DOI:
10.1039/D0GC03254A
(Paper)
Green Chem., 2021,
23, 526-535
Pd(II)-Catalyzed [4 + 1 + 1] cycloaddition of simple o-aminobenzoic acids, CO and amines: direct and versatile synthesis of diverse N-substituted quinazoline-2,4(1H,3H)-diones†
Received
25th September 2020
, Accepted 2nd December 2020
First published on 10th December 2020
Abstract
The mild, efficient, and straightforward synthesis of pharmaceutically and biologically active N3-substituted and N1,N3-disubstituted quinazoline-2,4-(1H,3H)-diones from simple and readily available substrates has been a huge challenge. Described here is a Pd(II)-catalyzed [4 + 1 + 1] modular synthesis of diverse quinazoline-2,4-(1H,3H)-diones through one-pot cascade reactions of cyclocondensation of o-(alkyl)aminobenzoic acids with CO, amidation of the intermediate isatoic anhydrides with amines, and unprecedented carbonylation of the resulting o-aminobenzamides with CO under 1 atm and 60 °C conditions. The chemoselective and versatile multicomponent reaction allows for the diversities of the products including N3-substituted and N1,N3-disubstituted products, and even makes the substituents on N1,N3 the same or different from each other, which cannot be achieved by most traditional synthetic strategies.
Introduction
As an important class of heterocyclic compounds, N3-substituted and N1,N3-disubstituted quinazoline-2,4-(1H,3H)-dione derivatives have drawn much attention due to their various pharmacological and biological activities, such as antihypertensive,1 antipsychotic,1a anticancer,2 antitumor,3 anticonvulsant,4 antidiabetic,5 antiviral,6 herbicidal,7 antibacterial,8 antimicrobial,9 and antifungal10 properties and chitin synthase,10,11 cytosolic phospholipase A2,12 and thromboxane synthetase13 inhibitory activities. Examples shown in Fig. 1 are typical representatives of commercial drugs or biologically active compounds of N3-substituted and N1,N3-disubstituted quinazoline-2,4-(1H,3H)-diones.1a,14
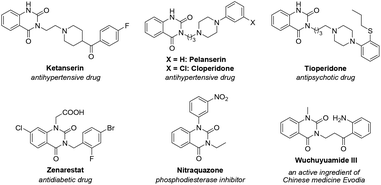 |
| Fig. 1 Representative examples of biologically active N3-substituted and N1,N3-disubstituted quinazoline-2,4(1H,3H)-diones. | |
Owing to their immense and vital biological activities, substantial efforts have been dedicated to their synthetic protocols. Although construction and then selective monoalkylation of the unsubstituted quinazoline-2,4-(1H,3H)-dione core on the N3- or on N1- and N3-positions with differential substituents can achieve this goal,5b,15 this strategy is extremely limited owing to multistep synthesis, low overall efficiency and low yields due to the lack of regioselectivity between the two nitrogens, leading to a mixture of N-mono- and dialkylated products5b,14b,16 or low atom economy due to the lengthy and special process.5b,15,16a,17 As a consequence, much attention has been paid to the construction of quinazoline-2,4-(1H,3H)-diones directly with mono substituents embedded in the N3-position and differential substituents embedded in N1- and N3-positions from the beginning rather than selective alkylation of the parent quinazolinedione. Among them, the approaches based on o-(alkyl)aminobenzoic acids or their derivatives are commonly used to synthesize such compounds (Scheme 1), including (1) the reaction of isatoic anhydrides with aryl isocyanates18 (Route A, which can be generated from simple and easily obtainable o-aminobenzoic acid via a cyclocondensation reaction19 with phosgene or its complex derivatives (ethyl chloroformate, di-tert-butyl dicarbonate or triphosgene) or CO); (2) cyclocarbonylation of o-aminobenzamides (Route B, which can be generated from amidation of o-aminobenzoic acids12,20 or aminolysis of isatoic anhydrides21) with carbonylation reagents such as phosgene or its complex derivatives14a,20b,22 (carbonyl diimidazole, ethyl chloroformate, di-tert-butyl dicarbonate or triphosgene) or pressurized CO (about 30 atm);23 (3) addition of o-aminobenzoic acids or their esters to phenyl isocyanates and then cyclization of the resulting urea derivatives in situ or stepwise (Route C);7b,8a,24 (4) N-carbalkoxylation of o-aminobenzoic acids with chloroformate or carbonate, followed by amidation and cyclization strategies (Route D).21b,25 In addition, cyclocarbonylation of o-nitrobenzamides26 with triphosgene or pressurized CO (30 atm), Pd-catalyzed insertion and cycloaddition of CO2 and tertiary alkyl isocyanide into o-haloanilines14b,27 or cyclocarbonylation of aryl ureas generated from the addition of o-haloanilines to isocyanates28 and cyclization of o-halobenzoyl ureas16a,29 are also reported in the literature for this purpose. Despite the above important progress, these methods suffer from some major drawbacks to some extent, such as complex, expensive and inaccessible starting materials; special or highly toxic reagents like aryl isocyanate, tertiary alkyl isocyanide and phosgene; harsh reaction conditions such as high CO or CO2 pressure and/or reaction temperature, anhydrous conditions and/or nitrogen atmosphere; long reaction time; multistep and tedious workup procedures; low atom and/or step economy; low overall reaction efficiency and/or (total) yields. Moreover, the approaches based on o-nitrobenzamides and o-haloanilines can only be applied to the preparation of N3-substituted rather than N1,N3-disubstituted quinazoline-2,4-(1H,3H)-diones. Therefore, the development of more efficient, facile, straightforward, and cost-effective processes for the versatile synthesis of N-substituted quinazoline-2,4(1H,3H)-diones would be highly desirable.
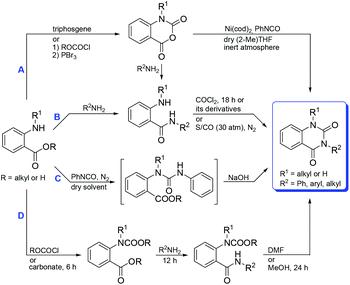 |
| Scheme 1 Approaches to N-substituted quinazoline-2,4-(1H,3H)-diones based on o-(alkyl)aminobenzoic acids or their derivatives. | |
Palladium-catalyzed carbonylation reactions can serve as effective tools for the synthesis of heterocyclic-containing carbonyl moieties,28b,30 while cascade reactions have emerged as a powerful and environmentally friendly tool for the rapid assembly of complex molecules from simple and readily available starting materials in a one-pot manner with significant advantages such as high efficiency, chemo-/regio-/stereoselectivity, atom/step economy and low time, energy and solvent consumption as well as low waste generation.31 Since N-substituted quinazoline-2,4(1H,3H)-diones could be derived from o-aminobenzoic acids via cyclocondensation (Scheme 2A), amidation (Scheme 2B) and cyclocarbonylation (Scheme 2C) in a stepwise sequence, an intriguing question is whether simple and easily obtainable o-(alkyl)aminobenzoic acids, CO and amines can be used as the substrates for the direct and versatile synthesis of such important compounds via Pd-catalyzed [4 + 1 + 1] cascade cycloaddition reactions under mild conditions (Scheme 2). This proposed protocol, combining the advantages of Pd-catalyzed carbonylation and cascade reactions, to the best of our knowledge, has not been explored to date, and would provide a completely new facile, green, efficient, versatile and cost-effective access to these target compounds with high atom/step economy if it works. To realize this assumption, however, there are at least two major challenges that should be overcome: (1) an appropriate catalytic system for efficiently activating CO should be explored to promote the cyclocarbonylation of o-aminobenzamides at atmospheric pressure, which, as far as we know, has not been achieved so far; (2) the chemoselectivity has to be controlled to inhibit the formation of symmetrical/unsymmetrical ureas and amides via self-carbonylation of amines and cross carbonylation/condensation of amines with o-aminobenzoic acids during the reaction process. Herein, we present the first example of direct and versatile synthesis of N-substituted quinazoline-2,4(1H,3H)-diones from Pd(II)-catalyzed [4 + 1 + 1] cascade cycloaddition of simple o-(alkyl)aminobenzoic acids with CO and amines under mild conditions (Scheme 2). The present novel protocol has several significant advantages: (1) this is the first example of direct synthesis of N-substituted quinazoline-2,4(1H,3H)-diones from readily available o-(alkyl)aminobenzoic acids with simple CO and amines under very mild conditions. The chemoselective and multicomponent cascade reaction characteristics enable more product diversity and even make the substituents on N1,N3 the same or different from each other, which can't be achieved by most traditional synthetic strategies; (2) an unprecedented carbonylation of o-aminobenzamides with atmospheric pressure CO under mild conditions is disclosed for the first time, which may open a new prospect for the discovery of new methodologies in CO chemistry; (3) the broad substrate scope, mild reaction conditions, operational simplicity and high step economy make this Pd-catalyzed [4 + 1 + 1] protocol very simple, green, practical, and easy to handle.
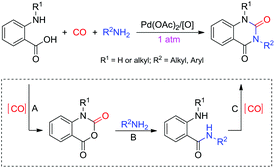 |
| Scheme 2 Pd(II)-Catalyzed [4 + 1 + 1] strategy for assembling N-substituted quinazoline-2,4(1H,3H)-diones. | |
Results and discussion
To test the feasibility of this Pd(II)-catalyzed one-pot strategy, the reaction of o-methylaminobenzoic acid (1b) with CO (bubble) and n-butylamine (2a) was initially investigated as a model reaction in acetonitrile with Cu(OAc)2/O2 as the oxidant and KI as the additive (Table 1). The essential roles of KI32 here are generally believed to promote the reactivity and selectivity of the palladium catalyst most probably due to its two functions; one is that it can act as a stabilizer of low oxidation state Pd(II) to prevent it from decomposing and depositing metal palladium, and the other is that it may also act as a promoter by making the palladium catalyst more nucleophilic through its complexation with the catalyst. In order to promote the amination of isatoic anhydride and inhibit the formation of symmetrical/unsymmetrical ureas and amides during the reaction process, another additive AcOH was chosen as the promoter and added to the reaction system together with 2a after the formation of isatoic anhydride (about 6 h after the reaction started). To our delight, after the reaction proceeded at 30 °C for 10 h, the target product 3-butyl-1-methylquinazoline-2,4(1H,3H)-dione (3-1b) could be obtained in 53% yield (Table 1, entry 1) along with a certain amount of the by-product 1,3-dibutylurea and a small amount of isatoic anhydride and N-butyl-o-methylaminobenzamide. The conversion rate of 1b and the yield of 3-1b increased accordingly with the increase of the reaction temperature and reached the maximum at 60 °C (Table 1, entries 2 and 3). As expected, when the reaction was carried out by adding a mixture of 2a and AcOH dropwise, a lower product yield was obtained due to the enhanced competitive self-carbonylation of 2a in a low concentration of isatoic anhydride (Table 1, entry 5). Next, to further simplify this protocol, the reaction efficiency was tested in the absence of O2 and AcOH and the results were very gratifying (Table 1, entries 6 and 7), indicating that neither AcOH nor O2 was indispensable in this model reaction. Finally, a screening of several common solvents including acetonitrile, toluene, DMSO, DMF and 1,4-dioxane revealed that only acetonitrile was optimal (Table 1, entries 7–11).
Table 1 Optimization of the reaction conditionsa
With the optimal conditions in hand (Table 1, entry 7), we firstly evaluated the scope of o-aminobenzoic acids 1 (Table 2). To our delight, the reaction of o-aminobenzoic acid 1a could proceed smoothly to afford the corresponding free (N1–H) N3-substituted product 3-1a in 80% yield, indicating that N3-substituted quinazoline-2,4(1H,3H)-diones could be easily prepared with o-aminobenzoic acids through this protocol. Correspondingly, when the reaction proceeded with N-substituted o-alkylaminobenzoic acids, N1,N3-disubstituted quinazoline-2,4(1H,3H)-diones could be obtained expediently. As shown in Table 2, most of the o-alkylaminobenzoic acids smoothly underwent this protocol and gave the expected products in good to excellent yields (75%–90%). The substrates bearing less bulky groups on the amino group, such as methyl or ethyl (1b and 1c), showed a little higher reactivity than those bearing the bulky ones such as the isopropyl group (1d), whereas the N-phenyl o-aminobenzoic acid (1e) was not reactive in this reaction. Both the site and electronic nature of the electron-donating or the weakly electron-withdrawing substituents on the benzene ring of the o-methylaminobenzoic acids (1f–1l) did not exert considerable influence on the reaction performance and provided satisfactory yields (75%–89%). However, when the o-methylaminobenzoic acids bearing strong electron-withdrawing substituents (1m–1p) were applied in this protocol, poor results were obtained due to their low reactivity (3-1m–3-1p).
Table 2 Scope of o-(alkyl)aminobenzoic acidsa
Reaction conditions: 1 (1.0 mmol), 2a (3.0 mmol), Cu(OAc)2 (1.0 mmol), KI (0.2 mmol), CH3CN (10 mL); 2a was added 6 h later. Isolated yields.
AcOH (5.0 mmol) was added along with 2a 6 h later, 30 h.
|
|
To further investigate the substrate generality, a series of amines 2 were also investigated. As shown in Table 3, this protocol showed good tolerance to a variety of aliphatic and aromatic amines, affording the desired products in moderate to excellent yields. Generally, compared with aromatic amines, aliphatic amines worked better in this system and gave the corresponding products (3-2b–3-2g) in higher yields ranging from 74% to 91%. To our pleasure, although AcOH was found to have no obvious effect on aliphatic amines in this strategy, it could promote the amination of isatoic anhydride with aromatic amines to ensure the smooth progress of subsequent cyclocarbonylation reaction. Therefore, AcOH was applied in this protocol when the reaction proceeded with aromatic amines. Generally, the steric hindrance of amines 2 influenced the reaction efficiency to some degree. Correspondingly, the reactions of amines with low steric hindrance gave higher yields than those with high hindrance (2b–evs.2f; 2hvs.2i, 2l and 2p). The electronic effect was also evaluated in this transformation. We were delighted to observe that the reaction could tolerate both moderate electron-donating substituents such as methyl and electron-withdrawing substituents such as Cl and Br attached on the benzene ring of the aromatic amines and gave the corresponding N1,N3-disubstituted quinazoline-2,4-(1H,3H)diones (3-2i–3-2o) in moderate to good yields (38%–78%). In addition, the efficiency of the substrate bearing the strong electron-withdrawing substituent such as 4-nitrobenzenamine was also tested and found that the result was not satisfactory, leading to the desired product 3-2q in only 9% yield. Subsequently, 1-naphthylamine was applied as a representative of polycyclic aromatic amines to assess its applicability; to our delight, we found that it could work well in this system (3-2r, 73%). Finally, the efficiency of heterocyclic aromatic amines such as benzo[d]thiazol-2-amine, thiazol-2-amine and pyridin-2-amine (2s–2u) was also evaluated and it was found that they were less active in this system. The yields of the target products (3-2s–3-2u) were still not satisfactory even if the reaction was extended to 30 h.
Reaction conditions: 1b (1.0 mmol), 2 (3.0 mmol), Cu(OAc)2 (1.0 mmol), KI (0.2 mmol), CH3CN (10 mL); 2 was added 6 h later. Isolated yields.
AcOH (5.0 mmol) was added along with 2 6 h later.
30 h.
|
|
In order to demonstrate its practical utility, the reported protocol has been applied to the synthesis of the antihypertensive drug Pelanserin and it was found that it can be obtained in 47% yield by conducting the Pd(II)-catalyzed cycloaddition reaction of o-aminobenzoic acid (1a) and 3-(4-phenylpiperazin-1-yl)propan-1-amine (2v) in the presence of CO in 17 h (Scheme 3).
 |
| Scheme 3 Application to the synthesis of Pelanserin. | |
As mentioned in the optimization section, a small amount of by-products isatoic anhydride and o-methylaminobenzamide was formed in this [4 + 1 + 1] cascade cycloaddition reaction. In order to verify their generation pathway and gain some insight into the mechanism of this cycloaddition reaction, the following experiments were carried out and the results are depicted in Scheme 4. Firstly, cyclocondensation of o-methylaminobenzoic acid with CO under standard reaction conditions could afford the intermediate N-methylisatoic anhydrides in 83% yield (Scheme 4a). Next, aminolysis of N-methylisatoic anhydride with N-butylamine could proceed efficiently in acetonitrile at 60 °C for 5 min, leading to the corresponding N-butyl-o-methylaminobenzamide in 97% yield (Scheme 4b). Subsequently, the unprecedented cyclocarbonylation of N-butyl-o-methylaminobenzamide with atmospheric pressure CO was successfully realized for the first time under standard conditions for 10 h and could give the desired 3-butyl-1-methylquinazoline-2,4(1H,3H)-dione in 90% yield (Scheme 4c). These results indicate that isatoic anhydride can be easily generated from the cyclocondensation of o-methylaminobenzoic acid with CO; then, it can be followed by aminolysis with amine to give the corresponding o-methylaminobenzamide, which can undergo cyclocarbonylation smoothly with CO to afford the desired N-substituted quinazoline-2,4-(1H,3H)-dione product.
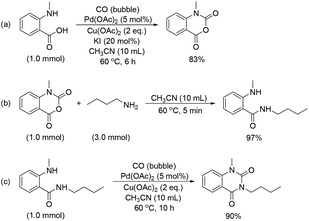 |
| Scheme 4 Mechanistic studies. | |
Based on the above experimental results and previous reports,32,33 a plausible pathway accounting for the formation of N-substituted quinazoline-2,4-(1H,3H)-dione is shown below (Scheme 5). First, Pd(OAc)2 is transformed into PdI2Ln in the presence of excess KI. Next, metathesis of o-alkylaminobenzoic acids 1 and PdI2Ln generates the intermediate I, which undergoes CO coordination and insertion to give intermediate II. Subsequently, reductive elimination of intermediate II affords the corresponding isatoic anhydride III and Pd0Ln. Then, the reaction of Pd0Ln with Cu(OAc)2 and KI to generate the PdI2Ln catalyst and isatoic anhydride III undergoes aminolysis with an amine to give o-alkylaminobenzamide IV. Finally, metathesis of amide intermediate IV and PdI2Ln, followed by CO insertion and nucleophilic attack of the amino group on the acylpalladium moiety of the intermediate VI, affords the desired N-substituted quinazoline-2,4-(1H,3H)-dione 3 and Pd0Ln; the latter is then converted to PdI2Ln once again in the presence of Cu(OAc)2 and KI for the next catalytic cycle.
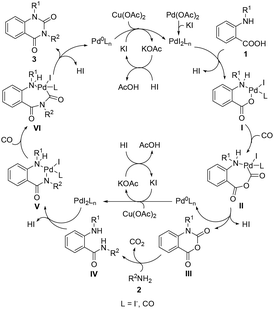 |
| Scheme 5 Proposed pathway for the synthesis of 3. | |
Conclusions
In summary, a direct and versatile approach to the synthesis of N-substituted quinazoline-2,4-(1H,3H)-diones from Pd(II)-catalyzed one-pot cascade reactions of o-(alkyl)aminobenzoic acids with CO and amines has been developed. This protocol can be applicable to a wide range of substrates and proceed efficiently under atmospheric pressure at 60 °C, affording the desired N3-substituted or N1,N3-disubstituted quinazoline-2,4(1H,3H)-diones expediently mostly in moderate to excellent yields. Simple and readily available substrates, mild reaction conditions, good product diversity, low costs, high step economy, high efficiency and simple manipulation render this one-pot reaction very convenient, green and practical.
Experimental section
General procedure for the synthesis of quinazoline-2,4(1H,3H)-diones
o-(Alkyl)aminobenzoic acid (1.0 mmol), Pd(OAc)2 (0.05 mmol), Cu(OAc)2 (1.0 mmol), KI (0.2 mmol) and CH3CN (10 mL) were charged in a 50 mL three-necked round-bottom flask equipped with a stir bar, a condenser and a T-branch pipe connected with a CO balloon. The flask was then put into an oil bath preheated to 60 °C, followed by stirring with continuous bubbling of CO. Upon the complete conversion of o-(alkyl)aminobenzoic acid, an amine (3.0 mmol) [along with AcOH (5.0 mmol) when aromatic amine was applied] was added into the reaction solution and the reaction continued for another 4 h. Then the reaction mixture was cooled to room temperature and the CO balloon was removed. Subsequently, saturated NaCl solution (30 mL) was added into the flask and the mixture was extracted with EtOAc (3 × 10 mL). The combined organic layers were dried over anhydrous Na2SO4 and then evaporated in vacuo. The residue was purified by column chromatography on silica gel to afford the corresponding N-substituted quinazoline-2,4-(1H,3H)-dione 3 with petroleum ether/ethyl acetate (8
:
1–1
:
3) as the eluent.
3-Butylquinazoline-2,4(1H,3H)-dione (3-1a).
White solid; mp 153–154 °C (Lit.34 156–157 °C); 1H NMR (400 MHz, CDCl3) δ 9.86 (s, 1H), 8.14 (dd, J = 7.9, 1.0 Hz, 1H), 7.63–7.59 (m, 1H), 7.25–7.21 (m, 1H), 7.10 (d, J = 8.0 Hz, 1H), 4.10 (t, J = 8.0 Hz, 2H), 1.75–1.67 (m, 2H), 1.49–1.39 (m, 2H), 0.98 (t, J = 8.0 Hz, 3H); 13C NMR (150 MHz, CDCl3) δ 162.4, 152.5, 138.7, 134.9, 128.3, 123.3, 115.1, 114.7, 40.9, 30.0, 20.2, 13.8.
3-Butyl-1-methylquinazoline-2,4(1H,3H)-dione (3-1b).
White solid; mp 67–68 °C (Lit.35 70–72 °C); 1H NMR (400 MHz, DMSO-d6) δ 8.05 (dd, J = 7.8, 1.5 Hz, 1H), 7.79–7.75 (m, 1H), 7.45 (d, J = 8.4 Hz, 1H), 7.30 (t, J = 7.6 Hz, 1H), 3.96–3.93 (m, 2H), 3.52 (s, 3H), 1.60–1.52 (m, 2H), 1.36–1.26 (m, 2H), 0.91 (t, J = 7.2 Hz, 3H); 13C NMR (150 MHz, CDCl3) δ 161.7, 151.0, 140.5, 135.0, 128.9, 122.9, 115.6, 113.4, 41.8, 30.7, 30.0, 20.3, 13.8.
3-Butyl-1-ethylquinazoline-2,4(1H,3H)-dione (3-1c).
Red brown liquid; 1H NMR (400 MHz, CDCl3) δ 8.24 (dd, J = 7.9, 1.6 Hz, 1H), 7.68–7.64 (m, 1H), 7.26–7.20 (m, 2H), 4.20 (q, J = 7.2 Hz, 2H), 4.11–4.07 (m, 2H), 1.72–1.63 (m, 2H), 1.46–1.39 (m, 2H), 1.35 (t, J = 7.2 Hz, 3H), 0.98–0.95 (m, 3H); 13C NMR (150 MHz, CDCl3) δ 161.8, 150.5, 139.6, 134.9, 129.2, 122.7, 115.8, 113.3, 41.7, 38.7, 29.9, 20.3, 13.8, 12.6; HRMS Calcd (ESI) m/z for [C14H18N2O2Na]+ [M + Na]+: 269.1260, found: 269.1258.
3-Butyl-1-isopropylquinazoline-2,4(1H,3H)-dione (3-1d).
White solid; mp 55–57 °C; 1H NMR (400 MHz, CDCl3) δ 8.24 (dd, J = 7.9, 1.6 Hz, 1H), 7.65–7.60 (m, 1H), 7.36 (d, J = 8.4 Hz, 1H), 7.24–7.20 (m, 1H), 5.06 (s, 1H), 4.08–4.04 (m, 2H), 1.71–1.65 (m, 2H), 1.62 (d, J = 6.8 Hz, 6H), 1.47–1.36 (m, 2H), 0.96 (t, J = 7.2 Hz, 3H); 13C NMR (150 MHz, CDCl3) δ 161.8, 150.4, 140.0, 134.4, 129.3, 122.5, 116.3, 114.1, 48.7, 41.6, 30.0, 20.3, 19.8, 13.8. HRMS Calcd (ESI) m/z for [C15H20N2O2Na]+ [M + Na]+: 283.1417, found: 283.1417.
3-Butyl-1,8-dimethylquinazoline-2,4(1H,3H)-dione (3-1f).
White solid; mp 52–53 °C; 1H NMR (400 MHz, CDCl3) δ 8.08 (dd, J = 7.8, 1.5 Hz, 1H), 7.44 (dd, J = 7.4, 0.7 Hz, 1H), 7.15 (t, J = 7.6 Hz, 1H), 4.06 (t, J = 7.6 Hz, 2H), 3.69 (s, 3H), 2.62 (s, 3H), 1.71–1.63 (m, 2H), 1.45–1.35 (m, 2H), 0.96 (t, J = 7.4 Hz, 3H); 13C NMR (150 MHz, CDCl3) δ 161.0, 151.7, 140.4, 138.0, 125.9, 123.8, 122.4, 116.8, 40.8, 36.7, 28.9, 21.9, 19.2, 12.8; HRMS calcd (ESI) m/z for [C14H18N2O2Na]+ [M + Na]+: 269.1260, found: 269.1256.
3-Butyl-1,7-dimethylquinazoline-2,4(1H,3H)-dione (3-1g).
White solid; mp 83–84 °C; 1H NMR (400 MHz, CDCl3) δ 8.10 (d, J = 8.0 Hz, 1H), 7.07 (d, J = 8.0 Hz, 1H), 6.98 (s, 1H), 4.10–4.06 (m, 2H), 3.59 (s, 3H), 2.48 (s, 3H), 1.71–1.63 (m, 2H), 1.45–1.36 (m, 2H), 0.96 (t, J = 7.4 Hz, 3H); 13C NMR (150 MHz, CDCl3) δ 161.7, 151.2, 146.2, 140.6, 128.8, 124.1, 113.7, 113.3, 41.7, 30.6, 30.0, 22.3, 20.3, 13.8; HRMS calcd (ESI) m/z for [C14H18N2O2Na]+ [M + Na]+: 269.1260, found: 269.1261.
3-Butyl-1,6-dimethylquinazoline-2,4(1H,3H)-dione (3-1h).
White solid; mp 76–77 °C; 1H NMR (400 MHz, CDCl3) δ 8.02 (s, 1H), 7.47 (dd, J = 8.5, 2.0 Hz, 1H), 7.09 (d, J = 8.4 Hz, 1H), 4.11–4.07 (m, 2H), 3.58 (s, 3H), 2.41 (s, 3H), 1.71–1.63 (m, 2H), 1.45–1.36 (m, 2H), 0.96 (t, J = 7.4 Hz, 3H); 13C NMR (150 MHz, CDCl3) δ 161.8, 151.0, 138.4 135.9, 132.6, 128.6, 115.4, 113.4, 41.8, 30.6, 30.0, 20.5, 20.3, 13.8; HRMS calcd (ESI) m/z for [C14H18N2O2Na]+ [M + Na]+: 269.1260, found: 269.1259.
3-Butyl-6-methoxy-1-methylquinazoline-2,4(1H,3H)-dione (3-1i).
White solid; mp 114–115 °C; 1H NMR (400 MHz, DMSO-d6) δ 7.49 (d, J = 2.8 Hz, 1H), 7.43–7.37 (m, 2H), 3.97–3.93 (m, 2H), 3.83 (s, 3H), 3.50 (s, 3H), 1.60–1.52 (m, 2H), 1.35–1.23 (m, 2H), 0.90 (t, J = 7.4 Hz, 3H); 13C NMR (150 MHz, CDCl3) δ 161.7, 155.4, 150.7, 134.7, 123.9, 116.2, 115.1, 109.8, 55.8, 41.9, 30.8, 30.0, 20.3, 13.8; HRMS calcd (ESI) m/z for [C14H18N2O3Na]+ [M + Na]+: 285.1210, found: 285.1210.
3-Butyl-8-chloro-1-methylquinazoline-2,4(1H,3H)-dione (3-1j).
White solid; mp 68–69 °C; 1H NMR (400 MHz, CDCl3) δ 8.23 (dd, J = 7.9, 1.6 Hz, 1H), 7.70–7.65 (m, 1H), 7.20 (d, J = 8.4 Hz, 1H), 4.11–4.08 (m, 2H), 3.61 (s, 3H), 1.72–1.64 (m, 2H), 1.46–1.37 (m, 2H), 0.96 (t, J = 7.2 Hz, 3H); 13C NMR (150 MHz, CDCl3) δ 161.7, 151.0, 140.5, 135.0, 128.9, 122.9, 115.6, 113.4, 41.8, 30.7, 30.0, 20.3, 13.8; HRMS calcd (ESI) m/z for [C13H16ClN2O2]+ [M + H]+: 267.0895, found: 268.0897.
3-Butyl-7-chloro-1-methylquinazoline-2,4(1H,3H)-dione (3-1k).
White solid; mp 79–80 °C; 1H NMR (400 MHz, CDCl3) δ 8.15 (d, J = 8.4 Hz, 1H), 7.23–7.20 (m, 2H), 4.07 (t, J = 7.6 Hz, 2H), 3.58 (s, 3H), 1.70–1.63 (m, 2H), 1.45–1.36 (m, 2H), 0.96 (t, J = 7.4 Hz, 3H); 13C NMR (150 MHz, CDCl3) δ 161.0, 150.8, 141.4, 141.3, 130.4, 123.3, 114.1, 113.7, 41.9, 30.8, 29.9, 20.2, 13.8; HRMS calcd (ESI) m/z for [C13H15ClN2O2Na]+ [M + Na]+: 289.0714, found: 289.0714.
3-Butyl-6-chloro-1-methylquinazoline-2,4(1H,3H)-dione (3-1l).
White solid; mp 103–104 °C (Lit.36 106 °C); 1H NMR (400 MHz, CDCl3) δ 8.19 (d, J = 2.8 Hz, 1H), 7.61 (dd, J = 8.9, 2.5 Hz, 1H), 7.14 (d, J = 9.2 Hz, 1H), 4.10–4.06 (m, 2H), 3.59 (s, 3H), 1.70–1.63 (m, 2H), 1.45–1.36 (m, 2H), 0.96 (t, J = 7.4 Hz, 3H); 13C NMR (150 MHz, CDCl3) δ 160.7, 150.7, 139.0, 134.9, 128.7, 128.3, 116.8, 115.1, 42.0, 30.9, 29.9, 20.2, 13.8.
3-Butyl-1-methyl-6-(trifluoromethyl)quinazoline-2,4(1H,3H)-dione (3-1m).
White solid; mp > 300 °C; 1H NMR (400 MHz, DMSO-d6) δ 8.22 (s, 1H), 8.07 (d, J = 8.8 Hz, 1H), 7.63 (d, J = 8.8 Hz, 1H), 3.92 (t, J = 7.3 Hz, 2H), 3.54 (s, 3H), 1.59–1.52 (m, 2H), 1.35–1.26 (m, 2H), 0.89 (t, J = 7.3 Hz, 3H); 13C NMR (100 MHz, DMSO-d6) δ 160.7, 150.6, 143.6, 131.8 (q, J = 3.4 Hz), 125.2 (q, J = 4.0 Hz), 124.3 (q, J = 271.6 Hz), 123.4 (q, J = 33.2 Hz), 116.5, 115.4, 41.5, 31.4, 29.7, 20.1, 14.1. HRMS calcd (ESI) m/z for [C14H15F3N2O2Na]+ [M + Na]+: 323.0978, found: 323.0977.
6-Acetyl-3-butyl-1-methylquinazoline-2,4(1H,3H)-dione (3-1n).
White solid; mp 104–105 °C; 1H NMR (400 MHz, CDCl3) δ 8.75 (d, J = 2.1 Hz, 1H), 8.30 (dd, J = 8.8, 2.2 Hz, 1H), 7.27 (s, 1H), 4.10 (t, J = 7.4 Hz, 2H), 3.64 (s, 1H), 2.66 (s, 1H), 1.70–1.63 (m, 2H), 1.41 (q, J = 7.4 Hz, 2H), 0.96 (t, J = 7.4 Hz, 3H);13C NMR (100 MHz, CDCl3) δ 196.0, 161.2, 150.8, 143.7, 134.3, 131.8, 130.2, 115.1, 114.0, 42.0, 31.0, 29.9, 26.5, 20.2, 13.8; HRMS calcd (ESI) m/z for [C15H19N2O3]+ [M + H]+: 275.1390, found: 275.1392.
1,3-Dimethylquinazoline-2,4(1H,3H)-dione (3-2b).
White solid; mp 161–162 °C (Lit.37 164–165 °C); 1H NMR (400 MHz, DMSO-d6) δ 8.05 (dd, J = 7.8, 1.6 Hz, 1H), 7.80–7.75 (m, 1H), 7.45 (d, J = 8.4 Hz, 1H), 7.32–7.28 (m, 1H), 3.52 (s, 3H), 3.31 (s, 3H); 13C NMR (150 MHz, CDCl3) δ 162.0, 151.2, 140.4, 135.0, 128.9, 122.9, 115.4, 113.5, 30.8, 28.5.
3-Ethyl-1-methylquinazoline-2,4(1H,3H)-dione (3-2c).
White solid; mp 129–30 °C (Lit.38 133–34 °C); 1H NMR (400 MHz, DMSO-d6) δ 8.05 (d, J = 7.6 Hz, 1H), 7.79–7.75 (m, 1H), 7.44 (d, J = 8.4 Hz, 1H), 7.32–7.28 (m, 1H), 3.99 (q, J = 7.2 Hz, 2H), 3.52 (s, 3H), 1.16 (t, J = 7.2 Hz, 3H); 13C NMR (150 MHz, CDCl3) δ 161.5, 150.8, 140.5, 134.9, 128.8, 122.8, 115.6, 113.4, 37.1, 30.6, 13.1.
1-Methyl-3-propylquinazoline-2,4(1H,3H)-dione (3-2d).
White solid; mp 91–92 °C (Lit.35 90–92 °C); 1H NMR (400 MHz, DMSO-d6) δ 8.05 (dd, J = 7.8, 1.5 Hz, 1H), 7.80–7.75 (m, 1H), 7.45 (d, J = 8.4 Hz, 1H), 7.32–7.28 (m, 1H), 3.93–3.89 (m, 2H), 3.52 (s, 3H), 1.65–1.56 (m, 2H), 0.88 (t, J = 7.6 Hz, 3H); 13C NMR (150 MHz, CDCl3) δ 161.8, 151.0, 140.5, 135.0, 128.9, 122.9, 115.6, 113.4, 43.5, 30.7, 21.1, 11.4.
3-Isobutyl-1-methylquinazoline-2,4(1H,3H)-dione (3-2e).
White solid; mp 83–84 °C; 1H NMR (400 MHz, DMSO-d6) δ 8.05 (dd, J = 7.9, 1.5 Hz, 1H), 7.80–7.76 (m, 1H), 7.45 (d, J = 8.4 Hz, 1H), 7.32–7.28 (m, 1H), 3.80 (d, J = 7.2 Hz, 2H), 3.52 (s, 3H), 2.13–2.03 (m, 1H), 0.87 (d, J = 6.8 Hz, 6H); 13C NMR (150 MHz, CDCl3) δ 162.0, 151.3, 140.5, 135.0, 129.0, 122.8, 115.6, 113.4, 48.7, 30.7, 27.2, 20.2; HRMS calcd (ESI) m/z for [C13H16N2O2Na]+ [M + Na]+: 255.1104, found: 255.1103.
3-Cyclohexyl-1-methylquinazoline-2,4(1H,3H)-dione (3-2f).
White solid; mp 158–159 °C; CAS registry number: 878994-46-8; 1H NMR (400 MHz, CDCl3) δ 8.20 (dd, J = 7.9, 1.5 Hz, 1H), 7.87–7.83 (m, 1H), 7.25–7.22 (m, 1H), 7.16 (d, J = 8.4 Hz, 1H), 4.98–4.90 (m, 1H), 3.57 (s, 3H), 2.51–2.44 (m, 2H), 1.86 (d, J = 13.2 Hz, 2H), 1.70–1.67 (m, 3H), 1.47–1.25 (m, 3H); 13C NMR (150 MHz, CDCl3) δ 162.2, 151.0, 140.6, 134.8, 129.0, 122.7, 116.0, 113.3, 55.0, 30.6, 28.8, 26.4, 25.4.
3-Benzyl-1-methylquinazoline-2,4(1H,3H)-dione (3-2g).
White solid; mp 126–127 °C (Lit.39 131 °C); 1H NMR (400 MHz, DMSO-d6) δ 8.08 (dd, J = 7.8, 1.6 Hz, 1H), 7.82–7.78 (m, 1H), 7.48 (d, J = 8.4 Hz, 1H), 7.34–7.28 (m, 5H), 7.26–7.22 (m, 1H), 5.15 (s, 2H), 3.53 (s, 3H); 13C NMR (150 MHz, CDCl3) δ 161.8, 151.1, 140.5, 137.1, 135.2, 129.1, 129.0, 128.4, 127.6, 123.0, 115.6, 113.5, 45.0, 30.8.
1-Methyl-3-phenylquinazoline-2,4(1H,3H)-dione (3-2h).
White solid; mp 224–225 °C (Lit.40 228 °C); 1H NMR (400 MHz, DMSO-d6) δ 8.06 (dd, J = 7.8, 1.6 Hz, 1H), 7.85–7.81 (m, 1H), 7.53–7.43 (m, 4H), 7.35–7.30 (m, 3H), 3.54 (s, 3H); 13C NMR (150 MHz, CDCl3) δ 161.9, 151.2, 140.8, 135.6, 135.5, 129.4, 129.3, 128.8, 128.4, 123.2, 116.0, 113.7, 30.8.
1-Methyl-3-(o-tolyl)quinazoline-2,4(1H,3H)-dione (3-2i).
White solid; mp 177–179 °C (Lit.40 182 °C); 1H NMR (400 MHz, CDCl3) δ 8.28 (dd, J = 7.8, 1.5 Hz, 1H), 7.77–7.73 (m, 1H), 7.37–7.28 (m, 5H), 7.16 (d, J = 6.8 Hz, 1H), 3.66 (s, 3H), 2.16 (s, 3H); 13C NMR (150 MHz, CDCl3) δ 161.5, 150.7, 141.0, 135.8, 135.5, 134.8, 131.1, 129.4, 129.1, 128.3, 127.1, 123.1, 115.9, 113.8, 30.9, 17.5.
1-Methyl-3-(m-tolyl)quinazoline-2,4(1H,3H)-dione (3-2j).
White solid; mp 164–165 °C; 1H NMR (400 MHz, CDCl3) δ 8.27 (dd, J = 7.8, 1.5 Hz, 1H), 7.76–7.71 (m, 1H), 7.40 (t, J = 7.6 Hz, 1H), 7.32–7.27 (m, 3H), 7.07 (d, J = 8.4 Hz, 2H), 3.65 (s, 3H), 2.40 (s, 3H); 13C NMR (150 MHz, CDCl3) δ 162.0, 151.3, 140.8, 139.5, 135.6, 135.5, 129.7, 129.3, 129.3, 128.9, 125.3, 123.1, 116.0, 113.8, 30.8, 21.4; HRMS calcd (ESI) m/z for [C16H14N2O2Na]+ [M + Na]+: 289.0947, found: 289.0943.
1-Methyl-3-(p-tolyl)quinazoline-2,4(1H,3H)-dione (3-2k).
White solid; mp 194–195 °C (Lit.41 190 °C); 1H NMR (400 MHz, CDCl3) δ 8.27 (dd, J = 7.8, 1.5 Hz, 1H), 7.75–7.71 (m, 1H), 7.32–7.26 (m, 4H), 7.16–7.14 (m, 2H), 3.64 (s, 3H), 2.42 (s, H); 13C NMR (150 MHz, CDCl3) δ 162.0, 151.3, 140.8, 138.7, 135.4, 133.0, 130.2, 129.3, 128.0, 123.1, 116.0, 113.7, 30.9, 21.3.
3-(2-Chlorophenyl)-1-methylquinazoline-2,4(1H,3H)-dione (3-2l).
White solid; mp 153–155 °C; 1H NMR (400 MHz, CDCl3) δ 8.28 (dd, J = 7.8, 1.5 Hz, 1H), 7.78–7.74 (m, 1H), 7.60–7.55 (m, 1H), 7.44–7.40 (m, 2H), 7.35–7.29 (m, 3H), 3.66 (s, 3H); 13C NMR (150 MHz, CDCl3) δ 161.2, 150.3, 141.0, 135.7, 133.6, 132.8, 130.3, 130.3, 129.4, 127.9, 123.2, 115.7, 113.9, 30.9; HRMS calcd (ESI) m/z for [C15H11ClN2O2Na]+ [M + Na]+: 309.0401, found: 309.0395.
3-(3-Chlorophenyl)-1-methylquinazoline-2,4(1H,3H)-dione (3-2m).
White solid; mp 186–187 °C; CAS registry number: 878995-13-2; 1H NMR (400 MHz, CDCl3) δ 8.26 (dd, J = 7.8, 1.6 Hz, 1H), 7.78–7.73 (m, 1H), 7.46–7.44 (m, 2H), 7.33–7.28 (m, 3H), 7.20–7.17 (m, 1H), 3.65 (s, 3H); 13C NMR (150 MHz, CDCl3) δ 161.7, 150.9, 140.8, 136.6, 135.7, 134.9, 130.3, 129.3, 129.1, 129.0, 126.9, 123.3, 115.8, 113.9, 30.9.
3-(4-Chlorophenyl)-1-methylquinazoline-2,4(1H,3H)-dione (3-2n).
White solid; mp 224–226 °C (Lit.42 223–225 °C); 1H NMR (400 MHz, CDCl3) δ 8.26 (dd, J = 7.9, 1.6 Hz, 1H), 7.77–7.73 (m, 1H), 7.50–7.47 (m, 2H), 7.33–7.27 (m, 2H), 7.24–7.19 (m, 2H), 3.65 (s, 1H); 13C NMR (150 MHz, CDCl3) δ 161.8, 151.0, 140.8, 135.7, 134.7, 134.1, 129.9, 129.7, 129.3, 123.3, 115.8, 113.8, 30.9.
3-(4-Bromophenyl)-1-methylquinazoline-2,4(1H,3H)-dione (3-2o).
White solid; mp 209–211 °C (Lit.42 214–216 °C); 1H NMR (400 MHz, CDCl3) δ 8.26 (dd, J = 7.9, 1.5 Hz, 1H), 7.77–7.73 (m, 1H), 7.65–7.62 (m, 2H), 7.33–7.27 (m, 2H), 7.17–7.15 (m, 2H), 3.64 (s, 3H); 13C NMR (150 MHz, CDCl3) δ 161.7, 150.9, 140.8, 135.7, 134.6, 132.6, 130.2, 129.3, 123.3, 122.8, 115.8, 113.8, 30.9.
3-(2,6-Dimethylphenyl)-1-methylquinazoline-2,4(1H,3H)-dione (3-2p).
White solid; mp 177–179 °C (Lit.43 183 °C); 1H NMR (400 MHz, DMSO-d6) δ 8.10 (dd, J = 7.8, 1.6 Hz, 1H), 7.89–7.85 (m, 1H), 7.57 (d, J = 8.4 Hz, 1H), 7.39–7.35 (m, 1H), 7.27–7.18 (m, 3H), 3.58 (s, 3H), 2.00 (s, 6H); 13C NMR (150 MHz, CDCl3) δ 161.1, 150.2, 141.1, 135.5, 135.4, 134.0, 129.4, 128.8, 128.6, 123.1, 115.7, 113.8, 30.9, 17.8.
1-Methyl-3-(4-nitrophenyl)quinazoline-2,4(1H,3H)-dione (3-2q).
White solid; mp 254–255 °C; 1H NMR (400 MHz, CDCl3) δ 8.39–8.37 (m, 2H), 8.27 (dd, J = 7.9, 1.5 Hz, 1H), 7.80–7.76 (m, 1H), 7.51–7.47 (m, 2H), 7.36–7.30 (m, 2H), 3.66 (s, 3H); 13C NMR (150 MHz, CDCl3) δ 161.5, 150.5, 147.7, 141.3, 140.8, 136.0, 130.0, 129.4, 124.6, 123.6, 115.6, 114.0, 30.9; HRMS calcd (ESI) m/z for [C15H11N3O4Na]+ [M + Na]+: 320.0642, found: 320.0641.
1-Methyl-3-(naphthalen-1-yl)quinazoline-2,4(1H,3H)-dione (3-2r).
Red brown solid; mp 187–188 °C (Lit.40 191 °C); 1H NMR (400 MHz, CDCl3) δ 8.31–8.29 (m, 1H), 7.98–7.92 (m, 2H), 7.81–7.76 (m, 1H), 7.62–7.57 (m, 2H), 7.52–7.43 (m, 3H), 7.35–7.31 (m, 2H), 3.69 (s, 3H); 13C NMR (150 MHz, CDCl3) δ 162.0, 151.2, 141.1, 135.6, 134.6, 132.4, 130.0, 129.5, 129.5, 128.7, 127.2, 126.5, 126.3, 125.7, 123.3, 121.7, 115.9, 113.9, 31.0.
3-(Benzo[d]thiazol-2-yl)-1-methylquinazoline-2,4(1H,3H)-dione (3-2s).
White solid; mp 276–278 °C; 1H NMR (400 MHz, CDCl3) δ 8.28 (dd, J = 7.9, 1.5 Hz, 1H), 8.12–8.10 (m, 1H), 7.94–7.92 (m, 1H), 7.80–7.76 (m, 1H), 7.56–7.46 (m, 2H), 7.36–7.29 (m, 2H), 3.66 (s, 3H); 13C NMR (150 MHz, CDCl3) δ 161.3, 155.5, 150.3, 150.0, 140.8, 136.7, 136.2, 129.4, 126.4, 126.2, 124.3, 123.6, 121.8, 115.5, 114.1, 30.9; HRMS calcd (ESI) m/z for [C16H11N3O2SNa]+ [M + Na]+: 332.0464, found: 332.0463.
1-Methyl-3-(thiazol-2-yl)quinazoline-2,4(1H,3H)-dione (3-2t).
White solid; mp 224–225 °C; 1H NMR (400 MHz, CDCl3) δ 8.25 (d, J = 7.0 Hz, 1H), 7.87 (d, J = 3.4 Hz, 1H), 7.76 (t, J = 7.3 Hz, 1H), 7.56 (d, J = 3.4 Hz, 1H), 7.32–7.29 (m, 2H), 3.64 (s, 1H); 13C NMR (150 MHz, CDCl3) δ 161.7, 155.6, 150.4, 141.4, 140.9, 136.2, 129.6, 123.7, 122.6, 115.6, 114.1, 31.0; HRMS calcd (ESI) m/z for [C12H10N3O2S]+ [M + H]+: 260.0488, found: 260.0489.
1-Methyl-3-(pyridin-2-yl)quinazoline-2,4(1H,3H)-dione (3-2u).
White solid; mp 268–269 °C; 1H NMR (400 MHz, CDCl3) δ 8.70 (dd, J = 4.8, 1.3 Hz, 1H), 8.27–8.25 (m, 1H), 7.94–7.89 (m, 1H), 7.77–7.72 (m, 1H), 7.44–7.41 (m, 1H), 7.36 (d, J = 8.0 Hz, 1H), 7.32–7.27 (m, 2H), 3.64 (s, 3H); 13C NMR (150 MHz, CDCl3) δ 161.8, 150.8, 150.0, 149.4, 141.0, 138.6, 135.6, 129.2, 124.2, 123.9, 123.2, 116.0, 113.8, 30.6; HRMS calcd (ESI) m/z for [C14H11N3O2Na]+ [M + Na]+: 276.0743, found: 276.0741.
3-(3-(4-Phenylpiperazin-1-yl)propyl)quinazoline-2,4(1H,3H)-dione (Pelanserin).
White solid; mp 173–174 °C; CAS registry number: 2208-51-7; 1H NMR (400 MHz, CDCl3) δ 10.39 (s, 1H), 8.13 (d, J = 7.8 Hz, 1H), 7.58 (t, J = 7.5 Hz, 1H), 7.23 (dd, J = 14.5, 6.9 Hz, 4H), 7.10 (d, J = 8.1 Hz, 1H), 6.89 (d, J = 8.1 Hz, 2H), 6.84 (t, J = 7.3 Hz, 1H), 4.20 (t, J = 7.2 Hz, 2H), 3.14 (d, J = 4.4 Hz, 4H), 2.62 (s, 4H), 2.56 (t, J = 7.0 Hz, 2H), 2.00–1.96 (m, 2H); 13C NMR (100 MHz, CDCl3) δ 162.5, 152.2, 151.3, 138.6, 135.0, 129.1, 128.4, 123.4, 119.7, 116.0, 115.0, 114.7, 56.0, 53.1, 49.1, 39.6, 24.9.
Conflicts of interest
There are no conflicts to declare.
Acknowledgements
We are grateful to the National Natural Science Foundation of China (NSFC) (21772033 and U1604285), the Program for Innovative Research Team in Science and Technology in Universities of Henan Province (20IRTSTHN005) and the 111 Project (D17007) for financial support.
References
-
(a) S. Biswas, R. Khatun, M. Dolai, I. H. Biswas, N. Haque, M. Sengupta, M. S. Islam and S. M. Islam, New J. Chem., 2020, 44, 141–151 RSC;
(b) I. Khan, A. Ibrar, N. Abbas and A. Saeed, Eur. J. Med. Chem., 2014, 76, 193–244 CrossRef CAS PubMed.
-
(a) K. El-Adl, A. G. A. El-Helby, H. Sakr and S. S. A. El-Hddad, Arch. Pharm., 2020, 353, e2000068 CrossRef PubMed;
(b) H. Akgun, D. U. Yilmaz, R. C. Atalay and D. Gozen, Lett. Drug Des. Discovery, 2016, 13, 64–76 CrossRef CAS.
-
(a) J. Zhou, M. Ji, H. P. Yao, R. Cao, H. L. Zhao, X. Y. Wang, X. G. Chen and B. L. Xu, Org. Biomol. Chem., 2018, 16, 3189–3202 RSC;
(b) A. Aboelmagd, E. M. S. Salem, I. A. I. Ali and M. S. Gomaa, ARKIVOC, 2018, iii, 20–35 Search PubMed.
-
(a) M. K. Prashanth, M. Madaiah, H. D. Revanasiddappa and B. Veeresh, Spectrochim. Acta, Part A, 2013, 110, 324–332 CrossRef CAS PubMed;
(b) V. Colotta, O. Lenzi, D. Catarzi, F. Varano, L. Squarcialupi, C. Costagli, A. Galli, C. Ghelardini, A. M. Pugliese, G. Maraula, E. Coppi, D. E. Pellegrini-Giampietro, F. Pedata, D. Sabbadin and S. Moro, Eur. J. Med. Chem., 2012, 54, 470–482 CrossRef CAS PubMed.
-
(a) I. Crespo, J. Giménez-Dejoz, S. Porté, A. Cousido-Siah, A. Mitschler, A. Podjarny, H. Pratsinis, D. Kletsas, X. Parés, F. X. Ruiz, K. Metwally and J. Farrés, Eur. J. Med. Chem., 2018, 152, 160–174 CrossRef CAS PubMed;
(b) S. Goto, H. Tsuboi, M. Kanoda, K. Mukai and K. Kagara, Org. Process Res. Dev., 2003, 7, 700–706 CrossRef CAS.
-
(a) D. G. Piotrowska, G. Andrei, D. Schols, R. Snoeck and M. Łysakowska, Eur. J. Med. Chem., 2017, 126, 84–100 CrossRef CAS PubMed;
(b) D. W. Kang, H. Zhang, Z. X. Zhou, B. S. Huang, L. Naesens, P. Zhan and X. Y. Liu, Bioorg. Med. Chem. Lett., 2016, 26, 5182–5186 CrossRef CAS PubMed.
-
(a) B. He, F. X. Wu, L. K. Yu, L. Wu, Q. Chen, G. F. Hao, W. C. Yang, H. Y. Lin and G. F. Yang, J. Agric. Food Chem., 2020, 68, 5059–5067 CrossRef CAS PubMed;
(b) D. W. Wang, H. Y. Lin, R. J. Cao, Z. Z. Ming, T. Chen, G. F. Hao, W. C. Yang and G. F. Yang, Pest Manage. Sci., 2015, 71, 1122–1132 CrossRef CAS PubMed.
-
(a) D. W. Pan, H. Du, X. Y. Lü and X. P. Bao, Chin. J. Org. Chem., 2016, 36, 818–825 CrossRef CAS;
(b) K. J. Aldred, H. A. Schwanz, G. Q. Li, S. A. McPherson, C. L. Turnbough, Jr., R. J. Kerns and N. Osheroff, ACS Chem. Biol., 2013, 8, 2660–2668 CrossRef CAS PubMed.
- Q. G. Ji, D. Yang, Q. Deng, Z. Q. Ge and L. J. Yuan, Med. Chem. Res., 2014, 23, 2169–2177 CrossRef CAS.
- Q. G. Ji, D. Yang, X. Wang, C. Y. Chen, Q. Deng, Z. Q. Ge, L. J. Yuan, X. L. Yang and F. Liao, Bioorg. Med. Chem., 2014, 22, 3405–3413 CrossRef CAS PubMed.
- N. A. Noureldin, H. Kothayer, E. S. M. Lashine, M. M. Baraka, Y. R. Huang, B. Li and Q. G. Ji, Eur. J. Med. Chem., 2018, 152, 560–569 CrossRef CAS PubMed.
- S. J. Kirincich, J. Xiang, N. Green, S. Tam, H. Y. Yang, J. Shim, M. W. H. Shen, J. D. Clark and J. C. McKew, Bioorg. Med. Chem., 2009, 17, 4383–4405 CrossRef CAS PubMed.
- W. B. Wright, Jr., A. S. Tomcufcik, P. S. Chan, J. W. Marsico and J. B. Press, J. Med. Chem., 1987, 30, 2277–2283 CrossRef PubMed.
-
(a) H. Chen, P. Li, R. F. Qin, H. Yan, G. Li and H. H. Huang, ACS Omega, 2020, 5, 9614–9623 CrossRef CAS PubMed;
(b) P. Mampuys, H. Neumann, S. Sergeyev, R. V. A. Orru, H. J. Jiao, A. Spannenberg, B. U. W. Maes and M. Beller, ACS Catal., 2017, 7, 5549–5556 CrossRef CAS;
(c) H. Z. Jin, J. L. Du, W. D. Zhang, H. S. Chen, J. H. Lee and J. J. LEE, J. Asian Nat. Prod. Res., 2007, 9, 685–688 CrossRef CAS PubMed.
- M. A. H. Ismail, S. Barker, D. A. Abou El Ella, K. A. M. Abouzid, R. A. Toubar and M. H. Todd, J. Med. Chem., 2006, 49, 1526–1535 CrossRef CAS PubMed.
-
(a) E. Durham, D. Perkins, J. S. Scott, J. Wang and S. Watson, Synlett, 2016, 27, 965–968 CAS;
(b) C. O. Usifoh and G. K. E. Scriba, Arch. Pharm., 2000, 333, 261–266 CrossRef CAS.
- I. E. G1owacka, J. Balzarini and A. E. Wróblewski, Eur. J. Med. Chem., 2013, 70, 703–722 CrossRef PubMed.
-
(a) W. Wertjes, S. Ayers, Q. Gao, E. M. Simmons and G. L. Beutner, Synthesis, 2018, 4453–4461 CAS;
(b) G. L. Beutner, Y. Hsiao, T. Razler, E. M. Simmons and W. Wertjes, Org. Lett., 2017, 19, 1052–1055 CrossRef CAS PubMed.
-
(a) M. M. Khalifa, S. C. Philkhana and J. E. Golden, J. Org. Chem., 2020, 85, 464–481 CrossRef CAS PubMed;
(b) K. Scheidt, A. Lee, J. Zhu, T. Feoktistova, A. Brueckner and P. Cheong, Angew. Chem., Int. Ed., 2019, 58, 5941–5945 CrossRef PubMed;
(c) C. Verma, S. Sharma and A. Pathak, Tetrahedron Lett., 2013, 54, 6897–6899 CrossRef CAS;
(d) X. L. Chen, H. W. Jia, Z. Li and X. Y. Xu, Chin. Chem. Lett., 2019, 30, 1207–1213 CrossRef CAS;
(e) X. F. Zhang, X. M. Ma, W. Q. Qiu, J. Evans and W. Zhang, Green Chem., 2019, 21, 349 RSC;
(f) S. Gao, M. Chen, M. N. Zhao, W. Du, Z. H. Ren, Y. Y. Wang and Z. H. Guan, J. Org. Chem., 2014, 79, 4196–4200 CrossRef CAS PubMed.
- Y. S. Zhang, J. Labelled Compd. Radiopharm., 2012, 55, 419–422 CrossRef CAS.
-
(a) G. Beutner, R. Carrasquillo, P. Geng, Y. Hsiao, E. C. Huang, J. Janey, K. Katipally, S. Kolotuchin, T. L. Porte, A. Lee, P. Lobben, F. Lora-Gonzalez, B. Mack, B. Mudryk, Y. P. Qiu, X. H. Qian, A. Ramirez, T. M. Razler, T. Rosner, Z. P. Shi, E. Simmons, J. Stevens, J. J. Wang, C. Wei, S. R. Wisniewski and Y. Zhu, Org. Lett., 2018, 20, 3736–3740 CrossRef CAS PubMed;
(b) C. W. Yu, P. Y. Hung, H. T. Yang, Y. H. Ho, H. Y. Lai, Y. S. Cheng and J. W. Chern, J. Med. Chem., 2019, 62, 857–874 CrossRef CAS PubMed.
-
(a) Y. Fukase, A. Sato, Y. Tomata, A. Ochida, M. Kono, K. Yonemori, K. Koga, T. Okui, M. Yamasaki, Y. Fujitani, H. Nakagawa, R. Koyama, M. Nakayama, R. Skene, B. C. Sang, I. Hoffman, J. Shirai and S. Yamamoto, Bioorg. Med. Chem., 2018, 26, 721–736 CrossRef CAS PubMed;
(b) W. Chen, Z. Huang, N. E. S. Tay, B. Giglio, M. Wang, H. Wang, Z. H. Wu, D. A. Nicewicz and Z. B. Li, Science, 2019, 364, 1170–1174 CrossRef CAS PubMed;
(c) H. Y. Qian, Z. L. Wang, Y. L. Pan, L. L. Chen, X. Xie and J. Z. Chen, ACS Med. Chem. Lett., 2017, 8, 678–681 CrossRef CAS PubMed.
- T. Miyata, T. Mizuno, Y. Nagahama, I. Nishiguchi and T. Hirashima, Heterocycl. Commun., 1991, 2, 473–475 CAS.
-
(a) A. Castro, M. J. Jerez, C. Gil, F. Calderon, T. Domenech, A. Nueda and A. Martınez, Eur. J. Med. Chem., 2008, 43, 1349–1359 CrossRef CAS PubMed;
(b) E. F. Ismail, I. A. I. Ali, W. Fathalla, A. A. Alsheikh and E. S. E. Tamneya, ARKIVOC, 2017, 4, 104–120 Search PubMed;
(c) B. O. Buckman and R. Mohan, Tetrahedron Lett., 1996, 37, 4439–4442 CrossRef CAS.
-
(a) H. Y. P. Choo, M. Kim, S. K. Lee, S. W. Kimb and I. K. Chung, Bioorg. Med. Chem., 2002, 10, 517–523 CrossRef;
(b) A. L. Smith, C. G. Thomson and P. D. Leeson, Bioorg. Med. Chem., 1996, 6, 1483–1486 CrossRef CAS;
(c) L. Gouilleux, J. A. Fehrentz, F. Winternitz and J. Martinez, Tetrahedron Lett., 1996, 37, 7031–7034 CrossRef CAS.
-
(a) D. Q. Shi, G. L. Dou, Z. Y. Li, S. N. Ni, X. Y. Li, X. S. Wang, H. Wu and S. J. Ji, Tetrahedron, 2007, 63, 9764–9773 CrossRef CAS;
(b) X. W. Wu and Z. K. Yu, Tetrahedron Lett., 2010, 51, 1500–1503 CrossRef CAS.
-
(a) W. Z. Zhang, H. l. Li, Y. Zeng, X. Y. Tao and X. B. Lu, Chin. J. Chem., 2018, 36, 112–118 CrossRef CAS;
(b) P. Xu, F. Wang, T. Q. Wei, L. Yin, S. Y. Wang and S. J. Ji, Org. Lett., 2017, 19, 4484–4487 CrossRef CAS PubMed.
-
(a) B. Roberts, D. Liptrot, T. Luker, M. J. Stocks, C. Barber, N. Webb, R. Dods and B. Martin, Tetrahedron Lett., 2011, 52, 3793–3796 CrossRef CAS;
(b) C. Larksarp and H. Alper, J. Org. Chem., 2000, 65, 2773–2777 CrossRef CAS PubMed.
- T. P. Tran, E. L. Ellsworth, B. M. Watson, J. P. Sanchez, H. D. H. Showalter, J. R. Rubin, M. A. Stier, J. Yip, D. Q. Nguyen, P. Bird and R. Singh, J. Heterocycl. Chem., 2005, 42, 669 CrossRef CAS.
-
(a) Z. P. Yin, J. X. Xu and X. F. Wu, ACS Catal., 2020, 10, 6510–6531 CrossRef CAS;
(b) H. Q. Li, L. He, H. Neumann, M. Beller and X. F. Wu, Green Chem., 2014, 16, 1336–1343 RSC;
(c) F. Doro, P. Winnertz, W. Leitner, A. Prokofieva and T. E. Müller, Green Chem., 2011, 13, 292–295 RSC;
(d) E. Mizushima, T. Hayashi and M. Tanaka, Green Chem., 2001, 3, 76–79 RSC.
-
(a) R. Barakov, N. Shcherban, P. Yaremov, I. Bezverkhyy, J. Čejka and M. Opanasenko, Green Chem., 2020, 22, 6992–7002 RSC;
(b) L. Chen, R. Huang, K. Li, X. H. Yun, C. L. Yang and S. J. Yan, Green Chem., 2020, 22, 6943–6953 RSC;
(c) J. X. Li, Y. D. Wu, M. Hu, C. Li, M. Li, D. D. He and H. F. Jiang, Green Chem., 2019, 21, 4084–4089 RSC;
(d) M. M. Heravi, V. Zadsirjan, M. Dehghani and T. Ahmadi, Tetrahedron, 2018, 74, 3391–3457 CrossRef CAS;
(e) D. Vaughan, A. B. Naidu and A. Jha, Curr. Org. Synth., 2012, 9, 613–649 CrossRef CAS;
(f) F. Z. Peng and Z. H. Shao, Curr. Org. Synth., 2011, 15, 4144–4160 CAS.
-
(a) J. L. Hu, J. J. Li, Y. L. Gu, Z. H. Guan, W. L. Mo, Y. M. Ni, T. Li and G. X. Li, Appl. Catal., A, 2010, 386, 188–193 CrossRef CAS;
(b) P. M. Maitlis, A. Haynes, B. R. James, M. Catellanic and G. P. Chiusolic, Dalton Trans., 2004, 3409–3419 RSC;
(c) B. Gabriele, M. Costa, G. Salernoe and G. P. Chiusoli, J. Chem. Soc., Perkin Trans. 1, 1994, 83–87 RSC;
(d) M. Queirolo, A. Vezzani, R. Mancuso, B. Gabriele, M. Costa and N. D. Ca’, J. Mol. Catal. A: Chem., 2015, 398, 115–126 CrossRef CAS;
(e) S. Fukuoka, M. Chono and M. Kohno, J. Org. Chem., 1984, 49, 1458–1460 CrossRef CAS.
-
(a) F. Pancrazzi, N. Sarti, P. P. Mazzeo, A. Bacchi, C. Carfagna, R. Mancuso, B. Gabriele, M. Costa, A. Stirling and N. D. Ca’, Org. Lett., 2020, 22, 1569–1574 CrossRef CAS PubMed;
(b) K. Karami, S. Abedanzadeh, O. Vahidnia, P. Herves, J. Lipkowski and K. Lyczko, Appl. Organomet. Chem., 2017, 31, e3768 CrossRef;
(c) Z. H. Guan, M. Chen and Z. H. Ren, J. Am. Chem. Soc., 2012, 134, 17490–17493 CrossRef CAS PubMed;
(d) S. Gao, M. Chen, M. N. Zhao, W. Du, Z. H. Ren, Y. Y. Wang and Z. H. Guan, J. Org. Chem., 2014, 79, 4196–4200 CrossRef CAS PubMed;
(e) I. A. Rivero, L. Guerrero, K. A. Espinoza, M. C. Meza and J. R. Rodríguez, Molecules, 2009, 14, 1860–1868 CrossRef CAS PubMed;
(f) E. C. Gaudino, S. Tagliapietra, G. Palmisano, K. Martina, D. Carnaroglio and G. Cravotto, ACS Sustainable Chem. Eng., 2017, 5, 9233–9243 CrossRef;
(g) K. Orito, M. Miyazawa, T. Nakamura, A. Horibata, H. Ushito, H. Nagasaki, M. Yuguchi, S. Yamashita, T. Yamazaki and M. Tokuda, J. Org. Chem., 2006, 71, 5951–5958 CrossRef CAS PubMed.
- A. Elansary, H. Kadry, E. Ahmed and A. Sonousi, Med. Chem. Res., 2012, 21, 3557–3567 CrossRef CAS.
- R. Kuryazov, N. Mukhamedov and K. Shakhidoyatov, Chem. Heterocycl. Compd., 2009, 45, 1508–1514 CrossRef CAS.
- M. Farouk, A. Mohamed, M. Kamal-Eldin and M. Kamal-Eldin, J. Chem. Soc., 1971, 1055–1058 Search PubMed.
- C. M. Lee, J. Heterocycl. Chem., 1964, 1, 235–238 CrossRef CAS.
- N. Lange and F. Sheibley, J. Am. Chem. Soc., 1933, 55, 2113–2116 CrossRef CAS.
- D. Schwinn, H. Glatz and W. Bannwarth, Helv. Chim. Acta, 2003, 86, 188–195 CrossRef CAS.
- G. Beutner, Y. Hsiao, T. Razler, M. Simmons and W. Wertjes, Org. Lett., 2017, 19, 1052–1055 CrossRef CAS PubMed.
- J. Deck and F. Dains, J. Am. Chem. Soc., 1933, 55, 4986–4991 CrossRef CAS.
- H. Mohamed, M. Saied, I. Farahate and E. Ashry, J. Mol. Struct., 2016, 1108, 667–679 CrossRef.
- W. William, A. Sloan, G. Qi, E. Simmons and G. Beutner, Synthesis, 2018, 50, 4453–4461 CrossRef.
Footnote |
† Electronic supplementary information (ESI) available: General information, 1H and 13C NMR spectrum. See DOI: 10.1039/d0gc03254a |
|
This journal is © The Royal Society of Chemistry 2021 |