Simplified and rapid determination of Ca, K, Mg, and Na in fruit juices by flowing liquid cathode atmospheric glow discharge optical emission spectrometry†
Received
13th April 2021
, Accepted 26th May 2021
First published on 30th May 2021
Abstract
Novel atmospheric pressure glow discharge (APGD) plasma sustained between a miniaturized flowing liquid cathode (FLC) and a pin-type anode was applied as an excitation source for the determination of Ca, K, Mg, and Na in fruit (apple, banana, blackcurrant, lemon, lime, pomegranate, quince, and tomato) juices by optical emission spectrometry. A simplified sample preparation procedure of juices, involving only their 1000-fold dilution and acidification with HNO3 to a concentration of 0.1 mol L−1, was proposed. The content of K and Na was determined using simple standard solutions for calibration, while the determination of Ca and Mg required the use of the standard addition method, due to matrix effects. Under the optimal conditions, the developed method assured instrumental detection limits of 5.69 (Ca), 0.14 (K), 0.63 (Mg), and 0.02 (Na) μg L−1. Owing to such low detection limits, the content of all studied metals could be determined using only one sample dilution. The proposed FLC APGD OES method was established to provide reliable results with high accuracy and very good precision.
1. Introduction
Fruit juices are a rich source of nutritionally important compounds, such as antioxidants, carbohydrates, carotenoids, flavonoids, macroelements, phenolic acids, pectins, proteins, vitamins and others.1–3 Due to increasing knowledge about their nutritional and health benefits, along with their good taste, fruit juices are widely consumed beverages by different age groups all over the world.2,4,5 Considering elements that are present in different types of juices, they can be divided into three groups: macroelements (e.g., Ca, K, Mg, Na), microelements (e.g., Cu, Fe, Zn), and trace elements (e.g., Cd, Cr, Mn, Pb).1,2,6 As the total content of elements in these beverages may reach 10 g L−1, their contribution to the human diet is significant.1 It was previously revealed that the consumption of fruit juices on a daily basis significantly covers recommended daily allowances (RDAs) of many nutritionally important elements, such as Al (up to 19%), Ca (up to 32%), Cr (up to 87%), Cu (up to 35%), Fe (up to 26%), K (up to 55%), Mg (up to 22%), Na (up to 14%), and Zn (up to 21%).6 Hence, it is of great concern to develop quick and simple methods for the elements determination in different types of juices.3,7
Among various methods that are applied for elemental analysis of juices, spectrometric techniques are the prevailing ones and they include: flame atomic absorption spectrometry (FAAS),3,8,9 graphite furnace atomic absorption spectrometry (GF AAS),9,10 inductively coupled plasma optical emission spectrometry (ICP OES),11–14 and inductively coupled plasma mass spectrometry (ICP MS).15–17 However, direct analysis of juices with the aid of the aforesaid techniques causes many troubles due to a complex organic matrix of samples, their high viscosity as well as the presence of dissolved solids in them.1 For this reason, samples of juices usually have to be either digested before the analysis, in order to remove the organic matter, or significantly diluted to lower the organic substances concentration.1,15,16,18 Although these procedures allow to overcome the adverse impact of the matrix substances, they require the use of relevant amounts of reagents, including concentrated acids, and have to be subjected to prolonged high temperature treatments that may be a source of sample contamination.1,15
Apart from the above, a number of disadvantages have been recognized in regard to the application of those techniques, including bulky and complex instrumentation, high power and gas consumption, and high purchasing and operating costs.
To overcome those inconveniences, the attention of many researchers has been attracted to the development of novel miniaturized excitation sources of simplified design used for OES measurements which would assure similar or better (to the currently applied large-scale ICP instrumentation) analytical characteristics at low costs, reduced power, and reduced/no gas consumption.19–21 Discharges generated in contact with liquids enjoy a special interest and a number of such techniques has been proposed for the time being, e.g., electrolyte jet cathode glow discharge (EJC GD),22 discharge on boiling in a channel (DBC),23 liquid electrode plasma (LEP),24 electrolyte cathode discharge (ELCAD),25 solution cathode glow discharge (SCGD),26 flowing liquid cathode-atmospheric pressure glow discharge (FLC APGD),27 liquid sampling-atmospheric pressure glow discharge (LS APGD),28 solution anode glow discharge (SAGD),29 flowing liquid anode-atmospheric pressure glow discharge (FLA APGD),30 liquid drop anode-atmospheric pressure glow discharge (LDA APGD),31 and hanging drop electrode-atmospheric pressure glow discharge (HDE APGD).32 From all the aforesaid methods, FLC APGD and FLA APGD seem to be the most promising ones. FLA APGD offers detection limits (DLs) that are incomparably better than the ones reported for the other methods, nonetheless the FLA APGD technique suffers from significant matrix effects coming from concomitant ions, present in the FLA solution, which restricts the real sample analysis.30,33–36 Moreover, the application of the FLA APGD method is restricted to the determination of only 8 elements (namely Ag, Bi, Cd, In, Hg, Pb, Tl, and Zn) which are known to be transported to this excitation source in the form of volatile species.30,33,37 On the other hand, although the DLs achievable in the FLC APGD method are up to a few orders of magnitude higher and of a similar level to those obtained for the ICP OES method, this method is quite resistant to matrix effects.30,38–41 Therefore, the latter method could be successfully applied in the elemental analysis of different environmental and food samples, including aquatic plants,42 blood,40 coal fly ashes,43 human hair,44 ores,45 soils,46 stream sediments,44 tuna fish,42 and wastewater,47 after their digestion or extraction. Moreover, ground,43 mineral,48 natural,49 pond,46 river,50 and tap49 waters as well as brines51 and honeys52 were directly analyzed, proving high sensitivity, precision and accuracy of the developed systems.
Hence, the suitability of the APGD microsource generated between a small-sized FLC solution and a pin-type tungsten anode for the determination of Ca, K, Mg, and Na in different juice samples with a simplified sample preparation procedure, involving only their dilution and acidification, was examined. To the best of our knowledge, such analysis for juices using either the FLC APGD method or any other microplasma source has never been performed. To achieve that goal, the crucial operating parameters of the discharge were optimized at first. Subsequently, the DLs of the analytes were assessed in the optimized conditions. Furthermore, the impact of the dilution factor on the analytes signal intensities was studied to verify both the impact of the organic matrix on the analytes signals as well as the plasma resistance on this matrix. The proposed method was then applied for an analysis of 8 commercial fruit juices for the content of Ca, K, Mg, and Na. The results obtained for FLC APGD measurements were compared to those provided by a reference method (ICP OES with wet digestion) and discussed.
2. Experimental
2.1. Instrumentation
A schematic drawing of the studied FLC APGD system is displayed in Fig. 1. The discharge was sustained in an open-to-air chamber between a tungsten nozzle (OD/ID 3/1 mm, length 50 mm) and a vertically oriented tungsten rod (OD 2 mm, length 170 mm). The sample solutions acidified with HNO3 to its concentration up to 0.1 mol L−1, which served as the cathode, were pumped through the tungsten nozzle by means of a 3-channel REGLO ICC peristaltic pump (Ismatec, USA) at different flow rates being set in the 1.0–4.0 mL min−1 range. As the solution reached the tungsten tube's top it flowed down to a PTFE reservoir, from which it was pumped out with the aid of the previously mentioned peristaltic pump. The distance between the solution surface and the anode rod (so-called discharge gap) was set to approximately 1.5 mm. The electrical contact was provided directly to the tungsten rod and with the aid of a platinum spiral wrapped around the tungsten nozzle. The voltage being in the 800–1200 V range was applied to both electrodes by a HV dc power supply (model DP50H-024PH, DSC-Electronics, Germany). Its exact value was depended on the applied sample flow rate and the discharge current. To stabilize the discharge, a 6.9 kΩ ballast resistor was connected into the circuit.
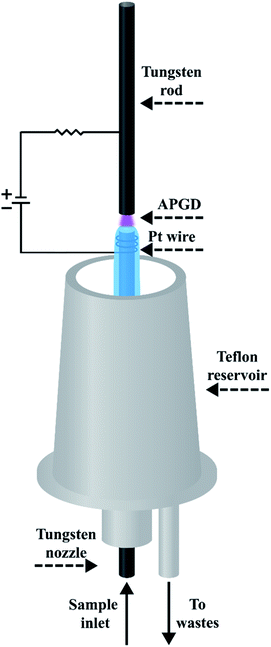 |
| Fig. 1 A schematic drawing of the developed FLC APGD systems. | |
The radiation emitted by APGD was imaged (1
:
1) on the entrance slit (10 μm) of a Shamrock 500i imaging spectrometer (Andor, UK), using an achromatic quartz lens (f = 80). The spectrometer was equipped with a holographic grating (1800 lines mm−1) and a Newton DU-920P-OE UV-Vis CCD camera (Andor, UK). The integration time was 10 s during all experiments and intensities of atomic emission lines of the studied elements were background corrected. The following wavelengths of the analytes were traced during all experiments: Ca (422.7 nm), K (766.5 nm), Mg (285.2 nm), and Na (589.0 nm).
An Agilent 5110 synchronous vertical dual view (SVDV) ICP OES instrument was used to measure total concentrations of studied elements in digested fruit juices versus simple standards solutions. The ICP OES spectrometer was equipped with an easy-fit quartz torch with a standard 1.8 mm injector and a sample introduction system consisted of a Seaspray nebulizer and a double-pass glass cyclonic spray chamber. The instrument was run under operating conditions given in Table SI-1,† combining axial and radial acquisition of radiation emitted by the vertical plasma over the entire wavelength range and in a single measurement.
2.2. Reagents and sample preparation
Deionized water (18.2 MΩ cm) from a Polwater water purification system (Labopol-Polwater, Poland) was used throughout the study. All chemicals were at least of analytical grade. Stock standard solutions of Ca, K, Mg, and Na (1000 mg L−1), obtained from Sigma-Aldrich (Germany), were utilized to prepare all working standard solutions. To acidify the FLC solutions, an ACS grade concentrated HNO3 (65% m/m) solution (Merck, Germany) was employed. The same concentrated HNO3 along with a solution of 30% H2O2 (Merck, Germany) were applied for samples digestion before their analysis with the aid of ICP OES.
Samples of 8 fruit juices were purchased at local stores. They were produced by 6 different manufacturers, which were encoded with the first letters of their names, i.e., E, F, H, X, K, and T, and included apple (A), banana (B), blackcurrant (C), lemon (L), lime (M), pomegranate (P), quince (Q), and tomato (T) juices.
Prior to FLC APGD OES measurements, the samples of banana and tomato juices were initially centrifuged at 15
000 rpm for 10 min to remove any dissolved particles. Followingly, appropriate aliquots of each of the analyzed juices were transferred into a twist-cup container and acidified with concentrated HNO3 to its concentration of 0.1 mol L−1. Finally, all samples were filled with deionized water to reach the final dilution of the juices of 1000-fold (on the weight basis). Such prepared samples were analyzed in regard to the external calibration curves with simple standards solutions.
All juice samples were also analyzed using the standard addition method for calibration. In this case, all the above mentioned steps of sample preparation were repeated, however, after the acidification, each sample was divided into four separate parts, and the standard addition was performed on the last three of them, using a mixed solution of Ca and Mg (10 mg L−1). Subsequently, all samples were filled with deionized water to reach the final dilution of the juices of 1000-fold (on the weight basis).
As regards ICP OES measurements, the aforesaid juice samples were wet-digested in order to destroy the organic matrix. For this purpose, samples of 5.0 g of each juice and concentrated HNO3 (5.0 mL) were transferred into a DigiPrep tube and heated for 180 min at 120 °C. After cooling, 5.0 mL of H2O2 (30%) was added and the samples were further heated for 60 min. Followingly, such prepared samples were filled with deionized water to 50.0 g. After that, appropriate amounts of digested solutions were transferred into a twist-cup container and filled with deionized water up to 30.0 g. Two dilutions of each juice sample were prepared, i.e., 12-fold and 750-fold (on the weight basis).
All the above mentioned samples were prepared in triplicates, besides, appropriate procedural blanks were prepared and considered in the final results. The determination of Ca, K, Mg, and Na was performed, for all samples, in regard to the external calibration curves with simple standards solutions.
3. Results and discussion
3.1. Optimization of working parameters
In order to successfully apply the proposed FLC APGD method for the elemental analysis of juices and considering especially the elements of poorer sensitivity and present at lower concentrations, i.e., Ca and Mg, the optimization of operating parameters was performed. The sample flow rate (1.0–4.0 mL min−1) along with the discharge current (30–60 mA) were identified to have a significant impact on the analytes signal intensities. The acid concentration was omitted during the optimization step as it was repeatedly shown that the most optimal acid concentration is 0.1 mol L−1 (or similar). Therefore, all studied samples were acidified with HNO3 to the concentration of 0.1 mol L−1. The optimization was carried out using a mixed standard solutions of Ca, K, Mg and Na at their concentrations of 0.1 (K, Na) or 0.5 mg L−1 (Ca, Mg). Based on our previous experience,33,35 it was to be expected that those parameters would be dependent on each other, therefore, there were optimized together. The results are presented in Fig. 2.
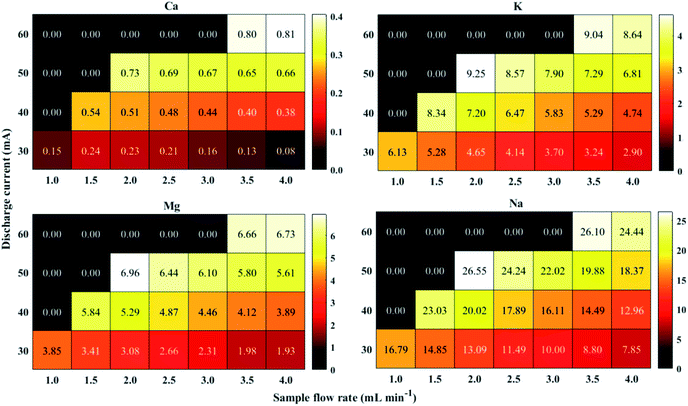 |
| Fig. 2 The effect of the sample flow rate and the discharge current on the signal to background ratio (SBR) of the analytical lines of Ca, K Mg, and Na. “0.00” indicates the parameters combination at which the discharge was unstable and the measurement could not be taken. | |
As it can be seen from the figure, the discharge could be stably maintained over the whole range of sample flow rates, but only for the discharge current of 30 mA. At higher amperages, the discharge was unstable when lower flow rates were applied. That being so, for the highest studied discharge current, i.e., 60 mA, the discharge could be maintained only for the samples flow rates of 3.5 and 4.0 mL min−1, however its visual stability was still slightly impaired. The reason for the observed lack of the stability was likely an insufficient electrical conductivity of the solution at lower samples flow rates.
As for the relationship between the applied discharge current and the received signal-to-background ratios (SBRs), it was revealed that SBRs of all studied elements increased with a growing discharge current. These results are consistent with what had previously been reported.53,54 The most probable explanation for the increase of the SBRs values along with the increase of the discharge current is higher electron density in the discharge. This in turn leads to better excitation conditions.
The results reported previously regarding the impact of the sample flow rate on the signal intensity/SBR are ambiguous. Usually, as the sample flow rate is growing, the response from the analytes is found to either increase or decrease up to a certain limit and increase afterward.39,45,55,56 However, a decreasing tendency of the response from the analytes over the whole range of the sample flow rates was noted as well.35,57 Herein, the SBR values generally declined for all analytes with the increasing sample flow rate, regardless of the discharge current applied. The root of the aforesaid results is unclear and a more in-depth study is needed to provide a reliable clarification, however, this was not the aim of this work.
It is noteworthy that the increasing tendency of the SBR values observed at higher discharge currents was compensated by the decreasing tendency coming from higher solution flow rates, as the discharge could not be maintained at the lower ones in these conditions. This made the evaluation of the optimal operating parameters not to be so obvious. The highest SBR for Ca was received for the discharge current of 60 mA and the solution flow rate of 4.0 mL min−1, while slightly higher SBR for the other analytes was obtained when the discharge current of 50 mA and the sample flow rate of 2.0 mL min−1 were applied. As an attempt of performing long-time analyses was made, the plasma stability was taken into consideration and the visual discharge stability was noted to be better at the discharge current of 50 mA and the sample flow rate of 2.0 mL min−1. Therefore, those parameters were established to be optimal for multi-element analysis, hence they were applied in all subsequent experiments.
3.2. Analytical characteristics
To have a better insight into the appropriate juice samples dilution for their analysis on the content of Ca, K, Mg, and Na in the further part of this study, the analytical performance of the developed FLC APGD system was determined under the optimized conditions. For that purpose, the DLs, the upper linearity ranges (ULRs) as well as the precision were determined for all studied elements (see Table 1). Instrumental DLs were calculated as 3s/a, where “3s” stands for 3 times the standard deviation of 10 consecutive measurements of an appropriate blank solution, and “a” stands for the sensitivity of a corresponding calibration curve. The upper linearity range was examined using 9 standard solutions in the concentration range of 0.01–10 mg L−1. The precision was expressed as the relative standard deviation (RSD) for 10 consecutive measurements of the standard solutions, containing Ca, K, Mg, and Na at the concentrations of 200, 10, 100 and 10 μg L−1, respectively. The RSD was measured 3 times and the averaged results were given. When comparing the received DLs with those reported over the last five years for similar FLC APGD systems,58 they were similar (in the case of K) or significantly improved (in the case of all analytes), i.e., 2–81 times for Ca, 0.9–2785 times for K, 3–540 times for Mg, and 2–2400 times for Na. The DLs presented herein are also similar or better to those achieved for other spectrometric techniques, such as FAAS,59–62 or ICP OES.60,63,64 In such a case, it was expected the obtained low DLs of Ca, K, Mg and Na would allow to apply higher dilutions of the analyzed juices, in order to avoid matrix effects coming from organic and inorganic constituents of the samples.
Table 1 The analytical performance of the studied FLC APGD OES systema
Element |
DL (μg L−1) |
ULR (mg L−1) |
R
2
|
Sensitivity (a.u. per mg per L) |
RSD (%) |
DL – detection limit. ULR – upper linearity range.
|
Ca |
5.69 |
10 |
0.9998 |
2.32 × 104 |
1.02 |
K |
0.14 |
10 |
0.9999 |
3.01 × 106 |
1.12 |
Mg |
0.63 |
10 |
0.9992 |
7.67 × 105 |
0.73 |
Na |
0.02 |
5 |
1.0000 |
1.02 × 107 |
0.89 |
The calibration curves were linear (R2 > 0.999) over the whole studied concentration range, i.e., up to 10 mg L−1. As for Na, its calibration curve was linear (R2 = 1) only up to the Na concentration of 5 mg L−1 due to its high sensitivity which led to the saturation of the CCD detector above this concentration. Such dynamic linearity ranges are suitable for multi-element analysis, therefore it was expected that it would be possible to analyze all studied elements preparing only one dilution of the analyzed samples.
Regarding the measurements precision, it was found that the developed FLC APGD OES method assured excellent precision, changing in the range of 0.73–1.12% for relatively low analytes concentrations.
3.3. Plasma stability and matrix effects
To assess the suitability of the developed FLC APGD method for determining the content of Ca, K, Mg, and Na in various fruit juices, only diluted in water (without any digestion) and acidified with HNO3 to 0.1 mol L−1, two aspects had to be considered at the beginning. The first one was the plasma robustness in reference to the juice matrix and the second one were the matrix effects, i.e., the impact of both organic and inorganic constituents of juices on the analytes signal intensities. Due to high variety of different elements as well as organic compounds, and their concentrations, present in different types of juice,65–68 the results obtained based on a solution containing a juice-like organic matrix would not be of great credibility. Therefore, both those factors were determined by measuring the analytes signal intensities in five different dilutions (namely 10-, 50-, 100, 500-, and 1000-fold) of each analyzed juice, corresponding to its concentration in a prepared sample solution of 10, 2, 1, 0.2 and 0.1%. To reliably assess the impact of each juice matrix on the analytes signal intensities, they were depicted on a graph presenting the relationship between the analyte signal intensity and the juice dilution (expressed as the abovementioned percentage concentration) and the linearity of the received relationships was investigated to evaluate the concentration ranges at which the curve is linear, meaning, supposedly there are no matrix effects. For better visibility, the data obtained from those graphs was shown in Table 2, which presents the upper linearity ranges, expressed as the highest percentage concentrations (corresponding to the possible lowest dilutions) at which the curves were still linear, the slopes of the received curves along with their R2, and the range of the RSD values, received for different dilutions of a given juice, for all analytes.
Table 2 The upper linearity ranges (ULR), expressed as the highest percentage juice concentrations (corresponding to the possible lowest dilutions) at which the curves of the signal intensity of the analytical line versus these concentrations are still linear, the slopes of the received curves along with their R2, and the range of relative standard deviation (RSD) values, received for different dilutions of analyzed juices, for Ca, K, Mg and Na during the FLC APGD OES analysis
Element |
Parameter |
AT |
BX |
CF |
LE |
ME |
PH |
QK |
TT |
Expressed as the RSD range for all dilutions measurements.
|
Ca |
ULR (%) |
10 (10-fold) |
10 (10-fold) |
10 (10-fold) |
2 (50-fold) |
2 (50-fold) |
2 (50-fold) |
2 (50-fold) |
2 (50-fold) |
Slope |
6.94 × 103 |
3.60 × 103 |
1.49 × 104 |
2.83 × 104 |
2.51 × 104 |
1.46 × 104 |
2.51 × 104 |
1.22 × 104 |
R
2
|
0.9952 |
0.9992 |
0.9962 |
0.9932 |
0.9976 |
0.9958 |
0.9976 |
0.9965 |
RSD (%)a |
0.36–8.15 |
0.87–8.41 |
0.27–4.58 |
0.60–3.90 |
0.41–3.26 |
0.36–4.73 |
0.10–2.89 |
0.42–2.97 |
K |
ULR (%) |
2 (50-fold) |
2 (50-fold) |
2 (50-fold) |
1 (100-fold) |
1 (100-fold) |
1 (100-fold) |
1 (100-fold) |
1 (100-fold) |
Slope |
2.40 × 107 |
2.37 × 107 |
1.45 × 107 |
4.27 × 107 |
4.65 × 107 |
5.67 × 107 |
3.97 × 107 |
5.45 × 107 |
R
2
|
0.9996 |
0.9994 |
0.9964 |
0.9993 |
0.9989 |
0.9997 |
0.9997 |
0.9984 |
RSD (%)a |
0.19–0.64 |
0.12–0.39 |
0.07–0.62 |
0.20–0.25 |
0.03–0.34 |
0.03–0.54 |
0.21–0.56 |
0.14–0.37 |
Mg |
ULR (%) |
10 (10-fold) |
10 (10-fold) |
10 (10-fold) |
10 (10-fold) |
10 (10-fold) |
10 (10-fold) |
10 (10-fold) |
10 (10-fold) |
Slope |
1.73 × 105 |
2.58 × 105 |
1.17 × 105 |
3.14 × 105 |
3.20 × 105 |
2.85 × 105 |
3.82 × 105 |
2.08 × 105 |
R
2
|
0.9969 |
0.9993 |
0.9978 |
0.9945 |
0.9945 |
0.9925 |
0.9931 |
0.9596 |
RSD (%)a |
0.29–0.96 |
0.19–1.16 |
0.20–1.42 |
0.26–1.61 |
0.06–1.91 |
0.43–2.13 |
0.71–1.52 |
0.65–2.62 |
Na |
ULR (%) |
10 (10-fold) |
10 (10-fold) |
10 (10-fold) |
2 (50-fold) |
2 (50-fold) |
2 (50-fold) |
2 (50-fold) |
0.2 (500-fold) |
Slope |
1.34 × 106 |
6.71 × 105 |
3.32 × 106 |
6.06 × 106 |
5.65 × 106 |
2.00 × 107 |
1.03 × 107 |
2.08 × 108 |
R
2
|
0.9952 |
0.9990 |
0.9999 |
0.9997 |
0.9998 |
0.9995 |
0.9998 |
0.9999 |
RSD (%)a |
0.11–1.71 |
0.15–0.96 |
0.16–0.89 |
0.07–0.53 |
0.53–0.71 |
0.08–0.67 |
0.39–2.11 |
0.40–0.59 |
As it can be seen from that table, the upper linearity ranges differed between elements and the type of juices. Only the signal of Mg increased linearly over the whole range of the studied dilutions, regardless of the juice type. For Ca and Na, the highest possible concentration giving the linear response differed between juices, however, it was the same for a given juice. Exemplary, the upper linearity range for Ca in quince (QK) and tomato (TT) juices are equal to 10 and 2% (10- and 50-fold dilution), respectively and the same upper linearity range is observed for Na in those (and any others) juices. It is also noteworthy that the mentioned upper linearity ranges for Ca and Na correlated well with the sensitivity of the received curves (the poorer sensitivity, the higher upper linearity range). The only exception from that rule is tomato (TT) juice, for which the dilutions above 500-fold (0.2%) could not be measured at all due to the saturation of the CCD detector, suggesting a very high concentration of this element in this juice. This was also the case for other juices (namely lemon (LE), lime (ME), pomegranate (PH), and quince (QK) juices) for Na but only when the juice concentration exceeded 2% (which corresponds to the dilutions being lower than 50-fold). Thus, it can be stated that the curves of Na were linear in the whole range which could be actually measured. However, there was no saturation of the CCD camera observed when Ca was measured, therefore, apparently, some matrix effects occur when a given juice contains higher content of Ca. As for K, its content in each of the analyzed juices was too high to be able to measure the whole dilution ranges and, similarly to Na, the obtained curves were linear in the whole possible-to-study range, i.e., 1 (lemon (LE), lime (ME), pomegranate (PH), quince (QK), and tomato (TT) juices) or 2% (apple (AT), banana (BX), and blackcurrant (CF) juices). The aforesaid results may suggest that there are no matrix effects for the majority of the analytes (with exception of Ca at 10% samples of some juices), regardless of the juice type and its dilution. Nevertheless, the possibility of measuring the signal intensities of K and Na should be taken into account when considering higher dilutions of juices samples.
The plasma stability was evaluated based on the RSD of 10 consecutive measurements of each analytical line as well as visual observations for all studied sample dilutions. It was established that the RSD of the signal intensities of K and Na was below 1% in all (K) or in the vast majority (Na) of cases, indicating excellent precision, regardless of the juice type and applied dilution. For Mg, the RSD values were slightly higher, however they still did not exceed 3% for any juice at any dilution. Nevertheless, relatively high RSD values (4.5–8.5%) were obtained for Ca but only for the dilution factor of 1000 and only for a few juice types. It was also noted that the RSD values of this element (in 1000-fold diluted samples) correlated well with the sensitivity of the obtained curves (the poorer sensitivity, the worse RSD), meaning that the observed impaired signal stability was due to a relatively low Ca concentration in some juice samples, leading quite low analyte signal intensity after their 1000-fold dilution. Visually, lower stability was observed only when a 10-fold diluted solution was introduced into the FLC APGD system, however, considering the abovementioned precision, it was concluded that it was stable enough to perform the analysis.
3.4. Determination of selected elements in juice samples
Concentrations of Ca, K, Mg, and Na were measured in 8 different fruit juices with the aid of the FLC APGD OES method. The analyzed samples were diluted 1000-fold and acidified with HNO3 to 0.1 mol L−1. It was expected that applying a high dilution factor, the sample matrix would not influence neither the background intensity nor the analytes sensitivity, which then would not deteriorate the method DLs of Ca, K, Mg and Na in regard to the instrumental DLs presented above.
At first, simple standard solutions were used for calibration. To assess the reliability of the proposed method, all the abovementioned juices were also analyzed by ICP OES, however in this case, the samples needed to be wet-digested before the analysis. The obtained results are displayed in Tables 3 and 4.
Table 3 The content of K and Na determined in the analyzed juice samples, i.e., apple (AT), banana (BX), blackcurrant (CF), lemon (LE), lime (ME), pomegranate (PH), quince (QK), and tomato (TT) juices, using simple standard solutions for calibrationa
Juice |
Method |
K |
Na |
Mean ± CI |
RSD (%) |
Mean ± CI |
RSD (%) |
CI – confidence interval (for 95% confidence level).
|
AP |
ICP OES |
931.18 ± 5.09 |
0.22 |
11.54 ± 0.15 |
0.54 |
FLC APGD |
1014.6 ± 24.69 |
0.98 |
11.94 ± 0.52 |
1.77 |
Recovery (%) |
109 |
103 |
BX |
ICP OES |
897.56 ± 21.64 |
0.97 |
6.21 ± 0.17 |
1.06 |
FLC APGD |
966.48 ± 17.76 |
0.74 |
6.65 ± 0.37 |
2.19 |
Recovery (%) |
108 |
107 |
CF |
ICP OES |
482.67 ± 23.38 |
1.95 |
30.72 ± 0.07 |
0.10 |
FLC APGD |
492.38 ± 17.74 |
1.45 |
34.08 ± 0.84 |
0.99 |
Recovery (%) |
102 |
111 |
LE |
ICP OES |
1610.92 ± 58.43 |
1.46 |
53.11 ± 6.31 |
4.78 |
FLC APGD |
1745.16 ± 44.67 |
1.03 |
56.8 ± 3.06 |
2.16 |
Recovery (%) |
108 |
107 |
ME |
ICP OES |
1766.92 ± 22.38 |
0.51 |
52.16 ± 0.32 |
0.24 |
FLC APGD |
1893.04 ± 31.50 |
0.67 |
55.03 ± 0.99 |
0.72 |
Recovery (%) |
107 |
106 |
PH |
ICP OES |
2157.64 ± 43.43 |
0.81 |
183.07 ± 7.45 |
1.64 |
FLC APGD |
2241.24 ± 47.33 |
0.85 |
177.43 ± 10.48 |
2.38 |
Recovery (%) |
104 |
96.9 |
QK |
ICP OES |
1438.33 ± 13.22 |
0.37 |
89.27 ± 1.14 |
0.51 |
FLC APGD |
1536.04 ± 38.93 |
1.02 |
92.31 ± 3.13 |
1.37 |
Recovery (%) |
107 |
103 |
TT |
ICP OES |
2261.23 ± 10.68 |
0.19 |
2250.12 ± 16.77 |
0.3 |
FLC APGD |
2326.93 ± 24.87 |
0.43 |
2263.95 ± 37.12 |
0.66 |
Recovery (%) |
103 |
101 |
Table 4 The content of Ca and Mg determined in the analyzed juice samples, i.e., apple (AT), banana (BX), blackcurrant (CF), lemon (LE), lime (ME), pomegranate (PH), quince (QK), and tomato (TT) juices, using standard addition method for calibrationa
Juice |
Method |
Ca |
Mg |
Mean ± CI |
RSD (%) |
Mean ± CI |
RSD (%) |
CI – confidence interval (for 95% confidence level).
|
AP |
ICP OES |
43.11 ± 0.67 |
0.62 |
45.17 ± 0.12 |
0.10 |
FLC APGD |
43.89 ± 1.91 |
1.76 |
49.39 ± 0.72 |
0.58 |
Recovery (%) |
102 |
109 |
BX |
ICP OES |
18.41 ± 2.58 |
5.67 |
70.35 ± 0.67 |
0.39 |
FLC APGD |
19.28 ± 0.32 |
0.68 |
67.61 ± 1.17 |
0.69 |
Recovery (%) |
105 |
96.1 |
CF |
ICP OES |
106.38 ± 0.97 |
0.37 |
30.55 ± 0.10 |
0.14 |
FLC APGD |
110.31 ± 4.02 |
1.47 |
30.27 ± 0.17 |
0.22 |
Recovery (%) |
104 |
99.1 |
LE |
ICP OES |
196.25 ± 41.93 |
8.60 |
125.15 ± 0.89 |
0.29 |
FLC APGD |
201.68 ± 5.37 |
1.07 |
128.73 ± 4.55 |
1.42 |
Recovery (%) |
103 |
103 |
ME |
ICP OES |
217.34 ± 3.23 |
0.60 |
107.25 ± 1.69 |
0.63 |
FLC APGD |
232.3 ± 10.33 |
1.79 |
109.34 ± 5.04 |
1.86 |
Recovery (%) |
107 |
102 |
PH |
ICP OES |
96.42 ± 1.09 |
0.46 |
78.33 ± 0.84 |
0.43 |
FLC APGD |
90.83 ± 12.17 |
5.40 |
77.14 ± 0.30 |
0.15 |
Recovery (%) |
94.2 |
98.5 |
QK |
ICP OES |
258.67 ± 10.53 |
1.64 |
113.35 ± 1.04 |
0.37 |
FLC APGD |
260.30 ± 34.98 |
5.41 |
109.84 ± 9.49 |
3.48 |
Recovery (%) |
101 |
96.9 |
TT |
ICP OES |
106.63 ± 1.37 |
0.52 |
105.26 ± 2.14 |
0.82 |
FLC APGD |
108.38 ± 5.59 |
2.08 |
96.74 ± 1.02 |
0.41 |
Recovery (%) |
102 |
91.9 |
Tables 3 and SI-2† present the content of Ca, K, Mg, and Na in the analyzed juices obtained from ICP OES measurements along with the corresponding results received from the FLC APGD OES measurements (using simple standard solutions for calibration), and the precision for all those results. The measured concentrations of Ca, K, Mg, and Na corresponded well to those reported for other juices of the same kind.1,11,12,69,70 Apparently, K was the most abundant mineral in all analyzed juices. Its concentration varied in the range of 0.5–2 g L−1, depending on the juice type. The content of Mg was roughly similar in the analyzed juices and changed in the 30–125 mg L−1 range. The concentrations of Ca and Na were the most diversified as they ranged within 18–259 and 6–2250 mg L−1, respectively. Nevertheless, in the analyzed juices of different types, a very favorable K-to-Na concentration ratio was observed, ranging from 13 to 145. The exception was tomato (TT) juice, for which these concentrations were similar, which indicates that the juice is salted. Also, the Ca-to-Na concentration ratio was similar and ranged from 2.6 to 4.2 with the exception of tomato (TT), pomegranate (PH), and quince (QK) juices.
As regards the results received for the FLC APGD OES measurements, the concentrations of K and Na were consistent with the results obtained from ICP OES. The recoveries of those elements varied in the 96–111% range, confirming quite good accuracy of the developed FLC APGD method. Unfortunately, the results received for Ca and Mg were lesser. In the case of Ca, good accuracy (91–109%) was obtained for half of the analyzed juices, i.e., apple (AP), blackcurrant (CF), lemon (LE), and quince (QK). However, for the other juices, the recoveries either changed in the 70–79% range (lime (ME), pomegranate (PH), and tomato (TT)) or was equal to 157% (banana (BX)). Even worse results were obtained for Mg, which recoveries changed in the range of 48–57% for all analyzed juices. These results were quite unexpected, bearing in mind high sample dilution and the apparent lack of matrix effects described above. Nevertheless, the obtained recovery values for Ca and (especially) Mg may be explained assuming that in all those juices there are organic compounds present, i.e., carboxylic acids, at high concentrations, that forms complexes with those metals. In such a case, the relationship between the signal intensity of their analytical lines and the juice percentage concentration would be still linear as the dilution does not change the analyte to interfering compounds concentrations ratio. Due to high variety of organic compounds existing in juices in very high abundances,68,71,72 it is difficult to designate a particular compound being responsible for the signal intensity decline, unambiguously. Considering the recovery values for Ca and Mg, it can be stated, however, that these compounds form stronger complexes rather with Mg than Ca.
Nevertheless, due to the unsatisfactory recovery values obtained for Ca and – especially – Mg, the determination of those two metals was repeated using the standard addition method for calibration. The results are presented in Table 4. The recoveries received for the standard addition method were fallen in the range of 91–109% and confirmed very good accuracy of the applied method.
Regarding the precision of results obtained from those two calibration methods for FLC APGD OES measurements, expressed as the RSD from 3 independently repeated analyses, it can be seen that it was very good as usually did not exceeded 5%. The RSD values ranged within 0.10–2.97% for Ca, 0.43–1.45% for K, 0.78–4.48% for Mg, and 0.66–2.38% for Na (the calibration curve with simple standard solutions method) as well as 0.68–5.41% for Ca and 0.22–3.48% for Mg (the calibration with the standard addition method). Higher RSD values (i.e., 5.40 and 5.41%) were obtained only for Ca and only in juices containing relatively small (in regard to the sensitivity of its analytical line) amounts of Ca after 1000-dilution, which resulted in quite small signals. It is also noteworthy that even worse RSD values (i.e., 5.67 and 8.60%) were obtained for some of the ICP OES measurements, likely owing to the digestion step which was required for this method to obtain reliable results.
4. Conclusions
It was demonstrated that the proposed FLC APGD OES method provides reliable results for the determination of Ca, K, Na, and Mg in different kinds of juices. The precision of the obtained results was comparable to the one obtained from the ICP OES measurements. The presented simplified method requires only sample dilution and acidification, completely omitting the wet digesting, which is time-consuming process and requires the use of concentrated acids (HNO3, HCl, HF, HClO4) as well as H2O2, a high amount of energy, and it usually takes a couple of hours. There is also the possibility of sample contamination, during this step. Moreover, the use of the proposed FLC APGD OES method is associated with all advantages regarding techniques being premised on microplasma excitation sources. As opposed to the commercially applied spectrometric techniques, the FLC APGD OES method offers the advantages of small size, no requirement of the use of noble gases, and stable operation at low electric current power consumption, which is particularly important and useful for routine analyses of juices with very different chemical matrices. All of this translates to significantly reduced operating costs. Taking all of this into account, it may be concluded that the proposed method is a reliable alternative for commercially applied bulky instrumentation, e.g., AAS or ICP OES. The only disadvantage of the developed method is the necessity of the standard addition method for calibration for the determination of Mg and Ca, due to the matrix effects, which cannot be overcome by the sample dilution. We believe, this finding is relevant as it will be true also for the juices determination by other spectrometric methods, e.g., ICP-OES, ICP-MS, AAS, AFS. Nevertheless, the standard addition method is still less time-consuming than performing wet digestion. Moreover, due to simple design and miniaturization of the proposed FLC-APGD system, it might be automated by coupling it with a diluter and/or a mixer (for the standard addition method), making the analysis even simpler and faster.
Conflicts of interest
There are no conflicts to declare.
Acknowledgements
Monika Gorska and Pawel Pohl acknowledge the financial support from Polish National Science Center (2019/33/B/ST4/00356).
References
- A. Szymczycha-Madeja, M. Welna, D. Jedryczko and P. Pohl, Developments and strategies in the spectrochemical elemental analysis of fruit juices, Trends Anal. Chem., 2014, 55, 68–80 Search PubMed.
- F. Demir, A. Seyhun Kipcak, O. Dere Ozdemir and E. Moroydor Derun, Determination of essential and non-essential element concentrations and health risk assessment of some commercial fruit juices in Turkey, J. Food Sci. Technol., 2020, 57, 4432–4442 Search PubMed.
- P. Pohl, A revisited FAAS method for very simple and fast determination of total concentrations of Cu, Fe, Mn and Zn in grape juices with sample preparation developed by modeling experimental design and optimization, Microchem. J., 2020, 157, 104998 Search PubMed.
- N. Jalbani, F. Ahmed, T. Gul Kazi, U. Rashid, A. Bano Munshi and A. Kandhro, Determination of essential elements (Cu, Fe and Zn) in juices of commercially available in Pakistan, Food Chem. Toxicol., 2010, 48, 2737–2740 Search PubMed.
- I. Maia Toaldo, F. Alves Cruz, T. de Lima Alves, J. Santos de Gois, D. L. G. Borges, H. Pamplona Cunha, E. Luiz Da Silva and M. T. Bordignon-Luiz, Bioactive potential of Vitis labrusca L. grape juices from the Southern Region of Brazil: phenolic and elemental composition and effect on lipid peroxidation in healthy subjects, Food Chem., 2015, 173, 527–535 Search PubMed.
-
B. K. Tiwari and G. Rajauria, Fruit Juices, Academic Press, London, 2018 Search PubMed.
- M. M. Ghuniem, M. A. Korshed and E. R. Souaya, Optimization and Validation of an Analytical Method for the Determination of Some Trace and Toxic Elements in Canned Fruit Juices Using Quadrupole Inductively Coupled Plasma Mass Spectrometer, J. AOAC Int., 2018, 262–270 Search PubMed.
- M. Conceicao Prudencio Da Dutra, L. Leonice Rodrigues, D. de Oliveira, G. Elias Pereira and M. Dos Santos Lima, Integrated analyses of phenolic compounds and minerals of Brazilian organic and conventional grape juices and wines: validation of a method for determination of Cu, Fe and Mn, Food Chem., 2018, 269, 157–165 Search PubMed.
- V. Luiz Cordoba Braganca, P. Melnikov and L. Z. Zanoni, Trace elements in fruit juices, Biol. Trace Elem. Res., 2012, 146, 256–261 Search PubMed.
- A. Paiva Oliveira, J. Anchieta Gomes Neto, J. Araujo Nobrega, P. Rogerio Miranda Correia and P. Vitoriano Oliveira, Determination of selenium in nutritionally relevant foods by graphite furnace atomic absorption spectrometry using arsenic as internal standard, Food Chem., 2005, 93, 355–360 Search PubMed.
- I. Juranovic Cindric, M. Zeiner, M. Kroppl and G. Stingeder, Comparison of sample preparation methods for the ICP-AES determination of minor and major elements in clarified apple juices, Microchem. J., 2011, 99, 364–369 Search PubMed.
- A. Szymczycha-Madeja and M. Welna, Evaluation of a simple and fast method for the multi-elemental analysis in commercial fruit juice samples using atomic emission spectrometry, Food Chem., 2013, 141, 3466–3472 Search PubMed.
- I. Cristina Da Silva Haas, I. Maia Toaldo, T. Margarida Gomes, A. S. Luna, J. Santos de Gois and M. T. Bordignon-Luiz, Polyphenolic profile, macro- and microelements in bioaccessible fractions of grape juice sediment using in vitro gastrointestinal simulation, Food Biosci., 2019, 27, 66–74 Search PubMed.
- A. Cristina Silva de Lima, D. Josino Soares, L. Morais Ribeiro Da Silva, R. Wilane de Figueiredo, P. Henrique Machado de Sousa and E. de Abreu Menezes, In vitro bioaccessibility of copper, iron, zinc and antioxidant compounds of whole cashew apple juice and cashew apple fibre (Anacardium occidentale L.) following simulated gastro-intestinal digestion, Food Chem., 2014, 161, 142–147 Search PubMed.
- L. Tormen, D. Placido Torres, I. Maria Dittert, R. G. O. Araujo, V. L. A. Frescura and A. Jose Curtius, Rapid assessment of metal contamination in commercial fruit juices by inductively coupled mass spectrometry after a simple dilution, J. Food Compos. Anal., 2011, 24, 95–102 Search PubMed.
- G. Cristea, A. Dehelean, C. Voica, I. Feher, R. Puscas and D. Alina Magdas, Isotopic and Elemental Analysis of Apple and Orange Juice by Isotope Ratio Mass Spectrometry (IRMS) and Inductively Coupled Plasma-Mass Spectrometry (ICP-MS), Anal. Lett., 2020, 1–15 Search PubMed.
- S. Millour, L. Noel, A. Kadar, R. Chekri, C. Vastel and T. Guerin, Simultaneous analysis of 21 elements in foodstuffs by ICP-MS after closed-vessel microwave digestion: method validation, J. Food Compos. Anal., 2011, 24, 111–120 Search PubMed.
- W. A. Simpkins, H. Louie, M. Wu, M. Harrison and D. Goldberg, Trace elements in Australian orange juice and other products, Food Chem., 2000, 71, 423–433 Search PubMed.
- P. Pohl, P. Jamroz, K. Swiderski, A. Dzimitrowicz and A. Lesniewicz, Critical evaluation of recent achievements in low power glow discharge generated at atmospheric pressure between a flowing liquid cathode and a metallic anode for element analysis by optical emission spectrometry, Trends Anal. Chem., 2017, 88, 119–133 Search PubMed.
- X. Peng and Z. Wang, Ultrasensitive Determination of Selenium and Arsenic by Modified Helium Atmospheric Pressure Glow Discharge Optical Emission Spectrometry Coupled with Hydride Generation, Anal. Chem., 2019, 91, 10073–10080 Search PubMed.
- M. Gorska, K. Greda and P. Pohl, On the coupling of hydride generation (HG) with flowing liquid anode atmospheric pressure glow discharge (FLA-APGD) for determination of traces of As, Bi, Hg, Sb and Se by optical emission spectrometry (OES), Talanta, 2021, 222, 121510 Search PubMed.
- V. V. Yagov, M. L. Getsina and B. K. Zuev, Use of Electrolyte Jet Cathode Glow Discharges as Sources of Emission Spectra for Atomic Emission Detectors in Flow-Injection Analysis, J. Anal. Chem., 2004, 59, 1037–1041 Search PubMed.
- B. K. Zuev, V. V. Yagov, M. L. Getsina and B. A. Rudenko, Discharge on Boiling in a Channel as a New Atomization and Excitation Source for the Flow Determination of Metals by Atomic Emission Spectrometry, J. Anal. Chem., 2002, 57, 907–911 Search PubMed.
- A. Kitano, A. Iiduka, T. Yamamoto, Y. Ukita, E. Tamiya and Y. Takamura, Highly sensitive elemental analysis for Cd and Pb by liquid electrode plasma atomic emission spectrometry with quartz glass chip and sample flow, Anal. Chem., 2011, 83, 9424–9430 Search PubMed.
- T. Cserfalvi, P. Mezei and P. Apai, Emission studies on a glow discharge in atmospheric pressure air using water as a cathode, J. Phys. D: Appl. Phys., 1993, 26, 2184–2188 Search PubMed.
- M. R. Webb, F. J. Andrade, G. Gamez, R. McCrindle and G. M. Hieftje, Spectroscopic and electrical studies of a solution-cathode glow discharge, J. Anal. At. Spectrom., 2005, 20, 1218–1225 Search PubMed.
- P. Jamroz and W. Zyrnicki, Spectroscopic Characterization of Miniaturized Atmospheric-Pressure dc Glow Discharge Generated in Contact with Flowing Small Size Liquid Cathode, Plasma Chem. Plasma Process., 2011, 31, 681–696 Search PubMed.
- H. W. Paing, K. A. Hall and R. Kenneth Marcus, Sheathing of the liquid sampling – atmospheric pressure glow discharge microplasma from ambient atmosphere and its implications for optical emission spectroscopy, Spectrochim. Acta, Part B, 2019, 155, 99–106 Search PubMed.
- X. Liu, Z. Zhu, D. He, H. Zheng, Y. Gan, N. Stanley Belshaw, S. Hu and Y. Wang, Highly sensitive elemental analysis of Cd and Zn by solution anode glow discharge atomic emission spectrometry, J. Anal. At. Spectrom., 2016, 31, 1089–1096 Search PubMed.
- K. Greda, K. Swiderski, P. Jamroz and P. Pohl, Flowing Liquid Anode Atmospheric Pressure Glow Discharge as an Excitation Source for Optical Emission Spectrometry with the Improved Detectability of Ag, Cd, Hg, Pb, Tl, and Zn, Anal. Chem., 2016, 88, 8812–8820 Search PubMed.
- P. Jamroz, K. Greda, A. Dzimitrowicz, K. Swiderski and P. Pohl, Sensitive Determination of Cd in Small-Volume Samples by Miniaturized Liquid Drop Anode Atmospheric Pressure Glow Discharge Optical Emission Spectrometry, Anal. Chem., 2017, 89, 5729–5733 Search PubMed.
- K. Swiderski, P. Pohl and P. Jamroz, A miniaturized atmospheric pressure glow microdischarge system generated in contact with a hanging drop electrode – a new approach to spectrochemical analysis of liquid microsamples, J. Anal. At. Spectrom., 2019, 34, 1287–1293 Search PubMed.
- K. Greda, M. Gorska, M. Welna, P. Jamroz and P. Pohl, In-situ generation of Ag, Cd, Hg, In, Pb, Tl and Zn volatile species by flowing liquid anode atmospheric pressure glow discharge operated in gaseous jet mode – evaluation of excitation processes and analytical performance, Talanta, 2019, 199, 107–115 Search PubMed.
- K. Greda, A. Szymczycha-Madeja and P. Pohl, Study and reduction of matrix effects in flowing liquid anode – atmospheric pressure glow discharge – optical emission spectrometry, Anal. Chim. Acta, 2020, 1123, 81–90 Search PubMed.
- M. Gorska, K. Greda and P. Pohl, Determination of bismuth by optical emission spectrometry with liquid anode/cathode atmospheric pressure glow discharge, J. Anal. At. Spectrom., 2021, 36, 165–177 Search PubMed.
- X. Liu, Z. Liu, Z. Zhu, D. He, S. Yao, H. Zheng and S. Hu, Generation of Volatile Cadmium and Zinc Species Based on Solution Anode Glow Discharge Induced Plasma Electrochemical Processes, Anal. Chem., 2017, 89, 3739–3746 Search PubMed.
- M. Yuan, X. Peng, F. Ge, Q. Li, K. Wang, D.-G. Yu and Z. Wang, Simplified design for solution anode glow discharge atomic emission spectrometry device for highly sensitive detection of Ag, Bi, Cd, Hg, Pb, Tl, and Zn, Microchem. J., 2020, 155, 104785 Search PubMed.
- J. Yu, L. Yin, Q. Lu, F. Feng, Y. Kang and H. Luo, Highly sensitive determination of mercury by improved liquid cathode glow discharge with the addition of chemical modifiers, Anal. Chim. Acta, 2020, 1131, 25–34 Search PubMed.
- Q. Lu, F. Feng, J. Yu, L. Yin, Y. Kang, H. Luo, D. Sun and W. Yang, Determination of trace cadmium in zinc concentrate by liquid cathode glow discharge with a modified sampling system and addition of chemical modifiers for improved sensitivity, Microchem. J., 2020, 152, 104308 Search PubMed.
- J. Yu, X. Zhang, Q. Lu, X. Wang, D. Sun, Y. Wang and W. Yang, Determination of calcium and zinc in gluconates oral solution and blood samples by liquid cathode glow discharge-atomic emission spectrometry, Talanta, 2017, 175, 150–157 Search PubMed.
- W. Zu, Y. Yang, Y. Wang, X. Yang, C. Liu and M. Ren, Rapid determination of indium in water samples using a portable solution cathode glow discharge-atomic emission spectrometer, Microchem. J., 2018, 137, 266–271 Search PubMed.
- R. Shekhar, Improvement of sensitivity of electrolyte cathode discharge atomic emission spectrometry (ELCAD-AES) for mercury using acetic acid medium, Talanta, 2012, 93, 32–36 Search PubMed.
- R. Shekhar, K. Madhavi, N. N. Meeravali and S. Jai Kumar, Determination of thallium at trace levels by electrolyte cathode discharge atomic emission spectrometry with improved sensitivity, Anal. Methods, 2014, 6, 732–740 Search PubMed.
- Z. Zhang, Z. Wang, Q. Li, H. Zou and Y. Shi, Determination of trace heavy metals in environmental and biological samples by solution cathode glow discharge-atomic emission spectrometry and addition of ionic surfactants for improved sensitivity, Talanta, 2014, 119, 613–619 Search PubMed.
- J. Yu, S. Yang, Q. Lu, D. Sun, J. Zheng, X. Zhang, X. Wang and W. Yang, Evaluation of liquid cathode glow discharge-atomic emission spectrometry for determination of copper and lead in ores samples, Talanta, 2017, 164, 216–221 Search PubMed.
- K. Greda, P. Jamroz and P. Pohl, The improvement of the analytical performance of direct current atmospheric pressure glow discharge generated in contact with the small-sized liquid cathode after the addition of non-ionic surfactants to electrolyte solutions, Talanta, 2013, 108, 74–82 Search PubMed.
- C. Huang, Q. Li, J. Mo and Z. Wang, Ultratrace Determination of Tin, Germanium, and Selenium by Hydride Generation Coupled with a Novel Solution-Cathode Glow Discharge-Atomic Emission Spectrometry Method, Anal. Chem., 2016, 88, 11559–11567 Search PubMed.
- P. Jamroz, P. Pohl and W. Zyrnicki, An analytical performance of atmospheric pressure glow discharge generated in contact with flowing small size liquid cathode, J. Anal. At. Spectrom., 2012, 27, 1032–1037 Search PubMed.
- Q. Xiao, Z. Zhu, H. Zheng, H. He, C. Huang and S. Hu, Significant sensitivity improvement of alternating current driven-liquid discharge by using formic acid medium for optical determination of elements, Talanta, 2013, 106, 144–149 Search PubMed.
- J. Mo, L. Zhou, X. Li, Q. Li, L. Wang and Z. Wang, On-line separation and pre-concentration on a mesoporous silica-grafted graphene oxide adsorbent coupled with solution cathode glow discharge-atomic emission spectrometry for the determination of lead, Microchem. J., 2017, 130, 353–359 Search PubMed.
- C. Yang, L. Wang, Z. Zhu, L. Jin, H. Zheng, N. Stanley Belshaw and S. Hu, Evaluation of flow injection-solution cathode glow discharge-atomic emission spectrometry for the determination of major elements in brines, Talanta, 2016, 155, 314–320 Search PubMed.
- K. Greda, P. Jamroz, A. Dzimitrowicz and P. Pohl, Direct elemental analysis of honeys by atmospheric pressure glow discharge generated in contact with a flowing liquid cathode, J. Anal. At. Spectrom., 2015, 30, 154–161 Search PubMed.
- J. Wang, P. Tang, P. Zheng and X. Zhai, Analysis of metal elements by solution cathode glow discharge-atomic emission spectrometry with a modified pulsation damper, J. Anal. At. Spectrom., 2017, 32, 1925–1931 Search PubMed.
- P. Zheng, X. Zhai, J. Wang and P. Tang, Analytical Characterization of a Solution Cathode Glow Discharge with an Interference Filter Wheel for Spectral Discrimination, Anal. Lett., 2018, 51, 2304–2315 Search PubMed.
- H. Yuan, D.-Z. Yang, X. Li, L. Zhang, X.-F. Zhou, W.-C. Wang and Y. Xu, A pulsed electrolyte cathode discharge used for metal element analysis by atomic emission spectrometry, Phys. Plasmas, 2019, 26, 53505 Search PubMed.
- P. Zheng, Y. Gong, J. Wang and X. Zeng, Elemental Analysis of Mineral Water by Solution-Cathode Glow Discharge–Atomic Emission Spectrometry, Anal. Lett., 2017, 50, 1512–1520 Search PubMed.
- P. Zheng, Y. Chen, J. Wang and S. Xue, A pulsed atmospheric-pressure discharge generated in contact with flowing electrolyte solutions for metal element analysis by optical emission spectrometry, J. Anal. At. Spectrom., 2016, 31, 2037–2044 Search PubMed.
- P. Pohl, P. Jamroz, K. Greda, M. Gorska, A. Dzimitrowicz, M. Welna and A. Szymczycha-Madeja, Five years of innovations in development of glow discharges generated in contact with liquids for spectrochemical elemental analysis by optical emission spectrometry, Anal. Chim. Acta, 2021, 338399 Search PubMed.
- P. Pohl, H. Stecka and P. Jamroz, Solid phase extraction with flame atomic absorption spectrometry for determination of traces of Ca, K, Mg and Na in quality control of white sugar, Food Chem., 2012, 130, 441–446 Search PubMed.
- A. Szymczycha-Madeja, M. Welna and P. Pohl, Determination of essential and non-essential elements in green and black teas by FAAS and ICP OES simplified – multivariate classification of different tea products, Microchem. J., 2015, 121, 122–129 Search PubMed.
- P. Pohl, M. Kalinka and M. Pieprz, Development of a very simple and fast analytical methodology for FAAS/FAES measurements of Ca, K, Mg and Na in red beetroot juices along with chemical fractionation of Ca and Mg by solid phase extraction, Microchem. J., 2019, 147, 538–544 Search PubMed.
- S. Ruella de Oliveira, J. Luiz Raposo and J. Anchieta Gomes Neto, Fast sequential multi-element determination of Ca, Mg, K, Cu, Fe, Mn and Zn for foliar diagnosis using high-resolution continuum source flame atomic absorption spectrometry: Feasibility of secondary lines, side pixel registration and least-squares background correction, Spectrochim. Acta, Part B, 2009, 64, 593–596 Search PubMed.
- R. Martins de Souza, L. Gustavo Leocadio and C. L. P. Da Silveira, ICP OES Simultaneous Determination of Ca, Cu, Fe, Mg, Mn, Na, and P in Biodiesel by Axial and Radial Inductively Coupled Plasma-Optical Emission Spectrometry, Anal. Lett., 2008, 41, 1615–1622 Search PubMed.
- H. M. Santos, J. P. Coutinho, F. A. C. Amorim, I. P. Lobo, L. S. Moreira, M. M. Nascimento and R. M. de Jesus, Microwave-assisted digestion using diluted HNO3 and H2O2 for macro and microelements determination in guarana samples by ICP OES, Food Chem., 2019, 273, 159–165 Search PubMed.
- A. Vallverdu-Queralt, A. Medina-Remon, I. Casals-Ribes and R. M. Lamuela-Raventos, Is there any difference between the phenolic content of organic and conventional tomato juices?, Food Chem., 2012, 130, 222–227 Search PubMed.
- G. R. Schmutzer, A. Dehelean, D. Alina Magdas, G. Cristea and C. Voica, Determination of Stable Isotopes, Minerals, and Volatile Organic Compounds in Romanian Orange Juice, Anal. Lett., 2016, 49, 2644–2658 Search PubMed.
- S. Medina, R. Perestrelo, R. Santos, R. Pereira and J. S. Camara, Differential volatile organic compounds signatures of apple juices from Madeira Island according to variety and geographical origin, Microchem. J., 2019, 150, 104094 Search PubMed.
- M. Dos Santos Lima, I. de Souza Veras Silani, I. Maia Toaldo, L. Claudio Correa, A. Camarao Telles Biasoto, G. Elias Pereira, M. T. Bordignon-Luiz and J. Luiz Ninow, Phenolic compounds, organic acids and antioxidant activity of grape juices produced from new Brazilian varieties planted in the Northeast Region of Brazil, Food Chem., 2014, 161, 94–103 Search PubMed.
- P. Pohl, A. Dzimitrowicz, P. Jamroz and K. Greda, Development and optimization of simplified method of fast sequential HR-CS-FAAS analysis of apple juices on the content of Ca, Fe, K, Mg, Mn and Na with the aid of response surface methodology, Talanta, 2018, 189, 182–189 Search PubMed.
- M. Dzugan, M. Wesolowska, G. Zagula and C. Puchalski, The comparison of the physicochemical parameters and antioxidant activity of homemade and commercial pomegranate juices, Acta Sci. Pol., Technol. Aliment., 2018, 17, 59–68 Search PubMed.
- J. Li, C. Zhang, H. Liu, J. Liu and Z. Jiao, Profiles of Sugar and Organic Acid of Fruit Juices: A Comparative Study and Implication for Authentication, J. Food Qual., 2020, 2020, 1–11 Search PubMed.
- C. Dani, L. S. Oliboni, R. Vanderlinde, D. Bonatto, M. Salvador and J. A. P. Henriques, Phenolic content and antioxidant activities of white and purple juices manufactured with organically- or conventionally-produced grapes, Food Chem. Toxicol., 2007, 45, 2574–2580 Search PubMed.
Footnote |
† Electronic supplementary information (ESI) available. See DOI: 10.1039/d1ja00127b |
|
This journal is © The Royal Society of Chemistry 2021 |