DOI:
10.1039/D0RA08900A
(Paper)
RSC Adv., 2021,
11, 3429-3438
Investigations into the structure–activity relationship in gemini QACs based on biphenyl and oxydiphenyl linker†
Received
19th October 2020
, Accepted 25th December 2020
First published on 18th January 2021
Abstract
Eighteen novel gemini quaternary ammonium compounds were synthesized to examine the effect of linker nature, aliphatic chain length and their relative position on antibacterial and antifungal activity. The synthesized compounds showed strong bacteriostatic activity against a panel of both Gram-positive and Gram-negative bacteria, including methicillin-resistant Staphylococcus aureus (MRSA) and two fungi. Some of these compounds exhibited a wider and more potent antimicrobial spectrum than commonly-used antiseptics, such as benzalkonium chloride (BAC), cetylpyridinium chloride (CPC), chlorhexidine digluconate (CHG) and octenidine dihydrochloride (OCT).
Introduction
Quaternary ammonium compounds (QACs) are commonly used in medicine and industry. These cationic surfactants are applied as preservatives, antiseptics and disinfectants.1 The first observations of antimicrobial activity among QAC were published in 1916,2 however full potential of this class of agents wasn't realized until 1930s, when benzalkonium chloride (BAC) and cetyl pyridinium chloride (CPC) were discovered.3
Symmetrical bis-quaternary ammonium compounds (bis-QACs), or gemini QACs, are built of two monomeric QAC molecules linked by a spacer (Fig. 1a).4,5 Bis-QACs activity against microorganisms is generally stronger comparing to corresponding monomeric compounds and depends on structure of the gemini molecule.4c,4g,5d Within a range of bis-QACs, cationic gemini bispyridinium salts play important role being broadly used as biocides. They possess strong antimicrobial effect on Gram-positive and Gram-negative bacteria, fungi and some viruses, even in very low concentrations.6 Usually they consist of two pyridine-containing heads, that are substituted with aliphatic, alkenilic or alkynilic chain (tail) in meta- or para-positions to a spacer. One of the most effective antiseptics – octenidine dihydrochloride – can be an example (Fig. 1b).
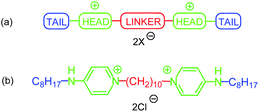 |
| Fig. 1 (a) General structure of cationic gemini surfactants, (b) structure of octenidine. | |
Structure–activity relation studies appear to be one of modern interdisciplinary approaches in organic chemistry.7 Spacers' nature is known to have significant impact on certain effects of bis-QACs’ including biocidal.4h Over the past decades plenty of spacer variations in bispyridinium salts have been obtained and showed antibacterial, antifungal and antimalarial activity.6,8 Amongst others, bis-QACs with benzene ring as a spacer were synthesized, for instance 4DCABP-P,12 (Fig. 2a)6b or 3PHBO-12,Br (Fig. 2b).8e These compounds have same activity with octenidine (MIC and MBC), but better in terms of cytotoxicity (normal human epidermal keratinocytes).9
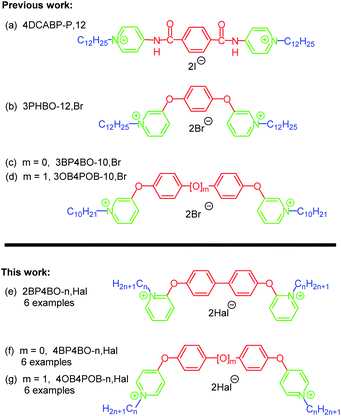 |
| Fig. 2 Structure of cationic gemini surfactants with benzene containing linker. | |
Recently we have got structural analogues of 3PHBO-12, that contain either biphenyl-4,4′-diol10a (3BP4BO-n,Hal; Fig. 2c), or 4,4′-oxydiphenol10b (3OB4POB-n,Hal; Fig. 2d) spacers. MIC values of the hit-compounds against Gram-negative Escherichia coli (ATCC 25922), Klebsiella pneumonia (ATCC 700603), Acinetobacter baumannii (ATCC 19606) and Pseudomonas aeruginosa (ATCC 27853) were lower than that of 3PHBO-12, BAC and CHG.10 It is important that linkers in bis-QACs 3BP4BO-n,Hal and 3OB4POB-n,Hal are in meta-positions to aliphatic tails. The new amphiphiles were obtained via reaction of dibromides (4,4′-dibromobiphenyl or 1,1′-oxybis(4-bromobenzene)) with 3-hydroxypyridine under basic conditions in presence of copper powder (Ullman-type reaction), followed by N-alkylation with alkyl halides of the preformed platforms 3BP4BO and 3OB4POB. Within this study we sought to examine the influence of different positions of the pyridinium head connection with the same aromatic linker on biological activity (Fig. 2e–g).
Results and discussion
Chemistry
Compound design. The aim was first to get ortho- and para-substituted derivatives with biphenyl-4,4′-diol (Scheme 1) and 4,4′-oxydiphenol (Scheme 2) spacers. Initially, the challenge was to synthesize in accordance with our previous work10 the platforms head-spacer-head: 2,2′-[biphenyl-4,4′-diylbis(oxy)]dipyridine (2BP4BO) 2 and 4,4′-[biphenyl-4,4′-diylbis(oxy)]dipyridine (4BP4BO) 9 from 2-hydroxy- and 4-hydroxypyridine respectively. But the approach did not lead to a desired result. We observed the reaction mass tarring. Afterwards an alternative method of head–spacer–head platforms synthesis was applied. We have got 2BP4BO 2 and 4BP4BO 9 from affordable biphenyl-4,4′-diol 1 and 2- and 4-halogenpyridines (Scheme 1) through Ullmann-type reaction.11 Bromopyridines are more reactive than chloropyridines. So, the conversion of diol 1 was 100% after 24 hours of heating in DMSO in inert atmosphere, and 2BP4BO 2 was obtained with 77% yield. When using 2-chloropyridine, full conversion of 1 under given conditions is achieved only after 72 hours and the yield of 2 was 62%. 4-Chloropyridine hydrochloride was used to obtain 4BP4BO 9. To accelerate the reaction its temperature was increased to 140 °C. 4BP4BO 9 was obtained with 85% yield after heating for 72 hours. Similarly, head-spacer-head platforms synthesis 2,2′-[oxybis(4,1-phenyleneoxy)]dipyridine (2OB4POB) 18 and 4,4′-[oxybis(4,1-phenyleneoxy)]dipyridine (4OB4POB) 21 containing a 4,4′-oxydiphenol spacer, have been carried out (Scheme 2). We performed the synthesis of commercially unavailable diol 17 via three steps from biphenyl ether 16 according to known method: Friedel–Crafts acylation of biphenyl ether, oxidation of the diacetyl derivative by mCPBA, alkaline hydrolysis of the resulting diester.12 At the second step of novel gemini amphiphiles synthesis the platforms quaternization with alkyl halides was performed. It was found that the nitrogen position in the pyridinium head relative to the linker is crucial for N-alkylation.
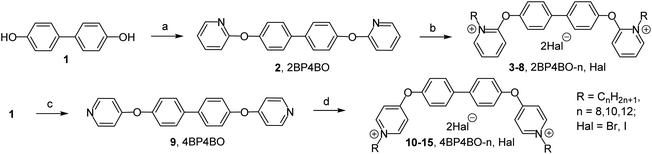 |
| Scheme 1 Reagents and conditions: (a) 2-bromopyridine, CuI(I), K3PO4, picolinic acid, DMSO, 90 °C, argon, 24 h, 77%; (b) RHal, acetonitrile, 82 °C, 7 days, 36–58%; (c) 4-chloropyridine hydrochloride, CuI(I), K3PO4, picolinic acid, DMSO, 140 °C, argon, 72 h, 85%; (d) RHal, acetonitrile, 82 °C, 72 h, 75–82%. | |
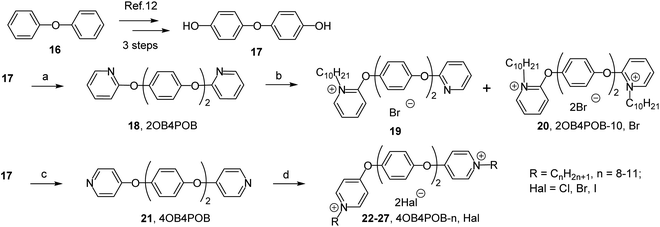 |
| Scheme 2 Reagents and conditions: (a) 2-bromopyridine, CuI(I), K3PO4, picolinic acid, DMSO, 90 °C, argon, 24 h, 77%; (b) RHal, acetonitrile, 82 °C, 7 days, 26–58%; (c) 4-chloropyridine hydrochloride, CuI(I), K3PO4, picolinic acid, DMSO, 140 °C, argon, 72 h, 85%; (d) RHal, acetonitrile, 82 °C, 72 h, 65–82%. | |
Indeed the alkylation process of ortho-platform 2 is very slow even in the presence of alkyl halides excess. 2,2′-[Biphenyl-4,4′-diylbis(oxy)]bis(1-alkylpyridinium) dihalides 2BP4BO-n,Hal 3–8 were obtained with 36–58% yields. For 18 platform alkylation is nonselective. With continuous refluxing of 18 in acetonitrile in excess of alkylbromide a mixture of mono-QAC 19 and bis-QAC 20 was obtained at ratio 1
:
2 (by NMR). The poor reactivity of ‘ortho’ nitrogen is known. For example, various data shows that even with excess of alkyl halide orthoquat alkylation leads to either low yield mono-QAC formation13a or does not occur at all.13b Alkylation of para-platforms 9 and 21 proceeded noticeably better. After 72 hours of refluxing in acetonitrile, complete conversion of the starting compounds and the formation of bis-QACs 10–15 in 75–82% yields and 22–27 in 65–82% yields was observed.
Biological evaluation
Next, we determined the influence of linkers, aliphatic tail length, their relative position and counterion's nature on antibacterial and antifungal activity. In vitro activity against a panel of five bacteria, including both Gram-positive [methicillin-resistant Staphylococcus aureus (MRSA), strain ATCC 43300] and Gram-negative (Escherichia coli, ATCC 25922; Klebsiella pneumoniae, ATCC 700603; Acinetobacter baumannii, ATCC 19606; Pseudomonas aeruginosa, ATCC 27853) strains, two fungi (Candida albicans, ATCC 90028; Cryptococcus neoformans var. Grubii, ATCC 208821), was evaluated for all synthesized ortho- and para-bis-QACs (Table 1). Cytotoxicity on human embryonic kidney cells (HEK-293, ATCC CRL-1573, CC50) and haemolytic activity on human red blood cells (RBC, HC50) were also tested (Table 1). Previously synthesized meta-bis-QACs containing biphenyl10a (3BP4BO-10,Br) and oxydiphenyl10b (3OB4POB-10,Br) linker and commonly-used antiseptics such as benzalkonium chloride (BAC), cetylpyridinium chloride (CPC), chlorhexidine digluconate (CHG) and octenidine dihydrochloride (OCT) were tested as reference compounds. Procedure and materials used in microbiological assays were performed by CO-ADD (the Community for Antimicrobial Drug Discovery).14
Table 1 MIC and cytotoxicity values (μg mL−1) for obtained bis-QACs
Compound |
MICa |
Cytotoxicityb |
Bacteria |
Fungi |
HEK-293 (CC50) |
RBC (HC50) |
MRSA |
E. coli |
K. pneumoniae |
A. baumannii |
P. aeruginosa |
C. albicans |
C. neoformans |
MRSA, methicillin-resistant Staphylococcus aureus (ATCC 43300); E. coli, Escherichia coli (ATCC 25922); K. pneumonia, Klebsiella pneumonia (ATCC 700603); A. baumannii, Acinetobacter baumannii (ATCC 19606); P. aeruginosa, Pseudomonas aeruginosa (ATCC 27853), C. albicans, Candida albicans (ATCC 90028); C. neoformans, Cryptococcus neoformans var. Grubii (ATCC 208821). HEK-293, human embryonic kidney cells (ATCC CRL-1573, CC50); RBC, human red blood cells (HC50). BAC – benzalkonium chloride, CPC – cetylpyridinium chloride, CHG – chlorhexidine digluconate, OCT – octenidine dihydrochloride. |
3, 2BP4BO-8,Br |
≤0.25 |
2 |
8 |
16 |
16 |
2 |
1 |
14.6 |
15.2 |
4, 2BP4BO-8,I |
≤0.25 |
4 |
16 |
32 |
16 |
4 |
8 |
19.3 |
18.4 |
5, 2BP4BO-10,Br |
≤0.25 |
16 |
32 |
16 |
16 |
≤0.25 |
≤0.25 |
12.4 |
2.7 |
6, 2BP4BO-10,I |
0.5 |
32 |
32 |
32 |
32 |
0.5 |
≤0.25 |
7.8 |
5.7 |
7, 2BP4BO-12,Br |
2 |
32 |
>32 |
>32 |
32 |
1 |
≤0.25 |
0.9 |
2.9 |
8, 2BP4BO-12,I |
1 |
32 |
>32 |
>32 |
32 |
0.5 |
0.5 |
3.1 |
2.7 |
10, 4BP4BO-8,Br |
≤0.25 |
≤0.25 |
16 |
16 |
16 |
1 |
1 |
11.6 |
27.0 |
11, 4BP4BO-8,I |
≤0.25 |
≤0.25 |
16 |
16 |
16 |
1 |
1 |
>32 |
>32 |
12, 4BP4BO-10,Br |
≤0.25 |
8 |
16 |
4 |
8 |
≤0.25 |
≤0.25 |
4.8 |
7.2 |
13, 4BP4BO-10,I |
≤0.25 |
8 |
32 |
16 |
16 |
≤0.25 |
1 |
14.4 |
>32 |
14, 4BP4BO-12,Br |
0.5 |
>32 |
>32 |
>32 |
>32 |
0.5 |
≤0.25 |
13.6 |
>32 |
15, 4BP4BO-12,I |
1 |
32 |
>32 |
>32 |
32 |
2 |
0.5 |
16.6 |
>32 |
19 + 20, (2OB4POB-10,Br) |
2 |
>32 |
>32 |
>32 |
>32 |
2 |
4 |
24.6 |
>32 |
22, 4OB4POB-8,Br |
≤0.25 |
≤0.25 |
8 |
16 |
32 |
≤0.25 |
2 |
2.8 |
17.9 |
23, 4OB4POB-9,Br |
≤0.25 |
≤0.25 |
≤0.25 |
≤0.25 |
≤0.25 |
≤0.25 |
4 |
1.2 |
11.9 |
24, 4OB4POB-10,Cl |
≤0.25 |
4 |
32 |
16 |
16 |
≤0.25 |
≤0.25 |
8.5 |
26.0 |
25, 4OB4POB-10,Br |
≤0.25 |
≤0.25 |
4 |
≤0.25 |
4 |
≤0.25 |
16 |
0.4 |
≤0.25 |
26, 4OB4POB-10,I |
≤0.25 |
≤0.25 |
32 |
≤0.25 |
4 |
≤0.25 |
32 |
3.2 |
0.6 |
27, 4OB4POB-11,Br |
≤0.25 |
4 |
>32 |
16 |
16 |
≤0.25 |
32 |
0.5 |
≤0.25 |
BAC |
0.5 |
16 |
>32 |
32 |
>32 |
0.5 |
1 |
2.8 |
3.4 |
CPC |
≤0.25 |
16 |
>32 |
>32 |
32 |
≤0.25 |
≤0.25 |
1.5 |
2.7 |
CHG |
≤0.25 |
1 |
32 |
8 |
8 |
32 |
>32 |
>32 |
>32 |
OCT |
≤0.25 |
≤0.25 |
≤0.25 |
≤0.25 |
0.25 |
≤0.25 |
8 |
1.6 |
4.2 |
3BP4BO-10,Br |
≤0.25 |
1 |
8 |
2 |
4 |
≤0.25 |
≤0.25 |
3.1 |
16.9 |
3OB4POB-10,Br |
≤0.25 |
1 |
4 |
2 |
4 |
≤0.25 |
≤0.25 |
3.2 |
4.3 |
A number of trends can be drawn based on the results of microbiological studies given in Table 1. The vast majority of QACs tested show high activity against MRSA and fungi (especially against C. albicans). Gram-negative bacteria are more resistant to new bis-QACs. The position of nitrogen atoms relative to the linker, as well as the length of the alkyl chain, are the determining factors of activity. Ortho-salts (3–8 and a mixture of 19 + 20) are significantly inferior in efficiency to both para- and meta-derivatives and in some cases are inactive. Bis-QACs with alkyl substituents in the C8–C10 range (compounds 10–13, 22–26) have the broadest spectrum of activity. These gemini amphiphiles are superior to BAC, CPC, and CHG in their activity against Gram-negative bacteria. Among the salts tested, a hit-compound is clearly visible. That is 4,4′-[oxybis(4,1-phenyleneoxy)]bis(1-nonylpyridinium)dibromide 23 containing an oxydiphenyl linker and a C9H19 alkyl substituent. This compound is not inferior to OCT in bacteriostatic effect on the entire spectrum of the studied strains. As well as OCT, it is toxic against HEK-293 and outperforms OCT against RBC. The HC50 of 23 is four times higher than the HC50 of octenidine. There is no clear dependence of the influence of the counterion on activity (see amphiphiles 24–26). However, this dependence is observed in the series of ortho-, meta-, para-derivatives. In general, antibacterial activity decreases in the order meta- > para- > ortho- for bis-QACs containing a biphenyl linker and in the para- > meta- > ortho- order for bis-QACs containing an oxybiphenyl linker.
Conclusions
Thus, a methodology for the simple synthesis of ortho, meta, and para bis-QACs containing biphenyl and oxybiphenyl linkers from available starting material was developed. The antibacterial and antifungal activity of the obtained compounds were studied on five pathogenic bacteria and two yeasts. The effect of the location of the linker relative to the pyridinium head, as well as the length of the alkyl substituent, on microbiological activity were established. In general, for bis-QACs containing a biphenyl linker, antibacterial activity decreases in the order meta- > para- > ortho-. For bis-QACs containing an oxybiphenyl linker, the antibacterial activity decreases in the para- > meta- > ortho- order. The gemini amphiphile 4OB4POB-9,Br, containing an oxydiphenyl linker and a C9H19 alkyl substituent were identified as a hit-compound. This bis-QAC is significantly superior to the widely used BAC, CPC, and CHG in bacteriostatic effect, comparable in activity to OCT, but superior to it in cytotoxicity to human red blood cells. These results indicate that 4OB4POB-9,Br can be successfully used as a new antiseptic.
Experimental
Chemistry
General experimental. Analytical thin layer chromatography (TLC) was performed with EM Science silica gel 60 F254 aluminium plates. Visualisation was carried out using a UV lamp (254 nm). Organic solutions were concentrated by rotary evaporation at 70–80 °C.
Materials. Unless otherwise noted, all purchased materials were used without purification. All standard solvents were purchased from Acros Organics.
Instrumentation. 1H and 13C NMR spectra were recorded on a Bruker AM300 (300 MHz for 1H, 75 MHz for 13C) and Bruker DRX500 (500 MHz for 1H, 125 MHz for 13C) spectrometers at ambient temperature in DMSO-d6 and CDCl3. Chemical shifts are reported relative to residual solvent peaks and coupling constants (J) are given in hertz. Bis-QACs purities were confirmed by HPLC on a Stayer 0892 series HPLC system with Luna® 5 μm C18 100 Å, LC column 250 × 4.6 mm. Mobile phase: 85
:
15 MeCN/H2O (0.25 M NaClO4, 0.1% H3PO4). All melting points were determined on a Gallenkamp melting point apparatus in open capillaries and are uncorrected. Mass spectra were recorded on a Finnigan MAT INCOS 50 mass-spectrometer. IR spectra were recorded with a Bruker ALPHA-T FT-IR spectrometer in KBr pellets.
Compound synthesis
Preparation of 2.
2,2′-[Biphenyl-4,4′-diylbis(oxy)]dipyridine (2BP4BO). The mixture of 4,4′-dihydroxy-1,1′-biphenyl (1) (1.86 g, 10 mmol), 2-bromopyridine (3.16 g, 20 mmol), potassium phosphate (8.48 g, 40 mmol), copper(I) iodide (1.90 g, 10 mmol) and picolinic acid (0.25 g, 2 mmol) in dry DMSO (50 mL) was heated at 90 °C for 24 hours in argon atmosphere. Solvent was removed under reduced pressure, ethyl acetate (50 mL) was added to crude residue, and the mixture was heated to reflux for 1 hour. Then mixture was filtered off. The organic filtrate was concentrated under reduced pressure and the residue was purified by recrystallization from heptane to afford 2,2′-[biphenyl-4,4′-diylbis(oxy)]dipyridine (2) (2.62 g, 77% yield).C22H16N2O2; Mw 340.4; white solid; mp 115–120 °C; 1H NMR (500 MHz, CDCl3): δ 6.99 (d, J = 8.2 Hz, 2H, 2CHpy), 7.04 (t, J = 6.4 Hz, 2H, 2CHpy), 7.23 (d, J = 8.5 Hz, 4H, CHAr), 7.63 (d, J = 8.5 Hz, 4H, CHAr), 7.73 (t, J = 8.2 Hz, 2H, 2CHpy), 8.26 (d, J = 6.4 Hz, 2H, 2CHpy) ppm; 13C NMR (125 MHz, CDCl3): δ 111.7 (2C), 119.2 (2C), 121.7 (4C), 127.9 (4C), 135.9 (2C), 140.3 (2C), 147.5 (2C), 153.4 (2C), 163.0 (2C) ppm.
Preparations of 3–8. Alkyl halide (10 mmol) was added to a solution of 2,2′-[biphenyl-4,4′-diylbis(oxy)]dipyridine (2) (0.34 g, 1 mmol) in acetonitrile (3 mL). The mixture was heated under reflux for 7 days, then allowed to cool to room temperature and filtered off. The solid was washed with 10 mL of cold acetone and dried to give a bis-QAC. The yields of bis-QACs 3–8 were 36–58% depend on alkyl halide.
2,2′-[Biphenyl-4,4′-diylbis(oxy)]bis(1-octylpyridinium)dibromide (3, 2BP4BO-8,Br). C38H50Br2N2O2; Mw 726.6; white solid (0.28 g, 38% yield); mp 123–125 °C; 1H NMR (300 MHz, DMSO-d6): δ 0.84 (t, J = 6.4 Hz, 6H, 2CH3), 1.18–1.51 (m, 20H, 10CH2), 1.91–2.07 (m, 4H, 2CH2), 4.68 (t, J = 6.8 Hz, 4H, 2CH2N+), 7.31 (d, J = 8.7 Hz, 2H, 2CHpy), 7.65 (d, J = 8.4 Hz, 4H, CHAr), 7.78 (t, J = 6.4 Hz, 2H, 2CHpy), 8.01 (d, J = 8.4 Hz, 4H, CHAr), 8.50 (t, J = 8.7 Hz, 2H, 2CHpy), 9.04 (d, J = 6.4 Hz, 2H, 2CHpy) ppm; 13C NMR (75 MHz, DMSO-d6): δ 13.9 (2C), 21.9 (2C), 25.6 (2C), 28.3 (2C), 28.4 (2C), 28.6 (2C), 31.1 (2C), 54.7 (2C), 113.5 (2C), 120.5 (2C), 121.6 (4C), 129.3 (4C), 138.1 (2C), 143.6 (2C), 148.4 (2C), 151.3 (2C), 158.8 (2C) ppm; m/z (%): 340 (100), 312 (14), 284 (7), 262 (6), 234 (4), 78 (8), 51 (3), 44 (2), 28 (4), 18 (6); νmax, (KBr): 3421, 2926, 2856, 1633, 1581, 1491, 1463, 1306, 1159, 781 cm−1.
2,2′-[Biphenyl-4,4′-diylbis(oxy)]bis(1-octylpyridinium)diiodide (4, 2BP4BO-8,I). C38H50I2N2O2; Mw 820.6; yellow solid (0.39 g, 48% yield); mp 194–198 °C; 1H NMR (300 MHz, DMSO-d6): δ 0.85 (t, J = 6.4 Hz, 6H, 2CH3), 1.18–1.47 (m, 20H, 10CH2), 1.91–2.05 (m, 4H, 2CH2), 4.64 (t, J = 6.8 Hz, 4H, 2CH2N+), 7.31 (d, J = 8.7 Hz, 2H, 2CHpy), 7.63 (d, J = 8.4 Hz, 4H, CHAr), 7.77 (t, J = 6.4 Hz, 2H, 2CHpy), 8.00 (d, J = 8.4 Hz, 4H, CHAr), 8.46 (t, J = 8.7 Hz, 2H, 2CHpy), 8.92 (d, J = 6.4 Hz, 2H, 2CHpy) ppm; 13C NMR (75 MHz, DMSO-d6): δ 13.9 (2C), 21.9 (2C), 25.6 (2C), 28.3 (2C), 28.4 (2C), 28.6 (2C), 31.1 (2C), 54.7 (2C), 113.5 (2C), 120.5 (2C), 121.6 (4C), 129.3 (4C), 138.1 (2C), 143.5 (2C), 148.4 (2C), 151.3 (2C), 158.8 (2C) ppm; m/z (%): 340 (100), 312 (16), 284 (9), 262 (8), 240 (10), 206 (8), 71 (41), 57 (26), 43 (24), 29 (10); νmax, (KBr): 3442, 2926, 2855, 1633, 1581, 1490, 1308, 1194, 1156, 773 cm−1.
2,2′-[Biphenyl-4,4′-diylbis(oxy)]bis(1-decylpyridinium)dibromide (5, 2BP4BO-10,Br). C42H58Br2N2O2; Mw 782.8; white solid (0.33 g, 42% yield); mp 174–175 °C; 1H NMR (300 MHz, DMSO-d6): δ 0.83 (t, J = 6.4 Hz, 6H, 2CH3), 1.18–1.49 (m, 28H, 14CH2), 1.91–2.05 (m, 4H, 2CH2), 4.64 (t, J = 6.8 Hz, 4H, 2CH2N+), 7.31 (d, J = 8.7 Hz, 2H, 2CHpy), 7.63 (d, J = 8.4 Hz, 4H, CHAr), 7.77 (t, J = 6.4 Hz, 2H, 2CHpy), 8.00 (d, J = 8.4 Hz, 4H, CHAr), 8.47 (t, J = 8.7 Hz, 2H, 2CHpy), 8.95 (d, J = 6.4 Hz, 2H, 2CHpy) ppm; 13C NMR (75 MHz, DMSO-d6): δ 13.9 (2C), 21.9 (2C), 25.6 (2C), 28.3 (2C), 28.6 (2C), 28.7 (4C), 28.8 (2C), 31.2 (2C), 54.7 (2C), 113.5 (2C), 120.5 (2C), 121.6 (4C), 129.3 (4C), 138.1 (2C), 143.6 (2C), 148.4 (2C), 151.3 (2C), 158.8 (2C) ppm; m/z (%): 340 (100), 312 (29), 284 (15), 262 (9), 234 (9), 137 (14), 78 (24), 71 (3), 55 (12), 41 (11); νmax, (KBr): 3433, 2923, 2854, 1632, 1579, 1491, 1463, 1302, 1155, 782 cm−1.
2,2′-[Biphenyl-4,4′-diylbis(oxy)]bis(1-decylpyridinium)diiodide (6, 2BP4BO-10,I). C42H58I2N2O2; Mw 876.8; yellow solid (0.35 g, 40% yield); mp 185–188 °C; 1H NMR (300 MHz, DMSO-d6): δ 0.83 (t, J = 6.4 Hz, 6H, 2CH3), 1.18–1.49 (m, 28H, 14CH2), 1.91–2.05 (m, 4H, 2CH2), 4.64 (t, J = 6.8 Hz, 4H, 2CH2N+), 7.31 (d, J = 8.7 Hz, 2H, 2CHpy), 7.63 (d, J = 8.4 Hz, 4H, CHAr), 7.76 (t, J = 6.4 Hz, 2H, 2CHpy), 8.00 (d, J = 8.4 Hz, 4H, CHAr), 8.46 (t, J = 8.7 Hz, 2H, 2CHpy), 8.92 (d, J = 6.4 Hz, 2H, 2CHpy) ppm; 13C NMR (75 MHz, DMSO-d6): δ 13.8 (2C), 21.9 (2C), 25.6 (2C), 28.3 (2C), 28.6 (C), 28.7 (4C), 28.8 (2C), 31.2 (2C), 54.7 (2C), 113.5 (2C), 120.5 (2C), 121.6 (4C), 129.3 (4C), 138.0 (2C), 143.5 (2C), 148.3 (2C), 151.2 (2C), 158.8 (2C) ppm; m/z (%): 340 (28), 268 (25), 155 (22), 141 (22), 127 (14), 85 (89), 71 (70), 57 (100), 43 (93), 29 (39); νmax, (KBr): 3427, 2925, 2853, 1634, 1581, 1490, 1462, 1307, 1156, 772 cm−1.
2,2′-[Biphenyl-4,4′-diylbis(oxy)]bis(1-dodecylpyridinium)dibromide (7, 2BP4BO-12,Br). C46H66Br2N2O2; Mw 838.9; white solid (0.3 g, 36% yield); mp 190–192 °C; 1H NMR (300 MHz, DMSO-d6): δ 0.83 (t, J = 6.4 Hz, 6H, 2CH3), 1.18–1.49 (m, 36H, 18CH2), 1.91–2.02 (m, 4H, 2CH2), 4.64 (t, J = 6.8 Hz, 4H, 2CH2N+), 7.30 (d, J = 8.7 Hz, 2H, 2CHpy), 7.62 (d, J = 8.4 Hz, 4H, CHAr), 7.76 (t, J = 6.4 Hz, 2H, 2CHpy), 8.01 (d, J = 8.4 Hz, 4H, CHAr), 8.47 (t, J = 8.7 Hz, 2H, 2CHpy), 8.94 (d, J = 6.4 Hz, 2H, 2CHpy) ppm; 13C NMR (75 MHz, DMSO-d6): δ 13.8 (2C), 22.0 (2C), 25.6 (2C), 28.3 (2C), 28.6 (C), 28.7 (2C), 28.8 (4C), 28.9 (4C), 31.2 (2C), 54.7 (2C), 113.5 (2C), 120.5 (2C), 121.6 (4C), 129.3 (4C), 138.0 (2C), 143.5 (2C), 148.3 (2C), 151.4 (2C), 158.7 (2C) ppm; m/z (%): 340 (74), 311 (9), 284 (6), 263 (3), 149 (8), 137 (56), 99 (3), 85 (4), 57 (10), 43 (100); νmax, (KBr): 3433, 2922, 2853, 1632, 1579, 1490, 1464, 1298, 1154, 782 cm−1.
2,2′-[Biphenyl-4,4′-diylbis(oxy)]bis(1-dodecylpyridinium)diiodide (8, 2BP4BO-12,I). C46H66I2N2O2; Mw 932.9; yellow solid (0.54 g, 58% yield); mp 233–234 °C; 1H NMR (300 MHz, DMSO-d6): δ 0.83 (t, J = 6.4 Hz, 6H, 2CH3), 1.18–1.49 (m, 36H, 18CH2), 1.91–2.02 (m, 4H, 2CH2), 4.63 (t, J = 6.8 Hz, 4H, 2CH2N+), 7.30 (d, J = 8.7 Hz, 2H, 2CHpy), 7.62 (d, J = 8.4 Hz, 4H, CHAr), 7.76 (t, J = 6.4 Hz, 2H, 2CHpy), 8.00 (d, J = 8.4 Hz, 4H, CHAr), 8.46 (t, J = 8.7 Hz, 2H, 2CHpy), 8.92 (d, J = 6.4 Hz, 2H, 2CHpy) ppm; 13C NMR (75 MHz, DMSO-d6): δ 13.8 (2C), 21.9 (2C), 25.6 (2C), 28.3 (2C), 28.6 (C), 28.7 (4C), 28.8 (4C), 28.9 (2C), 31.2 (2C), 54.5 (2C), 113.5 (2C), 120.5 (2C), 121.6 (4C), 129.3 (4C), 138.0 (2C), 143.5 (2C), 148.3 (2C), 151.2 (2C), 158.8 (2C) ppm; m/z (%): 340 (100), 312 (16), 284 (14), 169 (6), 155 (6), 127 (7), 85 (23), 71 (10), 57 (14), 43 (11); νmax, (KBr): 3444, 2924, 2853, 1634, 1581, 1491, 1462, 1306, 1157, 773 cm−1.
Preparation of 9.
4,4′-[Biphenyl-4,4′-diylbis(oxy)]dipyridine (4BP4BO). The mixture of 4,4′-dihydroxy-1,1′-biphenyl (1.86 g, 10 mmol), 4-chloropyridine hydrochloride (3.00 g, 20 mmol), potassium phosphate (16.96 g, 80 mmol), copper(I) iodide (1.90 g, 10 mmol) and picolinic acid (0.25 g, 2 mmol) in dry DMSO (50 mL) was heated to 140 °C for 72 hours in argon atmosphere. Solvent was removed under reduced pressure, ethyl acetate (50 mL) was added to crude residue, and the mixture was heated to reflux for 1 hour. Then mixture was filtered. The organic filtrate was concentrated under reduced pressure and the residue was purified by recrystallization from heptane to afford 4,4′-[biphenyl-4,4′-diylbis(oxy)]dipyridine (9) (2.89 g, 85% yield).C22H16N2O2; Mw 340.4; white solid; mp 138–142 °C; 1H NMR (300 MHz, CDCl3): δ 6.92 (d, J = 6.9 Hz, 4H, 4CHpy), 7.20 (d, J = 8.4 Hz, 4H, 4CHAr), 7.65 (d, J = 8.4 Hz, 4H, 4CHAr), 8.52 (d, J = 6.9 Hz, 4H, 4CHpy) ppm; 13C NMR (75 MHz, CDCl3): δ 121.0 (4C), 128.6 (4C), 136.4 (4C), 138.3 (2C), 151.7 (2C), 153.2 (4C), 163.7 (2C) ppm.
Preparations of 10–15. Alkyl halide (2.2 mmol) was added to a solution of 4,4′-[biphenyl-4,4′-diylbis(oxy)]dipyridine (9) (0.34 g, 1 mmol) in acetonitrile (3 mL). The mixture was heated under reflux for 72 h, then allowed to cool to room temperature and filtered off. The solid was washed with 10 mL of cold acetone and dried to give a bis-QAC. The yields of bis-QACs 10–15 were 36–58% depend on alkyl halide.
4,4′-[Biphenyl-4,4′-diylbis(oxy)]bis(1-octylpyridinium)dibromide (10, 4BP4BO-8,Br). C38H50Br2N2O2; Mw 726.6; white solid (0.55 g, 75% yield); mp 245–247 °C; 1H NMR (300 MHz, DMSO-d6): δ 0.85 (t, J = 6.6 Hz, 6H, 2CH3), 1.17–1.42 (m, 20H, 10CH2), 1.81–1.93 (m, 4H, 2CH2), 4.52 (t, J = 7.3 Hz, 4H, 2CH2N+), 7.49 (d, J = 8.4 Hz, 4H, 4CHAr), 7.62 (d, J = 6.9 Hz, 4H, 4CHpy), 7.95 (d, J = 8.4 Hz, 4H, 4CHAr), 9.01 (d, J = 6.9 Hz, 4H, 4CHpy) ppm; 13C NMR (75 MHz, DMSO-d6): δ 13.9 (2C), 22.1 (2C), 25.4 (2C), 28.4 (2C), 28.5 (2C), 30.7 (2C), 31.2 (2C), 59.1 (2C), 114.8 (4C), 121.6 (4C), 129.3 (4C), 137.8 (2C), 147.0 (4C), 151.9 (2C), 169.1 (2C) ppm; m/z (%): 340 (77), 263 (100), 185 (26), 157 (28), 135 (66), 128 (10), 71 (34), 57 (53), 51 (25), 43 (86); νmax, (KBr): 3454, 2927, 2854, 1642, 1487, 1284, 1201, 1007, 888, 845 cm−1.
4,4′-[Biphenyl-4,4′-diylbis(oxy)]bis(1-octylpyridinium)diiodide (11, 4BP4BO-8,I). C38H50I2N2O2; Mw 820.6; yellow solid (0.63 g, 77% yield); mp 174–177 °C; 1H NMR (300 MHz, DMSO-d6): δ 0.85 (t, J = 6.6 Hz, 6H, 2CH3), 1.17–1.42 (m, 20H, 10CH2), 1.79–1.93 (m, 4H, 2CH2), 4.49 (t, J = 7.3 Hz, 4H, 2CH2N+), 7.49 (d, J = 8.4 Hz, 4H, 4CHAr), 7.62 (d, J = 6.9 Hz, 4H, 4CHpy), 7.94 (d, J = 8.4 Hz, 4H, 4CHAr), 8.95 (d, J = 6.9 Hz, 4H, 4CHpy) ppm; 13C NMR (75 MHz, DMSO-d6): δ 13.8 (2C), 21.9 (2C), 25.2 (2C), 28.3 (2C), 28.4 (2C), 30.5 (2C), 31.0 (2C), 59.1 (2C), 114.8 (4C), 121.5 (4C), 129.2 (4C), 137.7 (2C), 146.8 (4C), 151.8 (2C), 169.0 (2C) ppm; m/z (%): 340 (79), 263 (63), 240 (12), 185 (20), 157 (25), 127 (16), 78 (25), 71 (68), 57 (89), 43 (100); νmax, (KBr): 3428, 2927, 2855, 1641, 1486, 1289, 1198, 1007, 889, 848 cm−1.
4,4′-[Biphenyl-4,4′-diylbis(oxy)]bis(1-decylpyridinium)dibromide (12, 4BP4BO-10,Br). C42H58Br2N2O2; Mw 782.8; white solid (0.52 g, 67% yield); mp 225–227 °C; 1H NMR (300 MHz, DMSO-d6): δ 0.85 (t, J = 6.6 Hz, 6H, 2CH3), 1.17–1.42 (m, 28H, 14CH2), 1.81–1.93 (m, 4H, 2CH2), 4.51 (t, J = 7.3 Hz, 4H, 2CH2N+), 7.49 (d, J = 8.4 Hz, 4H, 4CHAr), 7.62 (d, J = 6.9 Hz, 4H, 4CHpy), 7.95 (d, J = 8.4 Hz, 4H, 4CHAr), 8.99 (d, J = 6.9 Hz, 4H, 4CHpy) ppm; 13C NMR (75 MHz, DMSO-d6): δ 13.9 (2C), 22.0 (2C), 25.3 (2C), 28.3 (2C), 28.6 (2C), 28.7 (2C), 28.8 (2C), 30.6 (2C), 31.2 (2C), 59.1 (2C), 114.8 (4C), 121.5 (4C), 129.2 (4C), 137.7 (2C), 146.9 (4C), 151.8 (2C), 169.0 (2C) ppm; m/z (%): 340 (100), 263 (44), 185 (5), 157 (7), 137 (31), 85 (8), 78 (6), 69 (11), 57 (20), 43 (25); νmax, (KBr): 3451, 2923, 2854, 1640, 1486, 1289, 1200, 1006, 890, 844 cm−1.
4,4′-[Biphenyl-4,4′-diylbis(oxy)]bis(1-decylpyridinium)diiodide (13, 4BP4BO-10,I). C42H58I2N2O2; Mw 876.8; yellow solid (0.64 g, 73% yield); mp 232–236 °C; 1H NMR (300 MHz, DMSO-d6): δ 0.85 (t, J = 6.6 Hz, 6H, 2CH3), 1.17–1.42 (m, 28H, 14CH2), 1.81–1.93 (m, 4H, 2CH2), 4.49 (t, J = 7.3 Hz, 4H, 2CH2N+), 7.48 (d, J = 8.4 Hz, 4H, 4CHAr), 7.62 (d, J = 6.9 Hz, 4H, 4CHpy), 7.95 (d, J = 8.4 Hz, 4H, 4CHAr), 8.94 (d, J = 6.9 Hz, 4H, 4CHpy) ppm; 13C NMR (75 MHz, DMSO-d6): δ 13.9 (2C), 22.0 (2C), 25.2 (2C), 28.3 (2C), 28.6 (2C), 28.7 (2C), 28.8 (2C), 30.5 (2C), 31.2 (2C), 59.1 (2C), 114.8 (4C), 121.5 (4C), 129.2 (4C), 137.7 (2C), 146.8 (4C), 151.8 (2C), 169.0 (2C) ppm; m/z (%): 340 (94), 263 (73), 185 (11), 157 (18), 141 (12), 128 (12), 85 (52), 71 (53), 57 (100), 43 (98); νmax, (KBr): 3451, 2924, 2848, 1642, 1485, 1285, 1199, 1009, 889, 843 cm−1.
4,4′-[Biphenyl-4,4′-diylbis(oxy)]bis(1-dodecylpyridinium)dibromide (14, 4BP4BO-12,Br). C46H66Br2N2O2; Mw 838.9; white solid (0.59 g, 70% yield); mp 248–251 °C; 1H NMR (300 MHz, DMSO-d6): δ 0.85 (t, J = 6.6 Hz, 6H, 2CH3), 1.17–1.42 (m, 36H, 18CH2), 1.81–1.93 (m, 4H, 2CH2), 4.51 (t, J = 7.3 Hz, 4H, 2CH2N+), 7.49 (d, J = 8.4 Hz, 4H, 4CHAr), 7.62 (d, J = 6.9 Hz, 4H, 4CHpy), 7.95 (d, J = 8.4 Hz, 4H, 4CHAr), 8.99 (d, J = 6.9 Hz, 4H, 4CHpy) ppm; 13C NMR (75 MHz, DMSO-d6): δ 13.9 (2C), 22.0 (2C), 25.3 (2C), 28.4 (2C), 28.6 (2C), 28.7 (2C), 28.8 (4C), 28.9 (2C), 30.6 (2C), 31.2 (2C), 59.0 (2C), 114.7 (4C), 121.5 (4C), 129.2 (4C), 137.7 (2C), 146.9 (4C), 151.8 (2C), 169.0 (2C) ppm; 340 (100), 263 (52), 185 (6), 157 (13), 135 (5), 128 (10), 71 (34), 57 (53), 51 (15), 43 (86); νmax, (KBr): 3478, 2920, 2853, 1641, 1484, 1293, 1203, 1007, 889, 833 cm−1.
4,4′-[Biphenyl-4,4′-diylbis(oxy)]bis(1-dodecylpyridinium)diiodide (15, 4BP4BO-12,I). C46H66I2N2O2; Mw 932.9; yellow solid (0.76 g, 82% yield); mp 225–228 °C; 1H NMR (300 MHz, DMSO-d6): δ 0.85 (t, J = 6.6 Hz, 6H, 2CH3), 1.17–1.42 (m, 36H, 18CH2), 1.81–1.93 (m, 4H, 2CH2), 4.49 (t, J = 7.3 Hz, 4H, 2CH2N+), 7.49 (d, J = 8.4 Hz, 4H, 4CHAr), 7.62 (d, J = 6.9 Hz, 4H, 4CHpy), 7.95 (d, J = 8.4 Hz, 4H, 4CHAr), 8.95 (d, J = 6.9 Hz, 4H, 4CHpy) ppm; 13C NMR (75 MHz, DMSO-d6): δ 13.9 (2C), 22.0 (2C), 25.3 (2C), 28.3 (2C), 28.6 (2C), 28.7 (2C), 28.8 (4C), 28.9 (2C), 30.5 (2C), 31.2 (2C), 59.1 (2C), 114.8 (4C), 121.5 (4C), 129.2 (4C), 137.7 (2C), 146.8 (4C), 151.8 (2C), 169.0 (2C) ppm; m/z (%): 340 (15), 263 (40), 185 (9), 157 (11), 128 (12), 85 (36), 78 (11), 71 (34), 57 (85), 43 (100); νmax, (KBr): 3429, 2922, 2850, 1641, 1486, 1279, 1202, 1013, 887, 844 cm−1.
Preparation of 4,4′-diacetyldiphenyl ether. The mixture of diphenyl ether 16 (10.20 g, 60 mmol), acetyl chloride (9.42 g, 120 mmol) and DCM (50 mL) was slowly added to the suspension of aluminium chloride (9.42 g, 120 mmol) in DCM (50 mL) at 0 °C. After stirring for 1 d at rt, water (250 mL) was added. The resulting solution was extracted with chloroform (3 × 100 mL). The combined organic layers was washed with saturated aqueous sodium bicarbonate (50 mL) and dried over sodium sulfate. Then solvent was removed under reduced pressure to give 4,4′-diacetyldiphenyl ether (12.8 g, 84% yield).C16H14O3; Mw 254.3; white solid; mp 99–101 °C; 1H NMR (300 MHz, CDCl3): δ 2.61 (s, 6H, 2CH3), 7.10 (d, J = 8.8 Hz, 4H, 4CHAr), 8.01 (d, J = 8.8 Hz, 4H, 4CHAr) ppm.
Preparation of 4,4′-diacetoxydiphenyl ether. The mixture of 4,4′-diacetyldiphenyl ether (7.62 g, 30 mmol), mCPBA (30.4 g, 176 mmol) and DCM (150 mL) was stirred for 5 h at rt. The resulting solution was filtered. Filtrate was washed with aqueous sodium bicarbonate (50 mL) and dried over sodium sulfate. Then solvent was removed under reduced pressure to give 4,4′-diacetoxydiphenyl ether (7.46 g, 87% yield).C16H14O5; Mw 286.3; white solid; mp 109–110 °C; 1H NMR (300 MHz, CDCl3): δ 2.31 (s, 6H, 2CH3), 7.02 (d, J = 9.1 Hz, 4H, 4CHAr), 7.07 (d, J = 9.1 Hz, 4H, 4CHAr) ppm.
Preparation of 17.
4,4′-Dihydroxydiphenyl ether. The 25% solution of sodium hydroxide (2.0 g, 50 mmol) in water was slowly added to solution of 4,4′-diacetyldiphenyl ether (6.62 g, 23.2 mmol) in methanol (60 mL). After stirring for 2 h solvent was removed under reduced pressure. Then water was added to crude residue, and resulting solution was treated with hydrochloric acid until the end of precipitation. Then mixture was filtered. The solid remaining on the filter was washed with 100 mL of water, refluxed in hexane (100 mL), filtered off and dried to provide 4,4′-dihydroxydiphenyl ether (17) (4.22 g, 90% yield).C12H10O3; Mw 202.21; white solid; mp 162–164 °C; 1H NMR (300 MHz, CDCl3): 6.71 (d, J = 9.0 Hz, 4H, 4CHAr), 6.77 (d, J = 9.0 Hz, 4H, 4CHAr), 9.16 (s, 1H, OH) ppm.
Preparation of 18.
2,2′-[Oxybis(4,1-phenyleneoxy)]dipyridine (2OB4POB). The mixture of 4,4′-dihydroxydiphenyl ether (17) (2.02 g, 10 mmol), 2-bromopyridine (3.16 g, 20 mmol), potassium phosphate (8.48 g, 40 mmol), copper(I) iodide (1.90 g, 10 mmol) and picolinic acid (0.25 g, 2 mmol) in dry DMSO (50 mL) was heated to 90 °C for 24 hours in argon atmosphere. Solvent was removed under reduced pressure, ethyl acetate (50 mL) was added to crude residue, and the mixture was heated to reflux for 1 hour. Then mixture was filtered off. The organic filtrate was concentrated under reduced pressure and the residue was purified by recrystallization from heptane to afford 18 (2.37 g, 65% yield).C22H16N2O3; Mw 356.4; white solid; mp 95–98 °C; 1H NMR (300 MHz, CDCl3): δ 6.93 (d, J = 8.5 Hz, 2H, 2CHpy), 7.01 (t, J = 6.4 Hz, 2H, 2CHpy), 7.08 (d, J = 9.1 Hz, 4H, CHAr), 7.14 (d, J = 9.1 Hz, 4H, CHAr), 7.70 (t, J = 8.5 Hz, 2H, 2CHpy), 8.22 (d, J = 6.4 Hz, 2H, 2CHpy) ppm; 13C NMR (75 MHz, DMSO-d6): δ 111.2 (2C), 118.9 (2C), 119.5 (4C), 122.7 (4C), 140.1 (2C), 147.3 (2C), 149.3 (2C), 153.4 (2C), 163.1 (2C) ppm.
Preparation of mixture 19 + 20. Decyl bromide (10 mmol) was added to a solution of 2,2′-[oxybis(4,1-phenyleneoxy)]dipyridine (18) (0.36 g, 1 mmol) in acetonitrile (3 mL). The mixture was heated under reflux for 7 days, then allowed to cool to room temperature and filtered off. The filtered solid was washed with 5 mL of cold acetone and dried to give a mixture of mono- 19 and bis-QAC 20 with ratio 1
:
2 (by NMR).
Preparation of 21.
4,4′-[Oxybis(4,1-phenyleneoxy)]dipyridine (4OB4POB). The mixture of 4,4′-dihydroxydiphenyl ether (17) (2.02 g, 10 mmol), 4-chloropyridine hydrochloride (3.00 g, 20 mmol), potassium phosphate (16.96 g, 10 mmol) and picolinic acid (0.25 g, 2 mmol) in dry DMSO (50 mL) was heated to 140 °C for 72 hours in argon atmosphere. Solvent was removed under reduced pressure, ethyl acetate (50 mL) was added to crude residue, and the mixture was heated to reflux for 1 hour. Then mixture was filtered off. The organic filtrate was concentrated under reduced pressure and the residue was purified by recrystallization in heptane to afford 4,4′-[oxybis(4,1-phenyleneoxy)]dipyridine (21) (3.17 g, 89% yield).C22H16N2O3; Mw 356.4; white solid; mp 104–106 °C; 1H NMR (300 MHz, CDCl3): δ 6.86 (d, J = 6.9 Hz, 4H, 4CHpy), 7.11 (m, 8H, 8CHAr), 8.50 (d, J = 6.9 Hz, 4H, 4CHpy) ppm; 13C NMR (75 MHz, CDCl3): δ 121.0 (4C), 128.6 (4C), 136.4 (4C), 138.3 (2C), 151.7 (2C), 153.2 (4C), 163.7 (2C) ppm. 13C NMR (75 MHz, CDCl3): δ 112.0 (2C), 120.3 (4C), 122.3 (4C), 149.6 (2C), 151.5 (4C), 154.5 (4C), 164.9 (2C) ppm.
Preparations of 22–27. Alkyl halide (2.2 mmol) was added to a solution of 4,4′-[biphenyl-4,4′-diylbis(oxy)]dipyridin (21) (0.36 g, 1 mmol) in acetonitrile (3 mL). The mixture was heated under reflux for 72 h, then allowed to cool to room temperature and filtered off. The solid was washed with 10 mL of cold acetone and dried to give a bis-QAC. The yields of bis-QACs 22–27 were 65–82% depend on alkyl halide.
4,4′-[Oxybis(4,1-phenyleneoxy)]bis(1-octylpyridinium)dibromide (22, 4OB4POB-8,Br). C38H50Br2N2O3; Mw 742.6; white solid (0.56 g, 75% yield); mp 68–71 °C; 1H NMR (300 MHz, DMSO-d6): δ 0.85 (t, J = 6.6 Hz, 6H, 2CH3), 1.17–1.42 (m, 20H, 10CH2), 1.81–1.93 (m, 4H, 2CH2), 4.50 (t, J = 7.0 Hz, 4H, 2CH2N+), 7.29 (d, J = 8.4 Hz, 4H, 4CHAr), 7.42 (d, J = 8.4 Hz, 4H, 4CHAr), 7.61 (d, J = 6.9 Hz, 4H, 4CHpy), 8.96 (d, J = 6.9 Hz, 4H, 4CHpy) ppm; 13C NMR (75 MHz, DMSO-d6): δ 14.0 (2C), 22.1 (2C), 25.4 (2C), 28.5 (2C), 28.6 (2C), 30.7 (2C), 31.3 (2C), 59.1 (2C), 114.7 (4C), 121.0 (4C), 122.9 (4C), 147.0 (4C), 147.8 (2C), 155.1 (2C), 169.5 (2C) ppm; m/z (%): 356 (100), 279 (28), 171 (4), 137 (30), 85 (6), 78 (7), 69 (5), 55 (10), 43 (13), 29 (4); νmax, (KBr): 3423, 2926, 2855, 1641, 1486, 1294, 1241, 1182, 883, 852 cm−1.
4,4′-[Oxybis(4,1-phenyleneoxy)]bis(1-nonylpyridinium)dibromide (23, 4OB4POB-9,Br). C40H54Br2N2O3; Mw 770.7; white solid (0.55 g, 72% yield); mp 78–82 °C; 1H NMR (300 MHz, DMSO-d6): δ 0.85 (t, J = 6.6 Hz, 6H, 2CH3), 1.17–1.42 (m, 24H, 12CH2), 1.81–1.93 (m, 4H, 2CH2), 4.50 (t, J = 7.0 Hz, 4H, 2CH2N+), 7.29 (d, J = 8.4 Hz, 4H, 4CHAr), 7.42 (d, J = 8.4 Hz, 4H, 4CHAr), 7.61 (d, J = 6.9 Hz, 4H, 4CHpy), 8.96 (d, J = 6.9 Hz, 4H, 4CHpy) ppm; 13C NMR (75 MHz, DMSO-d6): δ 14.0 (2C), 22.1 (2C), 25.4 (2C), 28.5 (2C), 28.6 (2C), 28.8 (2C), 30.7 (2C), 31.3 (2C), 59.1 (2C), 114.7 (4C), 121.0 (4C), 122.9 (4C), 147.0 (4C), 147.8 (2C), 155.1 (2C), 169.5 (2C) ppm; m/z (%): 356 (100), 279 (29), 171 (4), 137 (22), 85 (6), 78 (3), 69 (7), 55 (10), 43 (13), 29 (8); νmax, (KBr): 3424, 2926, 2855, 1641, 1488, 1293, 1241, 1182, 883, 852 cm−1.
4,4′-[Oxybis(4,1-phenyleneoxy)]bis(1-decylpyridinium)dibromide (24, 4OB4POB-10,Br). C42H58Br2N2O3; Mw 798.8; white solid (0.60 g, 75% yield); mp 93–104 °C; 1H NMR (300 MHz, DMSO-d6): δ 0.85 (t, J = 6.6 Hz, 6H, 2H3), 1.17–1.42 (m, 28H, 14CH2), 1.81–1.93 (m, 4H, 2CH2), 4.50 (t, J = 7.0 Hz, 4H, 2CH2N+), 7.29 (d, J = 8.4 Hz, 4H, 4CHAr), 7.42 (d, J = 8.4 Hz, 4H, 4CHAr), 7.61 (d, J = 6.9 Hz, 4H, 4CHpy), 8.96 (d, J = 6.9 Hz, 4H, 4CHpy) ppm; 13C NMR (75 MHz, DMSO-d6): δ 14.0 (2C), 22.1 (2C), 25.4 (2C), 28.5 (2C), 28.7 (2C), 28.8 (2C), 28.9 (2C), 30.7 (2C), 31.3 (2C), 59.1 (2C), 114.7 (4C), 121.0 (4C), 122.9 (4C), 147.0 (4C), 147.8 (2C), 155.1 (2C), 169.5 (2C) ppm; m/z (%): 356 (100), 279 (29), 151 (6), 137 (36), 85 (6), 69 (5), 55 (8), 43 (11), 29 (7), 18 (6); νmax, (KBr): 3422, 2925, 2854, 1641, 1488, 1294, 1242, 1183, 883, 852 cm−1.
4,4′-[Oxybis(4,1-phenyleneoxy)]bis(1-decylpyridinium)dichloride (25, 4OB4POB-10,Cl). C42H58Cl2N2O; Mw 709.8; white solid (0.46 g, 65% yield); mp 90–93 °C; 1H NMR (300 MHz, DMSO-d6): δ 0.85 (t, J = 6.6 Hz, 6H, 2CH3), 1.17–1.42 (m, 28H, 14CH2), 1.81–1.93 (m, 4H, 2CH2), 4.50 (t, J = 7.0 Hz, 4H, 2CH2N+), 7.29 (d, J = 8.4 Hz, 4H, 4CHAr), 7.42 (d, J = 8.4 Hz, 4H, 4CHAr), 7.61 (d, J = 6.9 Hz, 4H, 4CHpy), 8.96 (d, J = 6.9 Hz, 4H, 4CHpy) ppm; 13C NMR (75 MHz, DMSO-d6): δ 14.0 (2C), 22.1 (2C), 25.4 (2C), 28.5 (2C), 28.7 (2C), 28.8 (2C), 28.9 (2C), 30.7 (2C), 31.3 (2C), 59.1 (2C), 114.7 (4C), 121.0 (4C), 122.9 (4C), 147.0 (4C), 147.8 (2C), 155.1 (2C), 169.5 (2C) ppm; m/z (%): 356 (100), 279 (25), 149 (10), 135 (34), 78 (9), 69 (8), 57 (19), 51 (13), 43 (25), 29 (9); νmax, (KBr): 3424, 2926, 2855, 1641, 1488, 1293, 1241, 1182, 883, 852 cm−1.
4,4′-[Oxybis(4,1-phenyleneoxy)]bis(1-decylpyridinium)diiodide (26, 4OB4POB-10,I). C42H58I2N2O3; Mw 892.8; yellow solid (0.73 g, 82% yield); mp 134–138 °C; 1H NMR (300 MHz, DMSO-d6): δ 0.85 (t, J = 6.6 Hz, 6H, 2CH3), 1.17–1.42 (m, 28H, 14CH2), 1.81–1.93 (m, 4H, 2CH2), 4.48 (t, J = 7.0 Hz, 4H, 2CH2N+), 7.29 (d, J = 8.4 Hz, 4H, 4CHAr), 7.42 (d, J = 8.4 Hz, 4H, 4CHAr), 7.59 (d, J = 6.9 Hz, 4H, 4CHpy), 8.92 (d, J = 6.9 Hz, 4H, 4CHpy) ppm; 13C NMR (75 MHz, DMSO-d6): δ 13.9 (2C), 22.0 (2C), 25.3 (2C), 28.3 (2C) 28.5 (2C), 28.7 (2C), 28.8 (2C), 30.5 (2C), 31.2 (2C), 59.1 (2C), 114.6 (4C), 120.8 (4C), 122.7 (4C), 146.7 (4C), 147.7 (2C), 154.9 (2C), 169.4 (2C) ppm; m/z (%): 356 (100), 279 (27), 268 (9), 155 (10), 141 (10), 85 (20), 71 (39), 57 (30), 43 (28), 29 (8); νmax, (KBr): 3442, 3032, 2922, 2853, 1641, 1489, 1297, 1242, 1183, 849 cm−1.
4,4′-[Oxybis(4,1-phenyleneoxy)]bis(1-undecylpyridinium)dibromide (27, 4OB4POB-11,Br). C44H62Br2N2O3; Mw 826.8; white solid (0.64 g, 78% yield); mp 87–94 °C; 1H NMR (300 MHz, DMSO-d6): δ 0.85 (t, J = 6.6 Hz, 6H, 2CH3), 1.17–1.35 (m, 32H, 16CH2), 1.81–1.93 (m, 4H, 2CH2), 4.50 (t, J = 7.0 Hz, 4H, 2CH2N+), 7.29 (d, J = 8.4 Hz, 4H, 4CHAr), 7.42 (d, J = 8.4 Hz, 4H, 4CHAr), 7.61 (d, J = 6.9 Hz, 4H, 4CHpy), 8.96 (d, J = 6.9 Hz, 4H, 4CHpy) ppm; 13C NMR (75 MHz, DMSO-d6): δ 13.8 (2C), 22.0 (2C), 25.2 (2C), 28.3 (2C), 28.6 (6C), 28.8 (2C), 30.5 (2C), 31.2 (2C), 59.0 (2C), 114.6 (4C), 120.8 (4C), 122.7 (4C), 146.8 (4C), 147.7 (2C), 154.9 (2C), 169.3 (2C) ppm; m/z (%): 356 (100), 279 (21), 149 (10), 135 (34), 78 (9), 69 (10), 57 (17), 51 (13), 43 (25), 29 (9); νmax, (KBr): 3424, 3024, 2924, 2853, 1640, 1488, 1294, 1242, 1181, 849 cm−1.
Biology
Sample preparation. Samples were provided by the collaborator and stored frozen at −20 °C. Samples were prepared in DMSO and water to a final testing concentration of 32 μg mL−1 or 20 μM (unless otherwise indicated in the data sheet) and serially diluted 1
:
2 fold for 8 times. Each sample concentration was prepared in 384-well plates, non-binding surface plate (NBS; Corning 3640) for each bacterial/fungal strain, tissue-culture treated (TC-treated; Corning 3712/3764) black for mammalian cell types and polypropylene 384-well (PP; Corning 3657) for haemolysis assays, all in duplicate (n = 2), and keeping the final DMSO concentration to a maximum of 0.5%. All the sample preparation was done using liquid handling robots.
Antibacterial assay.
Procedure. All bacteria were cultured in Cation-Adjusted Mueller Hinton Broth (CAMHB) at 37 °C overnight. A sample of each culture was then diluted 40-fold in fresh broth and incubated at 37 °C for 1.5–3 h. The resultant mid-log phase cultures were diluted (CFU per mL measured by OD600), then added to each well of the compound containing plates, giving a cell density of 5 × 105 CFU per mL and a total volume of 50 μL. All the plates were covered and incubated at 37 °C for 18 h without shaking.
Analysis. Inhibition of bacterial growth was determined measuring absorbance at 600 nm (OD600), using a Tecan M1000 Pro monochromator plate reader. The percentage of growth inhibition was calculated for each well, using the negative control (media only) and positive control (bacteria without inhibitors) on the same plate as references. The percentage of growth inhibition was calculated for each well, using the negative control (media only) and positive control (bacteria without inhibitors) on the same plate. The MIC was determined as the lowest concentration at which the growth was fully inhibited, defined by an inhibition ≥ 80%. In addition, the maximal percentage of growth inhibition is reported as DMax, indicating any compounds with partial activity. Hits were classified by MIC ≤ 16 μg mL−1 or MIC ≤ 10 μM in either replicate (n = 2 on different plates).
Antifungal assay.
Procedure. Fungi strains were cultured for 3 days on Yeast Extract-Peptone Dextrose (YPD) agar at 30 °C. A yeast suspension of 1 × 106 to 5 × 106 CFU per mL (as determined by OD530) was prepared from five colonies. The suspension was subsequently diluted and added to each well of the compound-containing plates giving a final cell density of fungi suspension of 2.5 × 103 CFU per mL and a total volume of 50 μL. All plates were covered and incubated at 35 °C for 36 h without shaking.
Analysis. Growth inhibition of C. albicans was determined measuring absorbance at 630 nm (OD630), while the growth inhibition of C. neoformans was determined measuring the difference in absorbance between 600 and 570 nm (OD600–570), after the addition of resazurin (0.001% final concentration) and incubation at 35 °C for 2 h. The absorbance was measured using a Biotek Multiflo Synergy HTX plate reader. In both cases, the percentage of growth inhibition was calculated for each well, using the negative control (media only) and positive control (fungi without inhibitors) on the same plate. The MIC was determined as the lowest concentration at which the growth was fully inhibited, defined by an inhibition ≥ 80% for C. albicans and an inhibition ≥ 70% for C. neoformans. Due to a higher variance in growth and inhibition, a lower threshold was applied to the data for C. neoformans. In addition, the maximal percentage of growth inhibition is reported as DMax, indicating any compounds with marginal activity. Hits were classified by MIC ≤ 16 μg mL−1 or MIC ≤ 10 μM in either replicate (n = 2 on different plates).
Cytotoxicity assay.
Procedure. HEK293 cells were counted manually in a Neubauer haemocytometer and then plated in the 384-well plates containing the compounds to give a density of 5000 cells per well in a final volume of 50 μL. DMEM supplemented with 10% FBS was used as growth media and the cells were incubated together with the compounds for 20 h at 37 °C in 5% CO2.
Analysis. Cytotoxicity (or cell viability) was measured by fluorescence, ex: 560/10 nm, em: 590/10 nm (F560/590), after addition of 5 μL of 25 μg mL−1 resazurin (2.3 μg mL−1 final concentration) and after incubation for further 3 h at 37 °C in 5% CO2. The fluorescence intensity was measured using a Tecan M1000 Pro monochromator plate reader, using automatic gain calculation. CC50 (concentration at 50% cytotoxicity) were calculated by curve fitting the inhibition values vs. log(concentration) using a sigmoidal dose–response function, with variable fitting values for bottom, top and slope. In addition, the maximal percentage of cytotoxicity is reported as DMax, indicating any compounds with partial cytotoxicity. The curve fitting was implemented using Pipeline Pilot's dose–response component, resulting in similar values to curve fitting tools such as GraphPad's Prism and IDBS's XlFit. Any value with > indicate sample with no activity (low DMax value) or samples with CC50 values above the maximum tested concentration (higher DMax value). Cytotoxic samples were classified by CC50 ≤ 32 μg mL−1 or CC50 ≤ 10 μM in either replicate (n = 2 on different plates). In addition, samples were flagged as partial cytotoxic if DMax ≥ 50%, even with CC50 > the maximum tested concentration.
Haemolysis assay.
Procedure. Human whole blood was washed three times with 3 volumes of 0.9% NaCl and then resuspended in same to a concentration of 0.5 × 108 cells per mL, as determined by manual cell count in a Neubauer haemocytometer. The washed cells were then added to the 384-well compound-containing plates for a final volume of 50 μL. After a 10 min shake on a plate shaker the plates were then incubated for 1 h at 37 °C. After incubation, the plates were centrifuged at 1000g for 10 min to pellet cells and debris, 25 μL of the supernatant was then transferred to a polystyrene 384-well assay plate.
Analysis. Haemolysis was determined by measuring the supernatant absorbance at 405 mm (OD405). The absorbance was measured using a Tecan M1000 Pro monochromator plate reader. HC10 and HC50 (concentration at 10% and 50% haemolysis, respectively) were calculated by curve fitting the inhibition values vs. log(concentration) using a sigmoidal dose–response function with variable fitting values for top, bottom and slope. In addition, the maximal percentage of haemolysis is reported as DMax, indicating any compounds with partial haemolysis. The curve fitting was implemented using Pipeline Pilot's dose–response component, resulting in similar values to curve fitting tools such as GraphPad's Prism and IDBS's XlFit. Any value with > indicate sample with no activity (low DMax value) or samples with HC10 values above the maximum tested concentration (higher DMax value). Haemolysis samples were classified by HC10 ≤ 32 μg mL−1 or HC10 ≤ 10 μM in either replicate (n = 2 on different plates). In addition, samples were flagged as partial haemolytic if DMax ≥ 50%, even with HC10 > the maximum tested concentration.
Conflicts of interest
There are no conflicts to declare.
Acknowledgements
This work was supported by the Russian Science Foundation (RSF grant 17-73-20260). The antimicrobial screening performed by CO-ADD (The Community for Antimicrobial Drug Discovery) was funded by the Wellcome Trust (UK) and the University of Queensland (Australia).
Notes and references
-
(a) S. L. Moore and D. N. Payne, Types of Antimicrobial Agents, in Russell, Hugo & Ayliffe's Principles and Practice of Disinfection, Preservation & Sterilization, A. P. Fraise, P. A. Lambert and J-Y. Maillard, Blackwell Publishing Ltd, 4th edn, 2004, ch. 2 Search PubMed;
(b) S. Gormann and E. Scott, Chemical disinfectants, antiseptics and preservatives, in Hugo and Russell's Pharmaceutical Microbiology, ed. S. P. Denyer, N. A. Hodges and S. P. Gorman, Blackwell Science Ltd, 7th edn, 2004, ch. 17 Search PubMed.
-
(a) W. A. Jacobs, M. Heidelberger and H. L. Amoss, J. Exp. Med., 1916, 23, 569 CrossRef CAS;
(b) W. A. Jacobs, M. Heidelberger and C. G. Bull, J. Exp. Med., 1916, 23, 577 CrossRef CAS.
-
(a) G. Domagk, Dtsch. Med. Wochenschr., 1935, 61, 829 CrossRef CAS;
(b) G. A. Knight and B. D. Shaw, J. Chem. Soc., 1938, 682 RSC.
- For books and reviews see:
(a) Gemini Surfactants: Synthesis, Interfacial and Solution-Phase Behavior, and Applications, ed. R. Zana and J. Xia, Dekker, New York, 2004 Search PubMed;
(b) Gemini Surfactants, in Surfactants and Interfacial Phenomena, ed. M. J. Rosen, John Wiley & Sons, Inc., 3rd edn, 2004, ch. 12 Search PubMed;
(c) F. M. Menger and J. S. Keiper, Angew. Chem., Int. Ed., 2000, 39, 1907 CrossRef CAS;
(d) S. K. Hait and S. P. Moulik, Curr. Sci., 2002, 89, 1101 Search PubMed;
(e) M. C. Morán, A. Pinazo, L. Pérez, P. Clapés, M. Angelet, M. T. García, M. P. Vinardell and M. R. Infante, Green Chem., 2004, 6, 233 RSC;
(f) M. Tischer, G. Pradel, K. Ohlsen and U. Holzgrabe, ChemMedChem, 2012, 7, 22 CrossRef CAS;
(g) L. Pérez, A. Pinazo, R. Pons and M. R. Infante, Adv. Colloid Interface Sci., 2014, 205, 134 CrossRef;
(h) S. Singh, A. Bhadani and M. Abe, Acc. Mater. Surf. Res., 2016, 1, 19 Search PubMed;
(i) D. Utreja, S. Sharma, A. Goyal, K. Kaur and S. Kaushal, Curr. Org. Chem., 2019, 23, 2271 CrossRef CAS.
-
(a) F. Devinsky, L. Masarova and I. Lacko, J. Colloid Interface Sci., 1985, 105, 235 CrossRef CAS;
(b) R. Zana, M. Benrraoa and R. Rueff, Langmuir, 1991, 7, 1072 CrossRef CAS;
(c) F. M. Menger and C. A. Littau, J. Am. Chem. Soc., 1993, 115, 10083 CrossRef CAS;
(d) M. Diz, A. Manresa, A. Pinazo, P. Erra and M. R. lnfante, J. Chem. Soc., Perkin Trans. 2, 1994, 1871 RSC;
(e) M. Pavlikova-Moricka, I. Lacko, F. Devinsky, L. Masarova and D. Mlynarcik, Folia Microbiol., 1994, 39, 176 CrossRef CAS;
(f) M. Tischer, L. Sologub, G. Pradel and U. Holzgrabe, Bioorg. Med. Chem., 2010, 18, 2998 CrossRef CAS;
(g) S. G. Silva, R. F. Fernandes, E. F. Marques and M. L. C. do Vale, Eur. J. Org. Chem., 2012, 345 CrossRef;
(h) A. K. Ghumare, B. V. Pawar and S. S. Bhagwat, J. Surfactants Deterg., 2013, 16, 85 CrossRef CAS;
(i) E. Obłak, A. Piecuch, K. Guz-Regner and E. Dworniczek, FEMS Microbiol. Lett., 2014, 350, 190 CrossRef;
(j) J. Li, P. Yang, Z. Sha, T. Li and Y. Liu, J. Surfactants Deterg., 2015, 18, 155 CrossRef CAS;
(k) S. Q. Fu, J. W. Guo, X. Zhong, Z. Yang and X. F. Lai, RSC Adv., 2016, 6, 16507 RSC;
(l) M. Huang, J. Ma, X. Wu, M. Zhao, L. Wang, F. Che and H. Qian, J. Surfactants Deterg., 2019, 22, 1289 CrossRef CAS.
-
(a) D. M. Bailey, C. G. DeGrazia, S. J. Hoff, P. L. Schulenberg, J. R. O'Connor, D. A. Paris and A. M. Slee, J. Med. Chem., 1984, 27, 1457 CrossRef CAS;
(b) K. Ohkura, A. Sukeno, H. Nagamune and H. Kourai, Bioorg. Med. Chem., 2005, 13, 2579 CrossRef CAS;
(c) H. Kourai, T. Yabuhara, A. Shirai, T. Maeda and H. Nagamune, Eur. J. Med. Chem., 2006, 41, 437 CrossRef CAS;
(d) M. Yoshikawa, K. Motoshima, K. Fujimoto, A. Tai, H. Kakuta and K. Sasaki, Bioorg. Med. Chem., 2008, 16, 6027 CrossRef CAS.
-
(a) A. N. Vereshchagin, Russ. Chem. Bull., Int. Ed., 2017, 66, 1765 CrossRef CAS;
(b) A. N. Vereshchagin, M. N. Elinson, Y. E. Anisina, F. V. Ryzhkov, R. A. Novikov and M. P. Egorov, ChemistrySelect, 2017, 2, 4593 CrossRef CAS.
-
(a) K. Ohkura, A. Sukeno, K. Yamamoto, H. Nagamune, T. Maeda and H. Kourai, Bioorg. Med. Chem., 2003, 11, 5035 CrossRef CAS;
(b) K. Fujimoto, D. Morisaki, M. Yoshida, T. Namba, K. Hye-Sook, Y. Wataya, H. Kourai, H. Kakuta and K. Sasaki, Bioorg. Med. Chem. Lett., 2006, 16, 2758 CrossRef CAS;
(c) C. K. L. Ng, V. Singhal, F. Widmer, L. C. Wright, T. C. Sorrell and K. A. Jolliffe, Bioorg. Med. Chem., 2007, 15, 3422 CrossRef CAS;
(d) X. Z. Sun, N. Wang, D. Cao, Z. Y. Hu, P. Mao, H. B. Zhang and X. H. Zhou, Chin. Chem. Lett., 2011, 22, 887 CrossRef CAS;
(e) M. Yamamoto, T. Takami, R. Matsumura, A. Dorofeev, Y. Hirata and H. Nagamune, Biocontrol Sci., 2016, 21, 231 CrossRef CAS;
(f) D. Obando, Y. Koda, N. Pantarat, S. Lev, X. Zuo, J. B. Oei, F. Widmer, J. T. Djordjevic, T. C. Sorell and K. A. Jolliffe, ChemMedChem, 2018, 13, 1421 CrossRef CAS.
- M. Yamamoto, R. Matsumura, Y. Hirata and H. Nagamune, Toxicol. In Vitro, 2019, 54, 75 CrossRef CAS.
-
(a) A. N. Vereshchagin, A. M. Gordeeva, N. A. Frolov, P. I. Proshin, K. A. Hansford and M. P. Egorov, Eur. J. Org. Chem., 2019, 4123 CrossRef CAS;
(b) A. N. Vereshchagin, N. A. Frolov, V. Y. Konyuhova, K. A. Hansford and M. P. Egorov, Mendeleev Commun., 2019, 29, 523 CrossRef CAS;
(c) A. N. Vereshchagin, N. A. Frolov, V. Y. Konyuhova, E. O. Dorofeeva, K. A. Hansford and M. P. Egorov, Mendeleev Commun., 2020, 30, 424 CrossRef CAS;
(d) A. N. Vereshchagin, N. A. Frolov, A. S. Pakina, K. A. Hansford and M. P. Egorov, Mendeleev Commun., 2020, 30, 703 CrossRef CAS;
(e) A. N. Vereshchagin, K. A. Karpenko and M. P. Egorov, Russ. Chem. Bull., Int. Ed., 2020, 69, 620 CrossRef CAS.
- F. Fan, J. Tang, M. Luo and X. Zeng, J. Org. Chem., 2018, 83, 13559 Search PubMed.
- L. Shaobo, CN 103724169, 2014.
-
(a) J. Zamocka, I. Lacko and F. Devinsky, Pharmazie, 1994, 49, 66 CAS;
(b) K. P. C. Minbiole, M. C. Jennings, L. E. Ator, J. W. Black, M. C. Grenier, J. E. LaDow, K. L. Caran, K. Seifert and W. M. Wuest, Tetrahedron, 2016, 72, 3559 CrossRef CAS.
-
(a) M. A. T. Blaskovich, J. Zuegg, A. G. Elliott and M. A. Cooper, ACS Infect. Dis., 2015, 1, 285 CrossRef CAS;
(b) K. A. Hansford, M. A. T. Blaskovich and M. A. Cooper, Future Med. Chem., 2016, 8, 925 CrossRef CAS.
Footnote |
† Electronic supplementary information (ESI) available. See DOI: 10.1039/d0ra08900a |
|
This journal is © The Royal Society of Chemistry 2021 |
Click here to see how this site uses Cookies. View our privacy policy here.