DOI:
10.1039/D0RA10071D
(Paper)
RSC Adv., 2021,
11, 18003-18008
Brucite shows antibacterial activity via establishment of alkaline conditions
Received
29th November 2020
, Accepted 10th May 2021
First published on 18th May 2021
Abstract
Brucite, a mineral form of magnesium hydroxide, has been used industrially as a useful refractory insulator. However, its antibacterial activity remains largely unknown. This study investigated the antibacterial activity of brucite in two bacterial strains, Escherichia coli (Gram-negative) and Staphylococcus aureus (Gram-positive). Brucite, compared to other minerals, showed a strong antibacterial activity against both bacterial strains. The surface structure of brucite examined using scanning electron microscopy (SEM) had no implications in its antibacterial activity. Brucite in lysogeny broth (LB) medium maintained the basicity (pH 9) of the solution for a prolonged period of time. The basicity of the medium was responsible for the antibacterial activity of brucite. The study results identified brucite as a potent antibacterial agent that required no further processing and also revealed the underlying mechanism of action responsible for its antibacterial activity.
Introduction
Microorganisms including bacteria, archaea, fungi, protozoa, algae, and viruses can grow by attaching themselves to abiotic surfaces, such as medical devices and aquatic systems, and living tissues, such as tooth enamels, heart valves, and lungs. Growth on living tissues results in the formation of biofilms.1–3 A biofilm can be defined as a community of microorganisms in which the organisms grow via irreversible attachment to each other or other surfaces. The extracellular polymers created during biofilm formation promote the adhesion of the microorganisms and the formation of a matrix.4,5 Bacterial biofilms are mainly formed when bacteria adapt to rapid changes in temperature, or survive under adverse conditions, such as starvation and desiccation.6,7 The accumulation of biofilms in the air circulation system of various devices such as air conditioners is the one of the reasons for allergic reactions of the respiratory organs.8 The formation of biofilms in water pipelines causes malfunction of water cooling systems with the pipes clogging.9 Therefore, exploring a new antibacterial material with low cost, high-stability, and environmental friendly mineral content might be profitable to industry.
To date, various studies have been conducted to inhibit the formation of bacterial biofilms.10,11 Some approaches include exploration of specific technologies that disrupt bacterial growth through the transmission of shock waves/sound waves, induction of physical damage to the bacterial membrane using the sharp edge of a nano-wall, and usage of chemical treatment to destroy bacterial membrane permeability.12–14
Minerals used as antibacterial agents can demonstrate various functions depending on the source and content, and can even play a novel role depending on the combination ratio of elements included in the composition. Additionally, the use of minerals has several advantages, such as high absorbance, surface area, heat capacity, exchange capacity, low price, sustainability, safety, and low resistance.15,16 Zeolite is a representative mineral with antibacterial activity. However, the antibacterial activity is evident only after ion exchange process. Since the ionization process of zeolite for obtaining antibacterial activity is industrially inefficient, research is warranted to discover minerals that exhibit antibacterial activity without the need for any processing.17,18
In this study, we examined the antibacterial activity of different minerals that were selected based on their crystal structure, mineral group, and industrial application.
Materials and methods
Minerals
The minerals used in this study were provided by Dongyang M&M Industry Co., Ltd, a company dealing with industrial minerals in Korea. Minerals for antibacterial testing were selected from commonly used industrial minerals due to easy access and the chemical compositions and crystal forms of the minerals are provided in Table 1. The minerals with various chemical composition were tested to verify the association of antibacterial activity with chemical reaction, whereas the crystal forms were tested to verify the association of antibacterial activity with mineral shapes in microscale. All the minerals were sterilized by autoclaving (121 °C, 1 h) before being used in experiments.
Table 1 Mineral properties
Name |
Formula |
Crystal system |
Baritite |
BaSO4 |
Orthorhombic |
Bauxite |
Al2O3·2H2O |
N/A |
Bentonite |
Al2Si4(OH) |
Monoclinic |
Brucite |
Mg(OH)2 |
Trigonal |
Calcite |
CaCO3 |
Trigonal |
Muscovite |
KAl2(AlSi3O10)(F,OH)2 |
Monoclinic |
Pyrophyllite |
Al2Si4O10(OH)2 |
Triclinic or monoclinic |
Sericite |
KAl2(Si3Al)O10(OH)2 |
Monoclinic |
Talc |
Mg3Si4O10(OH)2 |
Monoclinic or triclinic |
Wollastonite |
CaSiO3 |
Triclinic |
Zeolite |
MxDy[Al(x+2y)Sin−(x+2y)O2n]·mH2O |
Variety |
Antibacterial activity tests
Escherichia coli (E. coli) or Staphylococcus aureus (S. aureus) are known as the most representative bacteria, Gram-negative and Gram-positive, respectively, and are used in various antibacterial activity tests. Both Gram-positive and Gram-negative bacteria were used to determine the antibacterial activity of the minerals. Liquid medium or agar plates were prepared by including the minerals at different concentrations (0.1, 1.0, 10, and 100 mg ml−1) in lysogeny broth (LB) medium. A diluted bacterial culture obtained by serial dilution (1/104) of 100 μl E. coli or S. aureus culture suspensions were plated onto LB agar plates and incubated at 37 °C for 24 h. After incubation, the number of bacterial colonies was counted using a colony counting software (openCFU). Sterilized LB medium, each containing different minerals were inoculated with 500 μl of the bacterial suspension of E. coli and incubated in a shaking incubator at 37 °C. The optical density at 600 nm (OD600) was measured for the culture suspension at various time points (1–12 h) using the SpectraMax ABS Plus (Molecular Devices, Toronto, Canada).
Scanning electron microscopy (SEM) analysis
The surface of brucite mineral was investigated using the Zeiss Merlin scanning electron microscope (Carl Zeiss AG, Oberkochen, Germany). Brucite samples treated with the same conditions as that performed for the experimental samples were prepared by removing the supernatant from the sterilized LB medium containing brucite. Then the samples were dried at 50 °C. Images of two types of samples, brucite without any other treatment and brucite that reacted in the LB medium, were acquired at various magnifications to observe the surface structural features.
Determination of magnesium ion concentration
The concentration of magnesium ions was measured using the QuantiChrom magnesium assay kit (BioAssay Systems, Hayward, USA), according to the manufacturers' instructions. LB medium mixed with minerals was subjected to centrifugation (4500 g, 20 min) and the supernatant obtained was analyzed to measure the magnesium ion concentration using a microplate reader at 500 nm.
Statistical analysis
All experiments were performed at least thrice. Data are presented as mean ± standard error of the mean. Statistical analysis was performed using a two-tailed Student's t-test. A p-value < 0.05 indicated statistical significance. The details of the statistical analyses are described in the figure legends.
Results and discussion
Antibacterial activity of brucite
Ten representative minerals were selected according to their crystalline structure, mineral group, and industrial applicability (Table 1). Both Gram-positive (thick peptidoglycan) and Gram negative (thin peptidoglycan with outer lipid membrane) bacteria were selected to test the antibacterial activity.19,20 The antibacterial activity of the minerals against Gram-negative E. coli was determined by the number of bacterial colonies observed on solid LB agar plate containing different concentrations (0.1, 1.0, and 10 mg ml−1) of the 10 selected minerals (Fig. 1A). Similarly, the antibacterial activity of the minerals against Gram-positive S. aureus was also determined (Fig. 1B). With increasing concentration of brucite mineral, the number of E. coli colonies decreased by 44% and 83% compared to the control. Brucite also exhibited similar antibacterial effects against S. aureus. Remarkable antibacterial activity of brucite was observed at a concentration of 10 mg ml−1. Our results demonstrated a concentration-dependent antibacterial activity of brucite against both Gram-negative and Gram-positive bacteria, thereby suggesting brucite as a potential antibacterial agent.
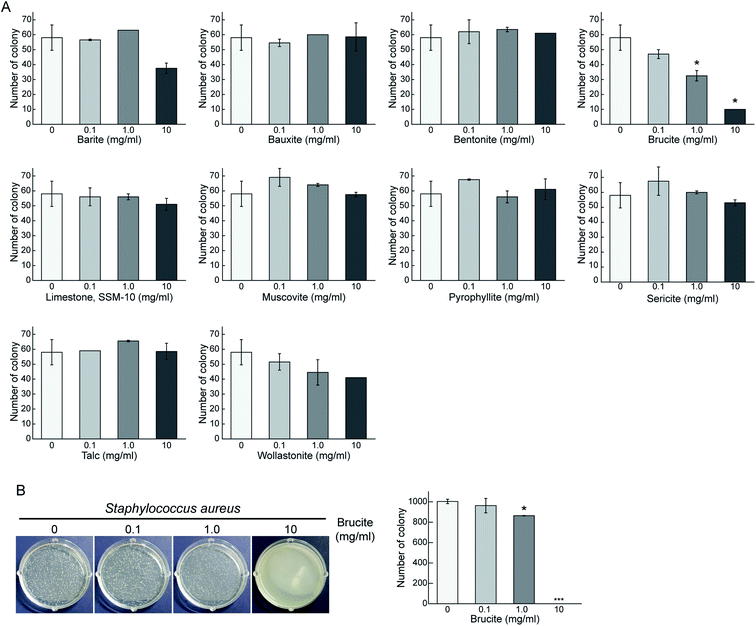 |
| Fig. 1 Antibacterial activity of brucite. (A) Gram-negative Escherichia coli (E. coli) was grown on solid lysogeny broth (LB) agar medium containing minerals at the indicated concentration. The graph represents the number of bacterial colonies on the surface of the LB medium after 24 h of incubation. (B) Gram-positive Staphylococcus aureus was grown on solid LB agar medium containing brucite at the indicated concentration. Colonies formed on the LB agar plate were represented by images (top) and the number of bacterial colonies (bottom). Bars represent mean ± standard error of the mean (n = 3). *p < 0.05 and ***p < 0.0005, compared to the respective controls. | |
Surface structure of brucite
The marked antibacterial activity of brucite motivated us to investigate the mechanism of action underlying bacterial growth inhibition. A recent study has revealed that the surface nano architecture of dragonfly (Diplacodes bipunctata) wings exerts a deformational stress on bacteria, thereby exhibiting antibacterial activity.21 Based on this finding, we examined whether the antibacterial activity of brucite was mediated by its surface structure in the medium (Fig. 2). Analysis of the SEM images of the surface structure of brucite showed no sharp structure that could physically inhibit the bacterial growth. Even after reaction in LB medium, similar surface structural features were observed, indicating that the surface structure of brucite had no role in its antibacterial activity.
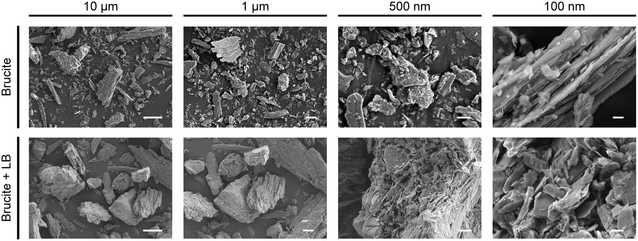 |
| Fig. 2 Surface structure of brucite does not affect the antibacterial activity. Surface structure was observed by scanning electron microscopy (SEM). Brucite without any other treatment (top) and brucite after reaction in the medium (bottom) were photographed. The white bar in the image represents the scale shown above. | |
Antibacterial activity of brucite extracts
Other factors that could potentially induce the antibacterial activity of brucite were investigated. We attempted to verify whether the eluted components of brucite were responsible for the antibacterial activity. First, the brucite and LB medium were mixed and sterilized, and then the precipitate and the medium supernatant were separated by centrifugation. Sterilization was performed to prevent any further changes by brucite to the medium. After inoculation of E. coli in the liquid medium made of the supernatant, the growth of bacteria was examined by measuring the absorbance every 1 h at 600 nm (Fig. 3A). The growth of E. coli on LB agar plates made of the supernatant was assessed by measuring the number of colonies formed after 24 h of incubation (Fig. 3B). E. coli barely grew in liquid medium, and the growth rate decreased by 69% and 92%, respectively, in solid medium containing the 10 and 100 mg ml−1 brucite. These results indicate that the antibacterial activity of brucite is due to the substances eluting from brucite.
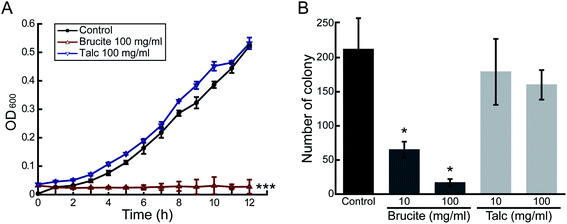 |
| Fig. 3 Brucite extracts possess antibacterial activity. The medium supernatants were separated from the minerals at the indicated concentration by centrifugation. (A) E. coli was incubated in the liquid supernatant medium. The graph shows the growth curve of bacteria and the absorbance measured at 600 nm, every h. (B) E. coli was grown for 24 h in solid LB agar medium. The graph represents the number of bacterial colonies formed on the surface of LB agar plate. Bars represent mean ± standard error of the mean of two independent experiments (n = 3). *p < 0.05, ***p < 0.0005, compared to the respective controls. | |
Brucite establishes alkaline conditions
Chemically, brucite is called magnesium hydroxide and has a chemical formula of Mg(OH)2. The crystal structure of brucite is composed of magnesium (Mg2+) cations and hydroxyl (OH−) anions arranged in layers in a trigonal structure. To elucidate the mechanisms of antibacterial activity of the brucite, we extracted the supernatant fraction of 100 mg ml−1 brucite medium separated by centrifugation (brucite extract medium). Using a commercial magnesium assay kit, we verified that the brucite extract medium contained the Mg2+ cations (Fig. 4A). In addition, we showed that the neutralization of the brucite extract medium by hydrogen chloride (HCl) increases the Mg2+ cations level, which means that the pH-dependent Mg2+ ions leaked from brucite.
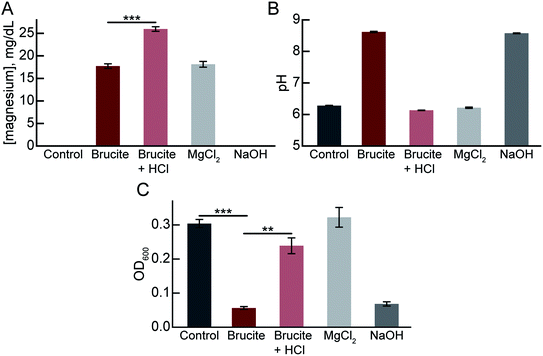 |
| Fig. 4 Antibacterial activity of brucite is mediated by pH change. The supernatant was separated from 100 mg ml−1 brucite medium by centrifugation to obtain a brucite extract medium. NaOH or MgCl2 was added to the LB medium. HCl was used to neutralizing the brucite extract medium. (A) The graph represents the concentrations of Mg2+ of the medium. (B) The average pH of the medium. (C) E. coli were incubated with the indicated media at 6 h in a 37 °C shaking incubator. Absorbance at 600 nm was measured for estimating the concentration of bacteria. Bars represent mean ± standard error of the mean (n = 3). **p < 0.005 and ***p < 0.0005. | |
The optimum pH range of the medium for growing E. coli is 6.5–7.5; culturing at a pH lower or higher than the optimum pH inhibits the bacterial growth. To evaluate the effects of brucite on pH of the medium, the pH of the brucite extract medium was measured. The pH of brucite extract medium was 8.62 (Fig. 4B). The pH of the brucite extract medium was adjusted to 6.13 (pH equal to the control medium) using HCl and the subsequent effect on the growth of bacteria was evaluated to confirm the role of high pH of the medium in mediating the antibacterial activity. The bacterial growth, which decreased by 19% in the brucite extract medium compared to the control medium, increased to 79% in the neutralized brucite supernatant medium (Fig. 4C). We confirmed that the medium used to adjust the pH to 8.57 using NaOH also had antibacterial activity. However, the MgCl2-treated medium with the same concentration of Mg2+ cations in brucite extract medium had no antibacterial activity (Fig. 4C). These results suggest that antibacterial activity of brucite is due to the increase in the pH of the medium, which, in turn, is due to the leakage of OH− anion from the magnesium hydroxide complex of brucite.
Brucite with potential antibacterial activity
Most of the minerals exhibiting antibacterial activity often require an additional processing step. For example, zeolite exhibits antibacterial effects only after the ion exchange process. Such an additional process is both time- and cost-intensive. In this study, brucite showed antibacterial activity only by dissolution in the medium and required no additional processing. Therefore, to compare the antibacterial activity of brucite and zeolite, number of E. coli colonies formed after 24 h of incubation on an LB agar plate containing 10 mg ml−1 of each mineral was measured. Brucite and zeolite reported 89% and 33% reduction in cell colony numbers, respectively (Fig. 5A). Thus, antibacterial activity of brucite was nearly 3 times higher than that of zeolite. Finally, the pH was measured periodically for 3 months to determine the duration in which brucite continued to change the pH of the LB medium. The medium was sterilized through autoclaving, and simultaneously, the conditions to accelerate the reaction when the actual antibacterial activity decreased due to high temperature and high pressure were established. The pH of brucite measured on the first day was 9.17, and the average of the pH values measured for 3 months was 9.06 (Fig. 5B). Considering that the deviation was 0.11, it was confirmed that the pH was maintained continuously for 3 months. After pH measurement, the settled powder used for 3 months was dried and the subsequent weight was measured. Compared to the initial weight of 3 g, weight of brucite obtained after 3 months was 2.66 g, indicating that 89% of the initial quantity of brucite remained. On the contrary, for zeolite which was used as a control, 96% of the initial amount remained. Brucite maintained the alkaline pH by continuously releasing ions into the medium.
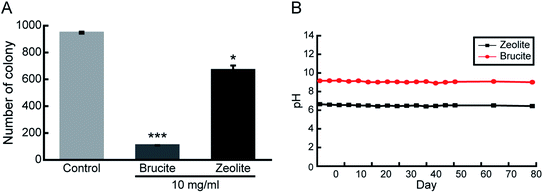 |
| Fig. 5 Brucite shows better antibacterial activity than zeolite. (A) E. coli was grown for 24 h on solid LB agar medium containing minerals at the indicated concentrations. The graph represents the number of bacterial colonies formed on the surface of the LB medium. (B) The pH of minerals was measured by periodically replacing the supernatant medium twice a week for two months and once every two weeks in the remaining one month. Bars represent mean ± standard error of the mean (n = 3). *p < 0.05 and ***p < 0.0005, compared to the respective controls. | |
Conclusions
This study reported antibacterial activity of brucite against both Gram-positive and Gram-negative bacteria via establishment of high alkaline pH conditions in the medium via release/leakage of Mg2+ cation and OH− anion, respectively. Compared to the other minerals, brucite demonstrates a considerable effect even at small quantities. Additionally, compared to zeolite, brucite is expected to have a greater advantage of cost and time efficiency as no additional processing is required. The Mohs scale of mineral hardness representing the resistance of a mineral surface to scratching/abrasion in the range of 1–10 was used to interpret the ease of processing brucite. The Mohs scale of hardness value of brucite ranges from 2.5–3, indicating the ease of processing. Although the study specifically focused on bacteria, it is worth exploring whether brucite is effective against other microorganisms, such as archaea, fungi, protozoa, algae, and viruses. Human skin maintains a weak acidity of pH 4–6 through various secretions including sweat, and there is a risk of increasing the risk of atopic dermatitis through the occurrence of acne and inflammation when exposed to alkaline conditions.22,23 Therefore, it is considered that additional experiments on the biological safety of brucite mixed with substances that are continuously exposed to human skin are necessary. Further studies evaluating the commercial value of brucite by confirming its antibacterial activity even when mixed with other materials, such as cement and paint, should be conducted to explore the applications of brucite.
Conflicts of interest
There are no conflicts to declare.
Acknowledgements
This work was supported by Chungnam National University.
References
- J. W. Costerton, P. S. Stewart and E. P. Greenberg, Science, 1999, 284, 1318–1322 CrossRef CAS PubMed.
- R. M. Donlan, Clin. Infect. Dis., 2001, 33, 1387–1392 CrossRef CAS PubMed.
- G. O'Toole, H. B. Kaplan and R. Kolter, Annu. Rev. Microbiol., 2000, 54, 49–79 CrossRef PubMed.
- E. Karatan and P. Watnick, Microbiol. Mol. Biol. Rev., 2009, 73, 310–347 CrossRef CAS PubMed.
- S. L. Percival, Br. J. Surg., 2017, 104, e85–e94 CrossRef CAS PubMed.
- P. M. S. Debjani Banerjee, P. Gautam, K. Misra, A. Sahoo and S. Samanta, Proc. Natl. Acad. Sci., India, Sect. B, 2020, 90, 243–259 CrossRef.
- A. Koerdt, J. Godeke, J. Berger, K. M. Thormann and S. V. Albers, PLoS One, 2010, 5, e14104 CrossRef PubMed.
- R. B. Simmons, L. J. Rose, S. A. Crow and D. G. Ahearn, Curr. Microbiol., 1999, 39, 141–145 CrossRef CAS PubMed.
- F. Di Pippo, L. Di Gregorio, R. Congestri, V. Tandoi and S. Rossetti, FEMS Microbiol. Ecol., 2018, 94, 1–13 Search PubMed.
- C. Bankier, R. K. Matharu, Y. K. Cheong, G. G. Ren, E. Cloutman-Green and L. Ciric, Sci. Rep., 2019, 9, 16074 CrossRef CAS PubMed.
- Q. Borjihan and A. Dong, Biomater. Sci., 2020, 8, 6867–6882 RSC.
- O. Akhavan and E. Ghaderi, ACS Nano, 2010, 4, 5731–5736 CrossRef CAS PubMed.
- M. Buonanno, G. Randers-Pehrson, A. W. Bigelow, S. Trivedi, F. D. Lowy, H. M. Spotnitz, S. M. Hammer and D. J. Brenner, PLoS One, 2013, 8, e76968 CrossRef CAS PubMed.
- A. F. Subramaniyam Rajalakshmi, J. R. Raoa and B. U. Nair, RSC Adv., 2014, 4, 32004–32012 RSC.
- M. R. A.-D. Shehab and A. Lafi, Egypt. Acad. J. Biol. Sci., G Microbiol., 2011, 3(1), 75–81 Search PubMed.
- L. B. Williams and S. E. Haydel, Int. Geol. Rev., 2010, 52, 745–770 CrossRef PubMed.
- S. Demirci, Z. Ustaoglu, G. A. Yilmazer, F. Sahin and N. Bac, Appl. Biochem. Biotechnol., 2014, 172, 1652–1662 CrossRef CAS PubMed.
- M. M. Salim and N. Malek, Mater. Sci. Eng., C, 2016, 59, 70–77 CrossRef CAS PubMed.
- Z. Breijyeh, B. Jubeh and R. Karaman, Molecules, 2020, 25(6), 1340 CrossRef CAS PubMed.
- R. B. Moyes, J. Reynolds and D. P. Breakwell, Curr. Protoc. Microbiol., 2009, A.3C.1–A.3C.8 Search PubMed Appendix 3, Appendix 3C.
- E. P. Ivanova, J. Hasan, H. K. Webb, G. Gervinskas, S. Juodkazis, V. K. Truong, A. H. Wu, R. N. Lamb, V. A. Baulin, G. S. Watson, J. A. Watson, D. E. Mainwaring and R. J. Crawford, Nat. Commun., 2013, 4, 2838 CrossRef PubMed.
- E. Proksch, J. Dermatol., 2018, 45, 1044–1052 CrossRef CAS PubMed.
- H. C. Korting, M. Kerscher, M. Schafer-Korting and U. Berchtenbreiter, Clin. Invest., 1993, 71, 644–648 CAS.
Footnote |
† Authors contributed equally. |
|
This journal is © The Royal Society of Chemistry 2021 |