DOI:
10.1039/D0RA08508A
(Paper)
RSC Adv., 2021,
11, 168-176
A novel donor–π–acceptor halochromic 2,6-distyrylnaphthalene chromophore: synthesis, photophysical properties and DFT studies†
Received
6th October 2020
, Accepted 9th December 2020
First published on 22nd December 2020
Abstract
In this study a new 2,6-distyryl naphthalene [2-((4-((E)-2-(6-((E)-2,4-bis(methylsulfonyl)styryl)naphthalen-2-yl)vinyl)phenyl)(ethyl)amino)ethan-1-ol; ASDSN] was synthesized successfully using Heck chemistry as the main reaction. The ASDSN compound is a donor–pi–acceptor (D–π–A) conjugated system with amino as electron donating and sulfonyl as electron withdrawing groups. The UV-vis absorption of ASDSN was observed in the range of 403–417 nm with high molar extinction coefficients (ε = 15
300–56
200 M−1 cm−1) in some different solvents. This new fluorescent 2,6-distyryl naphthalene compound emits in the yellow region of the visible spectrum (557 nm) with Stokes shifts of 5930 cm−1. ASDSN is a pH-responsive fluorescence compound that shows yellow fluorescence in neutral form and blue fluorescence in the protonated form. A white light emission (WLE) for the chromophore was observed at pH = 3.0. The ASDSN chromophore presented a satisfactory white light quantum yield (Φ) of 13% which was desirable for producing white light emitting devices. Density functional theory (DFT) and time-dependent (TD)-DFT were applied to study structural and electronic properties of the chromophore.
Introduction
The synthesis of fluorophores incorporating distyrylbenzene π-conjugated systems has attracted considerable attention because of their high potential applications in optoelectronic and sensory devices as well as fluorescent probes in medical utilization and in organic light-emitting diodes.1–6 On the other hand, organic molecules that possess intramolecular charge transfer (ICT) character are important in a large number of areas, such as organic solar cells, polarity probes and nonlinear optics.7,8
Donor–π–acceptor (D–π–A; where D is an electron-donating group, A is an electron-accepting group, and π is a conjugating moiety) systems with different configurations have been extensively developed because their absorption spectra and energy gaps can be readily tuned by controlling the ICT character using different electron-donor and electron-acceptor groups. This belonging plays an important role in the improvement of the photophysical properties of photonic devices.9–11
Distyrylbenzenes are a well-known type of fluorescent dyes that possess high photochemical and thermal stability.12,13 To synthesize these conjugated spacers, two important organic transformations, namely Wittig and Heck reactions, are used. Depends on the availability of starting materials and the strategy used to prepare the stilbene and distyrylbenzene molecules, one of the aforementioned reactions is preferred to be used. While sometimes, a combination of both reactions is required to obtain desired stilbene and distyrylbenzene compounds. Typically, feeding compounds participating in the above mentioned reactions are already modified with electron donating and electron withdrawing substitutes.
The emission of distyrylbenzenes highly depends on the nature of electron donating and the electron-acceptor groups. With increasing the strength of the donor and/or the acceptor groups, frequently, a bathochromic shift is observable. So that, by use of suitable electron-donating and the electron-acceptor groups, the ICT band can even appear in the range of near and far-infrared.14,15 Sulfone (–SO2R), is one of the most interesting electron-acceptor groups that features remarkable mobility of charge carriers which is an important photophysical property in π-conjugated systems. In addition, hydrophobic rigid backbone of sulfone group with excellent charge generation property can facilitate the electron migration in the structure of molecule, which is another essential character in photonics.16 Amine functional groups are considered as compelling candidates to be used as efficient electron-donating moieties. The electron pair of amino groups can act as a ligand or base to coordinate with metals and abstract protons, respectively. Consequently, the amino-functionalized stilbene-based fluorescent materials are examined as potential sensors for detecting metal ions.17–19 Moreover, substantial shifts in the absorption and emission of amino-functionalized stilbenes are observed upon protonation, representing pH-sensory capability of these materials.20 In other words, they are halochromic chromophores with tunable fluorescence through regulation of pH. More interestingly, they can emit white fluorescence at specific pH, because the emission of the neutral and protonated forms of amino-functionalized stilbenes is different due to the presence of different molecule forms simultaneously. Therefore, the emission spectra cover the entire visible region ranging from 400 nm to 700 nm leading to white light emission (WLE).21–28 This exceptional feature enables white light emitter chromophores to be utilized in single-component white organic light-emitting diodes (WOLEDs).29
In this study, in continuation of our previous reports13,30–33 we synthesized a new D–π–A 2,6-distyryl naphthalene molecule containing an amino group as electron-donating and sulfonyl group as electron-withdrawing group in the terminals of the π-conjugated system. Two sulfonyl groups were used in ortho and para positions of the benzene ring in the acceptor terminal of the molecule in order to have a strong push–pull effect. We showed that the ASDSN chromophore experience a hypsochromic shift from yellow to blue with the variation of pH and emit white light at pH = 3. The chromophore presents a remarkable quantum yield (Φ) with the maximum value of 13 and 49% in its protonated and neutral state, respectively.
Results and discussion
The Heck reaction using a palladium-catalyzed process was employed as a main organic transformation for the synthesis of the ASDSN chromophore (Scheme 1). The coupling partners in the Heck reaction are aryl halides and alkenes.13 Therefore, aryl halides A and B and also alkenes C and D were synthesized using available starting materials using organic transformations as shown in Scheme 1. To prepare the alkene intermediate D a controlled Heck reaction between aryl bromide A and alkene C was accomplished. Finally, the coupling reaction of alkene D and aryl iodide B afforded compound ASDSN with 87% isolated yield as a red solid.
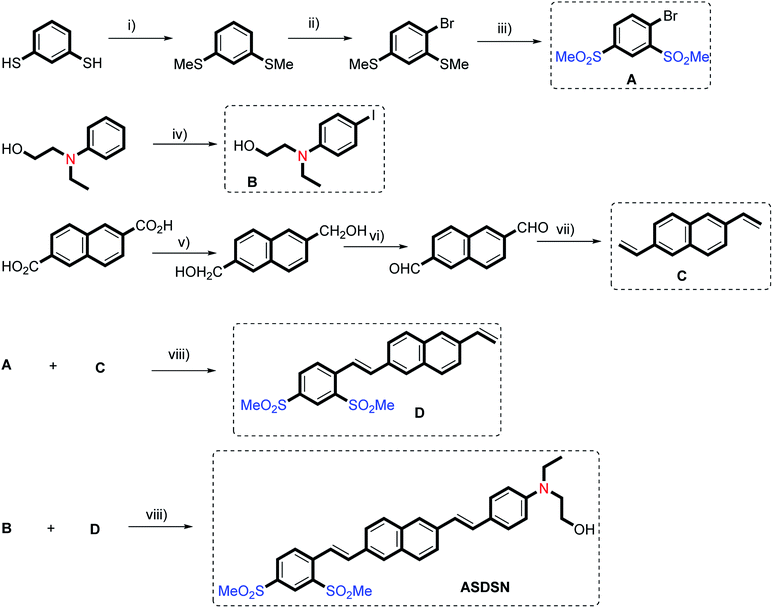 |
| Scheme 1 Synthetic routes to prepare compound ASDSN. (i) K2CO3, Me–I, acetone, 50 °C, 6 h. (ii) Bu4NBr (5 mol%), HNO3 (15% w/w), dichloroethane, 20 °C. (iii) AcOH, H2O2, CH2Cl2, 0 °C-reflux, 2 h. (iv) I2, dioxane/pyridine, 0 °C to rt, 3 h. (v) LiAlH4. (vi) PCC. (vii) Ph3PMeBr, KOtBu, THF, rt, 18 h. (viii) Pd(OAc)2 (1.2–1.8 mol%), bis[(2-diphenylphosphino)phenyl] ether (DPEPhos) (2.5–3.6 mol%), K2CO3, DMF, 120 °C, 6–12 h. | |
After synthesis and characterization of the ASDSN molecule, its photophysical properties were investigated. The absorption and emission spectra of the chromophore in different solvents from low to high polarity were obtained to study the effect of solvent polarity on the photophysical behavior of the chromophore. The obtained results are shown in Fig. 1 and Table 1.
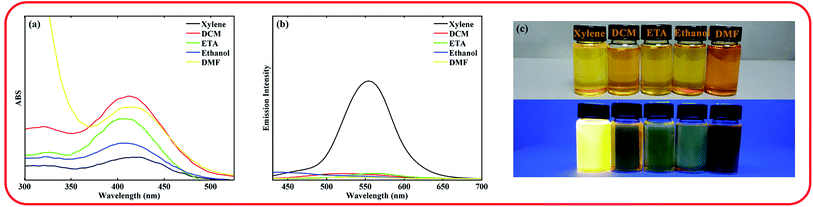 |
| Fig. 1 UV-vis spectra (a) and emission spectra (b) of ASDSN chromophore at concentration of 10−5 M in different solvents. The photographs of the chromophore in different solutions [from left to right, xylene, dichloromethane (DCM), ethyl acetate (ETA), ethanol, and dimethyl formamide (DMF)] were taken under natural daylight simulator (D65) lamps (top image), and irradiation of A-Class UV lamps (bottom image) (c). | |
Table 1 Optical data of the ASDSN chromophore
Solvent |
λab (nm) |
λem (nm) |
Stockes shift (cm−1) |
ε (L M−1 cm−1) |
Ea (eV) |
Φb |
Calculated from the absorption spectra by using the empirical formula of E (eV) = hc/λonset = 1240 (eV nm)/λmax (nm). 9,10-Diphenylanthracene was used as standard (Φ = 0.90 in cyclohexane). |
Xylene |
417 |
557 |
5930 |
15 300 |
2.61 |
0.49 |
ETA |
407 |
— |
— |
41 100 |
2.67 |
— |
DCM |
414 |
— |
— |
56 200 |
2.58 |
— |
Ethanol |
408 |
— |
— |
24 700 |
2.54 |
— |
DMF |
403 |
— |
— |
49 000 |
2.53 |
— |
The ASDSN chromophore showed a broad and structure-less absorption band around 400 nm attributed to the ICT transition arising from the push–pull effect of the donor (amine) and the acceptor groups (sulfonyl). The absorption band of ASDSN chromophore experienced a minor change with the variation of solvent polarity that is related to the low dipole moment of the ground state of ASDSN. ASDSN was found to be fluorescence only in xylene with an emission band of 557 nm as well as an excellent fluorescence quantum yield (Φ) of 0.49. However, its fluorescence was quenched in more polar solvents. The observed strong fluorescence quenching in more polar solvents may be attributed to the non-radiative relaxation process. In other words, polar solvents can stabilize the excited state of the chromophore, decrease the energy level between the ground and excited state, and promote radiation less decay.34–36
The absorption and emission spectra of the compound in xylene containing various amounts of trifluoroacetic acid (TFA) were recorded to investigate the effect of pH on its photophysical. The obtained results are shown in Fig. 2.
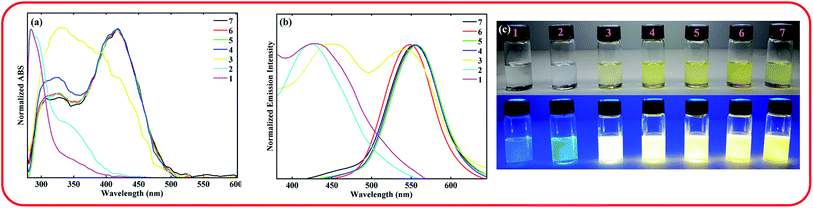 |
| Fig. 2 UV-vis spectra (a) and emission spectra (b) of the ASDSN chromophore in xylene at concentration of 10−5 M upon adjustment of pH from 1 to 7 by TFA. The photographs of the solutions (pH increases from left to right) were taken under natural daylight simulator (D65) lamps (top image), and irradiation of A-Class UV lamps (bottom image) (c). | |
As shown in Fig. 2, with the decrease of pH from 7 to 4, no significant shift was observed for both absorption and emission bands of the chromophore. Further decreasing of pH from 4 to 1 caused a significant blue shift in the absorption and emission bands of the ASDSN chromophore. The color of the solution containing the ASDSN chromophore was changed from yellow to colorless, and its fluorescence color was changed from yellow to blue. The blue shift values for the chromophore were found to be 121 nm (from 405 to 284 nm) and 132 nm (from 554 to 422 nm) for the absorption and emission bands, respectively, when pH was changed from 7 to 1. These results can be attributed to the protonation of the chromophore by the addition of TFA, which decreases the ICT character of the chromophore (Fig. 3a). In general, amine groups, as a strong electron donor in the push–pull fluorescent molecule, are protonated in the presence of acids and converted to an electron acceptor (quaternary ammonium salt; H-ASDSN). Consequently, the lack of electron-donating ability in the structure of the molecule interrupts the push–pull effect of the chromophores and decreases its ICT character.37–41 The most striking result to emerge from the data shown in Fig. 2 was the broad emission for the chromophore at pH = 3, which resulted in almost pure white fluorescence with the commission international de l'eclairage (CIE 1931) color coordinates of (0.33, 0.32). This observation indicated that at specific pH, neutral and protonated form of the chromophore whose emission bands are complementary with a broad distribution could simultaneously exist in the solution and cause in white light emission.42–45 Another surprising outcome that emerged from the data was a noticeable white light quantum yield (Φ) of 0.13. This finding represented the great potential of this chromophore for white lighting device fabrication.
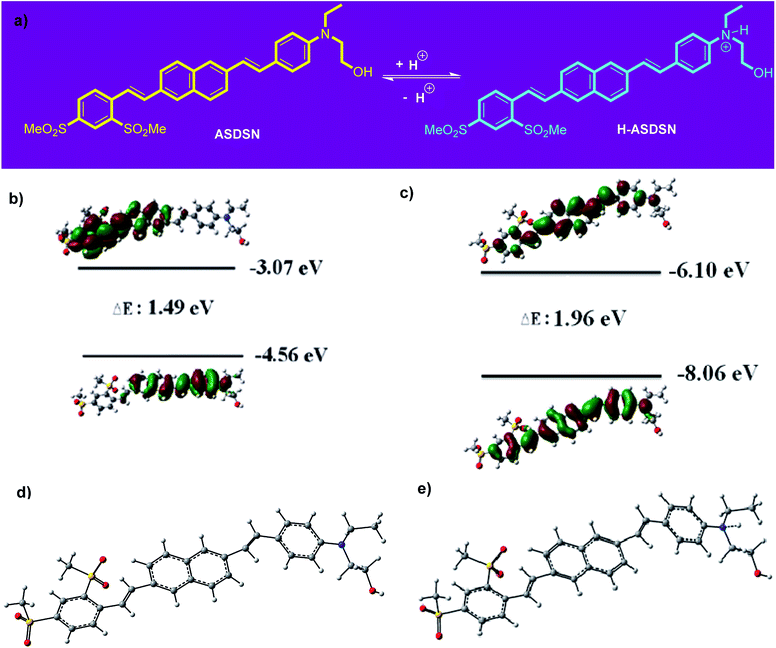 |
| Fig. 3 Protonation of ASDSN and formation of H-ASDSN form and their equilibrium in acidic environment (a). The HOMO–LUMO shapes of ASDSN and H-ASDSN and calculated energy gaps using DFT (b and c). Optimized structures of ASDSN (d) and H-ASDSN (e) using PBE/6-311++g (d, p) level of theory. | |
A DFT study was accomplished to have the frontier molecular orbitals of ASDSN and H-ASDSN [the highest occupied molecular orbital (HOMO) and lowest unoccupied molecular orbital (LUMO) orbitals] (Fig. 3b). The shape of orbitals shows that the HOMO and LUMO of ASDSN are both more centralized on donor (amino) and acceptor (sulfone) groups, respectively. While, H-ASDSN HOMO and LUMO orbitals are mostly centralized on π-conjugated system. The optimized geometry structure of both ASDSN and H-ASDSN are shown in Fig. 3d and e, respectively. It seems that the geometry of ASDSN molecule after protonation (in the form of H-ASDSN) not changed remarkably.
The interaction between ASDSN and solvents cause changing energy levels and re-distribution of electrons, excited state, dipole moment of the fluorophore, and substantial Stocks shift.42,43 These issues describe by Lippert–Mataga (LM) equation:
|
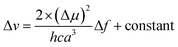 | (1) |
where Δ
ν, Δ
μ,
h,
c,
a and Δ
f are Stocks shift, difference of dipole moments, Planks constant, speed of light, radius of cavity, and solvent properties (below equation), respectively.
|
 | (2) |
This equation shows the re-orientation of solvent molecules with dielectric constant (ε) and refractive index (n). The used solvents as different environments mainly result in change of dipole moment of these π-conjugated molecules (Table 2).
Table 2 Calculated stocks shift (cm−1) and re-orientation of solvent molecules (Δf) in different solvents
Solvents |
νAa |
νEa |
Δν |
ε |
n |
Δf |
Wave number of absorption and emission . |
para-Xylene |
19 263 |
16 418 |
2845 |
2.2705 |
1.4958 |
0.0033 |
ETA |
19 415 |
16 356 |
3059 |
2.3832 |
1.4960 |
0.0138 |
DCM |
19 924 |
16 552 |
3372 |
8.9300 |
1.4244 |
0.2170 |
Ethanol |
20 741 |
17 016 |
3725 |
24.8520 |
1.3617 |
0.2890 |
DMF |
20 113 |
16 569 |
3544 |
37.2190 |
1.4305 |
0.2747 |
In order to have Lippert–Mataga plot, the Stocks shift vs. environmental polarity was plotted (Fig. 4). High R2 index (0.93) in the Lippert–Mataga plot mainly confirms enhancing of dipole moment of excited state with increasing solvent polarity.
 |
| Fig. 4 Lippert–Mataga plot for protic and aprotic solvents at selected level of theory. | |
Conclusion
In conclusion, a novel stilbene-based chromophore was synthesized through the Heck reaction. The compound showed a substantial hypsochromic shift from its neutral form with yellow fluorescence to its protonated form with blue emission. The chromophore displayed WLE at pH = 3 due to the simultaneous presence of its neutral and protonated forms representing pH-responsive fluorescence. Remarkable white light quantum yields of this fluorescent molecule either in its neutral or its protonated forms implied high potential application of this chromophore in the preparation of high-performance lighting devices. Theoretical study showed that the solvatochromic effect caused the changing of internal charge transfer (ICT) and dipole moment. Also, the protonation of luminophore mainly resulted in planarity and affected on the ASDSN properties.
Experimental section
General
Chemicals were purchased from Merck and Aldrich chemical companies. All the chemicals and solvents were used as received without further purification. For known products the spectral data were compared with those previously reported. For recording 1H NMR and 13C NMR spectra we used a Bruker (250 MHz) Avance DRX and samples were dissolved in pure deuterated CDCl3 with tetramethylsilane (TMS) as an internal standard. FT-IR spectroscopy (Shimadzu FT-IR 8300 spectrophotometer) was employed for characterization of the products. Melting points were determined in open capillary tubes in a Barnstead electro-thermal 9100 BZ circulating oil melting point apparatus. The reaction monitoring was accomplished by TLC on silica gel PolyGram SILG/UV254 plates. Column chromatography was carried out on columns of silica gel 60 (70–230 mesh).
1-Bromo-2,4-bis(methylsulfonyl)benzene (A)
In order to synthesis compound A, benzene-1,3-dithiol was used as available starting material. A 100 mL canonical flask was charged with benzene-1,3-dithiol (10 mmol, 1.42 g), K2CO3 (25 mmol, 3.45 g) and acetone (50 mL) at 0 °C. Then methyl iodide (22 mmol, 3.1 g) was added drop wise to the reaction mixture for 1 h. The reaction mixture was refluxed at 50 °C for 12 h. After completion of the reaction, the temperature was cool down to rt and the solvent was removed. The dense liquid was extracted with diethyl ether and the pure product was obtained by distillation (88%). 1H-NMR (250 MHz, DMSO-d6/TMS) δ (ppm): 2.41 (s, 6H), 7.52–7.96 (m, 3H). The spectral data was correspond to the previously reported method.46
For the bromination step, a 100 mL canonical flask was charged with KBr (20 mmol, 2.4 g), nitric acid (21% w/w, 40 mmol), dichloroethane (DCM, 20 mL), 1,3-bis(methylthio)benzene (10 mmol, 1.7 g), and tetrabutylammonium chloride (3 mol%, 0.9 g) and stirred at room temperature for 24 h. After the completion of the reaction, it was diluted with water (50 mL) and DCM (50 mL). The organic layer was separated from the aqueous layer and it was washed with saturated NaHCO3 and saturated NaCl. The organic layer was separated and dried over MgSO4. After concentration of organic layer under reduced pressure, the product was obtained as yellow oil (85%) which was used for next step without further purification.47
The obtained sulfide from previous step (2.0 g, 8 mmol) was dissolved in 15 mL of glacial acetic acid at 0 °C. Afterward, 30% hydrogen peroxide (6 mL) was added and it was refluxed for 2 h and then poured on crushed ice. Crud product was filtered, dried and purified by column chromatography using petroleum ether and ethyl acetate elunt to obtain pure product (A) as a white solid (2.0 g, 81%).46 1H-NMR (250 MHz, CDCl3/TMS) δ (ppm): 3.65 (s, 6H), 7.48–7.95 (m, 3H). 13C-NMR (62.5 MHz, CDCl3/TMS) δ (ppm): 47.9, 48.1, 126.8, 127.2, 135.1, 135.3, 140.8, 143.9. Anal. cal. C8H9BrO4S2 (313.18): C, 30.68; H, 2.90; S, 20.47; found: C, 30.56; H, 2.81 0; S, 20.41.
2-(Ethyl(4-iodophenyl)amino)ethan-1-ol (B)
This compound was synthesized as a white solid based on a known procedure in the literature.48 1H-NMR (250 MHz, CDCl3/TMS) δ (ppm): 1.16 (t, J = 7.0 Hz, 3H), 1.61 (brs, 1H), 3.35–3.43 (m, 4H), 3.75 (t, J = 5.5 Hz, 2H), 6.50 (d, J = 8.5 Hz, 2H), 7.42 (d, J = 8.5 Hz, 2H). 13C-NMR (62.5 MHz, CDCl3/TMS) δ (ppm): 11.7, 45.5, 52.4, 60.0, 114.9, 123.8, 137.7, 147.6. Anal. cal. C10H14INO (291.13): C, 41.26; H, 4.85; N, 4.81; found: C, 41.17; H, 4.78; N, 4.75.
2,6-Divinylnaphthalene (C)
This material was prepared in three steps using known procedures in the literature starting from naphthalene-2,6-dicarboxylic acid as commercially available chemical. First, naphthalene-2,6-dicarboxylic acid was converted to 2,6-bis(hydroxymethyl)naphthalene.49 A white solid was obtained which its spectral data completely matched to the previously reported one. 1H-NMR (250 MHz, CDCl3/TMS) δ (ppm): 4.11 (brs, 2H), 4.71 (d, J = 5.8 Hz, 2H) 7.47–7.50 (m, 2H), 7.79 (s, 2H), 7.81–7.84 (m, 2H). Anal. cal. C12H12O2 (188.23): C, 76.57; H, 6.43; found: C, 76.51; H, 6.34.
Next, 2,6-bis(hydroxymethyl)naphthalene was converted to naphthalene-2,6-dicarbaldehyde using an oxidation process by use of a known procedure.50 1H-NMR (250 MHz, CDCl3/TMS) δ (ppm): 8.03–8.08 (m, 2H), 8.09–8.13 (m, 2H), 8.41 (s, 2H), 10.19 (s, 2H). Anal. cal. C12H8O2 (184.19): C, 78.25; H, 4.38; found: C, 78.18; H, 4.31.
Finally, naphthalene-2,6-dicarbaldehyde was transferred to 2,6-divinylnaphthalene (C) using a known procedure.51 A reaction vessel was charged with naphthalene-2,6-dicarbaldehyde (0.92 g, 5 mmol), KOtBu (1.7 g, 15 mmol) and methyltriphenylphosphonium bromide (4.3 g, 12 mmol) and it was degassed and filled with argon. The reaction temperature kept at 0–5 °C and then dry THF (30 mL) was added and it was stirred for 1 h at this temperature. Afterward, the reaction mixture was allowed to stir at room temperature for another 6 h. After completion of the reaction it was concentrated, extracted (with ether and water) and dried over Na2SO4. The obtained organic layer was concentrated and purified by column chromatography (n-pentane) (72%, 0.65 g). 1H-NMR (250 MHz, CDCl3/TMS) δ (ppm): 5.25 (d, J = 10.9 Hz, 2H), 5.81 (d, J = 17.5 Hz, 2H), 6.81 (dd, J = 17.5, 10.6 Hz, 2H), 7.52–7.77 (m, 6H). Anal. cal. C14H12 (180.25): C, 93.29; H, 6.71; found: C, 93.21; H, 6.65.
2-1-2-[2-(2,4-Bis-methanesulfonyl-phenyl)-vinyl]-6-vinyl-naphthalene (D)
A sealed Schlenk tube was charged with 1-bromo-2,4-bis-methanesulfonyl-benzene (A; 1 mmol, 0.313 g), 2,6-divinyl-naphthalene (C; 1 mmol, 0.18 g), and K2CO3 (2.5 mmol, 0.345 g), Pd(OAc)2 (1.2 mol%, 2.7 mg), DPEPhos ligand (2.4 mol%, 13.0 mg) and it was evacuated and backfilled with argon. Then 5 mL of dry DMF was added to the reaction mixture under fellow of argon and tube was sealed with a screw-cap and the resulting mixture was heated in an oil bath at 120 °C for 6 h. The reaction was followed by TLC. After completion of the reaction, the mixture was cooled down to room temperature and filtered and the remaining solid was washed with dichloromethane (3 × 5 mL) in order to separate the catalyst. After the extraction of dichloromethane from water, the organic extract was dried over Na2SO4. The products were purified by column chromatography (hexane/ethyl acetate: 10/1) to obtain the pure product (0.31 g, 75%). Yellow solid; mp 213–215 °C. IR (KBr): 3749, 3441, 1851.5, 1419, 1304, 1165, 1134, 1103, 964, 818, 763, 509 cm−1. 1H-NMR (250 MHz, CDCl3/TMS) δ (ppm): 2.47 (s, 3H), 2.48 (s, 3H), 5.38 (d, J = 11.0 Hz, 1H), 6.01 (d, J = 17.5 Hz, 1H), 6.89 (dd, J = 17.6, 10.7 Hz, 1H), 7.68 (s, 1H), 7.75–7.85 (m, 3H), 7.92–8.01 (m, 4H), 8.08 (d, J = 3.2 Hz, 1H), 8.28–8.32 (m, 1H), 8.41 (d, J = 1.7 Hz, 1H). 13C-NMR (62.5 MHz, CDCl3/TMS) δ (ppm): 41.2, 42.0, 113.3, 128.5, 128.9, 129.0, 131.0, 131.6, 132.2, 132.3, 133.8, 134.0, 134.5, 135.6, 135.8, 139.2, 139.3. Anal. cal. C22H20O4S2 (412.5): C, 64.05; H, 4.89; O, 15.51; S, 15.55; found: C, 64.11; H, 4.93.
2-2-2-{[4-(2-{6-[2-(2,4-Bis-methanesulfonyl-phenyl)-vinyl]-naphthalen-2-yl}-vinyl)-phenyl]-ethyl-amino}-ethanol (ASDSN)
A sealed Schlenk tube was charged with compound D (0.5 mmol, 0.2 g), 2-[ethyl-(4-iodo-phenyl)-amino]-ethanol (B; 0.5 mmol, 0.14 g), and K2CO3 (2.0 mmol, 0.28 g), Pd(OAc)2 (1.2 mol%, 1.4 mg), DPEPhos ligand (2.4 mol%, 7.0 mg) and it was evacuated and backfilled with argon. Then 5 mL of dry DMF was added to the reaction mixture under fellow of argon and tube was sealed with a screw-cap and the resulting mixture was heated in an oil bath at 120 °C for 12 h. After completion of the reaction, the mixture was filtered (in hot form) and the remaining solid was washed with DMF (2 mL) in order to separate the catalyst. Afterward, water (10 mL) was added to the solution in order to precipitate product. The obtained solid was purified by column chromatography (hexane/ethyl acetate: 10/2) to obtain the pure product (0.25 g, 87%). Red solid; mp 289–291 °C. IR (KBr): 3441, 2924, 1589, 1520, 1304, 1165, 1142, 957, 802, 764, 656, 509 cm−1. 1H-NMR (250 MHz, DMSO-d6/TMS) δ (ppm): 1.10 (t, J = 4.5 Hz, 3H), 2.50 (t, J = 1.2 Hz, 2H), 1.10 (t, J = 4.5 Hz, 3H), 3.35 (s, 2H), 3.54–3.58 (m, 2H), 4.80 (t, J = 3.2 Hz, 1H), 6.70 (d, J = 5.5 Hz, 2H), 7.22 (dd, J = 49.5, 10.2 Hz, 2H), 7.46 (d, J = 5.5 Hz, 1H), 7.74 (d, J = 10.0 Hz, 1H), 7.82–7.87 (m, 2H), 7.92–7.95 (m, 3H), 8.03–8.08 (m, 2H), 8.23 (dd, J = 5.2, 1.2 Hz, 1H), 8.36 (d, J = 5.2 Hz, 1H), 8.43 (d, J = 1.2 Hz, 1H). 13C-NMR (62.5 MHz, CDCl3/TMS) δ (ppm): 12.0, 43.4, 43.8, 44.6, 52.0, 59.3, 111.3, 121.8, 122.6, 123.8, 123.9, 124.3, 127.9, 128.0, 128.3, 128.4, 128.6, 128.9, 130.0, 132.0, 133.8, 136.7, 136.9, 137.7, 139.3, 141.4, 147.6. Anal. cal. C32H33NO5S2 (575.7): C, 66.76; H, 5.78; N, 2.43; O, 13.89; S, 11.14; found: C, 66.74; H, 5.73; N, 2.47.
Conflicts of interest
The authors declare no competing financial interests.
Acknowledgements
Financial support from the research councils of Shiraz University and Amirkabir University of Technology are gratefully acknowledged.
References
- J. Shi, M. A. Izquierdo, S. Oh, S. Y. Park, B. Milián-Medina, D. Roca-Sanjuán and J. Gierschner, Inverted Energy Gap Law for the Nonradiative Decay in Fluorescent Floppy Molecules: Larger Fluorescence Quantum Yields for Smaller Energy Gaps, Org. Chem. Front., 2019, 6(12), 1948–1954, 10.1039/C9QO00259F.
- J. Gierschner, L. Lüer, B. Milián-Medina, D. Oelkrug and H.-J. Egelhaaf, Highly Emissive H-Aggregates or Aggregation-Induced Emission Quenching? The Photophysics of All-Trans Para-Distyrylbenzene, J. Phys. Chem. Lett., 2013, 4(16), 2686–2697, DOI:10.1021/jz400985t.
- H. Zhang, E. A. Kotlear, S. Kushida, S. Maier, F. Rominger, J. Freudenberg and U. H. F. Bunz, Linear and Star-Shaped Extended Di- and Tristyrylbenzenes: Synthesis, Characterization and Optical Response to Acid and Metal Ions, Chem.–Eur. J., 2020, 26(36), 8137–8143, DOI:10.1002/chem.202000893.
- F. S. Miri, S. Gorji Kandi and F. Panahi, Photophysical Properties of a Donor-π-Acceptor Distyrylbenzene Derivative in Solution and Solid State, J. Fluoresc., 2020, 30(4), 917–926, DOI:10.1007/s10895-020-02567-2.
- S. E. Estrada-Flórez, F. S. Moncada, A. E. Lanterna, C. A. Sierra and J. C. Scaiano, Spectroscopic and Time-Dependent DFT Study of the Photophysical Properties of Substituted 1,4-Distyrylbenzenes, J. Phys. Chem. A, 2019, 123(30), 6496–6505, DOI:10.1021/acs.jpca.9b04492.
- A. Granados and A. Vallribera, Fluorous Hydrophobic Fluorescent (E)-Stilbene Derivatives for Application on Security Paper, Dyes Pigm., 2019, 170, 107597, DOI:10.1016/j.dyepig.2019.107597.
- Aruna, B. Rani, S. Swami, A. Agarwala, D. Behera and R. Shrivastava, Recent Progress in Development of 2,3-Diaminomaleonitrile (DAMN) Based Chemosensors for Sensing of Ionic and Reactive Oxygen Species, RSC Adv., 2019, 9(52), 30599–30614, 10.1039/C9RA05298D.
- H. Y. Chung, J. Oh, J.-H. Park, I. Cho, W. S. Yoon, J. E. Kwon, D. Kim and S. Y. Park, Spectroscopic Studies on Intramolecular Charge-Transfer Characteristics in Small-Molecule Organic Solar Cell Donors: A Case Study on ADA and DAD Triad Donors, J. Phys. Chem. C, 2020, 124(34), 18502–18512, DOI:10.1021/acs.jpcc.0c06741.
- M. Zhu, Y. Xu, L. Sang, Z. Zhao, L. Wang, X. Wu, F. Fan, Y. Wang and H. Li, An ICT-Based Fluorescent Probe with a Large Stokes Shift for Measuring Hydrazine in Biological and Water Samples, Environ. Pollut., 2020, 256, 113427, DOI:10.1016/j.envpol.2019.113427.
- X. Sun, T. Liu, J. Sun and X. Wang, Synthesis and Application of Coumarin Fluorescence Probes, RSC Adv., 2020, 10(18), 10826–10847, 10.1039/C9RA10290F.
- D. N. Kanekar, S. Chacko and R. M. Kamble, Synthesis and Investigation of the Photophysical, Electrochemical and Theoretical Properties of Phenazine–Amine Based Cyan Blue-Red Fluorescent Materials for Organic Electronics, New J. Chem., 2020, 44(8), 3278–3293, 10.1039/C9NJ06109F.
- J. Du, N. Xie, X. Wang, L. Sun, Y. Zhao and F. Wu, Optical Limiting Effects of Cyano Substituted Distyrylbenzene Derivatives, Dyes Pigm., 2016, 134, 368–374, DOI:10.1016/j.dyepig.2016.07.035.
- A. Mahmoodi, F. Panahi, F. Eshghi and E. Kimiaei, A Novel Tetra-Stilbene-Based Fluorescent Compound: Synthesis, Characterization and Photophysical Properties Evaluation, J. Lumin., 2018, 199, 165–173, DOI:10.1016/j.jlumin.2018.03.033.
- S. Zhang, D. Chen, L. Yan, Y. Xie, X. Mu and J. Zhu, A Near-Infrared Fluorescence Probe for Hydrazine Based on Dicyanoisophorone, Microchem. J., 2020, 157, 105066, DOI:10.1016/j.microc.2020.105066.
- G. Lv, Y. Xu, J. Yang, W. Li, C. Li and A. Sun, Novel D–π-A Type near-Infrared Fluorescent Probes for the Detection of Aβ40 Aggregates, Analyst, 2020, 145(20), 6579–6585, 10.1039/D0AN01180K.
- M. Sachs, R. S. Sprick, D. Pearce, S. A. J. Hillman, A. Monti, A. A. Y. Guilbert, N. J. Brownbill, S. Dimitrov, X. Shi, F. Blanc, M. A. Zwijnenburg, J. Nelson, J. R. Durrant and A. I. Cooper, Understanding Structure-Activity Relationships in Linear Polymer Photocatalysts for Hydrogen Evolution, Nat. Commun., 2018, 9(1), 4968, DOI:10.1038/s41467-018-07420-6.
- C. Huang and C. Ding, Dicyanostilbene-Derived Two-Photon Fluorescence Probe for Lead Ions in Live Cells and Living Tissues, Anal. Chim. Acta, 2011, 699(2), 198–205, DOI:10.1016/j.aca.2011.05.015.
- C. Huang, A. Ren, C. Feng and N. Yang, Two-Photon Fluorescent Probe for Silver Ion Derived from Twin-Cyano-Stilbene with Large Two-Photon Absorption Cross Section, Sens. Actuators, B, 2010, 151(1), 236–242, DOI:10.1016/j.snb.2010.09.013.
- J.-S. Yang, Y.-H. Lin and C.-S. Yang, Palladium-Catalyzed Synthesis of Trans-4-(N,N-Bis(2-Pyridyl)Amino)Stilbene. A New Intrinsic Fluoroionophore for Transition Metal Ions, Org. Lett., 2002, 4(5), 777–780, DOI:10.1021/ol017260l.
- A. J. Zucchero, J. Tolosa, L. M. Tolbert and U. H. F. Bunz, Bis(4′-Dibutylaminostyryl)Benzene: Spectroscopic Behavior upon Protonation or Methylation, Chem.–Eur. J., 2009, 15(47), 13075–13081, DOI:10.1002/chem.200900608.
- J. Tydlitát, S. Achelle, J. Rodríguez-López, O. Pytela, T. Mikýsek, N. Cabon, F. Robin-le Guen, D. Miklík, Z. Růžičková and F. Bureš, Photophysical Properties of Acid-Responsive Triphenylamine Derivatives Bearing Pyridine Fragments: Towards White Light Emission, Dyes Pigm., 2017, 146, 467–478, DOI:10.1016/j.dyepig.2017.07.043.
- S. Samanta, U. Manna and G. Das, White-Light Emission from Simple AIE–ESIPT-Excimer Tripled Single Molecular System, New J. Chem., 2017, 41(3), 1064–1072, 10.1039/C6NJ03070J.
- M. Takahashi, Y. Enami, H. Ninagawa and M. Obata, A Novel Approach to White-Light Emission Using a Single Fluorescent Urea Derivative and Fluoride, New J. Chem., 2019, 43(8), 3265–3268, 10.1039/C8NJ05105D.
- M. Li, Y. Yuan and Y. Chen, Acid-Induced Multicolor Fluorescence of Pyridazine Derivative, ACS Appl. Mater. Interfaces, 2018, 10(1), 1237–1243, DOI:10.1021/acsami.7b16050.
- D. K. Maiti, R. Bhattacharjee, A. Datta and A. Banerjee, Modulation of Fluorescence Resonance Energy Transfer Efficiency for White Light Emission from a Series of Stilbene-Perylene Based Donor–Acceptor Pair, J. Phys. Chem. C, 2013, 117(44), 23178–23189, DOI:10.1021/jp409042p.
- Y. I. Park, O. Postupna, A. Zhugayevych, H. Shin, Y.-S. Park, B. Kim, H.-J. Yen, P. Cheruku, J. S. Martinez, J. W. Park, S. Tretiak and H.-L. Wang, A New PH Sensitive Fluorescent and White Light Emissive Material through Controlled Intermolecular Charge Transfer, Chem. Sci., 2014, 6(1), 789–797, 10.1039/C4SC01911C.
- S. Bhattacharya and S. K. Samanta, Unusual Salt-Induced Color Modulation through Aggregation-Induced Emission Switching of a Bis-Cationic Phenylenedivinylene-Based π Hydrogelator, Chem.–Eur. J., 2012, 18(52), 16632–16641, DOI:10.1002/chem.201201940.
- J. Chaudhary, V. Mittal, S. Mishra, A. Daiya, R. Chowdhury, I. R. Laskar and R. K. Roy, A New Aggregation Induced Emission Active Halochromic White Light Emissive Molecule: Combined Experimental and Theoretical Study, J. Phys. Chem. C, 2020, 124(28), 15406–15417, DOI:10.1021/acs.jpcc.0c01067.
- C. P. Sharma, N. M. Gupta, J. Singh, R. A. K. Yadav, D. K. Dubey, K. S. Rawat, A. K. Jha, J.-H. Jou and A. Goel, Synthesis of Solution-Processable Donor–Acceptor Pyranone Dyads for White Organic Light-Emitting Devices, J. Org. Chem., 2019, 84(12), 7674–7684, DOI:10.1021/acs.joc.9b00293.
- H. Karimi-Alavijeh, F. Panahi and A. Gharavi, Photo-Switching Effect in Stilbene Organic Field Effect Transistors, J. Appl. Phys., 2014, 115(9), 093706 CrossRef.
- M. T. Sharbati, F. Panahi and A. Gharavi, Near-Infrared Organic Light-Emitting Diodes Based on Donor-Pi-Acceptor Oligomers, IEEE Photonics Technol. Lett., 2010, 22(22), 1695–1697 CAS.
- M. T. Sharbati, F. Panahi, A.-R. Nekoei, F. Emami and K. Niknam, Blue to Red Electroluminescence Emission from Organic Light-Emitting Diodes Based on π-Conjugated Organic Semiconductor Materials, J. Photonics Energy, 2014, 4(1), 043599 CrossRef.
- F. S. Miri, S. G. Kandi and F. Panahi, Photophysical Properties of a Donor-π-Acceptor Distyrylbenzene Derivative in Solution and Solid State, J. Fluoresc., 2020, 1–10 Search PubMed.
- V. Maffeis, R. Brisse, V. Labet, B. Jousselme and T. Gustavsson, Femtosecond Fluorescence Upconversion Study of a Naphthalimide–Bithiophene–Triphenylamine Push–Pull Dye in Solution, J. Phys. Chem. A, 2018, 122(25), 5533–5544, DOI:10.1021/acs.jpca.8b05177.
- Y. Zhang, M. Jiang, G.-C. Han, K. Zhao, B. Z. Tang and K. S. Wong, Solvent Effect and Two-Photon Optical Properties of Triphenylamine-Based Donor–Acceptor Fluorophores, J. Phys. Chem. C, 2015, 119(49), 27630–27638, DOI:10.1021/acs.jpcc.5b06762.
- H. Song, Z. Kuang, X. Wang, Y. Guo, Q. Guo, H. Zhang and A. Xia, Solvent Polarity Dependent Excited State Dynamics of 2′-Hydroxychalcone Derivatives, J. Phys. Chem. C, 2018, 122(27), 15108–15117, DOI:10.1021/acs.jpcc.8b03133.
- K. Anandhan, M. Cerón, V. Perumal, P. Ceballos, P. Gordillo-Guerra, E. Pérez-Gutiérrez, A. E. Castillo, S. Thamotharan and M. J. Percino, Solvatochromism and PH Effect on the Emission of a Triphenylimidazole-Phenylacrylonitrile Derivative: Experimental and DFT Studies, RSC Adv., 2019, 9(21), 12085–12096, 10.1039/C9RA01275C.
- A. J. Zucchero, P. L. McGrier and U. H. F. Bunz, Cross-Conjugated Cruciform Fluorophores, Acc. Chem. Res., 2010, 43(3), 397–408, DOI:10.1021/ar900218d.
- C. Dou, L. Han, S. Zhao, H. Zhang and Y. Wang, Multi-Stimuli-Responsive Fluorescence Switching of a Donor–Acceptor π-Conjugated Compound, J. Phys. Chem. Lett., 2011, 2(6), 666–670, DOI:10.1021/jz200140c.
- J. Tolosa, K. M. Solntsev, L. M. Tolbert and U. H. F. Bunz, Unsymmetrical Cruciforms, J. Org. Chem., 2010, 75(3), 523–534, DOI:10.1021/jo901961a.
- U. Balijapalli, S. Manickam, M. D. Thiyagarajan and S. K. Iyer, Highly Emissive, Naked-Eye Solvatochromic Probe Based on Styryl Tetrahydrodibenzo[a,i]Phenanthridine for Acidochromic Applications, RSC Adv., 2016, 6(63), 58549–58560, 10.1039/C6RA09359K.
- K. Yamaguchi, T. Murai, J.-D. Guo, T. Sasamori and N. Tokitoh, Acid-Responsive Absorption and Emission of 5-N-Arylaminothiazoles: Emission of White Light from a Single Fluorescent Dye and a Lewis Acid, ChemistryOpen, 2016, 5(5), 434–438, DOI:10.1002/open.201600059.
- S. Achelle, J. Rodríguez-López, C. Katan and F. Robin-le Guen, Luminescence Behavior of Protonated Methoxy-Substituted Diazine Derivatives: Toward White Light Emission, J. Phys. Chem. C, 2016, 120(47), 26986–26995, DOI:10.1021/acs.jpcc.6b08401.
- Z. Ibrahim Mohamed Allaoui, E. le Gall, A. Fihey, R. Plaza-Pedroche, C. Katan, F. Robin-le Guen, J. Rodríguez-López and S. Achelle, Push–Pull (Iso)Quinoline Chromophores: Synthesis, Photophysical Properties, and Use for White-Light Emission, Chem.–Eur. J., 2020, 26(36), 8153–8161, DOI:10.1002/chem.202000817.
- Y. Yang, T. Wang, P. Yin, W. Yin, S. Zhang, Z. Lei, M. Yang, Y. Ma, W. Duan and H. Ma, A General Concept for White Light Emission Formation from Two Complementary Colored Luminescent Dyes, Mater. Chem. Front., 2019, 3(3), 505–512, 10.1039/C8QM00599K.
- M. V. R. Reddy, S. Reddy, P. V. R. Reddy, D. B. Reddy and N. S. Reddy, Synthesis and cyclopropanation of E,E and Z,Z-1,3-bis(styrylsulfonyl)benzene, Phosphorus, Sulfur Silicon Relat. Elem., 1989, 44, 123–127, DOI:10.1080/10426508908043714.
- A. V. Joshi, M. Baidossi, S. Mukhopadhyay and Y. Sasson, Org. Process Res. Dev., 2004, 8, 568–570, DOI:10.1021/op030055w.
- C. Monnereau, E. Blart, V. Montembault, L. Fontaine and F. Odobela, Synthesis of new crosslinkable co-polymers containing a push–pull zinc porphyrin for non-linear optical applications, Tetrahedron, 2005, 61, 10113–10121, DOI:10.1016/j.tet.2005.07.094.
- B. Łukasik, J. Milczarek, R. Pawlowsk, R. Żurawiński and A. Chworos, Facile Synthesis of Fluorescent Distyrylnaphthalene Derivatives for Bioapplications, New J. Chem., 2017, 41, 6977–6980, 10.1039/C7NJ00004A.
- C. García-Simón, A. Monferrer, M. Garcia-Borràs, I. Imaz, D. Maspoch, M. Costas and X. Ribas, Size-selective encapsulation of C60 and C60-derivatives within an adaptable naphthalene-based tetragonal prismatic supramolecular nanocapsule, Chem. Commun., 2019, 55, 798–801, 10.1039/C8CC07886F.
- G. Schroer, J. Deischter, T. Zensen, J. Kraus, A.-C. Pöppler, L. Qi, S. Scott and I. Delidovich, Structure-performance correlations of crosslinked boronic acid polymers as adsorbents for recovery of fructose from glucose–fructose mixtures, Green Chem., 2020, 22, 550–562, 10.1039/C9GC03151K.
Footnotes |
† Electronic supplementary information (ESI) available. See DOI: 10.1039/d0ra08508a |
‡ These authors contributed equally. |
|
This journal is © The Royal Society of Chemistry 2021 |