DOI:
10.1039/D0RA07954E
(Paper)
RSC Adv., 2021,
11, 517-524
Development of a multicolor upconversion fluorescence immunoassay for the simultaneous detection of thiamethoxam and dextran by magnetic separation†
Received
17th September 2020
, Accepted 1st December 2020
First published on 23rd December 2020
Abstract
The contents of both pesticide residues and dextran are important parameters for evaluating the quality of sugarcane. In this study, a multicolor upconversion fluorescence immunoassay for the simultaneous detection of thiamethoxam and dextran was established on the basis of magnetic separation. Antigens of thiamethoxam and dextran were coupled to magnetic nanoparticles as the separation elements. Monoclonal antibodies of thiamethoxam (6C7D12) and dextran (3C6F7) were conjugated with the upconversion nanoparticles of NaYF4:Yb,Er with an emission wavelength at 544 nm and NaYF4:Yb,Tm with an emission wavelength at 477 nm to prepare the signaling elements, respectively. Due to the difference in the emission wavelength, the signaling elements bound on the separation elements could be detected simultaneously after separation by an external magnetic field. After optimization, the half-maximal inhibitory concentration (IC50) values of the immunoassay for thiamethoxam and dextran were 0.46 and 49.33 ng mL−1, respectively. The assay showed no cross-reactivity with the analogs of thiamethoxam and dextran except for clothianidin (8.7%). The average recoveries of thiamethoxam and dextran in sugarcane juice were 82.9–93.3% and 87.5–97.2%, respectively. The results indicated that the immunoassay could meet the requirements for the simultaneous quantitative detection of thiamethoxam and dextran.
1 Introduction
Thiamethoxam is a kind of high-efficiency and broad-spectrum insecticide that has been widely used to control aphids, sugarcane moth borers and whiteflies in sugarcane planting. Due to its high toxicity to honeybees, thiamethoxam has been temporarily prohibited by the European Commission.1 In addition, thiamethoxam has been observed to pose a risk to other nontarget organisms, including humans. Jameel et al. found that thiamethoxam could cause different hazardous effects by interaction with DNA as well as alteration in oxidative stress markers and biological parameters.2 Importantly, the toxic effect is continued in the next generation and might be at the genetic level. Caron-Beaudoin et al. proved that thiamethoxam was an endocrine disruptor due to its effects on aromatase activity and hormone production.3 Dextran, a high molecular weight polysaccharide, is commonly found in sugarcane, sugarcane juice and sugar,4 and it is produced by dextransucrase that is secreted by infected microorganisms (Leuconostoc mesenteroide).5 Dextran can cause some negative effects on the sugar industry, such as sugar loss, viscosity increases, influencing crystallization, and false increases in rotation.6,7 Therefore, it is necessary to detect thiamethoxam and dextran in sugarcane.
At present, the detection of thiamethoxam mainly depends on instrument-based methods, such as high-performance liquid chromatography (HPLC), HPLC-tandem mass spectrometry and so on.8,9 The methods are sensitive and accurate, but they have some shortcomings, such as expensive testing instruments, complicated sample pretreatment, time-consuming and laborious detection, and difficulty meeting the requirements of the rapid detection of a large number of samples. For dextran, the alcohol haze method10 and Roberts' copper method11 have been widely used in the sugar industry, but their sensitivity and specificity are unsatisfactory.12 The enzyme-HPLC method13 and Optical Activity Ltd. Polarimetric Method (DASA)14 have great sensitivity and specificity, but the test cost is high, and the test cannot be used routinely in the sugar industry.
Immunoassays have the advantages of high sensitivity and specificity, simple and rapid operation, low cost and a large analysis capacity,15,16 and they have been used in the rapid screening and monitoring of a large number of targets, including thiamethoxam and dextran. Kim et al. prepared an anti-thiamethoxam polyclonal antibody (pAb) and developed an enzyme-linked immunosorbent assay (ELISA), with the half-maximal inhibitory concentration (IC50) of 9.0 ng mL−1.17 Kim et al. prepared a monoclonal antibody (mAb, IC50 of 0.2 ng mL−1 in ELISA) for thiamethoxam and developed an automated flow fluorescent immunoassay.18 Ye et al. reported a mAb-based ELISA (IC50 of 0.87 ng mL−1) and immunochromatographic strip for the detection of thiamethoxam.19 For dextran, Liang et al. reported an anti-dextran mAb and immunonephelometry for the quantitative detection of dextran.20 Wang et al. developed a mAb-based sandwich ELISA (limit of detection, LOD of 3.9 ng mL−1)21 and competitive ELISA (IC50 of 204.2 ng mL−1)22 for dextran. However, there is currently no method for the simultaneous detection of thiamethoxam and dextran. In addition, immunoassays need to be developed toward more simple, rapid, sensitive and accurate methods.
In recent years, lanthanide-doped upconversion nanoparticles (UCNPs) have attracted increasing attention from researchers because they are excited by near-infrared (NIR) light (typically 980 nm) and emit different wavelengths of visible and ultraviolet light.23,24 UCNPs have the advantages of large Stokes shifts, great chemical and physical stability, high resistance to photobleaching and low potential biological toxicity.25–29 Compared with that of conventional fluorescence materials, the excitation light of UCNPs (NIR light) generally cannot be absorbed by the sample matrix, which can improve the signal-to-noise ratio and detection sensitivity of immunoassays.30,31 In addition, the UCNPs doped with different lanthanides possess varied luminescence spectra, which are very suitable for the simultaneous detection of multiple analytes by immunoassays under the same excitation light source.
In this paper, anti-thiamethoxam and anti-dextran mAbs were prepared by cell fusion, and a multicolor upconversion fluorescence immunoassay (UCFIA) for the simultaneous detection of thiamethoxam and dextran was developed by using UCNPs as signaling elements and magnetic nanoparticles (MNPs) as the separation elements. The sensitivity, specificity and accuracy of the immunoassay were evaluated by the detection of standards, analogs and spiked samples.
2 Materials and methods
2.1 Materials
The standards of thiamethoxam, imidacloprid, acetamiprid, thiacloprid, nitenpyram and clothianidin were purchased from Dr Ehrenstorfer (Germany). Imidaclothiz (97.82%) was provided by Nantong Jiangshan Agrochemical and Chemicals Co., Ltd. (Jiangsu, China). Dextran 2000 and its analogs were supplied by Pharmacia Company (Shanghai, China). Bovine serum albumin (BSA), ovalbumin (OVA) and tetramethylbenzidine (TMB) were purchased from Sigma Chemical Co., Ltd. (Missouri, USA). The antigens of dextran (dextran 40–BSA and dextran 40–OVA) were obtained from Medical College, Xiamen University. Gelatin was purchased from Saiguo Biotechnology Co., Ltd. (Guangdong, China). Goat anti-mouse immunoglobulin–horse radish peroxidase (GAM–HRP) conjugate was purchased from Boster Biological Technology Co., Ltd. (California, USA). Polyoxyethylene sorbitan monolaurate (Tween-20) was purchased from Beijing Solarbio Science & Technology Co., Ltd. (Beijing, China). H2O2 was purchased from Guanghua Technology Co., Ltd. (Guangdong, China). The amine-functionalized UCNPs of NaYF4:Yb,Er and NaYF4:Yb,Tm,31,32 and amine-functionalized Fe3O4 MNPs33 were prepared as previously described and stored in the laboratory. A 25% glutaraldehyde solution was supplied by Sinopharm Chemical Reagent Co., Ltd. (Shanghai, China). All other chemicals used in this study were of analytical grade. BALB/c mice were obtained from the Center of Comparative Medicine of Yangzhou University (Jiangsu, China). All animals used in this study and animal experiments were approved by Department of Science and Technology of Jiangsu Province (license number: SYXK (SU) 2017-0007).
2.2 Preparation of thiamethoxam antigens
The hapten of thiamethoxam was synthesized according to previous articles (Fig. S1†).17,19 The product was collected and characterized by electrospray ionization mass spectrometry (ESI-MS) and nuclear magnetic resonance (NMR) spectroscopy: ESI-MS, m/z, 362.1 [M + H]+; 1H NMR (400 MHz, DMSO) δ: 7.79 (s, 1H), 5.16 (s, 2H), 5.11 (s, 2H), 4.88 (s, 2H), 3.48 (t, J = 6.9 Hz, 2H), 2.98 (s, 3H), 2.84 (t, J = 6.9 Hz, 2H). The hapten was conjugated with BSA and OVA by the active ester method to prepare the immunogen and coating antigens, and the procedure was performed as described previously.34 The conjugates were identified by UV-vis spectroscopy, and the hapten densities were estimated by the following formula: (εconjugate − εcarrier protein)/εhapten. The molar ratios (hapten/protein) of immunogen and coating antigen were 20
:
1 and 10
:
1, respectively.
2.3 Preparation of mAb
The BSA conjugates (thiamethoxam hapten–BSA and dextran 40–BSA) were used to immunize BALB/c female mice by an intraperitoneal injection. The immunization strategy and the cell fusion procedures were performed as described in a previous article.35 After fusion, the cells were distributed into 96-well culture plates, and the supernatants from the plates were analyzed by indirect competitive ELISA (ic-ELISA) when hybridomas had grown to nearly 30% of the well area. The wells showing inhibitions in the ic-ELISAs were subcloned by the limiting dilution method. Ascites were obtained by the intraperitoneal injection of BALB/c female mice and purified by a protein A column (GE Healthcare, USA). After dialysis for 3 days, the mAbs were stored at −20 °C.
2.4 ic-ELISA protocol
All incubations were carried out at 37 °C, and the plates were washed five times with 10 mM phosphate-buffered saline containing 0.05% Tween 20 (PBST, pH = 7.4) after incubation. The 96-well microtiter plates (Corning) were coated with coating antigen (thiamethoxam hapten–OVA or dextran 40–OVA) in carbonate–bicarbonate buffer (50 mM, pH = 9.6) for 2 h. The plates were then blocked by the incubation of 1% gelatin in PBS (200 μL per well) for 1.5 h. Twenty-five microliters of analyte (thiamethoxam or dextran 2000) and antibody in PBS were added to the blocked plate, followed by incubation for 1 h. The diluted (1/20
000 in PBS) GAM–HRP (50 μL per well) was added to the plate for the incubation of 1 h. The bound GAM–HRP was detected by the addition of 50 μL per well TMB solution (100 μL of 1% hydrogen peroxide and 400 μL of 0.6% TMB in dimethyl sulfoxide added to 25 mL of citrate acetate buffer, pH = 5.5). After incubation for 15 min, the reaction was stopped by adding 25 μL of 2 M H2SO4, and the absorbance at 450 nm was measured by a SpectraMax M5 microplate reader (Molecular Devices, USA).
2.5 Preparation of antibody-conjugated UCNPs and antigen-conjugated MNPs
The antibody-conjugated UCNPs were prepared by the classical glutaraldehyde cross-linking method.36 Ten milligrams of amine-functionalized NaYF4:Yb,Er (or NaYF4:Yb,Tm) UCNPs were dispersed into 5 mL of PBS by ultrasonication for 30 min. Then, 100 mg of sodium borohydride and 1.25 mL of 25% glutaraldehyde were added and shaken for 1 h at room temperature (RT). The UCNPs were separated by centrifugation for 5 min at 6000 × g. After washing 3 times with PBS (20 mL), the precipitate was resuspended in 5 mL of PBS, and 0.6 mg of anti-thiamethoxam antibody (or anti-dextran antibody) was added and subjected to a shaking reaction for 6 h at RT. The unbound antibody was removed by centrifugation for 5 min at 6000 × g, and 5 mL of PBS containing 1% BSA was added to block the nonspecific and unreacted sites of the UCNPs by shaking reaction for 6 h. After washing 3 times with PBS, the antibody-conjugated UCNPs were dispersed in 5 mL of PBS and stored at 4 °C. The antigens (thiamethoxam hapten–OVA and dextran 40–OVA) were conjugated with MNPs by a similar method except that the MNPs were separated by an external magnetic field.
2.6 Procedures of multicolor UCFIA
Due to the interaction of antigens and antibodies, the signaling elements and separation elements formed immunocomplexes. In the presence of thiamethoxam and dextran, these compounds competed with the separation elements to bind signaling elements, thus dissociating the immunocomplexes. Under the action of an external magnetic field, the amount of separated signaling elements is reduced, which leads to a decrease in the fluorescence intensity. In addition, the emission wavelengths of NaYF4:Yb,Er (544 nm) and NaYF4:Yb,Tm (477 nm) are different, and thus, thiamethoxam and dextran could be detected simultaneously. Briefly, 400 μL of thiamethoxam and dextran 2000 standard or sample solution were mixed with 80 μL of thiamethoxam hapten–OVA-MNPs and dextran 40–OVA-MNPs (1
:
1, v/v) in a tube by vortexing for 1 min. Then, 400 μL of anti-thiamethoxam mAb–NaYF4:Yb,Er and anti-dextran mAb–NaYF4:Yb,Tm (1
:
1, v/v) in PBS was added for incubation for 1 h with shaking. After separation with a magnetic separator, the MNPs were washed 3 times with PBS. Subsequently, 500 μL of PBS was added, and the bound signaling elements were detected by a fluorescence spectrophotometer with a 980 nm laser transmitter (Changchun Laser Optoelectronics Technology Co., Ltd., China) at wavelengths of 544 nm and 477 nm.
2.7 Optimization of the multicolor UCFIA
The experimental parameters of the immunoassay, including the dosage of MNPs conjugated with antigens, incubation time, concentration of NaCl, pH value and methanol content, were optimized. The immunoassay was used to detect the standards of thiamethoxam and dextran 2000 under volumes of antigen-conjugated MNPs from 40 μL to 160 μL, incubation times from 10 to 70 min, concentrations of NaCl from 0.05 mol L−1 to 0.4 mol L−1, pH values from 5 to 9, and methanol concentrations from 0% to 20%. The standard curves of thiamethoxam and dextran were established by plotting the changes in the fluorescence intensities at 544 nm and 477 nm, respectively, versus the logarithm of the analyte concentration. The changes in the fluorescence intensity were calculated by the following equation: ΔI = I0 − I (I0 and I represent the fluorescence intensity without and with analyte, respectively). The parameters that resulted in the immunoassay with the highest Fmax/IC50 were desirable.
2.8 Cross-reactivity (CR)
The specificity of the immunoassay was determined by the CR. The analogs of thiamethoxam and dextran were detected by the immunoassay. The CRs were calculated by the following formula: CR = (IC50 of analyte/IC50 of analog) × 100%.
2.9 Analysis of spiked samples
Thiamethoxam-free sugarcane (confirmed by ultraperformance liquid chromatography coupled to tandem mass spectrometry37) was collected from a market in Nanjing, China; the sugarcane was juiced, and the solid residue was removed by filtration. Ten grams of juice was spiked with thiamethoxam and dextran simultaneously at concentrations of 20 ng g−1 thiamethoxam and 20 μg g−1 dextran 2000, 100 ng g−1 thiamethoxam and 40 μg g−1 dextran 2000, and 400 ng g−1 thiamethoxam and 80 μg g−1 dextran 2000. After appropriate dilution, the concentrations of thiamethoxam and dextran 2000 in the spiked samples were simultaneously detected by the immunoassay, and the recoveries were calculated. Each spiked sample was analyzed in triplicate.
3 Results and discussion
3.1 Determination of the mAb subtype and sensitivity in ic-ELISA
After subcloning, one hybridoma cell line that stably secreted anti-thiamethoxam mAb was named 6C7D12. The subtype of the mAb was determined as IgG3 by an Isotyping Kit for Mouse Monoclonal Antibody (Sino Biological Inc., China). The IC50, LOD (IC10) and linear range (IC10 to IC90) of the ic-ELISA developed by the mAb were 0.36 ng mL−1, 0.02 ng mL−1 and 0.02–5.88 ng mL−1, respectively. The sensitivity of the presented mAb for thiamethoxam was better than that of the pAb prepared by Kim et al. (IC50 of 9.0 ng mL−1)17 and the mAb prepared by Ye et al. (IC50 of 0.87 ng mL−1)19 and slightly lower than that of the mAb prepared by Kim et al. (IC50 of 0.2 ng mL−1).18
For dextran, three hybridoma cell lines that stably secreted anti-dextran mAba were obtained and named 3C6F7, 3E5D2 and 4B9A5. The subtype of 3C6F7 and 3E5D2 mAbs was IgG1, and that of 4B9A5 mAb was IgG2a. Among them, 3C6F7 had the highest titer and the best sensitivity, and the IC50, LOD and linear range in the ic-ELISA were 120.1 ng mL−1, 12.8 ng mL−1 and 12.8–1217.3 ng mL−1, respectively. The sensitivity of the presented mAb was better than that of the mAb prepared by Wang et al. (IC50 of 204.2 ng mL−1 in ic-ELISA).21
3.2 Specificity of the mAbs in ic-ELISA
The analogs of thiamethoxam, including imidaclothiz, imidacloprid, acetamiprid, thiacloprid, nitenpyram and clothianidin, were used to evaluate the specificity of the ic-ELISA developed by the anti-thiamethoxam mAb (6C7D12). The ic-ELISA showed CRs of 11.3% and 1.1% for clothianidin and imidacloprid, respectively. The other analogs of thiamethoxam exhibited no CR (less than 0.1%) by the ic-ELISA determination. For dextran, the ic-ELISA developed by the anti-dextran mAb (3C6F7) showed no CR (less than 0.1%) with sucrose, glucose, starch and β-glucan. These results indicated that the anti-thiamethoxam and anti-dextran mAbs showed high specificity in the ic-ELISAs.
3.3 Optimization of the multicolor UCFIA
As shown in Fig. 1, under the excitation wavelength of 980 nm, the signaling element of thiamethoxam (NaYF4:Yb,Er UCNPs conjugated with anti-thiamethoxam mAb) had four obvious emission peaks at 409, 526, 544 and 657 nm, among which the fluorescence intensity at 544 nm was the highest. For the signaling element of dextran (NaYF4:Yb,Tm UCNPs conjugated with anti-dextran mAb), there were three obvious emission peaks at 362, 452 and 477 nm, among which 477 nm had the highest fluorescence intensity. When the two signaling elements were mixed equally, the emission spectra did not overlap at 544 nm and 477 nm. Therefore, the fluorescence intensities at 544 nm and 477 nm were selected for the simultaneous detection of thiamethoxam and dextran in the multicolor UCFIA.
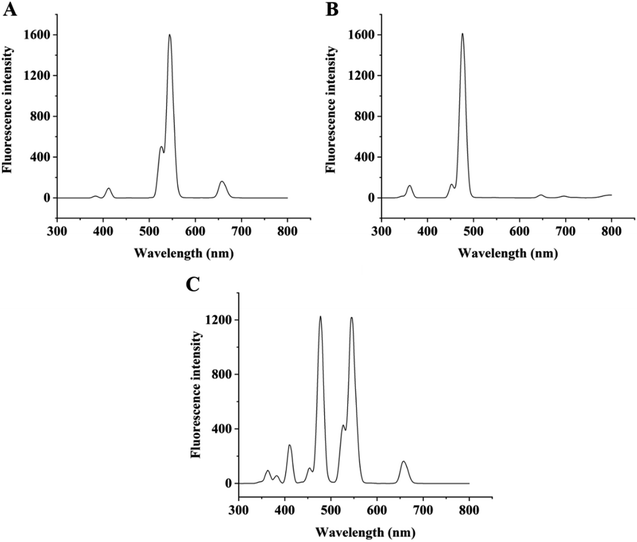 |
| Fig. 1 The emission spectra of signaling elements. (A) NaYF4:Yb,Er UCNPs conjugated with anti-thiamethoxam mAb; (B) NaYF4:Yb,Tm UCNPs conjugated with anti-dextran mAb; (C) the mixture of thiamethoxam and dextran signaling elements. | |
To construct a sensitive method, the multicolor UCFIA was optimized. The optimal volume of MNPs conjugated with thiamethoxam and dextran antigens was 40 μL because the multicolor UCFIA showed the highest fluorescence intensities at 544 nm (thiamethoxam) and 477 nm (dextran) (Fig. S2†). When the incubation time was 60 min, the value of the fluorescence intensity reached a plateau (Fig. S3†). As shown in Fig. S4,† the optimal concentrations of NaCl for the detection of thiamethoxam and dextran were 0.2 mol L−1 and 0.14 mol L−1, respectively. Since thiamethoxam and dextran were detected simultaneously, the optimal conditions should be selected according to the results of both compounds. Therefore, 0.2 mol L−1 NaCl was selected. The multicolor UCFIA showed a higher Fmax/IC50 in the detection of both thiamethoxam and dextran when the pH value was 7.4 (Fig. S5†). Although the optimal methanol content for thiamethoxam and dextran was 2.5% and 0%, respectively, organic solvent was required as a cosolvent in the preparation of the standard solution and sample extraction. Thus, 2.5% methanol was selected as the optimal methanol content. In summary, the optimal conditions of the immunoassay were 80 μL of thiamethoxam hapten–OVA-MNPs and dextran 40–OVA-MNPs (1
:
1, v/v), incubation for 60 min, 0.2 mol L−1 NaCl, pH = 7.4 and 2.5% methanol.
3.4 Sensitivity of the multicolor UCFIA
The calibration curves of thiamethoxam and dextran under the optimal conditions are shown in Fig. 2. The linear regression equations of thiamethoxam and dextran were y = 255.29x + 308.81 (R2 = 0.9960) and y = 137.77x − 52.31 (R2 = 0.9989), respectively. The IC50, limit of detection (LOD, IC10) and linear range (IC10 to IC90) of the multicolor UCFIA for thiamethoxam were 0.46 ng mL−1, 0.09 ng mL−1 and 0.09–2.34 ng mL−1, respectively. For dextran 2000, the IC50, LOD and linear range were 49.33 ng mL−1, 4.39 ng mL−1 and 4.39–544.43 ng mL−1, respectively. Compared with that of the ic-ELISAs for thiamethoxam and dextran in this study (see the section “Determination of mAb subtype and sensitivity in ic-ELISA”), the sensitivity of the multicolor UCFIA was similar with that of the ic-ELISA. However, the multicolor UCFIA had significant advantages in the detection time and operation procedure. More importantly, the multicolor UCFIA could detect thiamethoxam and dextran simultaneously.
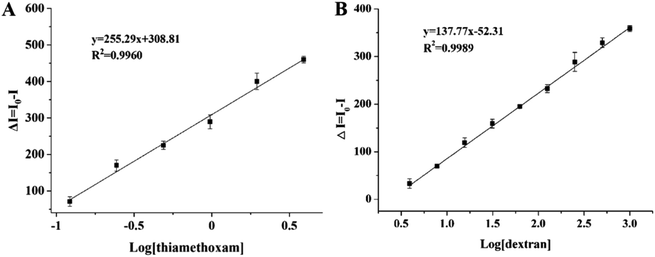 |
| Fig. 2 The calibration curve of the multicolor UCFIA for (A) thiamethoxam and (B) dextran 2000. | |
3.5 Specificity of the multicolor UCFIA
As shown in Tables 1 and 2, the multicolor UCFIA had no CR with the analogs of thiamethoxam and dextran, except for clothianidin (8.7% CR) and imidacloprid (1.5% CR). These CRs are mainly derived from the anti-thiamethoxam mAb, which was also present in the ic-ELSA for thiamethoxam (see the section “Specificity of the mAbs in ic-ELISA”). The results indicated that the multicolor UCFIA had good specificity and was similar to the ic-ELISAs developed with the same mAbs.
Table 1 Cross-reactivity of thiamethoxam toward some of its analogs
Analyte |
Structure |
IC50 (ng mL−1) |
Cross-reactivity (%) |
Thiamethoxam |
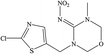 |
0.46 |
100 |
Imidaclothiz |
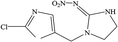 |
>1000 |
<0.05 |
Imidacloprid |
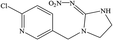 |
30.4 |
1.5 |
Acetamiprid |
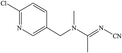 |
>1000 |
<0.05 |
Thiacloprid |
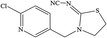 |
>1000 |
<0.05 |
Nitenpyram |
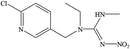 |
>1000 |
<0.05 |
Clothianidin |
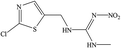 |
5.3 |
8.7 |
Table 2 Cross-reactivity of dextran 2000 toward some of its analogs
Compound |
IC50 (ng mL−1) |
Cross-reactivity (%) |
Dextran 2000 |
49.33 |
100 |
Sucrose |
>10 000 |
<0.5 |
Glucose |
>10 000 |
<0.5 |
Starch |
>10 000 |
<0.5 |
β-Glucan |
>10 000 |
<0.5 |
3.6 Analysis of spiked samples by the multicolor UCFIA
Before detection of the spiked samples, the matrix interference was evaluated by dilution with PBS. One gram of sugarcane juice that was not spiked with analytes was diluted with PBS f10 times and used to prepare standard curves of thiamethoxam. As shown in Fig. 3, the standard curve prepared using the matrix with an 80-fold dilution was similar to those in the matrix-free buffer, which meant that the matrix effect was eliminated. Since dextran is usually present in sugarcane, the original concentration of dextran in sugarcane juice was detected by a serial dilution. The detection results are summarized in Table 3. The original concentration of dextran in sugarcane was out of the linear range under the 50- and 100-fold dilutions. The results of the 200-, 400- and 800-fold dilutions were 99
740, 102
240 and 97
200 ng g−1, respectively. The average original concentration of dextran in sugarcane was 99
727 ng g−1, with a relative standard deviation (RSD) of 2.53%, which indicated that the 200-fold dilution could remove the matrix interference. To detect thiamethoxam and dextran simultaneously, 200-fold dilution was used in the detection of spiked samples.
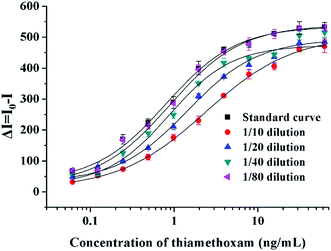 |
| Fig. 3 The matrix interference of sugarcane on the multicolor UCFIA for the detection of thiamethoxam. | |
Table 3 The original concentration of dextran in the sugarcane juice
Dilution factor |
50 |
100 |
200 |
400 |
800 |
Detection results (ng g−1) |
>554 |
>554 |
498.7 |
255.6 |
121.5 |
Original concentration (ng g−1) |
>27 200 |
>55 400 |
99 740 |
102 240 |
97 200 |
Table 4 shows the detection results of the spiked samples. The average recoveries of thiamethoxam and dextran 2000 in sugarcane juice were 82.9–93.3% with an RSD of 3.1–6.5% and 87.5–97.2% with an RSD of 2.1–4.6%, respectively. These results indicated that the accuracy of the multicolor UCFIA could meet the requirements for the simultaneous detection of thiamethoxam and dextran 2000 in sugarcane juice.
Table 4 Average spiked recoveries of thiamethoxam and dextran 2000 in the spiked sugarcane juice
Analyte |
Original concentration |
Spiked concentration |
Detection value |
Recovery (%) |
RSD (%) |
Thiamethoxam (μg kg−1) |
0 |
20 |
16.6 ± 0.5 |
82.9 |
3.1 |
100 |
93.3 ± 6.1 |
93.3 |
6.5 |
400 |
354.8 ± 17.6 |
88.7 |
5.0 |
Dextran 2000 (μg g−1) |
99.7 |
20 |
109.3 ± 5.0 |
91.3 |
4.6 |
40 |
122.2 ± 2.5 |
87.5 |
2.1 |
80 |
174.7 ± 6.3 |
97.2 |
3.6 |
4 Conclusion
In this study, mAbs of thiamethoxam and dextran were prepared, and they showed good sensitivity and specificity through ELISA evaluations. Based on the different emission wavelengths of the UCNPs NaYF4:Yb,Er and NaYF4:Yb,Tm, the mAbs of thiamethoxam and dextran were conjugated with these UCNPs to develop a multicolor UCFIA by using MNPs as a separation element. The IC50 and the linear range were 0.46 ng mL−1 and 0.09–2.34 ng mL−1 for thiamethoxam, respectively, and 49.33 ng mL−1 and 4.39–554.43 ng mL−1 for dextran. The multicolor UCFIA showed high sensitivity and significant advantages in the detection time and operation procedure. More importantly, the multicolor UCFIA realized the simultaneous detection of thiamethoxam and dextran. The multicolor UCFIA had no CR with the analogs of thiamethoxam and dextran, except for clothianidin (8.7%) and imidacloprid (1.5%). The recoveries of spiked samples showed that the assay was accurate and could be used to detect thiamethoxam and dextran. Therefore, this study presented an alternative tool for the simultaneous determination of thiamethoxam and dextran.
Conflicts of interest
There are no conflicts to declare.
Acknowledgements
This work was supported by the National Key Research and Development Program of China (Grant 2017YFF0210200).
References
- A. Brandt, A. Gorenflo, R. Siede, M. Meixner and R. Büchler, J. Insect Physiol., 2016, 86, 40–47 CrossRef CAS.
- M. Jameel, K. Jamal, M. F. Alam, F. Ameen, H. Younus and H. R. Siddique, Chemosphere, 2020, 254, 126875 CrossRef CAS.
- E. Caron-Beaudoin, R. Viau, A. Hudon-Thibeault, C. Vaillancourt and J. T. Sanderson, Toxicol. Appl. Pharmacol., 2017, 332, 15–24 CrossRef CAS.
- T. Miura, N. Ohno, N. N. Miura, Y. Adachi, S. Shimada and T. Yadomae, FEMS Immunol. Med. Microbiol., 1999, 24, 131–139 CAS.
- A. Jeanes, W. C. Haynes, C. A. Wilham, J. C. Rankin, E. H. Melvin, M. J. Austin, J. E. Cluskey, B. E. Fisher, H. M. Tsuchiya and C. E. Rist, J. Am. Chem. Soc., 1954, 20, 5041–5052 CrossRef.
- F. K. E. Imrie and R. H. Tilbury, Sugar Technol. Rev., 1972, 1, 291–361 CAS.
- D. F. Day, Sugar J., 1984, 46, 16–17 Search PubMed.
- C. Camargo, D. D. Snow, S. Onanong, T. E. Hunt and B. D. Siegfried, Crop Prot., 2019, 119, 134–140 CrossRef CAS.
- A. L. C. de Barros, C. G. de Abreu, C. C. R. F. da Cunha, D. A. D. Rodrigues, R. J. D. F. Afonso and G. A. da Silva, Environ. Sci. Pollut. Res., 2019, 26, 31609–31622 CrossRef.
- C. Kubik, E. Galas and B. Sikora, Int. Sugar J., 1994, 96, 376 Search PubMed.
- M. A. Clarke and M. A. Godshall, J. - Assoc. Off. Anal. Chem., 1988, 71, 276–279 CrossRef CAS.
- S. Y. Wang, Z. Li, X. J. Wang, E. R. Ni, L. Q. Zeng, F. H. Luo, J. H. Yan and D. F. Liang, J. Food Qual., 2016, 39, 408–414 CrossRef CAS.
- C. F. Brown and P. A. Inkerman, J. Agric. Food Chem., 1992, 40, 227–233 CrossRef CAS.
- V. Singleton, J. Horn, C. Bucke and M. Adlard, J. Am. Soc. Sugar Cane Technol., 2002, 22, 112–119 Search PubMed.
- Z. Z. Yu, Y. Tang, G. N. Cai, R. R. Ren and D. P. Tang, Anal. Chem., 2019, 91, 1222–1226 CrossRef CAS.
- L. T. Huang, J. L. Chen, Z. H. Yu and D. P. Tang, Anal. Chem., 2020, 92, 2809–2814 CrossRef CAS.
- H. J. Kim, S. Z. Liu, Y. S. Keum and Q. X. Li, J. Agric. Food Chem., 2003, 51, 1823–1830 CrossRef CAS.
- H. J. Kim, W. L. Shelver, E. C. Hwang, T. Xu and Q. X. Li, Anal. Chim. Acta, 2006, 571, 66–73 CrossRef CAS.
- L. Y. Ye, X. L. Wu, L. G. Xu, Q. K. Zheng and H. Kuang, Food Agric. Immunol., 2018, 29, 1173–1183 CrossRef CAS.
- D. F. Liang, J. H. Yan, L. Q. Zeng, S. Y. Wang, Y. H. Li, E. Ni and L. Yu, Int. Sugar J., 2011, 113, 654–659 CAS.
- S. Y. Wang, Z. Li, X. J. Wang, E. Ni, L. Q. Zeng, F. H. Luo, J. H. Yan and D. F. Liang, J. Food Qual., 2016, 39, 408–414 CrossRef CAS.
- S. Y. Wang, Z. Li, X. J. Wang, S. Lv, Y. Yang, L. Q. Zeng, F. H. Luo, J. H. Yan and D. F. Liang, Monoclonal Antibodies Immunodiagn. Immunother., 2014, 33, 334–339 CrossRef CAS.
- W. Feng, C. Han and F. Li, Adv. Mater., 2013, 25, 5287–5303 CrossRef CAS.
- Z. B. Luo, Q. G. Qi, L. J. Zhang, R. J. Zeng, L. S. Su and D. P. Tang, Anal. Chem., 2019, 91, 4149–4156 CrossRef CAS.
- P. Y. Qiu, N. Zhou, H. Y. Chen, C. L. Zhang, G. Gao and D. X. Cui, Nanoscale, 2013, 5, 11512–11525 RSC.
- J. M. Lan, L. Li, Y. X. Liu, L. Yan, C. Y. Li, J. H. Chen and X. S. Chen, Microchim. Acta, 2016, 183, 3201–3208 CrossRef CAS.
- Z. B. Luo, L. J. Zhang, R. J. Zeng, L. S. Su and D. P. Tang, Anal. Chem., 2018, 90, 9568–9575 CrossRef CAS.
- Z. L. Qiu, J. Shu, J. F. Liu and D. P. Tang, Anal. Chem., 2019, 91, 1260–1268 CrossRef CAS.
- S. Z. Lv, K. Y. Zhang, L. Zhu and D. P. Tang, Anal. Chem., 2020, 92, 1470–1476 CrossRef CAS.
- L. Zhou, B. He, J. Huang, Z. Cheng, X. Xu and C. Wei, ACS Appl. Mater. Interfaces, 2014, 6, 7719–7727 CrossRef CAS.
- H. J. You, X. D. Hua, L. Feng, N. N. Sun, Q. Rui, L. M. Wang and M. H. Wang, Microchim. Acta, 2017, 184, 1085–1092 CrossRef CAS.
- Z. X. Tao, J. Q. Deng, Y. Wang, H. Chen, Y. Ding, X. D. Hua and M. H. Wang, Environ. Sci. Pollut. Res., 2019, 26, 23471–23479 CrossRef CAS.
- X. D. Hua, H. J. You, P. W. Luo, Z. X. Tao, H. Chen, F. Q. Liu and M. H. Wang, Anal. Bioanal. Chem., 2017, 409, 6885–6892 CrossRef CAS.
- G. L. Qian, L. M. Wang, Y. R. Wu, Q. Zhang, Q. Sun, Y. Liu and F. Q. Liu, Food Chem., 2009, 117, 364–370 CrossRef CAS.
- R. C. Nowinski, M. E. Lostrom, M. R. Tam, M. R. Stone and W. N. Burnette, Virology, 1979, 93, 111–126 CrossRef CAS.
- X. Hun and Z. Zhang, Biosens. Bioelectron., 2007, 22, 2743–2748 CrossRef.
- R. Romero-González, A. Garrido Frenich and J. L. Martínez Vidal, Talanta, 2008, 76, 211–225 CrossRef.
Footnote |
† Electronic supplementary information (ESI) available. See DOI: 10.1039/d0ra07954e |
|
This journal is © The Royal Society of Chemistry 2021 |