DOI:
10.1039/D1FO02974F
(Paper)
Food Funct., 2022,
13, 437-450
Flavonoid constituents of Amomum tsao-ko Crevost et Lemarie and their antioxidant and antidiabetic effects in diabetic rats – in vitro and in vivo studies†
Received
7th September 2021
, Accepted 23rd November 2021
First published on 3rd December 2021
Abstract
Amomum tsao-ko Crevost et Lemarie (A. tsao-ko) is a well-known dietary spice and traditional Chinese medicine. This study aimed to identify the flavonoids in A. tsao-ko and evaluate their antioxidant and antidiabetic activities in in vitro and in vivo studies. A. tsao-ko methanol extracts possessed a high flavonoid content (1.21 mg QE per g DW) and a total of 29 flavonoids were identified by employing UPLC-MS/MS. In vitro, A. tsao-ko demonstrated antioxidant activity (ORAC value of 34276.57 μM TE/100 g DW, IC50 of ABTS of 3.49 mg mL−1 and FRAP value of 207.42 μM Fe2+ per g DW) and α-amylase and α-glucosidase inhibitory ability with IC50 values of 14.23 and 1.76 mg mL−1, respectively. In vivo, type 2 diabetes mellitus (T2DM) models were induced by a combined high-fat diet (HFD) and streptozotocin (STZ) injection in rats. Treatment with the A. tsao-ko extract (100 mg freeze-dried powder per kg bw) for 6 weeks could significantly improve impaired glucose tolerance, decrease the levels of fasting blood glucose (FBG), insulin, and malondialdehyde (MDA), and increase the superoxide dismutase (SOD) level. Histopathology revealed that the A. tsao-ko extract preserved the architecture and function of the pancreas. In conclusion, the flavonoid composition of A. tsao-ko exhibits excellent antioxidant and antidiabetic activity in vitro and in vivo. A. tsao-ko could be a novel natural material and developed as a related functional food and medicine in T2DM management.
1. Introduction
Diabetes mellitus (DM) is a chronic metabolic disease characterized by persistent hyperglycemia due to poor insulin action and/or defective secretion.1 Among the types of diabetes, more than 90% is type 2 diabetes mellitus (T2DM), which affects more than 380 million people worldwide.2 Inhibition of pancreatic α-amylase and enteral α-glucosidase, which are key enzymes for carbohydrate digestion, would delay carbohydrate absorption and the increase of postprandial blood glucose levels. So, this could be an important method to treat the occurrence and development of type 2 diabetes.3 Currently, α-glucosidase inhibitors such as acarbose and voglibose are some of the main drugs for treating diabetes. However, the use of these drugs can cause some side effects, including abdominal cramps, diarrhea, flatulence and vomiting.4,5 Therefore, the exploitation of hypoglycemic supplemental drugs from natural plants with high safety and efficiency seems to be a cost-effective solution.
Oxidative stress refers to the imbalance in the generation and clearance of reactive oxygen species (ROS) and reactive nitrogen species in metabolism and is reported to be one of the major mechanisms in the pathophysiology of T2DM and its complications.6–8 Oxidative stress occurs when the rate of free radical generation exceeds the antioxidant defense systems, leading to the toxic effects of free radicals.9 Free-radical-mediated peroxidation of membrane lipids and oxidative damage impair normal insulin signal transduction and glucose homeostasis. Therefore, the use of antioxidants as complementary medicines to T2DM treatment becomes pertinent.
Amomum tsao-ko Crevost et Lemarie (A. tsao-ko), a perennial herbaceous plant of the Zingiberaceae family, is mainly distributed in southwest China (Guangxi, Guizhou, Yunnan), North Vietnam and some other parts of Asia.10 In addition to being an important spice, its dried mature fruit called “Tsao-ko” or “Caoguo” is a traditional Chinese medicinal material and widely used for the treatment of malaria, diarrhea, abdominal pain, throat infections, dyspepsia, stomach disorders, nausea and vomiting.11 The diverse active constituents of A. tsao-ko namely bicyclic nonanes, essential oils, diterpenoids, diarylheptanoids, flavonoids, and phenolic compounds have been reported, which exhibit antimicrobial, antioxidant, anti-obesity, anti-inflammatory, antiproliferative, and neuroprotective properties.12–15 The intake of dried powder and methanol extracts could lower the weight and plasma and liver triacylglycerol concentrations besides showing hypoglycemic and antioxidative abilities in normal mice.16,17 A recent study reported that the hypoglycemic effect is due to the presence of flavanol–fatty alcohol hybrids in A. tsao-ko with α-glucosidase and protein tyrosine phosphatase 1B (PTP1B) dual inhibition.18 However, only a few reports have referred to the identification of flavonoids in A. tsao-ko and their hypoglycemic effect in diabetic rats.
In this work, firstly, the flavonoid components in A. tsao-ko were analyzed by UPLC-MS/MS. Then, the antioxidant activities of A. tsao-ko methanol extracts were detected by oxygen radical absorbance capacity (ORAC), 2,2′-azinobis(3-ethylbenzothiazoline-6-sulfonic acid (ABTS) radical scavenging activity and ferric reducing antioxidant power (FRAP) methods, and the α-amylase and α-glucosidase inhibition abilities were also measured in vitro. Moreover, the antidiabetic properties of ethanol extracts were further investigated using diabetic rats.
2. Materials and methods
2.1. Chemicals
2,2′-Azinobis(3-ethylbenzothiazoline-6-sulfonic acid) diammonium salt (ABTS), 2,4,6-tri(2-pyridyl)-s-triazine (TPTZ), α-glucosidase from Saccharomyces cerevisiae, and streptozotocin (STZ) were purchased from Sigma-Aldrich Co. (St Louis, MO, USA). Quercetin, 4-nitrophenyl-α-D-glucopyranoside (PNPG), α-amylase, 6-hydroxy-2,5,7,8-tetramethylchromane-2-carboxylic acid (Trolox), 2,2′-azobis(2-methylpropionamidine) dihydrochloride (AAPH), 3,5-dinitrosalicylic acid and fluorescein sodium salt were purchased from Aladdin (Shanghai China). Metformin hydrochloride sustained-release tablets were purchased from Beijing Tai-yang Pharmaceutical Co., Ltd, China. Other reagents were all analytical grade.
2.2. Plant materials and preparation of A. tsao-ko extracts
Dried mature fruits were purchased from Zhang Zhongjing Pharmacy (Zhengzhou, China). A. tsao-ko were freeze-dried in a vacuum freeze dryer (LGJ-18B, Beijing, China), pulverized using a high speed shredder (XFB-200, Jishou, China) and sieved (40 mesh). The dried powder materials were stored in a desiccator until further use.
The A. tsao-ko powders were degreased with carbon tetrachloride (w/v = 1
:
10 g mL−1) for 24 h, filtered and dried in the fume hood. The defatted powders were extracted with methanol and ethanol (w/v = 1
:
10 g mL−1) at room temperature for 24 h, respectively. The filtrates obtained from three-time extracted defatted powders were combined and concentrated using a rotary evaporator (YRE2000B, Henan, China). Then the concentrated solutions were frozen at −80 °C in a refrigerator (DW-86L386, Qingdao, China) and freeze-dried in a vacuum freeze dryer. The freeze-dried powder was stored at 4 °C until further use. The methanol freeze-dried powder was used in in vitro experiments and flavonoid component identification. The ethanol extracts were used in animal experiments.
2.3. Determination of the total flavonoid content
The total flavonoid content (TFC) was assessed using the modified Dowd method.19 Briefly, 100 μL of the extract solution from A. tsao-ko or quercetin was added to a 96-well microplate and mixed with 100 μL of 2% AlCl3 methanol solution. The mixture was incubated at 25 °C for 10 min and absorbance was measured at 415 nm with a microplate reader (Spectra Max M2, Shanghai, China). Quercetin was used as the standard substance, and the final result was expressed as mg of quercetin equivalent (QE) per g dry weight (DW).
2.4. Determination of flavonoid compounds of A. tsao-ko extracts by UPLC-MS/MS
Chromatographic separation was performed in a Thermo Ultimate 3000 system equipped with an ACQUITY UPLC® BEH C18 column (100 × 2.1 mm, 1.7 μm, Waters) column maintained at 40 °C. The mobile phases were 0.1% formic acid in water (A) and 0.1% formic acid in acetonitrile (B). The program of gradient elution was as follows: 0–1 min, 20% B; 1–9 min, 20% B-50% B; 9–12 min, 50% B-98% B; 12–13.5 min, 98% B; 13.5–14 min, 98% B-20% B; 14–17 min, 20% B. The injection volume of the sample was 2 μL and the elution flow rate was kept at 0.25 mL min−1. The scanning range of UV detection wavelength was 200–400 nm.
The ESI-MSn experiments were executed on a Thermo Q Exactive Focus mass spectrometer with the spray voltage of 3.5 kV and −2.5 kV in positive and negative modes, respectively. Sheath gas and auxiliary gas were set at 30 and 10 arbitrary units, respectively. The capillary temperature was 325 °C. The analyzer scanned over a mass range of m/z 150–1000 for the full scan at a mass resolution of 70
000. Data dependent acquisition (DDA) MS/MS experiments were performed with a HCD scan. The normalized collision energy was 10 eV, 50 eV and 60 eV. Dynamic exclusion was implemented to remove some unnecessary information in the MS/MS spectra.
2.5. Determination of antioxidant activity
2.5.1. Oxygen radical absorbance capacity (ORAC) assay.
The ORAC assay was performed according to the method of Etsassala et al. with a slight modification.20 20 μL of A. tsao-ko extracts and 150 μL of fluorescein sodium (8.16 × 10−2 μM) were transferred into 96-black well microplates. The plate was covered with a lid and incubated in a preheated (37 °C) multimode reader for 10 min with 3 min of shaking during this time. After adding 25 μL of AAPH solution, the plate was shaken for 1 min immediately. Fluorescence intensity was continuously measured every minute for 1 h at the excitation wavelength and emission set at 485 and 530 nm, respectively. The result was expressed as μM Trolox equivalents per 100 g DW of A. tsao-ko powder (μM TE/100 g DW).
2.5.2. ABTS radical scavenging assay.
The ABTS assay was determined as described by Zhu et al. with slight modifications.21 Briefly, ABTS working solution was prepared by mixing 7 mM ABTS solution and 2.45 mM potassium persulfate solution (1
:
1, v/v) at 25 °C in darkness for 12 h. Ethanol was used to dilute the working solution for the absorbance to reach 0.7 ± 0.02 at 740 nm. Then, 20 μL of the test sample or Trolox standards and 180 μL of ABTS working solution were added into the 96-well plate, reacted at 25 °C in darkness for 3 min and measured at 734 nm subsequently. The scavenging activity was expressed as IC50 value (mg mL−1).
2.5.3. Ferric reducing antioxidant power (FRAP) assay.
The FRAP assay was performed according to the previous method.22 The FRAP reagent was prepared by mixing 0.3 M acetate buffer (PH 3.6), 0.01 M TPTZ and 0.02 M FeCl3 in a ratio of 10
:
1
:
1 (v/v/v). 50 μL of the suitable concentration of sample was mixed with 260 μL FRAP reagent in the 96-well plate and incubated at 37 °C for 30 min. The absorbance was measured at 595 nm. With the standard solution of FeSO4 as the reference substance, the ferric reduction capacity of the extract was calculated, which meant to achieve the required concentration of FeSO4 with the same absorbance. The result was expressed as equivalent to the number of micromoles of Fe2+ per gram of dry weight (μM Fe2+ per g DW).
2.6. Determination of anti-diabetic enzyme inhibitory activity in vitro
2.6.1. α-Amylase inhibition assay.
α-Amylase inhibitory activities of the A. tsao-ko methanol extract was assessed using a previous method with slight modifications.23,24 50 μL of the sample and 50 μL of α-amylase solution (≥2.5 U mL−1) were taken in a centrifuge tube and incubated at 25 °C for 10 min. 50 μL of 0.5% starch solution was added into the tube and incubated at 25 °C for 3 min. 100 μL of DNS colour reagent solution was added and the mixture was boiled for 10 min. Then, 100 μL of the mixture was transferred into another centrifuge tube containing 1400 μL of pure water, mixed and cooled to 25 °C. Finally, 200 μL of the mixture was transferred into a 96-well microplate and the absorbance was recorded at 540 nm. Results were expressed as IC50 value (μg mL−1).
2.6.2. α-Glucosidase inhibition assay.
The procedure of α-glucosidase inhibitory activity was performed following the reported method.25 Briefly, α-glucosidase and PNPG were dissolved in phosphate buffer (0.1 M, pH 6.8) at 0.2 U mL−1 and 2.5 mM, respectively. 8 μL of the sample, 112 μL of the buffer and 20 μL of α-glucosidase solution were mixed in a 96-well microplate and incubated at 37 °C for 15 min. Then, the 20 μL of PNPG solution was added and incubated at 37 °C for 15 min. Finally, the reaction was stopped by the addition of 80 μL of 0.2 M Na2CO3 solution and measured at 405 nm. Methanol and acarbose were negative and positive controls, respectively. The α-glucosidase inhibitory activity was expressed as IC50 value (μg mL−1).
2.7. Determination of antidiabetic activity in vivo
2.7.1. Animals.
Six-week-old male specific-pathogen-free Sprague-Dawley (SD) rats (n = 35) were purchased from the Experimental Animal Center of Henan (SCXK (Yu) 2017-0001, Zhengzhou, China) and acclimated for 1 week. The animal permit number was No. 41003100005143. Animals were housed in a controlled environment (temperature 18–24 °C, humidity 45%, 12 h light/12 h dark cycle) with free access to normal pellet diet and water of the College of Public Health of Zhengzhou University, which permit number was SYXK (Yu) 2018-0005. All animal procedures were performed in accordance with the Guidelines for Care and Use of Laboratory Animals of Zhengzhou University and approved by the Animal Ethics Committee of Zhengzhou University (approval number is ZZUIRB 2021-132).
2.7.2. Development of T2DM and experimental design.
After 1 week of acclimatization, all rats were randomly divided into two groups: a control group (Con, n = 8) and a high-fat diet group (HFD, n = 27). From 2 to 5 weeks, the Con group was fed with a normal pellet diet while the HFD group was fed with a high-fat diet (normal pellet diet 57.4%, sucrose 20%, lard 10%, egg yolk powder 10%, cholesterol 2.5% and bile salts 0.1%).26 In week 6, the rats in the Con group were injected intraperitoneally with citrate buffer and those in the HFD group were injected intraperitoneally with streptozotocin (STZ) solution in citrate buffer (pH 4.4) at 30 mg kg−1. Seventy-two hours after injection, the rats in the HFD group with random blood glucose level ≥16.7 mmol L−1 were selected as T2DM rats. One rat in the Con group died due to the injection of citrate buffer during the model building and 7 rats remained. A total of 21 rats in the HFD group were confirmed to be successfully established as T2DM rats in week 7 for subsequent experiments.
Twenty-one T2DM rats were randomized into three groups (n = 7 per group) as follows: the A. tsao-ko group (T-K), the metformin group (Met) and the model group (Mod). During week 8 to week 13, all rats were given oral intervention once a day, the T-K group was treated freeze-dried powder (100 mg per kg bw), the Met group was given metformin (100 mg per kg bw), and the Mod group was administered with normal saline (100 mg per kg bw). Similarly, the Con group was given normal saline (100 mg per kg bw). Body weight was recorded on the first day of every week and the intervention dose of each rat was adjusted accordingly. The rats in the Con group were fed with a normal pellet diet and in the other three groups were fed a high-fat diet until the end of the experiment. On the last day of the 5th, 7th and 13th week, rats were housed in metabolic cages for 24 h, and the volume of food intake, water intake and urine were measured and the urine specific gravity was calculated. After the experiment, fasting rats were anaesthetized using chloral hydrate solution (7%, 0.5 mL per 100 g bw). Subsequently, 5 mL of abdominal aortic blood sample was collected from each rat and centrifuged at 3000 rpm for 10 min. Serum sample was divided and stored at −80 °C until further use. Pancreases were collected for histopathological examinations.
2.7.3. Oral glucose tolerance test (OGTT).
The OGTT was conducted one day before the sacrifice to assess glucose tolerance ability of rats from every group. After overnight fasting, all animals were orally ingested with a single dose of glucose solution (2 g per kg BW). The blood glucose levels of the tail tip were measured at 0 (baseline), 15, 30, 60, 120 min after the oral ingestion, using a one-touch glucometer (Accu-Chek Active, Roche, Mannheim, Germany). The integrated glucose response (area under the curve, AUC) over a period of 120 min after glucose overload was also calculated.27
2.7.4. Biological analysis.
Fasting blood glucose (FBG), superoxide dismutase (SOD) and malondialdehyde (MDA) in serum were measured using colorimetric methods according to the instructions of commercial kits (Nanjing JianCheng Bioengineering Institute, China). Serum insulin was quantified by an enzyme-linked immunosorbent assay (ELISA) kit (Cusabio Biotech Co., Ltd, China). HOMA (HOMA-IR and HOMA-β) were estimated in terms of FBG and fasting insulin.28
2.7.5. Histopathological analysis.
Pancreatic tissues were removed directly after sacrificing, washed with normal saline and stored in 4% paraformaldehyde for 24 h. Then, the paraffin-embedded tissues of the pancreas were sectioned with 4 μm thickness (Finesse 325, Thermo Fisher Scientific, Shanghai, China) and stained by the hematoxylin–eosin (HE) staining method. Finally, the histological characteristics were observed under an optical microscope.
2.8. Statistical analysis
All experiments were repeated three times and data were presented as mean ± standard deviation (SD). Statistical analysis was performed using SPSS21.0. One-way analysis of variance followed by Tukey's-SHD and Student's t test were performed to assess the statistical significance between different groups. P values < 0.05 were considered statistically significant.
3. Results
3.1. Identification of flavonoid compounds
Flavonoids are phenolic compounds that are the active ingredients found in A. tsao-ko. The TFC from A. tsao-ko of the methanol extract determined by the modified Dowd method was 1.21 mg QE per g DW. Furthermore, UPLC-MS/MS analysis in positive and negative ionization modes was performed to determine the flavonoids of A. tsao-ko. First, their exact molecular weight was confirmed (molecular weight error ≤30 ppm). Then, the fragment information (including the mass nuclear ratio, retention time and peak area) obtained according to the MS/MS mode was matched in the KEGG (http://www.kegg.jp/pathway) and BioDeepDB (http://www.biodeep.cn/) databases to obtain accurate metabolite information. The Plant metabolite annotation toolbox (PlantMAT) (https://sourceforge.net/projects/plantmat/) was also used to predict flavonoids through combinatorial enumeration of aglycone, glycosyl, and acyl subunits.29 Finally, a total of 29 flavonoid constituents were identified in the methanol extract of A. tsao-ko. Procyanidin B1 (1), nobiletin (2), procyanidin B2 (3), epigallocatechin 3-gallate (6), 6-hydroxykaempferol 3-β-rutinoside (7), morin (8), liquiritigenin (10), taxifolin (11), 5,7-dihydroxychromone (14), 3-(3-hydroxyphenyl)-2-phenyl-4-[(E)-2-phenylethenyl]-2,3-dihydro-1-benzofuran-6-ol (15), (+)-catechin (17), bavachin (23), sec-O-glucosylhamaudol (24), polygalaxanthone III (25), isoastilbin (26), isoschaftoside (27), neohesperidin dihydrochalcone (28) and quercetin 3-O-sophoroside-7-O-rhamnoside (29) were detected under positive ionization mode (Fig. 1A). 6′′-O-Acetylgenistin (4), ephedrannin A (5), dihydromyricetin (9), reynoutrin (12), 12a-hydroxyrotenone (13), mulberrin (16), kaempferol (18), isorhamnetin (19), cyclomorusin (20), barbaloin (21) and (−)-epicatechin (22) were identified under negative ionization mode (Fig. 1B). The results are summarised in Table 1 and the chemical structures of flavonoid compounds identified from A. tsao-ko are shown in the ESI Fig. 1.† The results indicated that flavonoid compounds of A. tsao-ko may be responsible for its antioxidant and antidiabetic activities.
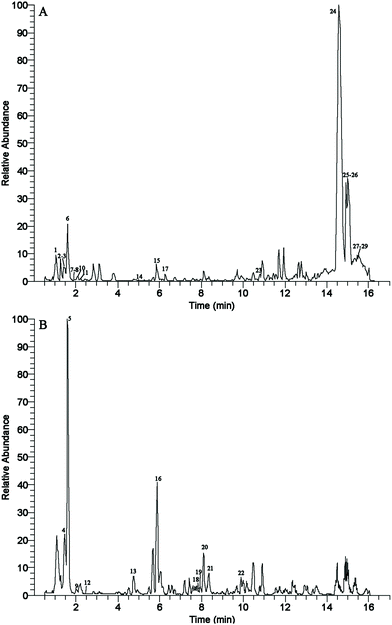 |
| Fig. 1 The base peak chromatogram (BPC) for flavonoid compounds of A. tsao-ko methanol extract in the ESI+ (A) and ESI− (B) modes. | |
Table 1 Identification of flavonoid compounds in A. tsao-ko by UPLC-MS/MS
No. |
RT (min) |
Ion adduction |
Experimental (m/z) |
Theoretical (m/z) |
Error (ppm) |
Fragments (m/z) |
Formula |
Identification |
Compared with the KEGG database (http://www.kegg.jp/pathway).
Compared with the BioDeepDB database (http://www.biodeep.cn/).
Compared with the PlantMAT database (https://sourceforge.net/projects/plantmat/).
|
1 |
1.06 |
[M − H]+ |
579.1498 |
578.1424 |
0.2 |
579.15, 163.04, 139.04, 135.04, 127.04, 123.04 |
C30H26O12 |
Procyanidin B1a,b |
2 |
1.31 |
[M − H]+ |
403.1410 |
402.1315 |
3.9 |
202.09, 188.07, 187.06, 152.06, 150.04, 146.02, 131.97, 118.01, 95.05 |
C21H22O8 |
Nobiletina,b |
3 |
1.37 |
[M − H]+ |
561.1327 |
578.1424 |
12.5 |
393.21, 153.04, 152.06, 150.02 |
C30H26O12 |
Procyanidin B2a,b |
4 |
1.46 |
[M − H]− |
619.1431 |
620.1504 |
4.2 |
624.07, 212.57, 177.02, 165.02, 161.02, 156.21, 151.04, 150.50, 149.02, 137.02, 125.02, 123.04, 109.03, 96.96, 92.42, 81.15, 81.03, 79.64, 78.80, 75.07, 71.81, 53.77, 52.07 |
C23H22O11 |
6′′-O-Acetylgenistinc |
5 |
1.58 |
[M − H]− |
863.1692 |
864.1765 |
1.8 |
243.03, 175.04, 161.02, 149.02, 137.02, 125.02, 123.04, 109.03 |
C30H20O11 |
Ephedrannin Ac |
6 |
1.59 |
[M − H]+ |
591.1333 |
590.1261 |
1.9 |
591.20, 139.04, 123.04, 137.06 |
C22H18O11 |
Epigallocatechin 3-gallatea,c |
7 |
1.88 |
[M − H]+ |
611.1607 |
610.521 |
3.5 |
303.05 |
C27H30O16 |
6-Hydroxykaempferol 3-β-rutinosideb |
8 |
1.88 |
[M − H]+ |
303.0498 |
302.0427 |
0.5 |
303.05 |
C15H10O7 |
Morina,b |
9 |
1.92 |
[M − H]− |
319.0492 |
320.0532 |
10.2 |
193.01, 191.03, 139.04, 125.02, 57.03 |
C15H12O8 |
Dihydromyricetina,b |
10 |
2.01 |
[M − H]+ |
257.0810 |
256.0736 |
0.6 |
183.04, 170.03, 163.06, 147.00, 135.10, 131.97, 118.04, 113.96, 100.03 |
C15H12O4 |
Liquiritigenina,b |
11 |
2.27 |
[M − H]+ |
287.0547 |
304.0583 |
2.9 |
163.08, 150.94, 135.06, 131.99, 118.04, 105.00, 100.02 |
C15H12O7 |
Taxifolina,b |
12 |
2.58 |
[M − H]− |
433.0791 |
434.0849 |
3.0 |
433.08, 271.02, 255.03 |
C20H18O11 |
Reynoutrinb |
13 |
4.76 |
[M − H]− |
555.1882 |
556.1955 |
|
96.96 |
C23H22O7 |
12a-Hydroxyrotenonea,c |
14 |
5.08 |
[M − H]+ |
179.0339 |
178.027 |
0.1 |
133.01, 105.07, 56.97 |
C9H6O4 |
5,7-Dihydroxychromonea,b |
15 |
5.86 |
[M − H]+ |
729.2730 |
728.2657 |
4.9 |
729.26, 577.21, 177.06, 175.04, 163.04, 151.04, 147.04, 139.04, 135.04, 123.04, 107.05 |
C28H22O3 |
3-(3-Hydroxyphenyl)-2-phenyl-4-[(E)-2-phenylethenyl]-2,3-dihydro-1-benzofuran-6-olc |
16 |
5.87 |
[M − H]− |
685.2506 |
686.2579 |
0.6 |
639.30, 287.20, 85.03, 71.01, 59.01 |
C25H26O6 |
Mulberrinc |
17 |
6.33 |
[M − H]+ |
291.0864 |
290.079 |
0.3 |
139.04, 136.06, 123.04 |
C15H14O6 |
(+)-Catechina,b |
18 |
7.69 |
[M − H]− |
285.0408 |
286.0477 |
1.2 |
285.04 |
C15H10O6 |
Kaempferola,b |
19 |
7.93 |
[M − H]− |
315.0506 |
316.0583 |
0.9 |
315.05, 151.00, 107.0 1 |
C16H12O7 |
Isorhamnetina,b |
20 |
8.08 |
[M − H]− |
755.2190 |
756.2263 |
0.3 |
96.96 |
C25H22O6 |
Cyclomorusina,c |
21 |
8.36 |
[M − H]− |
945.2856 |
946.2929 |
2.7 |
873.22, 704.69, 384.09, 219.32, 172.29, 152.70, 124.41, 109.03, 102.55, 101.73, 91.59, 67.74 |
C21H22O9 |
Barbaloina,c |
22 |
9.94 |
[M − H]− |
289.0708 |
290.079 |
2.1 |
289.07, 146.96, 130.98, 125.02, 123.04, 109.03, 97.03 |
C15H14O6 |
(−)-Epicatechina,b |
23 |
10.67 |
[M − H]+ |
325.1435 |
324.1362 |
0.3 |
107.05 |
C20H20O4 |
Bavachina,b |
24 |
14.58 |
[M − H]+ |
921.5643 |
920.5570 |
0.9 |
921.57, 921.73, 920.73, 312.00, 81.07, 67.05 |
C21H26O10 |
Sec-O-glucosylhamaudola,c |
25 |
15.00 |
[M − H]+ |
775.5490 |
774.5419 |
0.0 |
184.07, 125.00, 119.09, 107.09, 105.07, 95.09, 93.07, 91.05, 86.10, 81.07, 79.05, 69.07, 67.05, 55.06 |
C25H28O15 |
Polygalaxanthone IIIc |
26 |
15.00 |
[M − H]+ |
815.5065 |
814.4992 |
3.3 |
816.59, 815.62, 815.48, 814.48, 184.07, 125.00, 86.10, 81.07, 67.06 |
C21H22O11 |
Isoastilbinc |
27 |
15.51 |
[M − H]+ |
856.5828 |
856.5828 |
4.6 |
857.61, 856.73, 95.09, 93.07, 81.07, 79.05, 69.07, 67.05 |
C26H28O14 |
Isoschaftosidec |
28 |
15.51 |
[M − H]+ |
890.6553 |
890.6553 |
0.2 |
891.71, 890.63, 889.63, 107.09, 95.09, 93.07, 83.01, 81.07, 79.05, 69.07, 67.05 |
C28H36O15 |
Neohesperidin dihydrochalconec |
29 |
15.51 |
[M − H]+ |
899.7016 |
898.6943 |
0.7 |
899.72, 898.76, 95.09, 93.07, 81.07, 79.05, 69.07, 67.05 |
C33H40O21 |
Quercetin 3-O-sophoroside-7-O-rhamnosidec |
3.2. Antioxidant properties of A. tsao-ko methanolic extract
3.2.1. Oxygen radical absorbance capacity.
Using Trolox as the positive control, fluorescence attenuation curves of different Trolox concentrations were measured as shown in Fig. 2A. The linear regression analysis between net AUC and Trolox concentrations gave a significant straight line (Y = 0.314X + 0.4957, R2 = 0.9958). The antioxidant capacity of A. tsao-ko was calculated by the standard curve above and net AUC that are shown in Fig. 2B. In the study, the ORAC value was 34
276.57 μM Trolox per 100 g DW expressed by the average ORAC of three concentrations.
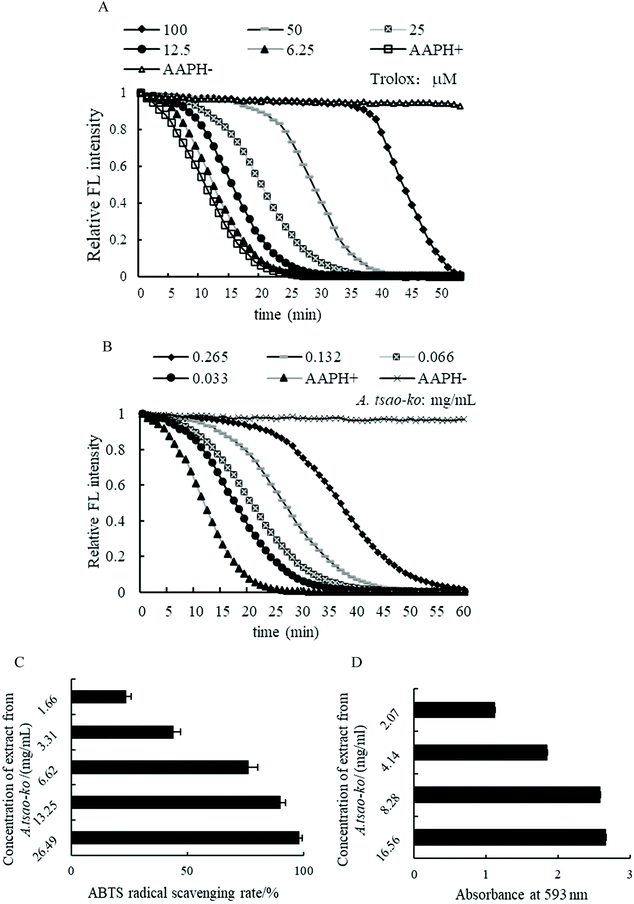 |
| Fig. 2 The antioxidative abilities of methanol extract in A. tsao-ko. Relative fluorescence intensity of Trolox standard solutions with time attenuation diagram curve (A), relative fluorescence intensity of A. tsao-ko extract with time attenuation diagram curve (B), ABTS radical scavenging rate with the concentration of A. tsao-ko (C) and change of absorbance with the concentration of A. tsao-ko (D). | |
3.2.2. ABTS radial scavenging activity.
The result of the ABTS radical scavenging activity of A. tsao-ko methanol extract is presented in Fig. 2C. It showed that the fruit methanol extract exhibited better activity on ABTS free radical when the concentration was increased and the IC50 was 3.30 mg mL−1. The extract might neutralize the ABTS free radicals via both hydrogen atom and single electron transfer.30
3.2.3. Ferric reducing antioxidant power.
The FRAP method measures the total antioxidant activity in the sample and evaluates the ability of the antioxidant to reduce Fe3+-TPTZ to Fe2+. The antioxidant mechanism of FRAP mainly depends on single electron transfer.31 The linear relation between absorbance and FeSO2 concentrations gave a significant straight line (Y = 0.0024X + 0.0864, R2 = 0.999). As shown in Fig. 2D, the absorbance increased as the concentration increased and the FRAP value was 207.42 μM Fe2+ per g DW. A. tsao-ko extract showed important reducing power capacity, which might provide a significant indication of the potential antioxidant activity of the fruits.
3.3. Inhibitory activities of anti-diabetic enzymes in vitro
The hypoglycemic potential of methanol extract from A. tsao-ko was investigated by α-amylase and α-glucosidase inhibitory activity assays (Fig. 3A and B). In this study, the inhibition rate of A. tsao-ko on the two enzymes was concentration dependent. The inhibitory activity on α-amylase (Fig. 3A) and α-glucosidase (Fig. 3B) ranged from 8.14% to 99.46% (concentration range of 2.07 to 132.47 mg mL−1) and 34.06% to 97.44% (concentration range of 1.03 to 8.28 mg mL−1), respectively. The IC50 of α-amylase and α-glucosidase were 14.23 and 1.76 mg mL−1. These results indicated that A. tsao-ko exhibited strong inhibition of α-amylase and α-glucosidase activity.
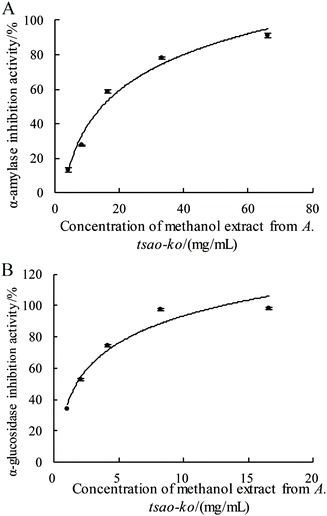 |
| Fig. 3 The determination of α-amylase (A) and α-glucosidase (B) inhibition activity of methanol extract in A. tsao-ko. | |
3.4. Antidiabetic activity of A. tsao-ko treatment in HDF-STZ-induced diabetic rats
3.4.1. Changes of metabolism in rats during the experiment.
Changes in drink, diet, urine volume and urine specific gravity of rats in different groups during the experiment are shown in Table 2. There was no significant difference in the drink and volume of urine in each group before the injection of STZ (the 5th week). However, after the injection of STZ (the 7th week), the Mod group, T-K group and Met group rats displayed a significant (p < 0.01) increase in drink and volume of urine compared with the Con group rats. After intervention of 6 weeks (the 13th week), the drinking water and volume of urine of the Mod group, T-K group and Met group were significantly higher than that of the Con group (p < 0.05). On the other hand, A. tsao-ko-treated rats and metformin-treated rats showed a tendency to reverse the drinking water and volume of urine and this change was significant when compared with the Mod group (p < 0.05). During the modeling period, there was no significant difference in the diet, urine specific gravity and weights of the rats over the course of the experiment. However, after 6 weeks, the weights of the rats in the Mod group continued to decline, which was significantly lower than that of the Con group (p < 0.05), but the intervention of A. tsao-ko and metformin could reverse this weight loss. The results showed that the symptoms of polydipsia, polyphagia, polyuria, weariness and emaciation appeared in the experimental diabetic rats induced by HFD and STZ, which were consistent with the symptoms of diabetic patients.
Table 2 Recordings of water, diet, volume urine, urine specific gravity and weight changes of the rats (n = 7, mean ± SD)
Group |
Drinking water (mL) |
Diet (g) |
Volume urine (mL) |
Urine specific gravity |
Weight (g) |
Con: control group, Mod: model group, T-K: A. tsao-ko group, Met: metformin group. Different superscript letters indicate statistically significant differences between group (p < 0.05). |
The 5th week |
Con |
25.43 ± 2.44a |
20.57 ± 5.41a |
15.17 ± 2.99a |
1.01 ± 0.05a |
385.29 ± 25.18a |
Mod |
30.00 ± 4.00a |
21.00 ± 1.41a |
14.90 ± 3.68a |
1.08 ± 0.08a |
388.75 ± 33.37a |
T-K |
30.50 ± 0.71a |
20.50 ± 2.12a |
16.15 ± 4.17a |
1.03 ± 0.05a |
375.25 ± 33.93a |
Met |
27.00 ± 9.00a |
21.67 ± 0.58a |
15.10 ± 0.95a |
0.98 ± 0.03a |
378.88 ± 29,27a |
The 7th week |
Con |
45.57 ± 4.08a |
32.57 ± 3.64a |
15.90 ± 3.66a |
1.01 ± 0.02a |
410.43 ± 39.67a |
Mod |
150.14 ± 30.68b |
30.43 ± 5.48a |
81.38 ± 28.61b |
1.01 ± 0.04a |
385.00 ± 46.42a |
T-K |
144.14 ± 59.20b |
28.25 ± 6.07a |
84.95 ± 29.46b |
1.01 ± 0.04a |
378.25 ± 36.02a |
Met |
147.50 ± 35.31b |
30.00 ± 7.56a |
83.39 ± 26.66b |
1.02 ± 0.05a |
380.13 ± 29.44a |
The 13th week |
Con |
73.25 ± 20.47b |
23.50 ± 4.36a |
24.04 ± 4.84c |
1.00 ± 0.03a |
512.50 ± 57.55a |
Mod |
180.00 ± 31.34a |
29.60 ± 10.80a |
121.54 ± 21.26a |
1.04 ± 0.07a |
366.17 ± 50.19b |
T-K |
132.57 ± 39.39a |
28.57 ± 10.80a |
81.59 ± 16.35b |
1.02 ± 0.01a |
395.60 ± 48.42b |
Met |
133.88 ± 32.01a |
30.50 ± 10.27a |
98.32 ± 20.95ab |
1.04 ± 0.03a |
405.86 ± 54.37b |
3.4.2. Effects of A. tsao-ko treatment on FBG, insulin and HOMA.
The FBG is the most remarkable and distinct indicator of diabetes detection. As shown in Fig. 4A, the FBG level was lower than in the Mod group (29.28 ± 4.70 mM) when A. tsao-ko (24.76 ± 2.87 mmol L−1, p > 0.05) and metformin (23.74 ± 2.84 mM, p < 0.05) were administered in diabetic rats. As shown in Fig. 4B, there was a slight decrease (p > 0.05) in the insulin level in the T-K group rats compared with that in the Mod group. HOMA-IR of the Con group was significantly (p < 0.01) less than in the other three groups as shown in Fig. 4C. At the same time, HOMA-IR of the T-K group and Met group presented in Fig. 4D was lower than that of the Mod group, although there was no statistical difference (p > 0.05). In addition, the trend of HOMA-S in each group was opposite to that of HOMA-IR.
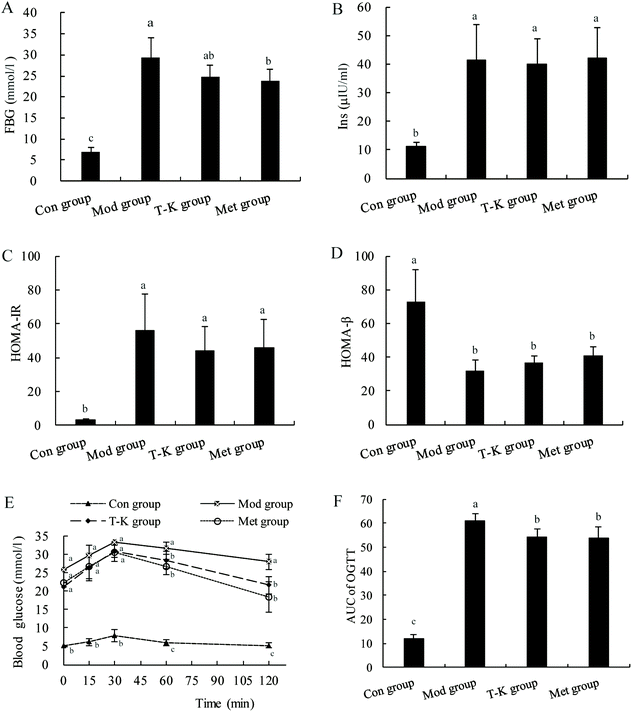 |
| Fig. 4 FBG, insulin, homeostasis model assessment and OGTT in HFD and STZ induced diabetic rats (n = 7, mean ± SD). Fasting plasma glucose (A), insulin (B), HOMA-IR (C), HOMA-β (D), the curve of blood glucose changes with time by OGTT test (E), area under OGTT curve (F). Different superscript letters indicated statistically significant differences between groups (p < 0.05). | |
3.4.3. Effect of A. tsao-ko treatment on OGTT.
As shown in Fig. 4E, intragastric administration of glucose did not cause significant increase in the Con group rats (from 5.28 ± 0.46 to 7.90 ± 1.56 mM), but the Mod group rats displayed severe and significant glucose tolerance impairment (from 26.02 ± 3.09 to 33.30 ± 0.00 mM). Blood glucose level reached the highest point at 30 min and exhibited a descending tendency. After intervention with the A. tsao-ko for six weeks, the T-K group rats showed significant decrease in blood glucose level, indicating that the impairment of diabetic rats could be improved by A. tsao-ko. The AUC of OGTT, as shown in Fig. 4F, was higher in the Mod group than in the T-K group and Met group (p < 0.01).
3.4.4. Effect of A. tsao-ko treatment on SOD and MDA.
As shown in Fig. 5, the activity of total SOD significantly increased (p < 0.01) after 6 weeks of intervention with A. tsao-ko and metformin, compared with rats in the Mod group (Fig. 5A). After the intervention with A. tsao-ko, the serum MDA contents of the T-K group were lower than that in the Mod group (p < 0.01) (Fig. 5B).
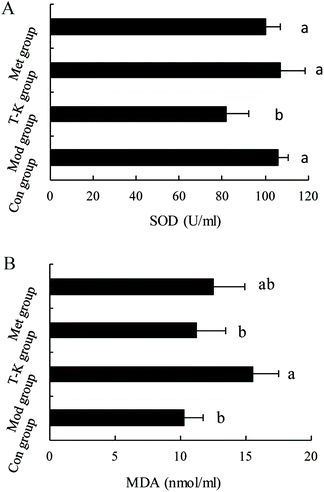 |
| Fig. 5 SOD (A) and MDA (B) in HFD and STZ induced diabetic rats (n = 7, mean ± SD). Different superscript letters indicated statistically significant differences between groups (p < 0.05). | |
3.4.5. Histopathology of the HFD-STZ diabetic rat model.
The effect of A. tsao-ko treatment on pancreatic tissues is shown in Fig. 6. In the Con group (Fig. 6A), the pancreatic islet was oval shaped and well-structured, with clear islet boundaries, full shape and tight arrangement. Compared with the Con group, the Mod group (Fig. 6B) had irregular islet shapes, and some exocrine acinar cells penetrated deep into the islet. However, in the T-K group (Fig. 6C), the islet cell cytoplasm was slightly loose. Meanwhile, there were no significant abnormalities observed in the Met group (Fig. 6D).
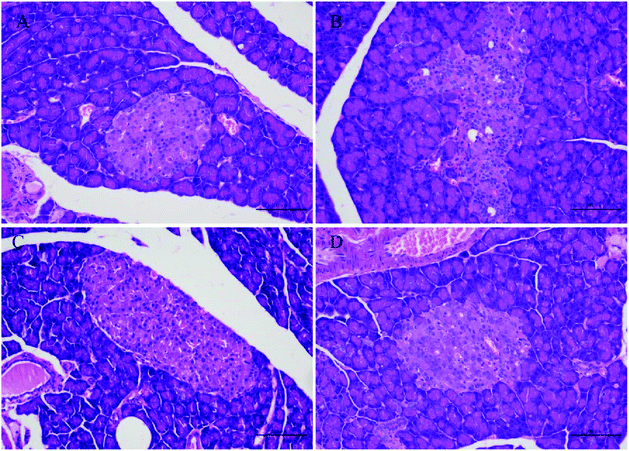 |
| Fig. 6 Effect of A. tsao-ko treatment on pancreatic tissues. (A) Con group, (B) Mod group, (C) T-K group, (D) Met group (scale, 200×). | |
4. Discussions
Flavonoids belong to a group of polyphenols, mainly found in fruits, vegetables, herbs, chocolate, tea, seeds, flowers, and red wine. Flavonoids can be divided into flavanols, flavanones, flavonols, flavones, anthocyanins and isoflavonoids depending on their chemical structure.32 These compounds exhibit high pharmacological activities including antioxidant, antibacterial, hepatoprotective, anti-inflammatory, anti-apoptotic, anti-hyperlipidemic, cardioprotective, and estrogenic effects which are associated with a wide range of health benefits.33,34The antioxidative properties of flavonoids can ameliorate diabetes by acting on biological targets such as α-glucosidase, glucose co-transporter, or aldose reductase.35 Many clinical trials have indicated that a diet abundant in flavonoid-rich foods could be one dietary therapy for the prevention and management of T2DM.36–38 Flavonoids extracted from plants are considered as a potential source of antioxidant molecules, which are associated with a wide range of health benefits.
A. tsao-ko, an important functional food and medicine, has traditionally used for the treatment of digestive and stomach disorders.39 Recent studies have demonstrated that the rich active ingredients contained in A. tsao-ko possess antioxidant, anti-inflammatory, anti-obesity, and anti-proliferative activities.12,13,18,40 In this study, the flavonoid constituents from extract of A. tsao-ko were investigated. Results showed that A. tsao-ko extract has higher TFC and 29 flavonoids were identified by UPLC-MS/MS. Six types of flavonols in A. tsao-ko, including morin (8), dihydromyricetin (9), reynoutrin (12), kaempferol (18), isorhamnetin (19) and isoastilbin (26) were identified. Flavonols are widely distributed flavonoids in foods. Evidences indicated that flavonols can reduce intracellular ROS by activating Nrf2 and superoxide dismutase, thereby improving the oxidative environment.41,42 Three flavanols were identified in A. tsao-ko, namely epigallocatechin 3-gallate (6), (+)-catechin (17) and (−)-epicatechin (22). Studies have shown that flavanols have antioxidant properties to improve metabolic syndrome and related disorders by scavenging free radicals in the body and inhibiting the formation of ROS.41 In addition, 3 flavones, namely nobiletin (2), mulberrin (16) and isoschaftoside (27) and 3 flavanones, namely liquiritigenin (10), taxifolin (11) and bavachin (23) were identified in A. tsao-ko. Flavones and flavanones could ameliorate oxidative stress by lowering ROS levels and augmenting SOD, GPx and CAT activity in vitro.34 In the present study, we also identified flavonoids such as procyanidin B1 (1) and procyanidin B2 (2), 6′′-O-acetylgenistin (4), 6-hydroxykaempferol 3-β-rutinoside (7), cyclomorusin (20), barbaloin (21), quercetin 3-O-sophoroside-7-O-rhamnoside (29). These flavonoids have been shown strong scavenging activity against free radicals such as the stable radical, 1,1 diphenyl-2-picryl-hydrazyl (DPPH), and the oxygen free radicals and HO˙.43 In several studies, kaempferol, (+)-catechin, (−)-epicatechin and taxifolin were reported in extracts of A. tsao-ko,11,44–48 which is consistent with this research. These results suggested that the antioxidant and antidiabetic effects of A. tsao-ko may be attributed to the biological activities of the flavonoids components.
STZ-induced DM is characterized by polyphagia, polyuria, polydipsia and severe loss in body weight, which associated with the β-cell cytotoxic effects of STZ.49 In this experiment, compared with control rats, diabetic rats displayed a significant increase in drink and volume of urine during the modelling period. After the intervention of A. tsao-ko and metformin, there was a tendency of reversal in drinking water and volume of urine. In addition, the extract of the A. tsao-ko reduced the decline in body weight in diabetic rats. Previous studies on anti-diabetic have also obtained similar results, indicating its anti-diabetic effect.50,51 The results of our study have shown that the administration of extracts of the A. tsao-ko could improve the clinical symptoms of T2DM and have anti-diabetic effects.
It is well known that oxidative stress plays an important role in hyperglycemia-induced tissue injury and in the development of T2DM, which can lead to a decrease in the body's antioxidant defense system or an increase in the production of reactive oxygen species.52,53 According to the previous literature, the essential oil of A. tsao-ko showed weak antioxidant effect by the DPPH radical assay, thiobarbituric acid (TBA) test and FRAP assay.54 The methanol extract of A. tsao-ko possessed high DPPH scavenging activities as part of its antioxidant activity.55 Phenolic components, especially flavonoids, contributed significantly to the antioxidant properties of the medicinal herbs.56 In this study, the antioxidant activities of A. tsao-ko extract in vitro were determined by ORAC, ABTS and FRAP methods. The results showed that the flavonoids in A. tsao-ko have strong oxygen radical absorbance capacity, ABTS radical scavenging activity and ferric reducing antioxidant power, which might provide a significant indication of the potential antioxidant activity of A. tsao-ko.
The digestive enzyme inhibition activities of A. tsao-ko extract was also studied. The α-amylase and α-glucosidase inhibitory activity assays are widely used to determine the hypoglycemic effects of bioactive compounds from natural sources.57A. tsao-ko also exhibited effective α-amylase and α-glucosidase inhibition activities in vitro, suggesting the promising antidiabetic effect of A. tsao-ko. Consumption of foods rich in flavonoids and polyphenols may delay the decomposition of polysaccharides by inhibiting the activity of related enzymes, thereby reducing glucose absorption.5 Previous studies have shown that 2,6-epoxydiarylheptanoids and diarylheptanoids isolated from the fruits of A. tsao-ko have α-glucosidase inhibitory activity.12,40 The presence of flavanol–fatty alcohol hybrids in A. tsao-ko with α-glucosidase and PTP1B dual inhibition.18 These results provide new insights for the use of A. tsao-ko as an anti-diabetic adjuvant.
In our work, the T2DM rat model was established to further assess the hypoglycemic effect of A. tsao-ko. The results showed that the significantly higher HOMA-IR score and the lower HOMA-β score in the T-K group compared with the Con group confirmed that the HFD-STZ-induced insulin resistance and partial pancreatic β cell dysfunction. After treatment, the blood glucose level of diabetic rats induced by HFD and STZ was decreased, indicating the hypoglycemic activity of A. tsao-ko. Previous studies had reported that diabetic rats showed similar reductions in FBG levels after administration of extracts from other medicinal plants.58,59 This might be due to extracts of polyphenolic chemicals such as epicatechin, catechol and other flavonoids.60 Moreover, this antidiabetic ability might be due to the inhibitory effect of A. tsao-ko on the activity of α-amylase and α-glucosidase. The mechanisms of lowering blood glucose mainly included stimulation of pancreatic islet β-cells to release insulin, inhibition of hormone release that raises blood glucose, an increase the number, affinity or sensitivity of insulin receptors, and stimulation of glucose by using peripheral organs.61 Further studies are needed to explore the possible mechanisms of A. tsao-ko in lowering blood glucose. OGTT is used to assess glucose metabolism, and it shows improved glucose tolerance in A. tsao-ko extract-treated rats, which might be related to the fact that the flavonoid content of A. tsao-ko could chelate sugars, lipids, and fibers and inhibit the glucose transporter.62 Possible mechanism is that the extract of A. tsao-ko extract may increase the sensitivity of β-cell insulin receptors in diabetic rats.
Studies have shown that persistent hyperglycemia of diabetes also leads to an increase in oxidative stress in the body. Antioxidant therapy has attractive benefits in preventing or delaying diabetes.63 SOD, a well-known antioxidant enzyme, plays an important role in safeguarding the cells against the oxidative stress. An increase in the content of MDA indicates an increase in the level of lipid peroxidation in the body, which causes oxidative stress.64,65 In our results, the extract of A. tsao-ko significantly restored the changes of antioxidant levels by improving the level of SOD and decreasing MDA in diabetic rats, which can increase the response of antioxidant defense systems to oxidative stress and might be one of the possible reasons for its antidiabetic effect. Oxidative stress is defined as the unbalanced metabolite or ROS between the generation and reactivity of free radicals and its elimination through protective mechanisms, including antioxidants. The Kelch-like ECH-related protein 1 (Keap1)-nuclear factor erythrocyte 2 related factor 2 (Nrf2)-antioxidant response element (ARE) pathway is the center of the day-to-day biological response to oxidative stress. It regulates the transcription of many antioxidant genes that maintain cellular homeostasis.66 Therefore, we hypothesize that the possible mechanism may be that the flavonoids contained in the A. tsao-ko upregulated the expression of Nrf2 and downstream antioxidant genes HO-1, SOD through the Keap1-Nrf2/ARE signaling pathway and thereby exerted its antioxidant effect. Nevertheless, further research on the antioxidant effect and improvement of oxidative stress should be carried out to explore its specific antioxidant mechanism. Histological examination showed that diabetes induces structural disturbances in the pancreatic tissue of rats. In line with our study, researchers have shown pancreatic tissue structure alterations in diabetes, such as the shrinkage in the size of the islets, disrupted islet architecture and destruction of β-cells, reduction of β-cell cytoplasmic mass as well as atrophy and degranulation of β-cells with pronounced vacuolation and pycnotic cell nuclei.67 In this study, histopathological analysis of the pancreatic tissue revealed that treatment with A. tsao-ko ameliorates the structural damage of pancreatic tissue caused by diabetes in diabetic rats.
5. Conclusions
In this study, the flavonoid components of A. tsao-ko were investigated by employing UPLC-MS/MS for the first time and a total of 29 flavonoids were identified. Further biological activity research found that extracts of A. tsao-ko showed great antioxidant activity and α-amylase and α-glucosidase inhibition activity in vitro. The results of animal experiments showed that A. tsao-ko improved the antioxidant ability and exhibited anti-diabetic ability in diabetic rats. The findings in this work suggested that the flavonoid components from A. tsao-ko have great potential to be used as antidiabetic reagents. Further studies are required to identify the components with higher observed bioactivity and elucidate the detailed mechanism of action.
Author contributions
Xiao-Feng Zhang: conceptualization, investigation, visualization, data curation, writing – original draft, writing – reviewing and editing. Yu-Jun Tang: investigation, visualization, formal analysis, data curation, writing – original draft. Xiao-Xian Guan: investigation, visualization, formal analysis, data curation, writing – original draft. Xin Lu: investigation, visualization, writing – original draft. Jiao Li: investigation, visualization, writing – original draft. Xiao-Li Chen: data curation, writing – original draft. Jin-Lan Deng: data curation, writing – original draft. Jian-Ming Fan: supervision, writing – reviewing & editing.
Conflicts of interest
The authors declare that there is no financial interest relevant to this article.
Acknowledgements
This research was financially supported by the National Natural Science Foundation of China (31372453) and Science and Technology Department of Henan Province (152102310260).
References
- E. R. Pearson, Type 2 diabetes: a multifaceted disease, Diabetologia, 2019, 62, 1107–1112 CrossRef PubMed.
- L. M. M. Janssen, M. Hiligsmann, A. M. J. Elissen, M. A. Joore, N. C. Schaper, J. H. A. Bosma, C. D. A. Stehouwer, S. J. S. Sep, A. Koster, M. T. Schram and S. Evers, Burden of disease of type 2 diabetes mellitus: cost of illness and quality of life estimated using the Maastricht Study, Diabetic Med., 2020, 37, 1759–1765 CAS.
- Y. Tan, S. K. C. Chang and Y. Zhang, Comparison of alpha-amylase, alpha-glucosidase and lipase inhibitory activity of the phenolic substances in two black legumes of different genera, Food Chem., 2017, 214, 259–268 CrossRef CAS PubMed.
- R. R. Holman, R. L. Coleman, J. C. N. Chan, J. L. Chiasson, H. Feng, J. Ge, H. C. Gerstein, R. Gray, Y. Huo, Z. Lang, J. J. McMurray, L. Ryden, S. Schroder, Y. Sun, M. J. Theodorakis, M. Tendera, L. Tucker, J. Tuomilehto, Y. Wei, W. Yang, D. Wang, D. Hu and C. Pan, Effects of acarbose on cardiovascular and diabetes outcomes in patients with coronary heart disease and impaired glucose tolerance (ACE): a randomised, double-blind, placebo-controlled trial, Lancet Diabetes Endocrinol., 2017, 5, 877–886 CrossRef CAS.
- S. Jiang, X. Zhao, C. Liu, Q. Dong, L. Mei, C. Chen, Y. Shao, Y. Tao and H. Yue, Identification of phenolic compounds in fruits of Ribes stenocarpum Maxim. By UHPLC-QTOF/MS and their hypoglycemic effects in vitro and in vivo, Food Chem., 2021, 344, 128568 CrossRef CAS PubMed.
- P. A. Gerber and G. A. Rutter, The Role of Oxidative Stress and Hypoxia in Pancreatic Beta-Cell Dysfunction in Diabetes Mellitus, Antioxid. Redox Signal., 2017, 26, 501–518 CrossRef CAS PubMed.
- V. U. Nna, A. B. Abu Bakar, A. Ahmad, C. O. Eleazu and M. Mohamed, Oxidative Stress, NF-kappaB-Mediated Inflammation and Apoptosis in the Testes of Streptozotocin-Induced Diabetic Rats: Combined Protective Effects of Malaysian Propolis and Metformin, Antioxidants, 2019, 8, 465 CrossRef PubMed.
- H. Yaribeygi, T. Sathyapalan, S. L. Atkin and A. Sahebkar, Molecular Mechanisms Linking Oxidative Stress and Diabetes Mellitus, Oxid. Med. Cell. Longevity, 2020, 2020, 8609213 Search PubMed.
- S. Bekeschus, L. Brautigam, K. Wende and E. M. Hanschmann, Oxidants and Redox Signaling: Perspectives in Cancer Therapy, Inflammation, and Plasma Medicine, Oxid. Med. Cell. Longevity, 2017, 2017, 4020253 Search PubMed.
- M. R. Rahman, Z. Lou, F. Yu, P. Wang and H. Wang, Anti-quorum sensing and anti-biofilm activity of Amomum tsaoko (Amommum tsao-ko Crevost et Lemarie) on foodborne pathogens, Saudi J. Biol. Sci., 2017, 24, 324–330 CrossRef PubMed.
- T. T. Zhang, C. L. Lu and J. G. Jiang, Bioactivity evaluation of ingredients identified from the fruits of Amomum tsaoko Crevost et Lemaire, a Chinese spice, Food Funct., 2014, 5, 1747–1754 RSC.
- X. F. He, X. K. Zhang, C. A. Geng, J. Hu, X. M. Zhang, Y. Q. Guo and J. J. Chen, Tsaokopyranols A-M, 2,6-epoxydiarylheptanoids from Amomum tsao-ko and their alpha-glucosidase inhibitory activity, Bioorg. Chem., 2020, 96, 103638 CrossRef CAS PubMed.
- J. G. Kim, H. Jang, T. P. L. Le, H. R. Hong, M. K. Lee, J. T. Hong, D. Lee and B. Y. Hwang, Pyranoflavanones and Pyranochalcones from the Fruits of Amomum tsao-ko, J. Nat. Prod., 2019, 82, 1886–1892 CrossRef CAS PubMed.
- M. S. Kim, E. K. Ahn, S. S. Hong and J. S. Oh, 2,8-Decadiene-1,10-Diol Inhibits Lipopolysaccharide-Induced Inflammatory Responses Through Inactivation of Mitogen-Activated Protein Kinase and Nuclear Factor-kappaB Signaling Pathway, Inflammation, 2016, 39, 583–591 CrossRef CAS PubMed.
- Q. Cui, L. T. Wang, J. Z. Liu, H. M. Wang, N. Guo, C. B. Gu and Y. J. Fu, Rapid extraction of Amomum tsao-ko essential oil and determination of its chemical composition, antioxidant and antimicrobial activities, J. Chromatogr. B: Anal. Technol. Biomed. Life Sci., 2017, 1061–1062, 364–371 CrossRef CAS PubMed.
- L. Q. Yu and H. Suzuki, Effects of tsao-ko, turmeric and garlic on body fat content and plasma lipid glucose and liver lipid levels in mice (A comparative study of spices), Food Sci. Technol. Res., 2007, 13, 241–246 CrossRef CAS.
- L. Q. Yu, N. Shirai, H. Suzuki, N. Sugane, T. Hosono, Y. Nakajima, M. Kajiwara and K. Takatori, The effect of methanol extracts of tsao-ko (Amomum tsao-ko Crevost et Lemaire) on digestive enzyme and antioxidant activity in vitro, and plasma lipids and glucose and liver lipids in mice, J. Nutr. Sci. Vitaminol., 2010, 56, 171–176 CrossRef CAS PubMed.
- X. F. He, J. J. Chen, T. Z. Li, X. K. Zhang, Y. Q. Guo, X. M. Zhang, J. Hu and C. A. Geng, Nineteen New Flavanol-Fatty Alcohol Hybrids with alpha-Glucosidase and PTP1B Dual Inhibition: One Unusual Type of Antidiabetic Constituent from Amomum tsao-ko, J. Agric. Food Chem., 2020, 68, 11434–11448 CrossRef CAS PubMed.
- E. Valdes, C. Gonzalez, K. Diaz, Y. Vasquez-Martinez, C. Mascayano, C. Torrent, F. Cabezas, S. Mejias, M. Montoya, M. Cortez-San Martin, M. A. Munoz, P. Joseph-Nathan, M. Osorio and L. Taborga, Biological Properties and Absolute Configuration of Flavanones From Calceolaria thyrsiflora Graham, Front. Pharmacol., 2020, 11, 1125 CrossRef CAS PubMed.
- N. Etsassala, J. A. Badmus, J. L. Marnewick, E. I. Iwuoha, F. Nchu and A. A. Hussein, Alpha-Glucosidase and Alpha-Amylase Inhibitory Activities, Molecular Docking, and Antioxidant Capacities of Salvia aurita Constituents, Antioxidants, 2020, 9, 1149 CrossRef CAS PubMed.
- L. Zhu, W. Li, Z. Deng, H. Li and B. Zhang, The Composition and Antioxidant Activity of Bound Phenolics in Three Legumes, and Their Metabolism and Bioaccessibility of Gastrointestinal Tract, Foods, 2020, 9, 1816 CrossRef CAS PubMed.
- T. Diep, C. Pook and M. Yoo, Phenolic and Anthocyanin Compounds and Antioxidant Activity of Tamarillo (Solanum betaceum Cav.), Antioxidants, 2020, 9, 169 CrossRef CAS PubMed.
- M. S. Al-Omar, H. A. Mohammed, S. A. A. Mohammed, E. Abd-Elmoniem, Y. I. Kandil, H. M. Eldeeb, S. Chigurupati, G. M. Sulaiman, H. K. Al-Khurayyif, B. S. Almansour, P. M. Suryavamshi and R. A. Khan, Anti-Microbial, Anti-Oxidant, and alpha-Amylase Inhibitory Activity of Traditionally-Used Medicinal Herbs: A Comparative Analyses of Pharmacology, and Phytoconstituents of Regional Halophytic Plants’ Diaspora, Molecules, 2020, 25, 5457 CrossRef CAS PubMed.
- T. Noreen, M. Taha, S. Imran, S. Chigurupati, F. Rahim, M. Selvaraj, N. H. Ismail, J. I. Mohammad, H. Ullah, M. T. Javid, F. Nawaz, M. Irshad and M. Ali, Synthesis of alpha amylase inhibitors based on privileged indole scaffold, Bioorg. Chem., 2017, 72, 248–255 CrossRef CAS PubMed.
- H. Zhou, H. M. Li, Y. M. Du, R. A. Yan, S. Y. Ou, T. F. Chen, Y. Wang, L. X. Zhou and L. Fu, C-geranylated flavanones from YingDe black tea and their antioxidant and alpha-glucosidase inhibition activities, Food Chem., 2017, 235, 227–233 CrossRef CAS PubMed.
- C. Luo, H. Yang, C. Tang, G. Yao, L. Kong, H. He and Y. Zhou, Kaempferol alleviates insulin resistance via hepatic IKK/NF-kappaB signal in type 2 diabetic rats, Int. Immunopharmacol., 2015, 28, 744–750 CrossRef CAS PubMed.
- M. N. Bin-Jumah, Antidiabetic Effect of Monolluma quadrangula Is Mediated via Modulation of Glucose Metabolizing Enzymes, Antioxidant Defenses, and Adiponectin in Type 2 Diabetic Rats, Oxid. Med. Cell. Longevity, 2019, 2019, 6290143 Search PubMed.
- T. M. Wallace, J. C. Levy and D. R. Matthews, Use and abuse of HOMA modeling, Diabetes Care, 2004, 27, 1487–1495 CrossRef PubMed.
- F. Qiu, D. D. Fine, D. J. Wherritt, Z. Lei and L. W. Sumner, PlantMAT: A Metabolomics Tool for Predicting the Specialized Metabolic Potential of a System and for Large-Scale Metabolite Identifications, Anal. Chem., 2016, 88, 11373–11383 CrossRef CAS PubMed.
- D. M. Kasote, S. S. Katyare, M. V. Hegde and H. Bae, Significance of antioxidant potential of plants and its relevance to therapeutic applications, Int. J. Biol. Sci., 2015, 11, 982–991 CrossRef CAS PubMed.
- D. Huang, B. Ou and R. L. Prior, The chemistry behind antioxidant capacity assays, J. Agric. Food Chem., 2005, 53, 1841–1856 CrossRef CAS PubMed.
- X. F. Guo, Y. Ruan, Z. H. Li and D. Li, Flavonoid subclasses and type 2 diabetes mellitus risk: a meta-analysis of prospective cohort studies, Crit. Rev. Food Sci. Nutr., 2019, 59, 2850–2862 CrossRef CAS PubMed.
- A. Durazzo, M. Lucarini, E. B. Souto, C. Cicala, E. Caiazzo, A. A. Izzo, E. Novellino and A. Santini, Polyphenols: A concise overview on the chemistry, occurrence, and human health, Phytother. Res., 2019, 33, 2221–2243 CrossRef PubMed.
- A. L. Matos, D. F. Bruno, A. F. Ambrosio and P. F. Santos, The Benefits of Flavonoids in Diabetic Retinopathy, Nutrients, 2020, 12, 3169 CrossRef CAS PubMed.
- J. Y. Fang, C. H. Lin, T. H. Huang and S. Y. Chuang, In Vivo Rodent Models of Type 2 Diabetes and Their Usefulness for Evaluating Flavonoid Bioactivity, Nutrients, 2019, 11, 530 CrossRef CAS PubMed.
- R. M. van Dam, N. Naidoo and R. Landberg, Dietary flavonoids and the development of type 2 diabetes and cardiovascular diseases: review of recent findings, Curr. Opin. Lipidol., 2013, 24, 25–33 CrossRef CAS PubMed.
- Y. Kim, J. B. Keogh and P. M. Clifton, Polyphenols and Glycemic Control, Nutrients, 2016, 8, 17 CrossRef PubMed.
- N. P. Bondonno, F. Dalgaard, K. Murray, R. J. Davey, C. P. Bondonno, A. Cassidy, J. R. Lewis, C. Kyro, G. Gislason, A. Scalbert, A. Tjonneland and J. M. Hodgson, Higher Habitual Flavonoid Intakes Are Associated With A Lower Incidence Of Diabetes, J. Nutr., 2021, 151, 3533–3542 CrossRef PubMed.
- S. S. Hong, J. H. Lee, Y.-H. Choi, W. Jeong, E.-K. Ahn, S. H. Lym and J. S. Oh, Amotsaokonal A–C, benzaldehyde and cycloterpenal from Amomum tsao-ko, Tetrahedron Lett., 2015, 56, 6681–6684 CrossRef CAS.
- X. F. He, H. M. Wang, C. A. Geng, J. Hu, X. M. Zhang, Y. Q. Guo and J. J. Chen, Amomutsaokols A-K, diarylheptanoids from Amomum tsao-ko and their alpha-glucosidase inhibitory activity, Phytochemistry, 2020, 177, 112418 CrossRef CAS PubMed.
- J. Ouyang, K. Zhu, Z. Liu and J. Huang, Prooxidant Effects of Epigallocatechin-3-Gallate in Health Benefits and Potential Adverse Effect, Oxid. Med. Cell. Longevity, 2020, 2020, 9723686 Search PubMed.
- D. Kothari, W. D. Lee and S. K. Kim, Allium Flavonols: Health Benefits, Molecular Targets, and Bioavailability, Antioxidants, 2020, 9, 888 CrossRef CAS PubMed.
- B. Li, R. Fu, H. Tan, Y. Zhang, W. Teng, Z. Li and J. Tian, Characteristics of the interaction mechanisms of procyanidin B1 and procyanidin B2 with protein tyrosine phosphatase-1B: Analysis by kinetics, spectroscopy methods and molecular docking, Spectrochim. Acta, Part A, 2021, 259, 119910 CrossRef CAS PubMed.
- T. S. Martin, H. Kikuzaki, M. Hisamoto and N. Nakatani, Constituents of Amomum tsao-koand Their Radical Scavenging and Antioxidant Activities, J. Am. Oil
Chem. Soc., 2000, 77, 667 CrossRef CAS.
- J. Jiahong, C. Zhihong and C. Daofeng, Two new compounds and anti-complementary constituents from Amomum tsao-ko, Nat. Prod. Commun., 2013, 12, 1715–1718 Search PubMed.
- J. S. Shin, S. Ryu, D. S. Jang, Y. W. Cho, E. K. Chung and K. T. Lee, Amomum tsao-ko fruit extract suppresses lipopolysaccharide-induced inducible nitric oxide synthase by inducing heme oxygenase-1 in macrophages and in septic mice, Int. J. Exp. Pathol., 2015, 96, 395–405 CrossRef CAS PubMed.
- K. S. Shim, Y. H. Hwang, S. A. Jang, T. Kim and H. Ha, Ethanol Extract of Amomum tsao-ko Ameliorates Ovariectomy-Induced Trabecular Loss and Fat Accumulation, Molecules, 2021, 26, 784 CrossRef CAS PubMed.
- L. Liu, Y. Zhao, J. Ming, J. Chen, G. Zhao, Z. Y. Chen, Y. Wang and L. Lei, Polyphenol extract and essential oil of Amomum tsao-ko equally alleviate hypercholesterolemia and modulate gut microbiota, Food Funct., 2021, 12, 12008–12021 RSC.
- B. L. Furman, Streptozotocin-Induced Diabetic Models in Mice and Rats, Curr. Protoc. Pharmacol., 2015, 70, 5.47.1–5.47.20 Search PubMed.
- R. B. Jia, Z. R. Li, J. Wu, Z. R. Ou, Q. Zhu, B. Sun, L. Lin and M. Zhao, Physicochemical properties of polysaccharide fractions from Sargassum fusiforme and their hypoglycemic and hypolipidemic activities in type 2 diabetic rats, Int. J. Biol. Macromol., 2020, 147, 428–438 CrossRef CAS PubMed.
- Y. Li, D. Chen, C. Xu, Q. Zhao, Y. Ma, S. Zhao and C. Chen, Glycolipid metabolism and liver transcriptomic analysis of the therapeutic effects of pressed degreased walnut meal extracts on type 2 diabetes mellitus rats, Food Funct., 2020, 11, 5538–5552 RSC.
- K. Susztak, A. C. Raff, M. Schiffer and E. P. Bottinger, Glucose-induced reactive oxygen species cause apoptosis of podocytes and podocyte depletion at the onset of diabetic nephropathy, Diabetes, 2006, 55, 225–233 CrossRef CAS PubMed.
- T. F. Miranda Pedroso, T. R. Bonamigo, J. da Silva, P. Vasconcelos, J. M. Felix, C. A. L. Cardoso, R. I. C. Souza, A. C. Dos Santos, C. R. F. Volobuff, A. S. N. Formagio and V. D. K. Trichez, Chemical constituents of Cochlospermum regium (Schrank) Pilg. root and its antioxidant, antidiabetic, antiglycation, and anticholinesterase effects in Wistar rats, Biomed. Pharmacother., 2019, 111, 1383–1392 CrossRef PubMed.
- Y. Yang, Y. Yue, Y. Runwei and Z. Guolin, Cytotoxic, apoptotic and antioxidant activity of the essential oil of Amomum tsao-ko, Bioresour. Technol., 2010, 101, 4205–4211 CrossRef CAS PubMed.
- T.-T. Zhang, C.-L. Lu and J.-G. Jiang, Antioxidant and anti-tumour evaluation of compounds identified from fruit of Amomum tsaoko Crevost et Lemaire, J. Funct. Foods, 2015, 18, 423–431 CrossRef CAS.
- M. Yu, I. Gouvinhas, J. Rocha and A. Barros, Phytochemical and antioxidant analysis of medicinal and food plants towards bioactive food and pharmaceutical resources, Sci. Rep., 2021, 11, 10041 CrossRef CAS PubMed.
- Y. Y. Wang, J. Zhu, H. Ma, Z. C. Ding, L. Li and J. K. Yan, Antidiabetic activity of a polysaccharide-protein complex from Asian Clam (Corbicula fluminea) in streptozotoxin-induced diabetic rats and its underlying mechanism, Food Funct., 2019, 10, 5574–5586 RSC.
- O. L. Erukainure, O. A. Oyebode, O. M. Ijomone, C. I. Chukwuma, N. A. Koorbanally and M. S. Islam, Raffia palm (Raphia hookeri G. Mann & H. Wendl) wine modulates glucose homeostasis by enhancing insulin secretion and inhibiting redox imbalance in a rat model of diabetes induced by high fructose diet and streptozotocin, J. Ethnopharmacol., 2019, 237, 159–170 CrossRef CAS PubMed.
- D. Tang, L. Liu, D. Ajiakber, J. Ye, J. Xu, X. Xin and H. A. Aisa, Anti-diabetic Effect of Punica granatum Flower Polyphenols Extract in Type 2 Diabetic Rats: Activation of Akt/GSK-3beta and Inhibition of IRE1alpha-XBP1
Pathways, Front. Endocrinol., 2018, 9, 586 CrossRef PubMed.
- T. T. Zhang, C. L. Lu and J. G. Jiang, Bioactivity evaluation of ingredients identified from the fruits of Amomum tsaoko Crevost et Lemaire, a Chinese spice, Food Funct., 2014, 5, 1747–1754 RSC.
- O. Dzydzan, I. Bila, A. Z. Kucharska, I. Brodyak and N. Sybirna, Antidiabetic effects of extracts of red and yellow fruits of cornelian cherries (Cornus mas L.) on rats with streptozotocin-induced diabetes mellitus, Food Funct., 2019, 10, 6459–6472 RSC.
- M. L. Castro-Acosta, S. G. Stone, J. E. Mok, R. K. Mhajan, C. I. Fu, G. N. Lenihan-Geels, C. P. Corpe and W. L. Hall, Apple and blackcurrant polyphenol-rich drinks decrease postprandial glucose, insulin and incretin response to a high-carbohydrate meal in healthy men and women, J. Nutr. Biochem., 2017, 49, 53–62 CrossRef CAS PubMed.
- Z. Chen, C. Wang, Y. Pan, X. Gao and H. Chen, Hypoglycemic and hypolipidemic effects of anthocyanins extract from black soybean seed coat in high fat diet and streptozotocin-induced diabetic mice, Food Funct., 2018, 9, 426–439 RSC.
- X. Zhang, S. Jing, H. Lin, W. Sun, W. Jiang, C. Yu, J. Sun, C. Wang, J. Chen and H. Li, Anti-fatigue effect of anwulignan via the NRF2 and PGC-1alpha signaling pathway in mice, Food Funct., 2019, 10, 7755–7766 RSC.
- K. Kaleshkumar, R. Rajaram, N. Gayathri, T. Sivasudha, G. Arun, G. Archunan, B. Gulyas and P. Padmanabhan, Muscle extract of Arothron immaculatus regulates the blood glucose level and the antioxidant system in high-fat diet and streptozotocin induced diabetic rats, Bioorg. Chem., 2019, 90, 103072 CrossRef CAS PubMed.
- W. Tu, H. Wang, S. Li, Q. Liu and H. Sha, The Anti-Inflammatory and Anti-Oxidant Mechanisms of the Keap1/Nrf2/ARE Signaling Pathway in Chronic Diseases, Aging Dis., 2019, 10, 637–651 CrossRef PubMed.
- H. Oghbaei, G. Hamidian, M. R. Alipour, S. Alipour and R. Keyhanmanesh, The effect of prolonged dietary sodium nitrate treatment on the hypothalamus-pituitary-gonadal axis and testicular structure and function in streptozotocin-induced diabetic male rats, Food Funct., 2020, 11, 2451–2465 RSC.
Footnote |
† Electronic supplementary information (ESI) available. See DOI: 10.1039/d1fo02974f |
|
This journal is © The Royal Society of Chemistry 2022 |