides, flavors and so on, they can also be applied as organic building blocks for forming carbon–carbon and carbon–hetero bond (Fig. 1).1 An effective method for synthesizing aryl ketone is direct sp3 C–H oxidation of methylene compounds, especially for benzyl methylenes with m-positioning effect substituents (e.g. p-NO2, p-CN) on the ring, which are difficult to be synthesized through Friedel–Crafts acylation. A range of oxidants were used in methylene oxidations,2 of which oxygen is the most “green” one.
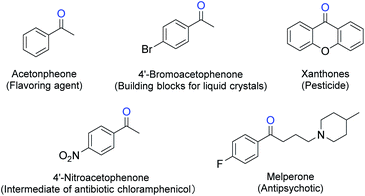 |
| Fig. 1 Examples of phenyl ketones as functional molecules or their intermediates. | |
Traditional homogenous aerobic oxidation of benzylic methylenes usually takes place at high temperature and high pressure with hazardous metal catalysis.3 Combined use of metal and organocatlyst could synergistically enhance the reaction efficiency thus reduce the temperature and pressure required in liquid aerobic oxidation.4
N-Hydroxyimides (NHIs) is one of the most promising organocatalysts for benzylic aerobic oxidation, it redrew interests among chemistry community recently.4a,5 The most studied NHIs is N-hydroxyphthalimide (NHPI), it has many merits such as easily-prepared, good catalytic performance, etc. However, there are several major defects that limit the industrial application of NHPI: (1) the self-decay at elevated temperature and prolonged reaction time, which causes comparatively large catalyst loading (usually 10–20 mol%) and makes it hardly recyclable;6 (2) requirement of hazardous metal (e.g. Co/Mn) to promote efficiency;4,7 (3) requirement of large amounts of polar organic solvent makes products and catalysts difficult to be isolated from reaction mixture;6 (4) hard to catalyze the aerobic benzylic sp3 C–H oxidation when there are electron-withdrawing substituents on phenyl ring.5–9
Among all drawbacks, decomposition of the organocatalyst in reaction condition is the major problem that makes catalyst not sustainable and influences its industrial application, although it is possible to recycle NHIs since N-hydroxy radical returns to NHIs molecule form during catalytic cycle.8 Thus, the key to make NHIs more practical and sustainable as catalyst in real oxidation is to find a combination of more stable N-hydroxyimide and comparatively “green” metal salts promoter, which could be beneficial to overcome the above obstacles.
Compared to many metals used for catalyzing aerobic benzylic oxidation,4,5,7 iron is cheap and comparatively harmless to both human and environment. Several reports of iron/organocatalyst combination cocatalyzed liquid-phase aerobic benzylic oxidation has been released recently. Miao group9a developed a Fe3+/NHPI catalyzed benzylic oxidation with O2 as oxidant (Scheme 1a). Although reaction condition is mild and no additive is needed, substrates with electron-withdrawing group (EWGs) only give moderate yields (54–63%) and catalyst loading is comparatively high without recycling. Humbeck et al.9b reported a selectivity-switchable oxidation of benzylic methylenes connects to phenyl or aza-heterocycles catalyzed by iron/ligand/NHIs or cobalt/NHPI. Products are mainly heterocyclic ketones with good selectivity when iron is used as catalyst (Scheme 1b). However, catalyst loading is high and yields are moderate, which makes the catalytic system not practical enough in industry. Recently, group of He9c released an iron/ligand/disulfide system for catalyzing benzylic aerobic oxidation of benzyl esters and amines (Scheme 1c). From the angle of green and sustainable synthesis,10 catalysts loading is high and each part of this catalytic system cannot be recovered.
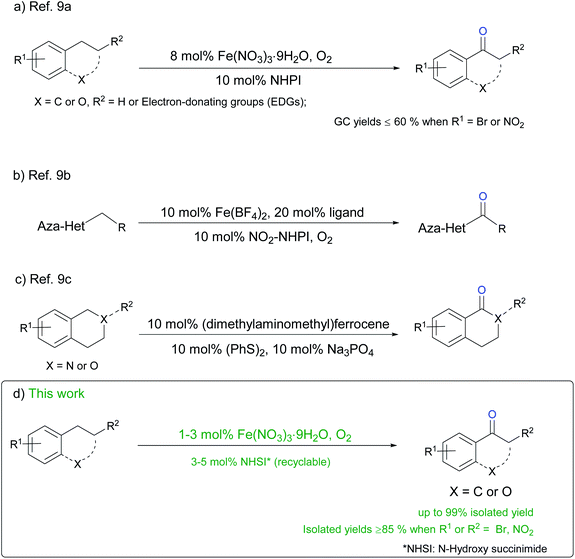 |
| Scheme 1 Homogeneous methylene aerobic oxidations catalyzed by organocatlyst/iron salts. | |
As discussed above, practicable and sustainable homogenous catalysis for aerobic benzylic oxidation remains one major challenge in synthetic chemistry. In this work, we present an efficient and practical methodology for benzyl methylene autoxidation with broad substrate scope and excellent organocatalyst recyclability (Scheme 1d).
2. Experimental section
2.1. Materials and instruments
All chemicals were purchased from commercial vendors (Bidepharm, Aladdin, Meryer, etc.). All reactions are performed in conventional oven-dried glassware. Analytical thin layer chromatography (TLC) was carried out using silica gel GF254. Gas chromatograph (GC) was performed at Shimadzu GC-2014C (capillary column: SE-54 type 30 m × 0.32 mm × 1.80 μm) with hydrogen ion flame detector. HPLC was performed on Waters 2695 (Waters 2996 detector, column: Diamonsil 5 um C18(2), 150 × 4.6 mm). NMR data was recorded by Bruker Avance III 500 MHz Spectrometer or Avance III HD 600 MHz Spectrometer, Chemical shifts (δ) are given in ppm.
2.2. General procedure of synthesis
Substrate (1, 2 mmol), Fe(NO3)3·9H2O (0.02 mmol in most cases, 8.08 mg), NHSI (0.06–0.1 mmol in most case, 6.90–11.52 mg) and PhCN (1–2 mL) are mixed at room temperature in a 10 mL flask with condenser (using liquid circulating cooler with ethanol as coolant at −20 °C) and O2 balloon. Mixture was stirred and heated in oil bath for several hours and monitored by GC or TLC until substrate is completely depleted (1a–1o) or not consumed any more (1p–1s). Then 10 mL water and 10 mL EtOAc were added to the reaction. The aqueous layer was wash by EtOAc (15 mL × 3 mL) and organic layer was collected. After removing EtOAc and part of PhCN under reduced pressure, the residue was isolated through column chromatography (EtOAc/PE) to get pure product.
2.3. Procedure of getting linear fitted curve and equation
2.3.1 NHPI. NHPI and diphenyl ether (PhOPh) were weighted (molar ration = 0.02, 0.05, 0.25, 0.3, 0.4, 0.45, 0.48, 0.5) and totally dissolved in large amount of methanol. These solutions are injected in to HPLC (methanol
:
water = 67
:
33 velocity of eluent = 1 mL min−1; column temperature: 30 °C) and the ration of peak area of NHPI
:
PhOPh were recorded (at 220 nm wave length) and linearly fitted to obtain regression line and regression equation (Fig. S2†).
2.3.2 NHSI. NHSI and PhOPh were weighted (molar ration = 0.02, 0.2, 0.4, 0.6, 0.8, 1.0) and totally dissolved in large amount of methanol. These solutions are injected into HPLC and the ration of peak area of NHSI
:
PhOPh were recorded (at 280 nm wave length) and linearly fitted to obtain regression line and regression equation (Fig. S3†).
2.4. Analysis procedure of NHPI and NHSI decomposition
EB (ethylbenzene, 1i, 2 mmol), Fe(NO3)3·9H2O (0.02 mmol), NHPI or NHSI (0.06 mmol), PhOPh (0.15 mmol) and PhCN (1 mL) are mixed at room temperature in a 10 mL flask with condenser (using liquid circulating cooler with ethanol as coolant at −20 °C for condensing) and O2 balloon. The mixture was heated and stirred in 90 °C oil bath. After certain hours, 2–3 drips of mixture form reaction (during stirring) was sampled with pipet. The sample was dissolved in 1 mL MeOH. Then the solution was diluted 100 times with MeOH and analyzed with HPLC according to its corresponding linear fitted regression equation (Fig. S2 or S3†).
2.5. Gram-scale synthesis of p-nitro acetophenone (2o)
p-Nitro EB (1o, 3 gram), Fe (2 mol%), NHSI (5 mol%), 15 mL PhCN are mixed at room temperature in a 50 mL-flask. The mixture was stirred and heated in 90 °C oil bath with condenser. The reaction was monitored by TLC until total depletion of 1o. Then the flask was connected with distillation device and PhCN was totally removed under vacuum at 70 °C oil bath. The regained solvent was directly used for next run of oxidation. After distillation, 50 mL water was added to the residue and stirred vigorously in room temperature for 10 min. Then filtration was performed, filter cake was wash with water (10 mL × 3 mL) and recrystallized in EtOAc/PE to give product 2o as yellow solid.
2.6. Catalyst and solvent recycling in gram-scale synthesis
After each run of gram synthesis, the flask was connected with distillation device and PhCN was totally removed under vacuum at 70 °C oil bath. The regained solvent was directly used for next run of oxidation. After distillation, 50 mL water was added to the residue and stirred vigorously in room temperature for 10 min. Then filtration was performed, the aqueous filtrate was evaporated and dried in vacuum to remove water. Substrate (1o, 3 gram), Fe (2 mol%), compensative PhCN (amount is shown in Table 3) was added to the residue and next run of oxidation was performed in 90 °C oil bath.
3. Results and discussion
3.1. Oxidation of ethyl benzene in the presence of different combination of iron salt and NHIs
We began our research by trying different combination of iron salt and NHIs with ethyl benzene (EB) as model substrate. As shown in Table 1, Fe(NO3)3 shows the best efficiency among iron salts tested (Table 1, entries 1–3). Nitrile solvents show great enhancing effect for this aerobic oxidation (Table 1, entries 4–7),11 and PhCN promotes this oxidation better than MeCN (Table 1, entries 6–7). Among all NHIs tested (Fig. S1 in ESI†), the cheapest and structurally-simplest two-NHPI and N-hydroxysuccinimide (NHSI)-show best catalytic activity (Table 1, entries 8–11, detailed screening are presented in Tables S1–S3 and Fig. S1 in ESI†), promoting us to further monitor their decomposition during aerobic oxidation.
Table 1 Screen of iron catalyst and solventa
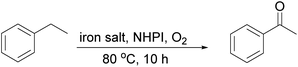
|
Entry |
Conditionb |
Catalyst |
NHIs |
Solvent |
Conversion (%) |
Reaction conditions: 2 mmol ethylbenzene and other reagents in 2 mL solvents are stirred at certain temperature in open system. Condition A: 10 mol% metal salts, 10 mol% NHPI, 100 °C oil bath for 10 h; condition B: 5 mol% metal salts, 5 mol% NHPI, 80 °C oil bath for 10 h; condition C: 5 mol% metal salts, 5 mol% NHIs, 90 °C oil bath for 10 h; condition D: 2 mol% metal salts, 2 mol% NHIs, 100 °C oil bath for 20 h. Conversion was determined by gas chromatography (GC) using area normalization method, numbers in the parentheses are selectivity toward acetophenone. |
1 |
A |
Iron tristearate |
NHPI |
AcOH |
78 |
2 |
A |
FeOH(CH3COO)2 |
NHPI |
AcOH |
80 |
3 |
A |
Fe(NO3)3·9H2O |
NHPI |
AcOH |
83 |
4 |
B |
Fe(NO3)3·9H2O |
NHPI |
AcOH |
23 |
5 |
B |
Fe(NO3)3·9H2O |
NHPI |
Toluene |
19 |
6 |
B |
Fe(NO3)3·9H2O |
NHPI |
CH3CN |
41 |
7 |
B |
Fe(NO3)3·9H2O |
NHPI |
PhCN |
57 |
8 |
C |
Fe(NO3)3·9H2O |
NHPI |
PhCN |
99 (99) |
9 |
C |
Fe(NO3)3·9H2O |
NHSI |
PhCN |
97 (99) |
10 |
D |
Fe(NO3)3·9H2O |
NHPI |
PhCN |
93 (99) |
11 |
D |
Fe(NO3)3·9H2O |
NHSI |
PhCN |
91 (99) |
3.2. Comparison of stability of NHPI and NHSI
As mentioned above, the main obstacle for industrial and scale-up application of NHPI in aerobic oxidation is difficulty for recycling due to its self-decomposition.6 Thus, we tried to compare the stability of NHSI and NHPI in reaction conditions. The decay of NHIs were usually studied in absence of substrate about their radical form, their molecular decomposition percentage when catalyzing aerobic oxidation are rarely reported5,6,12 probably due to the complexity of reaction mixture. After many trials of chromatography conditions, peaks of NHPI or NHSI and other byproducts from reaction mixture were separated well in HPLC. As shown in Fig. 2, NHSI decomposed much slower than NHPI in aerobic oxidation of ethyl benzene (EB). At this reaction condition (90 °C/1 mol% Fe(NO3)3/3 mol% NHIs/PhCN/O2), almost no NHPI detected in the mixture after 12 hours, while there are still more than 70% NHSI remain unchanged even after 35 hours.
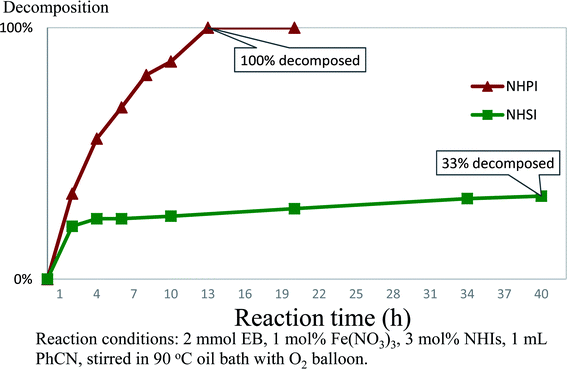 |
| Fig. 2 Self-decomposition curve of the orgnocatalysts in Fe(NO3)3 promoted aerobic oxidation of EB. | |
The main cause for self-decomposition of NHPI is its oligomerization to form dimer (13) or trimer (14) through PINO (phthalimide-N-oxyl radical, radical of NHPI, 11a) and acyl radical (12a) (Scheme 2).6d,12b,13 NHSI and NHPI are structurally different and so their radicals (one aromatic and the other aliphatic). The better stability of NHSI may possibly attribute to its aliphatic structure of radical (succinimide-N-oxyl radical, SINO, 11b) and acyl radical (12b), which are less stable when compared to those of NHPI (might be stabilized by aromatic conjugation). And thus, the rate of PINO oligomerization could be faster than SINO, which might be the main cause for faster decomposition of NHPI during catalyzing. Finally, NHSI is chosen as the organocatlyst for further optimization and application. NHSI was studied for aerobic benzylic oxidation before, while hazardous metal is necessary for co-catalyzation in all these reports.14 More importantly, advantages of NHSI as good stability and water solubility has never been exploited.
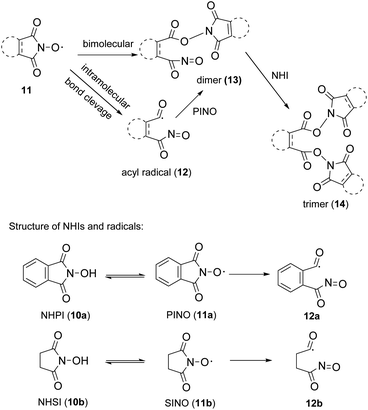 |
| Scheme 2 Possible pathway of self-decomposition of NHIs. | |
3.3. Optimization process of oxidation in the presence of NHSI
Next, catalyst loading, O2 source, amount of solvent, etc. was further optimized. After several trials (Tables 2 and S4 in ESI†) we found that only 1% Fe salts, 3 mol% NHSI and O2 balloon as oxygen source is sufficient for this oxidation. Besides, requirement of large amounts of VOC (volatile organic compounds) as solvent is also one major limitation for NHIs' industrial application. Although use of high boiling point VOC as reaction medium already decreases loss from volatilization during heating, we also tried to minimize the amount of PhCN used and it turns out that 1 mL for 2 mmol EB (5 eq.) is enough (Table 2, entries 2–3) for this oxidation. Different from reported Fe(NO3)3-catalyzed oxidation,15 KPF6 as additive shows no promotive effect in our system (Table 2, entry 5). Finally, 1 mmol% Fe(NO3)3/3 mmol% NHSI together as catalysts, 1 mL PhCN as solvent and O2 balloon as oxygen source (Table 2, entry 4) was chosen as the optimized condition for substrate scope expending.
Table 2 Further optimization of Fe(NO3)3/NHSI/O2 systema
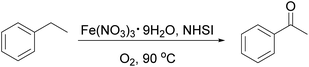
|
Entry |
Fe(NO3)3·9H2O (mol%) |
NHSI (mol%) |
PhCN (mL) |
Oxygen Sourceb |
Time (h) |
Yieldc (%) |
2 mmol ethylbenzene, Fe(NO3)3·9H2O and NHSI in PhCN are stirred in 90 °C oil bath. Oxygen source condition: A: air (open system); B: O2 balloon without purging. Determined by GC using diphenyl ether as internal standard. 20 mmol% KPF6 was added as additive. |
1 |
1 |
1 |
2 |
A |
24 |
57 |
2 |
1 |
3 |
2 |
A |
18 |
86 |
3 |
1 |
3 |
1 |
A |
16 |
86 |
4 |
1 |
3 |
1 |
B |
14 |
90 |
5d |
1 |
3 |
1 |
B |
16 |
89 |
As shown in Scheme 3, various substrates can be oxidized to their corresponding ketones. Methylenes between two aryl groups transform to diphenyl ketones in high yields (2a–2e), even with hetero-atom in ring or between two rings (2d–2e). Xanathon (2d) could function as ovicide, larvicide or other bioactive usage (Fig. 1).1e Benzo-(hetero)aliphatic alkanes are also oxidized to benzocyclic ketones efficiently (2f–2h). These results offer a new option for synthesizing (di)benzo(hetero)cyclic ketones. Also, different from most reported iron-catalyzed ligand-free liquid aerobic oxidations,9,15 chain-alkyl benzene are oxidized with high yields (more than 80%) in this system (2i–2j). Remarkably, EB derivatives with mild and strong electron-withdrawing group (EWG) can transformed to ketones with excellent yields in comparatively mild conditions (2k–2o), which distinguish this methodology from most reported NHIs-catalyzed benzylic aerobic oxidations. And bromo substituted aryl ketones are synthesized with very high yields (≥97%, 2k–2m), these ketones are useful building blocks in manufacturing functional molecules.1,16 To our surprise, EB derivatives with electron-donating group (EDG) undergo oxidation more difficultly than ones with EWG, even with higher catalyst loading and at elevated reaction temperature (2p–2s), the reason is still unclear and needs to be further studied.
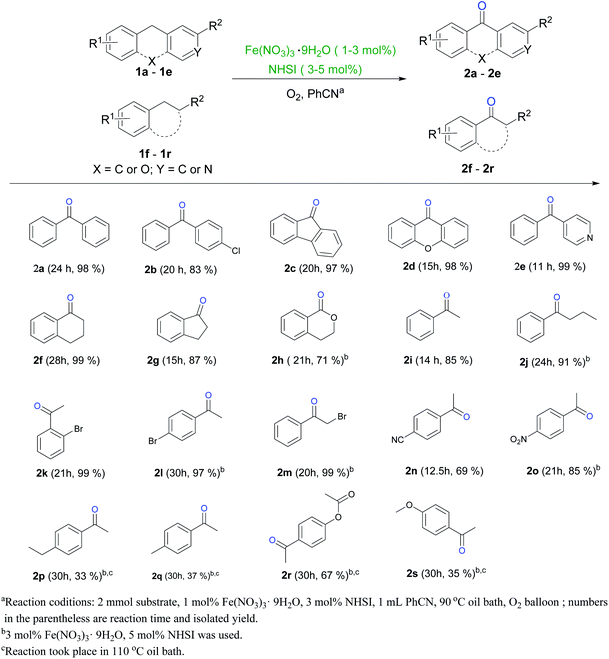 |
| Scheme 3 Substrate expension of this aerobic oxidation system. | |
3.4. Catalytic potential of NHSI
Subsequently, to further validate the applicability and catalysts recyclability of this methodology, we tried gram-scale application of this system in oxidation of p-nitro EB (1o) to p-nitro acetophenone (2o). 2o is widely used as the intermediate for producing chloramphenicol (Fig. 1) and other pharmaceutical intermediates,17,18 its industrial synthesis usually includes Co or Mn catalyzed aerobic oxidation of p-nitro EB in high oxygen pressure (1o).18 While in our attempt, 3 g 1o was oxidized to 2o in atmospheric pressure with good yield (Table 3). Markedly, NHSI can be isolated from reaction mixture simply by washing the mixture with water due to its good water-solubility. And it can be reused without significant loss of activity (Table 3, entries 4–6), which is in according with the results of decay monitoring (Fig. 2). Although direct use of dehydrated aqueous filtrate from reaction mixture as catalyst didn't show enough activity (Table 3, entry 2), adding 2 mmol% Fe(NO3)3 is sufficient for next run of oxidation (Table 3, entries 2–3). This phenomenon also indicates that iron salts has decomposed during oxidation. Of note, product (2o) is isolated form NHSI aqueous solution by simple filtration without any chromatography procedure in each run. Besides, reaction medium (PhCN) could be regained by simple distillation under reduced pressure with a little loss. The regained solvent could be directly used in next run of rection, although extra PhCN is needed to compensate the loss from distillation, otherwise side-products appears before substrate exhausts, which probably is due to over-concentrated substrate and catalyst in mixture (Table 3, entries 3–4).
Table 3 Synthesis of 2o with NHSI recyclinga
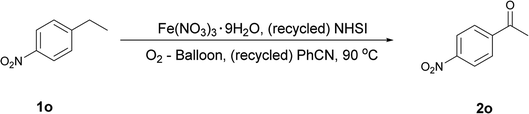
|
Entry |
Recycle times |
Reaction time (h) |
PhCN addedb (mL) |
Isolated yields (%) |
Reaction conditions: 3 g 1o, 2 mol% Fe(NO3)3·9H20, 5 mol% NHSI and 15 mL PhCN was mixed together and heated at 90 °C oil bath. The amount of extra PhCN was added before heating. 2 mmol% Fe(NO3)3 was added before heating. |
1 |
0 |
21 |
— |
83 |
2 |
1 |
48 |
2 |
51 |
3c |
1 |
24 |
0 |
61 |
4c |
1 |
24 |
2 |
82 |
5c |
2 |
27 |
3 |
79 |
6c |
3 |
34 |
5 |
75 |
3.5. Possible oxidation mechanism in the presence of NHSI
To preliminarily look into the mechanism, we performed several controlled reactions (Scheme 4 and Table S5 in ESI†). Visible light has no influence to the reaction (eqn (1), Scheme 4). NHSI or Fe(NO3)3 solely cannot efficiently catalyze the oxygenation (eqn (2) and (3)), while two catalysts together catalyze the aerobic oxidation synergistically (eqn (1)). And comparison of eqn (1) and (4), (5) indicates that NO3− is necessary for efficiency of Fe(NO3)3 as well as Fe3+ when NHSI is present. Besides, when radical scavenger BHT (2,6-di-tert-butyl-4-methylphenol) was added, oxidation was retarded. And the conversion of substrate decreased when more BHT is added, which indicates this oxidation proceeds though radical pathways (Table S5 in ESI†).
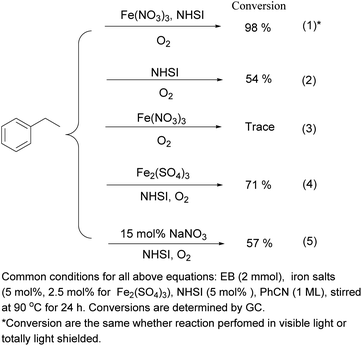 |
| Scheme 4 Controlled experiments of EB aerobic oxidation. | |
Based on results above with viewpoints of reported literature,5–8,12,19 possible mechanism is shown on Scheme 5. The radical process is initiated by Fe(NO3)3 with NHSI. SINO generated then abstract hydrogen from substrate to generate benzyl radical. And with the participation of oxygen, benzylic methylene of substrate is finally oxidized to ketones through peroxy intermediate. Each part of catalyst combination (Fe3+/NO3−/NHSI) is necessary for the oxidative activity, these three components synergistically catalyze aerobic oxidation of phenyl alkanes. The radical intermediate SINO can return to its original NHSI form, which outweigh its self-decomposition.
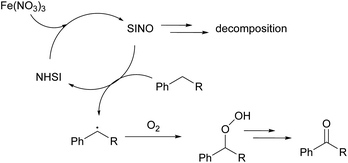 |
| Scheme 5 Possible mechanism of Fe(NO3)3/NHSI catalyzed aerobic oxidation. | |
4. Conclusions
In conclusion, an efficient and practical aerobic oxidation methodology is developed. Several advantages of this method might be emphasized and summarized as follows (compared to each corresponding drawback described in 3rd paragraph): (1) only 3–5 mol% commercially available organocatalyst-NHSI was used and it can be recovered with simple workup (washed with water) and reused without significant loss of activity; (2) only 1 mol% iron salts is used as promoter in most cases; (3) comparatively small amount (1 mL for 2 mmol substrate) of high-boiling point VOC is used as reaction medium with good recovery and product could possibly be isolated by simple procedure without chromatography (2o as showcase); (4) substrates with EWGs on phenyl ring can also be converted to its corresponding ketones efficiently. Further study of detailed mechanism and stability of other NHIs in different reaction conditions are currently ongoing in our group.
Conflicts of interest
There are no conflicts to declare.
Acknowledgements
This study was financially supported by Zhejiang Provincial Natural Science Foundation (No. LY21H300003), Department of Education of Zhejiang Province (No. Y201942551), Start-Up Funding from Hangzhou Medical College (No. 00004F1RCYJ1804).
Notes and references
-
(a) J. Barbera, R. Gimenez, J. L. Serrano, R. Alcala, B. Villacampa, J. Villalba, I. Ledoux and J. Zyss, Beta-diketone, pyrazole and isoxazole derivatives with polar groups: liquid crystalline and non-linear optical properties, Liq. Cryst., 1997, 22, 265 CrossRef CAS;
(b) C. T. Mbofana, E. Chong, J. Lawniczak and M. S. Sanford, Iron-Catalyzed Oxyfunctionalization of Aliphatic Amines at Remote Benzylic C–H Sites, Org. Lett., 2016, 18, 4258 CrossRef CAS;
(c) G. P. Romanelli, E. G. Virla, P. R. Duchowicz, A. L. Gaddi, D. M. Ruiz, D. O. Bennardi, E. Del Valle Ortiz and J. C. Autino, Sustainable Synthesis of Flavonoid Derivatives, QSAR Study and Insecticidal Activity against the Fall Armyworm, Spodoptera frugiperda (Lep.: Noctuidae), J. Agric. Food Chem., 2010, 58, 6290 CrossRef CAS;
(d) G. Schmaus, S. Lange and M. Pesaro, Flavour and fragrance compositions comprising acetophenone derivatives, Symrise AG, United States, 2015 Search PubMed;
(e) S. Basu and M. Mukherjee, in Reference Module in Materials Science and Materials Engineering, Elsevier, 2018, DOI:10.1016/b978-0-12-803581-8.11395-5.
-
(a) X. Han, Z. Zhou, C. Wan, Y. Xiao and Z. Qin, Co(acac)2-Catalyzed Allylic and Benzylic Oxidation by tert-Butyl Hydroperoxide, Synthesis, 2013, 45, 615 CrossRef CAS;
(b) Y. Hu, L. Zhou and W. Lu, Transition-Metal- and Halogen-Free Oxidation of Benzylic sp3 C–H Bonds to Carbonyl Groups Using Potassium Persulfate, Synthesis, 2017, 49, 4007 CrossRef CAS;
(c) M. R. Maurya, B. Sarkar, A. Kumar, N. Ribeiro, A. Miliute and J. C. Pessoa, New thiosemicarbazide and dithiocarbazate based oxidovanadium(iv) and dioxidovanadium(v) complexes. Reactivity and catalytic potential, New J. Chem., 2019, 43, 17620 RSC;
(d) K. N. Parida, S. Jhulki, S. Mandal and J. N. Moorthy, Oxidation of benzyl alcohols, benzyl halides, and alkylbenzenes with oxone, Tetrahedron, 2012, 68, 9763 CrossRef CAS.
-
(a) S. D. I. G. W. Parshall, Heterogeneous Catalysis, Wiley, New York, 2nd edn, 1992 Search PubMed;
(b) M. T. Musser, in Ullmann's Encyclopedia of Industrial Chemistry, 2012, DOI:10.1002/14356007.a08_217.pub2;
(c) C. S. Park and J. R Sheehan, Kirk-Othmer Encyclopedia of Chemical Technology, Wiley, New York, 4th edn, 1996 Search PubMed;
(d) W. Partenheimer, Methodology and scope of metal/bromide autoxidation of hydrocarbons, Catal. Today, 1995, 23, 69 CrossRef CAS.
-
(a) D. P. Hruszkewycz, K. C. Miles, O. R. Thiel and S. S. Stahl, Co/NHPI-mediated aerobic oxygenation of benzylic C–H bonds in pharmaceutically relevant molecules, Chem. Sci., 2017, 8, 1282 RSC;
(b) L. Schmieder-van de Vondervoort, S. Bouttemy, F. Heu, K. Weissenböck and P. 'L. Alsters, Low Temperature, High Conversion, Liquid-Phase Benzylic Oxidation with Dioxygen by Metal/NHPI-Catalyzed Co-Oxidation with Benzaldehyde, Eur. J. Org. Chem., 2003, 2003, 578 CrossRef;
(c) B. B. Wentzel, M. P. Donners, P. L. Alsters, M. C. Feiters and R. J. Nolte, N-Hydroxyphthalimide/cobalt(II) catalyzed low temperature benzylic oxidation using molecular oxygen, Tetrahedron, 2000, 56, 7797 CrossRef CAS;
(d) Y. Yoshino, Y. Hayashi, T. Iwahama, S. Sakaguchi and Y. Ishii, Catalytic Oxidation of Alkylbenzenes with Molecular Oxygen under Normal Pressure and Temperature by N-Hydroxyphthalimide Combined with Co(OAc)2, J. Org. Chem., 1997, 62, 6810 CrossRef CAS.
-
(a) M. A. Betiha, N. G. Kandile, A. M. Badawi, S. M. Solyman and A. S. Afify, Oxidation of p-toluic acid to terephthalic acid via a bromine-free process using nano manganese and manganese-copper mixed oxides, New J. Chem., 2018, 42, 6343 RSC;
(b) G. Dobras, M. Sitko, M. Petroselli, M. Caruso, M. Cametti, C. Punta and B. Orlińska, Solvent-Free Aerobic Oxidation of Ethylbenzene Promoted by NHPI/Co(II) Catalytic System: The Key Role of Ionic Liquids, ChemCatChem, 2020, 12, 259 CrossRef CAS;
(c) O. Kushch, I. Hordieieva, K. Novikova, Y. Litvinov, M. Kompanets, A. Shendrik and I. Opeida, Kinetics of N-oxyl Radicals' Decay, J. Org. Chem., 2020, 85, 7112 CrossRef CAS;
(d) H. Wang, J. Liu, J.-P. Qu and Y.-B. Kang, Overcoming Electron-Withdrawing and Product-Inhibition Effects by Organocatalytic Aerobic Oxidation of Alkylpyridines and Related Alkylheteroarenes to Ketones, J. Org. Chem., 2020, 85, 3942 CrossRef CAS.
-
(a) K. Chen, J. Yao, Z. Chen and H. Li, Structure-reactivity landscape of N-hydroxyphthalimides with ionic–pair substituents as organocatalysts in aerobic oxidation, J. Catal., 2015, 331, 76 CrossRef CAS;
(b) Y. Ishii, S. Sakaguchi and T. Iwahama, Innovation of Hydrocarbon Oxidation with Molecular Oxygen and Related Reactions, Adv. Synth. Catal., 2001, 343, 393 CrossRef CAS;
(c) L. Melone, S. Prosperini, G. Ercole, N. Pastori and C. Punta, Is it possible to implement N-hydroxyphthalimide homogeneous catalysis for industrial applications? A case study of cumene aerobic oxidation, J. Chem. Technol. Biotechnol., 2014, 89, 1370 CrossRef CAS;
(d) F. Recupero and C. Punta, Free Radical Functionalization of Organic Compounds Catalyzed by N-Hydroxyphthalimide, Chem. Rev., 2007, 107, 3800 CrossRef CAS;
(e) B. B. Wentzel, M. P. J. Donners, P. L. Alsters, M. C. Feiters and R. J. M. Nolte, N-Hydroxyphthalimide/Cobalt(II) Catalyzed Low Temperature Benzylic Oxidation Using Molecular Oxygen, Tetrahedron, 2000, 56, 7797 CrossRef CAS;
(f) L. Melone and C. Punta, Liquid Phase Aerobic Oxidation Catalysis: Industrial Applications and Academic Perspectives, Wiley-VCH, Weinheim, 2016 Search PubMed.
-
(a) W. Deng, W.-P. Luo, Z. Tan, Q. Liu, Z.-M. Liu and C.-C. Guo, Remarkable effect of simple aliphatic alcohols on the controlled aerobic oxidation of toluene catalyzed by (T(p-Cl)PP) MnF/NHPI, J. Mol. Catal. A: Chem., 2013, 372, 84 CrossRef CAS;
(b) B. Orlińska, N-Hydroxyphthalimide in combination with Cu(II), Co(II) or Mn(II) salts as catalytic systems for the oxidation of isopropyl-aromatic hydrocarbons with oxygen, Tetrahedron Lett., 2010, 51, 4100 CrossRef;
(c) R. D. Patil, B. Fuchs, N. Taha and Y. Sasson, Solvent-free and Selective Autooxidation of Alkylbenzenes Catalyzed by Co/NHPI under Phase Transfer Conditions, ChemistrySelect, 2016, 1, 3791 CrossRef CAS;
(d) Y. L. Tnay and S. Chiba, Copper-Catalyzed Aerobic CC Bond Cleavage of Lactols with N-Hydroxy Phthalimide for Synthesis of Lactones, Chem.–Asian J., 2015, 10, 873 CrossRef CAS.
-
(a) J. Liu, K.-F. Hu, J.-P. Qu and Y.-B. Kang, Organopromoted selectivity-switchable synthesis of polyketones, Org. Lett., 2017, 19, 5593 CrossRef CAS;
(b) I. Yavari and E. Karimi, N-Hydroxyphthalimide-catalyzed oxidative production of phthalic acids from xylenes using O2/HNO3 in an ionic liquid, Synth. Commun., 2009, 39, 3420 CrossRef CAS;
(c) Y. Yoshino, Y. Hayashi, T. Iwahama, S. Sakaguchi and Y. Ishii, Catalytic oxidation of alkylbenzenes with molecular oxygen under normal pressure and temperature by N-hydroxyphthalimide combined with Co (OAc)2, J. Org. Chem., 1997, 62, 6810 CrossRef CAS.
-
(a) C. Miao, H. Zhao, Q. Zhao, C. Xia and W. Sun, NHPI and ferric nitrate: a mild and selective system for aerobic oxidation of benzylic methylenes, Catal. Sci. Technol., 2016, 6, 1378 RSC;
(b) J. C. Cooper, C. Luo, R. Kameyama and J. F. Van Humbeck, Combined iron/hydroxytriazole dual catalytic system for site selective oxidation adjacent to azaheterocycles, J. Am. Chem. Soc., 2018, 140, 1243 CrossRef CAS;
(c) S. Geng, B. Xiong, Y. Zhang, J. Zhang, Y. He and Z. Feng, Thiyl radical promoted iron-catalyzed-selective oxidation of benzylic sp3 C–H bonds with molecular oxygen, Chem. Commun., 2019, 55, 12699 RSC.
-
(a) P. Anastas and N. Eghbali, Green Chemistry: Principles and Practice, Chem. Soc. Rev., 2010, 39, 301 RSC;
(b) P. T. Anastas and J. C. Warner, Green Chemistry: Theory and Practice, Oxford University Press, New York, 1998 Search PubMed;
(c) B. Török, in Green Chemistry, ed. B. Török and T. Dransfield, Elsevier, 2018, p. 49, DOI:10.1016/b978-0-12-809270-5.00003-0.
-
(a) Y. Ishii, T. Iwahama, S. Sakaguchi, K. Nakayama and Y. Nishiyama, Alkane Oxidation with Molecular Oxygen Using a New Efficient Catalytic System:
N-Hydroxyphthalimide (NHPI) Combined with Co(acac)n (n = 2 or 3), J. Org. Chem., 1996, 61, 4520 CrossRef CAS;
(b) F. Liang, W. Zhong, L. Xiang, L. Mao, Q. Xu, S. R. Kirk and D. Yin, Synergistic hydrogen atom transfer with the active role of solvent: preferred one-step aerobic oxidation of cyclohexane to adipic acid by N-hydroxyphthalimide, J. Catal., 2019, 378, 256 CrossRef CAS. -
(a) R. Amorati, M. Lucarini, V. Mugnaini, G. F. Pedulli, F. Minisci, F. Recupero, F. Fontana, P. Astolfi and L. Greci, Hydroxylamines as Oxidation Catalysts:
Thermochemical and Kinetic Studies, J. Org. Chem., 2003, 68, 1747 CrossRef CAS;
(b) P. Astolfi, P. Brandi, C. Galli, P. Gentili, M. F. Gerini, L. Greci and O. Lanzalunga, New mediators for the enzyme laccase: mechanistic features and selectivity in the oxidation of non-phenolic substrates, New J. Chem., 2005, 29, 1308 RSC;
(c) C. Ueda, M. Noyama, H. Ohmori and M. Masui, Reactivity of Phthalimide-N-oxyl: A Kinetic Study, Chem. Pharm. Bull., 1987, 35, 1372 CrossRef CAS. -
(a) N. Koshino, B. Saha and J. H. Espenson, Kinetic Study of the Phthalimide N-Oxyl Radical in Acetic Acid. Hydrogen Abstraction from Substituted Toluenes, Benzaldehydes, and Benzyl Alcohols, J. Org. Chem., 2003, 68, 9364 CrossRef CAS;
(b) Y. Cai, N. Koshino, B. Saha and J. H. Espenson, Kinetics of Self-Decomposition and Hydrogen Atom Transfer Reactions of Substituted Phthalimide N-Oxyl Radicals in Acetic Acid, J. Org. Chem., 2005, 70, 238 CrossRef CAS;
(c) R. Amorati, M. Lucarini, V. Mugnaini and G. F. Pedulli, Hydroxylamines as Oxidation Catalysts: Thermochemical and Kinetic Studies, J. Org. Chem., 2003, 68, 1747 CrossRef CAS;
(d) S. A. Hussain, T. C. Jenkins and M. J. Perkins, Oxidations with acylnitroxyls, Tetrahedron Lett., 1977, 36, 319 Search PubMed;
(e) E. Baciocchi, M. F. Gerini and O. Lanzalunga, Reactivity of Phthalimide N-Oxyl Radical (PINO) toward the Phenolic O–H Bond. A Kinetic Study, J. Org. Chem., 2004, 69, 8963 CrossRef CAS.
-
(a) I. K. Goncharova, K. P. Silaeva, A. V. Arzumanyan, A. A. Anisimov, S. A. Milenin, R. A. Novikov, P. N. Solyev, Y. V. Tkachev, A. D. Volodin and A. Korlyukov, Aerobic Co-/N-Hydroxysuccinimide-Catalyzed Oxidation of p-Tolylsiloxanes to p-Carboxyphenylsiloxanes: Synthesis of Functionalized Siloxanes as Promising Building Blocks for Siloxane-Based Materials, J. Am. Chem. Soc., 2019, 141, 2143 CrossRef CAS;
(b) M. A. Betiha, N. G. Kandile, A. M. Badawi, S. M. Solyman and A. S. Afify, Oxidation of p-toluic acid to terephthalic acid via a bromine-free process using nano manganese and manganese-copper mixed oxides, New J. Chem., 2018, 42, 6343 RSC;
(c) H. Falcon, J. M. Campos-Martin, S. M. Al-Zahrani and J. J. C. C. Fierro, Liquid-phase oxidation of p-xylene using N-hydroxyimides, Catal. Commun., 2010, 12, 5 CrossRef CAS.
- C. Hong, J. Ma, M. Li, L. Jin, X. Hu, W. Mo, B. Hu, N. Sun and Z. Shen, Ferric nitrate-catalyzed aerobic oxidation of benzylic sp3 C–H bonds of ethers and alkylarenes, Tetrahedron, 2017, 73, 3002 CrossRef CAS.
-
(a) L. Chen, H. Chen, P. Chen, W. Zhang, C. Wu, C. Sun, W. Luo, L. Zheng, Z. Liu and G. Liang, Development of 2-amino-4-phenylthiazole analogues to disrupt myeloid differentiation factor 88 and prevent inflammatory responses in acute lung injury, Eur. J. Med. Chem., 2019, 161, 22 CrossRef CAS;
(b) X. Dou, H. Huang, L. Jiang, G. Zhu, H. Jin, N. Jiao, L. Zhang, Z. Liu and L. Zhang, Rational modification, synthesis and biological evaluation of 3,4-dihydroquinoxalin-2(1H)-one derivatives as potent and selective c-Jun N-terminal kinase 3 (JNK3) inhibitors, Eur. J. Med. Chem., 2020, 201, 112445 CrossRef CAS;
(c) I. S. Hsieh, B. Gopula, C.-C. Chou, H.-Y. Wu, G.-D. Chang, W.-J. Wu, C.-S. Chang, P.-C. Chu and C. S. Chen, Development of Novel Irreversible Pyruvate Kinase M2 Inhibitors, J. Med. Chem., 2019, 62, 8497 CrossRef CAS;
(d) J. Lee, N. B. Vinh, N. Drinkwater, W. Yang, K. Kannan Sivaraman, L. S. Schembri, M. Gazdik, P. M. Grin, G. S. Butler, C. M. Overall, S. A. Charman, S. McGowan and P. J. Scammells, Novel Human Aminopeptidase N Inhibitors: Discovery and Optimization of Subsite Binding Interactions, J. Med. Chem., 2019, 62, 7185 CrossRef CAS;
(e) B. Narayana, K. K. Vijaya Raj, B. V. Ashalatha, N. S. Kumari and B. K. Sarojini, Synthesis of some new 5-(2-substituted-1,3-thiazol-5-yl)-2-hydroxy benzamides and their 2-alkoxy derivatives as possible antifungal agents, Eur. J. Med. Chem., 2004, 39, 867 CrossRef CAS.
-
(a) H. Rajak, R. Deshmukh, R. Veerasamy, A. K. Sharma, P. Mishra and M. D. Kharya, Novel semicarbazones based 2,5-disubstituted-1,3,4-oxadiazoles: one more step towards establishing four binding site pharmacophoric model hypothesis for anticonvulsant activity, Bioorg. Med. Chem. Lett., 2010, 20, 4168 CrossRef CAS;
(b) R. Sun, Y. Li, M. Lü, L. Xiong and Q. Wang, Synthesis, larvicidal activity, and SAR studies of new benzoylphenylureas containing oxime ether and oxime ester group, Bioorg. Med. Chem. Lett., 2010, 20, 4693 CrossRef CAS;
(c) K. Zilbeyaz and E. B. Kurbanoglu, Highly enantiomeric reduction of acetophenone and its derivatives by locally isolated Rhodotorula glutinis, Chirality, 2010, 22, 849 CrossRef CAS.
-
(a) N. G. Digurov, A. B. Shelud'ko, B. V. Nelyubin, T. V. Bukharkina and Y. V. Erofeev, p-Nitroacetophenone, Moscow Chemical-Technological Institute, USSR, 1987 Search PubMed;
(b) X. Guo, B. Han and J.-X. Shen, A pioneer of the Chinese pharmaceutical industry, Protein Cell, 2016, 7, 545 CrossRef;
(c) Y. She, Y. Yang and G. Li, CN109046458A, Zhejiang University of Technology, Peop. Rep. China, 2018, p. 14;
(d) C.-C. Shen, G.-H. Guan, C.-T. Young, G.-S. Liu, J. Z. Tsai, G.-I. Zhu, T.-F. Wang, C.-G. Young and T.-G. Lee, Synthesis of chloramphenicol. III. Autoxidation of p-nitroethylbenzene, a novel method for the preparation of p-nitroacetophenone, Acta Pharmacol. Sin., 1958, 6, 210 CAS;
(e) Y. Yang, G. Li, X. Mao and Y. She, Selective Aerobic Oxidation of 4-Ethylnitrobenzene to 4-Nitroacetophenone Promoted by Metalloporphyrins, Org. Process Res. Dev., 2019, 23, 1078 CrossRef CAS.
-
(a) N. Kuznetsova, L. Kuznetsova, O. Yakovina, I. Karmadonova and B. Bal'zhinimaev, An Improved Catalytic Performance of Fe (III)-promoted NHPI in the Oxidation of Hydrocarbons to Hydroperoxides, Catal. Lett., 2020, 150, 1020 CrossRef CAS;
(b) G. Tang, Z. Gong, W. Han and X. Sun, Visible light mediated aerobic photocatalytic activation of CH bond by riboflavin tetraacetate and N-hydroxysuccinimide, Tetrahedron Lett., 2018, 59, 658 CrossRef CAS.
Footnotes |
† Electronic supplementary information (ESI) available. See DOI: 10.1039/d0ra10475b |
‡ These authors contributed equally to this work. |
|
This journal is © The Royal Society of Chemistry 2021 |
Click here to see how this site uses Cookies. View our privacy policy here.