DOI:
10.1039/D0RA10208C
(Paper)
RSC Adv., 2021,
11, 4515-4522
Understanding the effect of thiophene sulfur on brominated petroleum coke for elemental mercury capture from flue gases
Received
3rd December 2020
, Accepted 3rd January 2021
First published on 22nd January 2021
Abstract
Developing highly efficient and inexpensive adsorbent is a critical technology for elemental Hg removal from the coal combustion flue gases worldwide. Here, we present a novel approach that a waste by-product of petroleum coke containing organic sulfur enhanced bromine binding during the bromine modification process and the brominated petroleum coke increased its mercury adsorption. Experiments and density functional theory reveal that the mercury adsorption capacity directly correlated with the surface organic sulfur and the binding bromine content. Our direct observations and theoretical modeling demonstrate that HgBr and Hg(Br) Br are the primary chemical forms chemisorbed on the surface of this new carbon-based sorbent, which is approaching to bind on the carbon site next to the S atom. The synergetic effect of the inherent thiophene sulfur and loaded bromine enhanced the Hg removal efficiency of the adsorbent.
1. Introduction
Industrial smog, containing SO2, NOx, mercury, particulates, and other trace elements, has become a significant issue to attract worldwide attention.1 One of the largest sources of smog is the coal combustion, from which elemental Hg is one of the most problematic elements. Because of the volatile and persistent nature of elemental Hg, it is eventually deposited in the lakes and rivers, and bio-accumulated in fish to cause serious threat to the public health by eating contaminated fish. Much work has been conducted to investigate the best method to reduce pollutants; moreover, government regulations were set to reduce the pollutants and the impact on the environment.2 Activated carbon (AC)-based sorbents have been proven to be the best approach to remove Hg from flue gases, and they are used currently in many power plants in North America.3 However, alternative sorbents have been studied to replace the activated carbon due to its high price and large demanding quantity to decrease the elemental Hg removal efficiency. Modified low-cost carbon-based sorbents, including fly ash and biomass ash, were developed with the bromine-impregnated and sulfur-impregnated ones showing the best effect to eliminate Hg from the coal combustion flue gas.4–13 Elemental sulfur was reported as a promising “soft” oxidant as a catalyst.14
Petroleum coke is formed as the final by-product of the petroleum industry during refining of crude bitumen or heavy oil. It is classified in the European Waste Catalogue with the code 050116,15 with large quantity produced worldwide. The certain technological reuse of the waste has globally become a problem, a portion of which has been used as graphite precursors, electrodes in aluminum, steel plants and even as inferior fuel. The high sulfur content limited the reuse application of petroleum coke. To date, certain researchers have used it as a precursor for preparing high surface area activated carbon because it is inexpensive and contains high carbon content.16–20
It was reported that organic sulfur, nitrogen, and oxygen were mainly in the forms of thiophene-containing polycyclic aromatic hydrocarbon, polycyclic aromatic hydrocarbons containing pyrrole or pyridine, and C
O or C–O–C, respectively in the structure of petroleum coke.21 Furthermore, >90% of sulfur was reported to be thiophene sulfur in the contents of petroleum coke.21 In our previous studies, petroleum coke has been obtained as a useful precursor to be modified with bromine for the adsorption of Hg from the flue gas.22 Both mercury reaction chemistry and macroscopic dynamic mechanism were studied, from where the synergetic effect of the bonded bromine and inherent organic sulfur was inferred for enhancing Hg adsorption.23,24 However, the micro-mechanism of the organic thiophene-sulfur affecting the bromine binding and elemental mercury binding continues to be unclear.
In this study, the petroleum coke material containing 5.85 wt% sulfur was obtained from the upgrading of oil sands deposits in Alberta, Canada, to make brominated carbon-based adsorbent for elemental Hg removal from the coal combustion flue gas.23 The bromine and Hg binding chemistry is analyzed through XRD, FTIR and XPS, thermal stability of Br and Hg in the adsorbent is performed to secure the potential regular correlation of them; however, the reaction pathways and mechanisms, nature of the active petroleum coke sites and the effects of thiophene-sulfur were performed by first-principles density functional theory (DFT) calculations to help understand the significant role of thiophene-sulfur in the petroleum coke for bromine-loading and elemental Hg capture.
2. Experimental
2.1. Materials preparation
The brominated petroleum coke adsorbent was prepared with a detailed description in our previous papers.22,23 The bromine modification process is known as the chemical-mechanical bromination process. The obtained petroleum coke was grounded using a ball-mill and sieved with a 100-mesh sieve, and then dried in a vacuum oven at 110 °C overnight. The prepared raw petroleum coke and liquid bromine with the weight ratio of 100
:
1 were placed in a sealed container, which was then tumbled for 30 min. After that, the received sample was placed in a vacuum oven at 200 °C for 30 min, and named as Br-PC.
Brominated petroleum coke (Br-PC) was heated to different temperatures of 200, 400, 600, 800 and 950 °C for 30 min under a nitrogen flow of 60 mL min−1. The resulting thermal treated samples were saturated with elemental Hg to investigate the mercury adsorption capacity through the mercury saturation procedure. The thermally treated samples were spread over the bottom of a glass vessel, with a smaller glass container containing elemental Hg being placed in the center of the vessel. The glass vessel should be well sealed and set in a fume hood. After a week, the Hg-saturated sorbents were tested by XPS to secure the adsorption mechanism.
2.2 Sorbent characterization
The surface morphology of the petroleum coke before and after the bromine loading was observed using a scanning electron microscope (SEM, Hitachi S-4800, Japan). The surface functional groups were measured by a X-ray diffractometer (XRD, Bruker AXS) and Fourier transform infrared spectrometer (FTIR, Nicolet iS5) produced by Thermo Electron Corporation. The XRD was equipped with a graphite monochromator filter, with the scanning area of 0.65–140°, the minimum wavelength step is 0.0001°. The spectral range of FTIR was 7800–350 cm−1, the spectral resolution is better than 0.5 cm−1, the wave number accuracy is better than 0.01 cm−1, and the signal-to-noise ratio is 40
000
:
1. The surface binding and elemental speciation was analyzed by X-ray photoelectron spectroscopy (XPS) using a Thermo ESCALAB 250XI X-ray photoelectron spectrometer, with the surface excitation at 1486.6 eV by an Al Ka X-ray source. The survey and high-resolution spectra of C1s, Br3d, S2p, Si2p and Hg4f were collected and calibrated with the binding energy of C1s at 284.9 eV. All the survey and high-resolution scans were analyzed with Thermo Avantage XPS software for surface chemical analysis.
2.3 DFT calculations
The calculation was performed using the Gaussian 16 suite program. The optimizations of the complex structures were performed by using B3LYP DFT. The LAN2DZ basis set was used to treat the mercury atom, whereas the 6-31G(d) basis set was used to treat all other atoms of C, H, S and Br. To identify the minimum point on the potential energy surface, set charge as 0; spin as 1, 3, 5 and 7 in the Guassian 16 software; and explore the spin value when the system energy is the lowest.
3. Results and discussion
3.1 Surface characterization
Surface characterization of the raw and brominated petroleum cokes was analyzed by SEM and XPS (Fig. 1). It was revealed that Br was successfully loaded on the surface of raw petroleum coke because the surface was much rougher and Br peaks appeared as well as no change of sulfur peaks. Strong S2s and S2p peaks are obtained in Fig. 1a. The peak at 164 eV is detected as S-containing complexes such as thiophene or thiols, which are reported to be possibly effective for the elemental Hg adsorption from the coal-fired flue gases.25 O1s peak at 532 eV is attributed to the formation of stable R–O–Hg complexes on the petroleum coke surface. The Br peaks of Br3s, Br3p, and Br3d can be obtained from Fig. 1b. It was reported that the strong peak at 70.0 ± 0.2 eV of Br3d(5/2) was bonded to sp2 and sp3 hybrid carbon atoms,26 which has a high potential for elemental Hg adsorption.
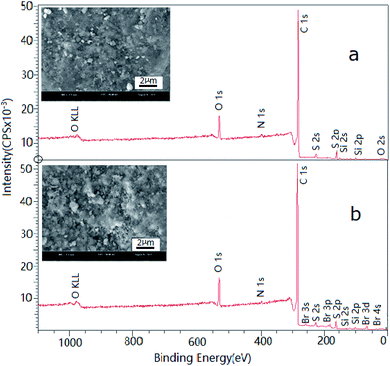 |
| Fig. 1 XPS survey scan and SEM images for the petroleum cokes before and after bromine modification. (a) Raw petroleum coke (b) brominated petroleum coke. | |
The XRD and FTIR patterns of raw and brominated petroleum cokes shown in Fig. 2 suggest that the prepared petroleum coke sorbent shows classical diffraction peaks and changes in the functional groups. Generally, we can distinguish whether the substance is crystalline or amorphous from the XRD spectrum by classifying whether the peaks are rich of fine peak structures or sharp peak structures. The petroleum coke is amorphous as revealed from Fig. 2a since a big fine peak appeared at 26°. After modification of the petroleum coke by bromine, a new fine peak appeared at 2θ of 13°, which indicated that the former structure is not changed, but other form of amorphous structure developed on the sorbent surface. The existence of big fine peaks in Fig. 2a shows that Br is primarily adsorbed on the surface of petroleum coke in the form of chemical loading, but not in the crystal form of Br molecules.
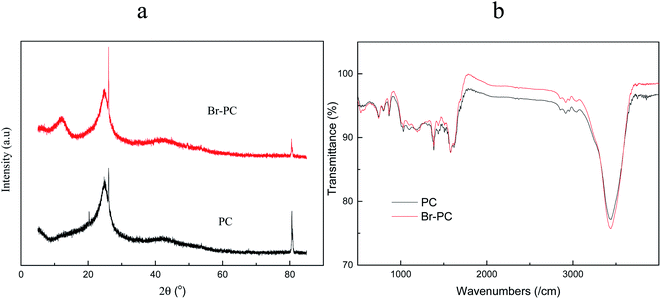 |
| Fig. 2 Sorbent characterization. (a) XRD analysis for petroleum coke before and after bromine modification (PC and Br-PC). (b) FTIR analysis for petroleum coke before and after bromine modification (PC and Br-PC). | |
Fourier transform infrared spectroscopy (FTIR) is commonly used to analyze the properties of functional groups on the surface of carbon-based adsorbents. The infrared absorption spectra of the petroleum coke before and after modification are illustrated in Fig. 2b. It can be observed in the figure that the types of functional groups on the surface of petroleum coke do not obviously change, but the number of functional groups changed during the bromine loading process.
The absorption peak at about 864 cm−1 is the stretching vibration peak of C–H, and the peak shape indicates that the C–H bond of the original petroleum coke breaks under the action of bromine, and some hydrogen is derived from the adsorbent. New stretching vibration between 2917 cm−1 and 3040 cm−1, 1030 and 1384 cm−1 and 864 and 1192 cm−1 regions indicate the appearance of new functional groups such as C–Br after bromine loading. It is well known that Br2 is a potent oxidant, and it is easy to open the edges of the graphite lamellar to facilitate the entry of Br molecules; therefore, the intercalation reaction begins at the edge of the graphite lamellar.27
The absorption peak at 744 cm−1 is the stretching vibration peak of heterocyclic thiophene,28 but the peak shape of thiophene does not change obviously, indicating that the loading of Br does not change the thiophene-sulfur functional groups in petroleum coke.
3.2 Thermal stability
To further secure the mechanism of mercury adsorption on the brominated petroleum coke and the synergetic effect of thiophene-sulfur and bonded bromine, high resolution XPS spectra over C1s, O1s, Br3d, S2p and Si2p/Hg4f regions were recorded and analyzed for all the steps of heating of the brominated petroleum cokes after Hg adsorption. XPS spectra (Fig. 3) show changes when the temperature is increasing. However, the peak at room temperature is basically the same as that at 200 °C, which is primarily due to the sample preparing process, at which all the prepared samples were vacuum dried at 200 °C for 30 min to let the unstable bonded bromine volatile before applying for Hg adsorption to ensure the samples are safe to deliver or test.22 Since Hg species such as HgBr2, Hg2Br2 and HgO are located at the binding energy between 100.7 eV and 104 eV, which overlap with the Si2p peaks, it is difficult to distinguish the Hg peaks from the XPS analysis. Much important information can be detected from the spectra via temperature changes.29 Notably, the Br3d and Si2p/Hg4f binding energy, which are sensitive to temperatures, indicate its un-stability in the sorbent. However, certain peaks of bromine and mercury still exist even for a temperature up to 950 °C.
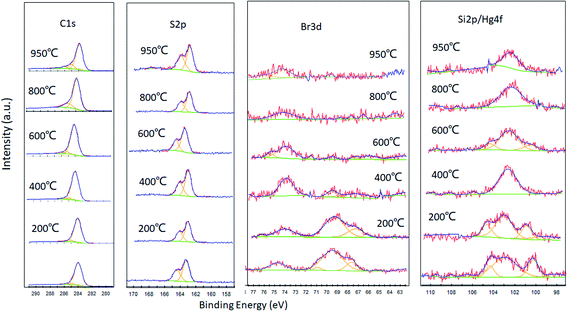 |
| Fig. 3 Temperature dependent X-ray photoelectron spectra for brominated petroleum coke after mercury capture. All heating steps were performed for 30 min in a N2 environment, with flow rate of 60 mL min−1 from 25 °C (room temperature) to 950 °C at a 10 °C min−1 heating rate. | |
The main peak of C1s is sp2/sp3 hybrid carbon and the peak of S2p is inherent organic sulfur such as thiophene and thion. Peaks of C1s and S2p are almost unchanged during the thermal treatment of the samples, indicating the C–C and C–S are difficult to break even for temperature up to 1000 °C.21
However, the number and the area of peaks of Br3d decline with increasing temperature. According to the previous studies, the Br3d 5/2 peak at 67.4 (±0.2) eV and 70.0 (±0.2) eV were obtained as Cn–Br2 and Cn–Br surface complexes.24,30 The Br3d peaks ranging between 72 and 76 eV was attributed to the bonded bromine on the carbon near the S atoms, since hydrogen on the thiophene rings can be easily replaced to form the derivatives of 2-bromothiophene.31 The surface complexes of Cn–Br2 and Cn–Br are sharply decreased; however, bromine near the sulfur atoms increased for the increase of temperature to 400 °C for 30 min; the surface complexes of Cn–Br2 and Cn–Br are further decreased, with no peaks detected at 67 and 70 eV, but strong peaks between 72 and 76 eV were seen when the sample was heated at 600 °C for 30 min. This indicates that bromine is almost volatilized from the surface of petroleum coke, but still strongly bonds with the carbon nearest to the S atom. The above results are consistent with the conclusion obtained from the XRF test in our previous study.22 When the temperature was increased up to 800 °C, the peak at 74 eV decreased, and is not obviously changed at 950 °C. In this situation, a certain amount of bromine is still binding to the surface of the sorbent, which has the potential ability for mercury capture.
Similarly, the peaks of Si2p/Hg4f decreased with increasing temperature, and the changing trend is almost the same as Br3d. The peak at 102.94 eV is almost constant for all the thermal treated samples, which should present the Si2p and part of the oxidized mercury. The existing form is predicted to be HgBr or Hg(Br)Br binding on the carbon atom nearest to the sulfur atoms. Other peaks at 98, 100.24, 104.35, 106.55 and 108.33 eV are Hg compounds of HgBr or Hg(Br)Br, which are not stable when high temperature applied.
3.3 Bromination model
First-principles density functional theory (DFT) calculations were carried out to help understand the reaction pathways, mechanisms and the active binding sites on the sorbent for bromine modification and mercury adsorption on the petroleum coke sorbent.
Details concerning the computational methods used were described, as well as the results for the initial modification of petroleum coke. The coupling of bromination intermediates was explored on the surface. Detailed structural optimizations were carried out on the petroleum coke model and over a range of bromine coverages to establish the lowest energy structures of the surfaces. The results for the model surface were used to determine the most favorable surface termination at the specific bromine chemical potentials.
The structure of petroleum coke and the sulfur form existing in the petroleum coke was studied by XPS and the computer models. Cai et al.32 reported that the sulfur structure in the petroleum coke was mainly organic sulfur, such as thiophene or similar thiophene rings. Xiao et al.33 outlined that the thiophene rings were on the outmost layer of the petroleum coke molecule, with at least three aromatic rings. Moreover, the inner layer of the petroleum coke molecule was aromatic; this configuration was regarded as graphite-like, and the carbon atoms were dominated by sp2 hybridization. Some small molecule fragments were around the outmost layer, including sulfur, oxygen, and nitrogen-containing fragments. Many studies have supported the zigzag carbine edge structure model for the carbon-based adsorbents on halogen elements modification, on which surface the active sites at the zigzag edge were reacted with the acid gas components, and new active sites were created for the elemental mercury oxidation.34–38 In our study, the zigzag carbine edge structure model was used as a precursor, with a thiophene-sulfur implanted in the model, to investigate the stability of bromine binding ability on the model (Fig. 4a and b).
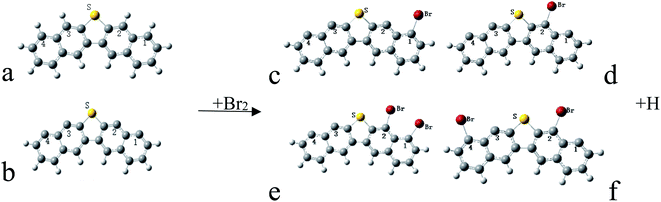 |
| Fig. 4 Chemical reaction equation for stable models of the Br atoms at different sites of petroleum coke molecule. (a and b) Stable petroleum coke molecules. (c) Br at next nearest of the S atom. (d) Br at the nearest of S atom. (e) Two Br atoms at the same side of the S atom. (f) Two Br atoms at the different side of the S atom. | |
Hg atoms adsorbed by petroleum coke are directly above the molecular plane of the pure petroleum coke, and the adsorption energy is −19.2 kJ mol−1, which belongs to weak physical adsorption. Bromine is a strongly oxidizing gas, which can easily open the edge of graphite lamellae, leading to the intercalation reaction and the carbon atoms becoming sp3 hybridized.39 In addition, the H near the S atom easily reacts with Br, due to the aromaticity of the molecule, which enhanced bromine binding on the sorbent. The above FTIR data also verified the breaking of C–H bond during the modification process, since the stretching peak of the C–H bond in the wavelength range of 3200 cm−1 to 2700 cm−1 decreased after bromination (Fig. 2). The intercalation reaction and the aromaticity of the petroleum coke molecule enhanced the activity of the adsorption site on the surface of petroleum coke and may produce more active sites for mercury capture, as shown in the chemical equation in Fig. 4.
The system total energy (STE) and the specific bond Mulliken population (BMP) are the important parameters to describe the stability of the model in DFT calculations; the lower the STE and higher the specific BMP, more stable is the model. Fig. 4c and d are the stable models of one Br atom bonded at different sites of the petroleum coke molecule. The STE of single bromine binding at carbon position 2 is 2.63 kJ mol−1 higher compared with position 1, and the C–Br BMP in position 1 is 0.2736 while it is 0.2954 in position 2. Both results show that Br tends to adsorb on the top of the carbon site nearest to the S. Fig. 4e and f are the stable models of two Br atoms binding at different sites of the petroleum coke molecule. The STE of the model of Fig. 4e is 42 kJ mol−1 higher than Fig. 4f, which indicates that Br is more inclined to adsorb on top of the relative carbon site close to S, thus forming a V-shaped structure.
3.4 Elemental mercury adsorption model
To explain more specifically what our study is expected to reveal, we illustrate the reaction process of Fig. 5 and 6 of the most relevant elemental mercury reaction on the brominated petroleum coke surface. The obtained stable mono-bromine and bi-bromine models are designed as reactants to react with the elemental Hg, the reacting processes are shown in Fig. 5 and 6. The intermediates and ultimate products of elemental Hg adsorbed on the surface model of brominated petroleum coke were obtained.
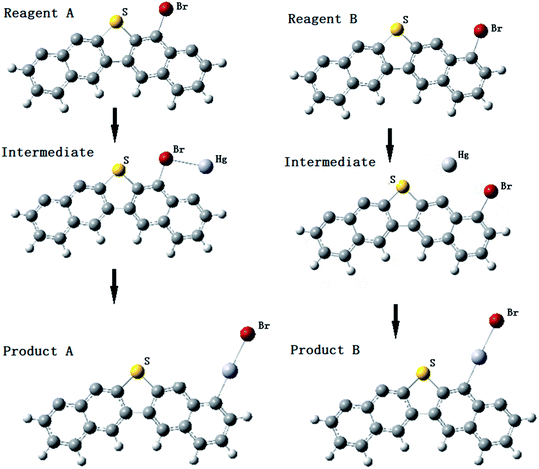 |
| Fig. 5 Hg0 adsorption process on brominated petroleum coke model with one Br atom at different carbon sites near sulfur. | |
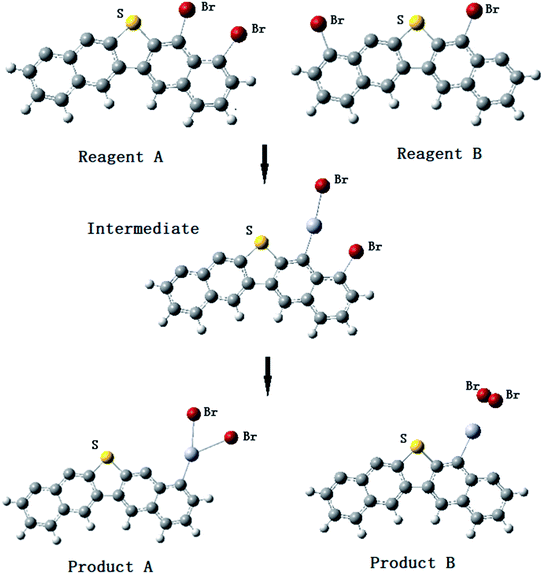 |
| Fig. 6 Hg0 adsorption process on brominated petroleum coke model with two Br atoms at different carbon sites near sulfur. | |
As shown in Fig. 5, the STE of product B is 14.26 kJ mol−1 lower than product A, and the C–Hg BMP of product A is 0.300 and 0.306 for product B. Both the results indicate product B is more stable than product A. In conclusion, HgBr is inclining to bind on the adjacent carbon site nearest to the S atom. These results agree with the XPS spectra shown in Fig. 3.
As shown in Fig. 6, both the reactant A and reactant B form stable final products. The STE of product B is 4.73 kJ mol−1 lower than product A, while the C–Hg BMP of product A is 0.315 and 0.298 for product B. Comparing the STE and the C–Hg BMP of the two products, product A is more stable than product B, which indicates that HgBr2 can be formed to bind to the carbon site nearest to the S atom.
Two mercury oxidized substances, HgBr and HgBr2, are formed on the surface of bromine-modified adsorbents as found by various researchers.40–42 However, compared the models of product B-HgBr and -HgBr2, the adsorption energies are about –472.05 kJ mol−1 and –221.92 kJ mol−1 separately. From the sight of the chemical reaction energy barrier, the activation energy required for HgBr2 is quite higher than HgBr. Thus, the results show that the main form of Hg0 on the surface of brominated carbon-based adsorbents is HgBr, and HgBr2 is usually decomposed into Br atom and the compound HgBr on the surface of the adsorbent, the chemical form of which is usually described as Hg(Br)Br. Brominated petroleum coke adsorbent is a typical carbon-based adsorbent, the adsorption of HgBr2 on the surface of petroleum coke is also unstable, which agreed with the results of other researchers.
4. Conclusions
The results reported demonstrate that high sulfur-containing petroleum coke is a potential carbon-based sorbent for bromine modification to capture elemental Hg from the coal combustion flue gases to reduce the threat of Hg to the environment. The important effect and mechanism of thiophene-sulfur in the petroleum coke on the binding of Br and Hg adsorption are revealed by XPS and DFT calculation. Bromine intercalation reaction and the aromaticity of thiophene are possibly leading to the C–H bond cleavage and ortho-substitution reaction, which was revealed from XRD and FTIR analysis. These features can enhance the Br loading during the modification process, and improve the Hg removal efficiency. The amount of Br reduced when the adsorbed Hg was decreased, which was revealed from the thermal treatment of the resulting samples. However, even for temperatures up to 600 °C, a portion of bromine is still stably bound to the carbon site nearest to the S atom, indicating the key effect of thiophene sulfur in the sorbent. The synergetic effect of inherent thiophene sulfur and the loaded bromine enhanced the Hg removal efficiency of the adsorbent.
DFT study showed that the Br atom was always likely to bind on the C atom nearest to the S atom, i.e., thiophene sulfur can significantly promote the loading of bromine on the surface of petroleum coke. When Hg0 reacts on the surface of the brominated petroleum coke, HgBr2 and HgBr are formed, which are both inclined to bound to the C atom nearest to the S atom. Moreover, HgBr2 is easily decomposed into HgBr and Br atoms adsorbing on the surface of petroleum coke independently, which is usually described as Hg(Br)Br. The synergistic effect of S and Br provides more active sites for the elemental mercury adsorption.
In conclusion, the ability of petroleum coke to adsorb Hg reveals major dependence on the inherent concentration of the organic sulfur and the bonded bromine. The higher the content of organic sulfur, the higher the concentration of effective bromine loaded on the surface and better the mercury adsorption capacity of the brominated petroleum coke. Thus, this study illustrates that high-sulfur petroleum coke is a potential material as a precursor in applying for the elemental mercury removal from the flue gases, corresponding to the bromine modification. Thiophene is predicted to be a possible modifier to impregnate with the carbon-based materials for elemental Hg capture.
Conflicts of interest
There are no conflicts to declare.
Acknowledgements
This work is supported by the National Fundamental Research Funds for the Central Universities (CUSF-DH-D-2017097), Shanghai New Teacher Training Program (ZZNL20012) and Scientific Research Project of Shanghai Vocational College of Agriculture and Forestry (KY2-0000-20-04). Professor Zhenghe Xu in University of Alberta (Alberta, Canada) is highly appreciated for supporting the experimental materials preparation.
References
- Environmental impacts of coal power: air pollution, http://www.ucsusa.org/clean_energy/coalvswind/c02c.html.
- Clean Air Act homepage, http://www.ec.gc.ca/cleanair-airpur/clean_air_act-WS1CA709C8-1_en.htm.
- Y. Liu, T. M. Bisson, H. Yang and Z. Xu, Recent developments in novel sorbents for flue gas clean up, Fuel Process. Technol., 2010, 91(10), 1175–1197 CrossRef CAS.
- E. Sasmaz, A. Kirchofer, A. D. Jew and A. Saha, Mercury chemistry on brominated activated carbon, Fuel, 2012, 99(9), 188–196 CrossRef CAS.
- E. C. Rupp and J. Wilcox, Mercury chemistry of brominated activated carbons – packed-bed breakthrough experiments, Fuel, 2014, 117(1), 351–353 CrossRef CAS.
- A. Saha, Investigation of changes in surface chemistries on virgin and brominated activated carbon sorbents during mercury capture: before and after regeneration, Energy Fuels, 2014, 28(6), 4021–4027 CrossRef CAS.
- T. M. Bisson, L. C. Maclean, Y. Hu and Z. Xu, Characterization of mercury binding onto a novel brominated biomass ash sorbent by x-ray absorption spectroscopy, Environ. Sci. Technol., 2012, 46(21), 12186–12193 CrossRef CAS.
- D. Karatza, A. Lancia, D. Musmarra and C. Zucchini, Study of mercury absorption and desorption on sulfur impregnated carbon, Exp. Therm. Fluid Sci., 2000, 21(1), 150–155 CrossRef CAS.
- K. S. Reddy, A. A. Shoaibi and C. Srinivasakannan, Elemental mercury adsorption on sulfur-impregnated porous carbon - a review, Environ. Technol., 2014, 35(1), 18–26 CrossRef.
- R. D. Vidic, M. T. Chang and R. C. Thurnau, Kinetics of vapor-phase mercury uptake by virgin and sulfur-impregnated activated carbons, J. Air Waste Manage. Assoc., 1998, 48(3), 247–255 CrossRef CAS.
- S. Vitolo and M. Seggiani, Mercury removal from geothermal exhaust gas by sulfur-impregnated and virgin activated carbons, Geothermics, 2002, 31(4), 431–442 CrossRef CAS.
- S. V. Krishnan, B. K. Gullett and W. Jozewicz, Sorption of elemental mercury by activated carbons, Environ. Sci. Technol., 1994, 28(8), 1506–1512 CrossRef CAS.
- Y. Otani, C. Kanaoka, H. Emi, I. Uchijima and H. Nishino, Removal of mercury vapor from air with sulfur-impregnated adsorbents, Environ. Sci. Technol., 1988, 22(6), 708–711 CrossRef CAS.
- Q. Zhu, S. L. Wegener, C. Xie, O. Uche, M. Neurock and T. J. Marks, Sulfur as a selective 'soft' oxidant for catalytic methane conversion probed by experiment and theory, Nat. Chem., 2013, 5(2), 104–109 CrossRef CAS.
- J. Olmeda, M. Frías, M. Olaya, B. Frutos and M. I. S. D. Rojas, Recycling petroleum coke in blended cement mortar to produce lightweight material for impact noise reduction, Cem. Concr. Compos., 2012, 34(10), 1194–1201 CrossRef CAS.
- C. Almansa, M. Molina-Sabio and F. Rodríguez-Reinoso, Adsorption of methane into ZnCl2activated carbon derived discs, Microporous Mesoporous Mater., 2004, 76(1), 185–191 CrossRef CAS.
- A. Perrin, A. Celzard, A. Albiniak, M. Jasienko-Halat, J. F. Marêché and G. Furdin, Naoh activation of anthracites: effect of hydroxide content on pore textures and methane storage ability, Microporous Mesoporous Mater., 2005, 81(1), 31–40 CrossRef CAS.
- M. Molina-Sabio and F. RodríGuez-Reinoso, Role of chemical activation in the development of carbon porosity, Colloids Surf., A, 2004, 241(1), 15–25 CrossRef CAS.
- M. A. Lillo-Ródenas, D. Cazorla-Amorós, A. Linares-Solano, F. Béguin, C. Clinard and J. N. Rouzaud, HRTEM study of activated carbons prepared by alkali hydroxide activation of anthracite, Carbon, 2004, 42(7), 1305–1310 CrossRef.
- B. Jiang, Y. Zhang, J. Zhou, K. Zhang and S. Chen, Effects of chemical modification of petroleum cokes on the properties of the resulting activated carbon, Fuel, 2008, 87(10–11), 1844–1848 CrossRef CAS.
- X. Jin, Q. Zhong, F. Li, J. D. Huang, Y. Zhang and B. Wang, Modeling the change of green coke to calcined coke by using qingdao high-sulfur petroleum coke, Energy Fuels, 2015, 29(5), 1504–1516 CrossRef.
- X. Yi, D. Pudasainee, R. Gupta, Z. Xu and Y. Diao, Bromination of petroleum coke for elemental mercury capture, J. Hazard. Mater., 2017, 336, 232–239 CrossRef.
- Y. Xiao, D. Pudasainee, R. Gupta, Z. Xu and Y. Diao, Elemental mercury reaction chemistry on brominated petroleum cokes, Carbon, 2017, 124, 89–96 CrossRef CAS.
- Y. Xiao, Y. Diao, L. Tian, Y. Lu and L. Zhan, Kinetic mechanism of novel brominated petroleum coke on trace Hg0 removal from flue gas, China Environ. Sci., 2018, 38(2), 508–515 Search PubMed.
- H. Hsi, M. J. Rood, M. Rostamabadi, A. Shiaoguo Chen and R. Chang, Effects of sulfur impregnation temperature on the properties and mercury adsorption capacities of activated carbon fibers (acfs), Environ. Sci. Technol., 2001, 35(13), 2785–2791 CrossRef CAS.
- N. D. Hutson, B. C. Attwood and K. G. Scheckel, XAS and XPS characterization of mercury binding on brominated activated carbon, Environ. Sci. Technol., 2007, 41(5), 1747–1752 CrossRef CAS.
- J. Hwang, J. P. Carbotte, S. Tongay, A. F. Hebard and D. B. Tanner, Ultrapure multilayer graphene in bromine-intercalated graphite, Phys. Rev. B, 2012, 84(4), 401–410 Search PubMed.
- P. J. Zhao, C. Ma and J. T. Wang, et al. Almost total desulfurization of high-sulfur petroleum coke by Na2CO3-promoted calcination combined with ultrasonic-assisted chemical oxidation, Carbon, 2019, 145, 774 CrossRef.
- J. Wilcox, E. Sasmaz, A. Kirchofer and S.-S. Lee, Heterogeneous mercury reaction chemistry on activated carbon, J. Air Waste Manage. Assoc., 2011, 61(4), 418 CrossRef CAS.
- E. Papirer, R. Lacroix, J. B. Donnet, G. Nanse and P. Fioux, XPS study of the halogenation of carbon black-part 1. bromination, Carbon, 1994, 32(7), 1341–1358 CrossRef CAS.
- A. K. Shigemoto, C. N. Virca, S. J. Underwood, L. R. Shetterly and T. M. Mccormick, Thiophene-based fluorescent mercury-sensors, J. Coord. Chem., 2016, 69(11–13), 1–21 Search PubMed.
- J. H. Cai, E. Morris and C. Q. Jia, Sulfur speciation in fluid coke and its activation products using k-edge x-ray absorption near edge structure spectroscopy, Sulfur Rep., 2009, 30(6), 555–569 CrossRef CAS.
- X. Jin, Q. Zhong, F. Li, J. D. Huang, Y. Zhang and B. Wang, Modeling the change of green coke to calcined coke by using qingdao high-sulfur petroleum coke, Energy Fuels, 2015, 29(5), 1504–1516 CrossRef.
- C. Rungnim, V. Promarak, S. Hannongbua, N. Kungwan and S. Namuangruk, Complete reaction mechanisms of mercury oxidation on halogenated activated carbon, J. Hazard. Mater., 2016, 310, 253–260 CrossRef CAS.
- L. R. Radovic, The mechanism of co chemisorption on zigzag carbon active sites: a computational chemistry study, Carbon, 2005, 43(5), 907–915 CrossRef CAS.
- X. Sun, J. Y. Hwang and S. Xie, Density functional study of elemental mercury adsorption on surfactants, Fuel, 2011, 90(3), 1061–1068 CrossRef CAS.
- F. H. Yang and R. T. Yang, Ab initio molecular orbital study of adsorption of atomic hydrogen on graphite: insight into hydrogen storage in carbon nanotubes, Carbon, 2002, 40(3), 437–444 CrossRef CAS.
- B. Padak and J. L. Wilcox, Understanding the Binding Mechanism of Mercury on Activated Carbon, Carbon, 2008, 47(12), 2855–2864 CrossRef.
- Y. Gao, J. Gu, W. Shen and F. Kang, Bromine-graphite intercalation compounds, Carbon Technol., 2000, 4, 21–25 Search PubMed.
- E. Sasmaz, A. Kirchofer, A. D. Jew and A. Saha, Mercury chemistry on brominated activated carbon, Fuel, 2012, 99(9), 188–196 CrossRef CAS.
- C. Rungnim, V. Promarak, S. Hannongbua, N. Kungwan and S. Namuangruk, Complete reaction mechanisms of mercury oxidation on halogenated activated carbon, J. Hazard. Mater., 2016, 310, 253–260 CrossRef CAS.
- J. Liu, W. Qu and C. Zheng, Theoretical studies of mercury–bromine species adsorption mechanism on carbonaceous surface, Proc. Combust. Inst., 2013, 34(2), 2811–2819 CrossRef CAS.
|
This journal is © The Royal Society of Chemistry 2021 |