DOI:
10.1039/D1FO00576F
(Paper)
Food Funct., 2021,
12, 6793-6808
Ginsenoside extract from ginseng extends lifespan and health span in Caenorhabditis elegans†
Received
24th February 2021
, Accepted 27th May 2021
First published on 10th June 2021
Abstract
Nutrition intervention has become a potential strategy to improve healthspan and prolong lifespan. Ginseng has been used for thousands of years and developed as a functional food to provide various protective effects to humans. An extract of total ginsenosides (TGS), a mixture of the main active ginsenosides from ginseng, has wide biological activities and health benefits for age-related diseases, including antioxidation and improvements in mitochondrial function. However, the molecular mechanism of TGS for prolonging lifespan and improving fitness and how exactly this is achieved under normal and stress conditions remain largely unclear. In this study, wild-type and mutant C. elegans strains are used to investigate the role and molecular mechanism of TGS-mediated longevity, health benefits, and stress resistance. The results showed that treatment with TGS at 0.2 mg mL−1 from the stage of day four to death significantly extended the lifespan of worms by 14.02% without effects on bacterial metabolism and food intake. Furthermore, TGS treatment obviously improved age-associated mobility, muscle fiber organization, lipofuscin accumulation, and enhanced resistance under oxidative stress. Importantly, these effects of TGS were achieved by activating the signaling pathways of anti-oxidant regulation and longevity, including the NRF2/SKN-1, SIRT1/SIR 2.1, and FOXO/DAF-16 signaling pathways. Finally, it was found that Rg1, Re, and Rb1 were the major effective components of anti-oxidative activity and longevity. Collectively, the protective effect of ginsenoside extract in healthy aging and stress responses provides new insights for the development and practical application of ginseng functional products.
1. Introduction
With changes in diets, environments, and lifestyles in modern society, aging is a major international concern that presents formidable challenges to healthcare systems.1 The physiological and pathological effects of aging, including shortened life, dyskinesia, and decreased stress resistance, contribute to a considerable share of human suffering. Therefore, the strategies of extending lifespan and improving healthspan are becoming the primary research directions. The aging process is a dynamic and chronological process characterized by the gradual accumulation of oxidative damage, imbalances in protein homeostasis, and mitochondrial dysfunction.2 Currently, Caenorhabditis elegans is widely utilized in aging-related research, and various typical signaling pathways and transcription factors have been identified that regulate the aging process, such as the nuclear factor (erythroid-derived 2)-like 2 (NRF2)/SKN-1, SIRT1/SIR 2.1, and FOXO/DAF-16, that maintain mitochondrial function.3,4 It has been reported that some natural products have shown functions for improving healthspan. For example, Lycium barbarum polysaccharides were shown to extend the lifespan and improve the abilities to withstand environmental stress by activating sir-2.1, daf-12, and daf-16.5 In addition, ethanol extract of Lonicera japonica significantly extended the lifespan and healthspan of C. elegans via the insulin/IGF-1 autophagy and stress resistance pathways.6 Therefore, many researchers are interested in searching for drugs and functional natural compounds to delay the aging process. Most natural products from nutraceutical foods have attracted the attention of food and biomedical researchers due to their role as free radical scavengers or anti-aging agents.7
Panax ginseng C. A. Meyer (ginseng), an herbaceous plant emerging to the family Araliaceae, is known as a traditional medical herb and functional food widely used in Korea, Japan, China, and the UK. Many studies have shown that it can effectively improve disease phenotypes in animal models of neurodegenerative diseases and metabolic syndrome.8,9 Furthermore, ginseng has been approved as a new source food in 2012 to speed up the development of ginseng health products, including cookies, candies, and beverages in China. It has been found that ginsenosides, as one of the primary active substances in ginseng, exerts multiple biological functions and health benefits. It also plays important roles in anti-oxidation, anti-inflammation, neuroprotection, and improvements in mitochondrial function. For example, ginsenosides protect the brain from D-gal-induced neuronal injury through oxidative stress and apoptosis via the NRF2 and SIRT1-mediated anti-oxidant pathways.10,11 Moreover, different ginsenosides have obvious health benefits to invertebrates (e.g. Drosophila melanogaster and C. elegans under specific circumstances).12–14 Therefore, ginsenosides may be potential natural products with anti-aging and lifespan-extending effects. Currently, only one study has reported that ginsenosides can restore growth, reproduction, and the lifespan of worms caused by cholesterol deprivation, and ginsenoside Rc, as the active component, prolonged the lifespan.12 However, the effect of the total ginsenosides (TGS) extract, a mixture of the primary active ginsenosides from ginseng, on the lifespan of C. elegans under normal and stress conditions have not been concluded, and the molecular mechanism, optimal dosage, and administration stage of TGS for prolonging lifespan and improving fitness are not clear.
In this study, wild-type and mutant C. elegans strains are used to investigate the molecular mechanism of TGS-mediated longevity, health benefits, and stress resistance. The results showed that TGS did prolong the lifespan of C. elegans, delayed age-related physiological functions, and enhanced stress resistance. Furthermore, these effects of TGS were achieved by activating the signaling pathways of anti-oxidant regulation and longevity, including the NRF2/SKN-1, SIRT1/SIR 2.1, and FOXO/DAF-16 signaling pathways. In addition, it was found that TGS treatment with 0.2 mg mL−1 from day four until death was the optimal stage to extend lifespan. Additionally, it was found that Rg1, Re, and Rb1 may be the major effective components with anti-oxidative activities to improve longevity. Overall, these findings provide new insights into the protective role of ginseng in healthy aging and the stress response.
2. Materials and methods
2.1. Preparation of the ginsenoside extract
Briefly, 50 g of dry roots of six-year-old ginseng from Jingyu (Jilin, China) was extracted three times using hot water to obtain the supernatants, which were adsorbed using a microporous resin column and precipitated with 95% ethanol. The crude extract of ginsenosides was filtered through decoloring resin, and activated carbon was to obtain the purified extract of total ginsenoside, as previously described.15
2.2. High-performance liquid chromatographic (HPLC) analysis of the TGS
The ginsenoside monomers, Rg1, Re, Ro, Rf, Rg2, Rh1 (R), Rb1, Rc, F1, Rb2, Rb3, Rd, F2, Rk3, Rg3, PPT, CK, Rh2(S), and PPD (the purity >98%), were purchased from the Shanghai Yuanye BioTechnology (Shanghai, China). The composition of the TGS was analyzed and quantified using high-performance liquid chromatographic (HPLC) analysis using a LC-2030C Plus equipped with a C18 column (5 μm, 4.6 × 250 mm; Kromasil, Sweden) via ultra-violet (UV) detection at 203 nm. A stepwise gradient elution was employed using solvent A (Water) and solvent B (Acetonitrile) and maintained at 30 °C. The flow rate was 1 mL min−1, and the sample injection volume was 10 μL. The detection wavelength was set at 203 nm.
2.3. Nematode strains and culture maintenance
The C. elegans strains were handled using standard nematode growth media (NGM) agar plates and fed with E. coli strain, OP50. All of the experiments were performed at 20 °C unless stated otherwise. Wide-type N2 worms and the following mutants and transgenic strains including EU1, skn-1(zu67) (IV)/nT1[unc-? (n754);let-?] (IV;V); EU31, skn-1(zu135) (IV)/nT1[unc-?(n754);let-?] (IV;V); VC199, sir-2.1(ok434) IV; CF1038, daf-16 (mu86); CL2166, dvIs19 [(pAF15)gst-4p::GFP::NLS]; SJ4100, zcIs13[hsp-6::GFP]; CF1553, muIs84 [(pAD76) sod-3p::GFP + rol-6(su1006)]; RW1596, myo-3(st386)V;stEx30[myo-3p::GFP + rol-6(su1006)], and TJ375, gpIs1[hsp-16.2p::GFP] were purchased from the Caenorhabditis Genetics Center (CGC, University of Minnesota, MN, United States).
2.4. Lifespan assay
According to the standard protocols described in a previous study,16 the TGS was freshly added from a 500 mg mL−1 stock solution into the Nematode Growth Medium (NGM) media before being poured onto the plates. The control population was treated with a concentration of dimethyl sulfoxide (DMSO) with less than a 0.04% final concentration, and 100 μl of a 5× concentrated E. coli OP50 suspension was seeded on the plates using UV irradiation at 254 nm for inactivation. A lifespan assay was performed at 20 °C on the solid NGM. The C. elegans were synchronized by performing a timed egg lay from reproductively active adults (10–15 worms). When the L4 stage arrived, the worms (30 worms per plate and 90 worms per condition at least) were transferred to the new plates containing DMSO or a different concentration of TGS. Fluorodeoxyuridine or any antibiotics were not added in the plates to avoid possible side-effects on the worms. The surviving and dead animals were counted every day until all had died. The animals that crawled off the plates, internally hatched, or were ruptured were censored. All of the experiments were repeated at least three times. The significance of the survival curve was calculated using the Log-rank (Mantel-Cox) test.
2.5. Worm phenotypic assays
2.5.1. Bacterial growth rates.
The experiment was performed as described.17E. coli OP50 (grown overnight in a Luria broth medium and diluted 1
:
1000) and various concentrations of TGS were mixed in 96-well plates and shaken at 37 °C to measure absorbance every hour at 595 nm using a microplate reader (Infinite M200 Pro Tecan, Männedorf, Switzerland). This process lasted at least 8 h. Each condition had five replicates, and the experiment was repeated twice.
2.5.2. Food preference assay.
To assess the preference for foods, the protocol was adapted as previously described.17 A total of 80 μl of OP50 solution was seeded on the two sides of a 10 cm NGM plate. After the OP50 lawn dried over two days, the control (DMSO) or TGS was added on the top of the lawn, and this was allowed to dry for another two days. Then, a drop of M9 buffer that contained 100–200 synchronized day-one worms was placed on the middle of the plates, as shown in Fig. 2b and S2.† In addition, the preference of the worms for either bacteria was counted after 4 h at room temperature.
2.5.3. Fecundity assay.
Ten synchronized L4 worms were laid on the NGM agar plates containing either DMSO or TGS (1 worm per plate) and allowed to lay eggs for 24 h. The mother animals needed to be transferred to new plates every day, and this was performed for five days. The number of progeny was counted to measure the fecundity after 72 h by allowing all of the eggs to develop to the L4 period.17
2.6. Locomotion assay
To measure the bending rate of the animals (the number of body bends per 30 s) during the different periods (young, middle, and old age), synchronized worms were placed on a plate (without OP50) containing a drop of M9 buffer, and they were allowed to adapt for 30 s. The number of bending movements made during locomotion in 30 s was recorded using a hand counter. This assay was performed at least twice, and 25 worms were required for each condition.18
2.7. Fluorescence microscopic imaging
The worms were immobilized using a 5 mM solution of levamisole in an M9 buffer and mounted on 2% agarose pads on glass slides. The images were acquired using a Lionheart Fx Automated Live Cell Imager (BioTek, VT, United States) under non-saturating exposure conditions. For each condition, 25 worms from five fields were observed and imaged. The quantification of the Green fluorescent protein (GFP) expression was analyzed using Image J software (Wayne Rasband, NIH, United States).
2.8. Stress resistance assays
For oxidative stress, the resistance to tBHP was performed essentially as described.19 The worms developed and grew on the NGM agar plates at 20 °C until they reached stage D4. After being transferred to plates containing either DMSO, TGS, or different ginsenosides for 24 h, the plates were exposed to 7 mM of tBHP. All of the experiments were conducted in dark conditions, and the worms were scored as dead if they did not respond to a gentle touch with a platinum wire pick every hour. The survival rate under stress was calculated to analyze the significance between the two groups.
2.9. ROS measurements
The measurements of the ROS levels in the lysed or living worms were assessed previously as described with minor modifications.17,20 Synchronized L4 worms or day four worms were treated with TGS or DMSO for 24 h and then inoculated with 0.25 ml of the M9 buffer containing 3.2 mM tBHP for 1 h as the oxidative stress condition. After being washed with the M9 buffer three times, the worms from the different groups were lysed and centrifugated at 12
000 rpm (4 °C) for 20 min to obtain the supernatants or directly transferred into 96-well black plates. A total of 10 μg of protein or 20 worms per well (80 worms, each group) were incubated using 250 μM of DCFH-DA in 100 μl of phosphate buffering solution (PBS) at 37 °C for 1 h or 5 h to measure the fluorescence intensity using a microplate reader (Infinite M200 Pro Tecan) at the excitation wavelength of 485 nm and the emission wavelength of 535 nm. The DCFH-DA solution in the M9 buffer as background fluorescence was used to normalize the ROS levels in the lysed or living worms.
2.10. mRNA extraction and quantitative real-time PCR
The total RNA was isolated using a total RNA kit (TIANGEN BIOTECH Co., LTD, Beijing, China), and 1 μg of RNA was reverse transcribed into cDNA using the script cDNA synthesis kit (Bio-Rad, CA, United States). Quantitative real-time PCR was performed in a Bio-Rad CFX96 system using the SYBR Premix Ex Taq Kit (Takara Biomedical Technology Co., Ltd, Beijing, China). Y45F10D.4 and act-1 genes were used to normalize the amount of mRNA obtained from a target gene and calculated using the 2−ΔΔCT. Absolute quantification of the mtDNA copy number in the worms were measured using real-time PCR as previously described.21 The relative values for nd-1 and act-3 were compared within each sample to generate a ratio representing the relative level of mtDNA per nuclear genome. The results obtained were confirmed using a second mitochondrial gene, MTCE.26. The average of at least three repeats was used for each biological data point, and the sequences of all the primers are listed in Table S4.†
2.11. NAD+ measurement
For NAD+ quantification, synchronized 1000 worms in each condition were collected from the M9 buffer and washed three times to remove the residual bacteria. Next, the worms with different conditions were placed into flash-frozen in liquid nitrogen, then stored at −80 °C until analysis. The NAD+ levels were measured using a commercial kit (Beyotime Biotechnology) following the manufacturer's instructions.
2.12. Statistical analysis
The data obtained from all the experiments were collected at least three times and all of the bars represent the means ± standard deviation (SD). Excel and GraphPad Prism 8.2 software were used to analyze and plot the experimental data. The log-rank (Mantel-Cox) analysis was performed on the data of the life cycle experiment, and the 95% confidence intervals are shown as the error bars. The statistical significance of the differences was determined using a Student's t test for two groups or a one-way analysis of variance (ANOVA) for the three groups. All p values <0.05 were significant.
3. Results
3.1. TGS extended the lifespan of C. elegans
The wild type N2 worms at different stages were first used to investigate the effect of the TGS on the survival rate of C. elegans, and a specific timing during the worms’ adult life for prolonging lifespan after TGS treatment was identified. As shown in Fig. 1a, the worms were transferred at different stages, such as egg, L4 (larval stage), day four (D4), and day eight (D8) from the control plates to the TGS (0.1 mg mL−1)-incubated plates, and the survival time of worms to death were calculated. The results showed that only worms at the stage of day 4 of the adults treated with TGS had extended their lifespan by 6.45% (p < 0.05). The TGS treatment using the whole life, L4 stage, or D8 of adulthood until death had no extension effect on worm survival (Fig. 1b and Table S1†). Furthermore, the effect of TGS was examined using various concentrations on the lifespan of the worms at the D4 stage. When the TGS was administered to C. elegans from day four of adulthood, the TGS treatment at 0.2 and 0.4 mg mL−1 increased the lifespan by 14.02% (p < 0.05) and 10.8% (p < 0.05) compared with that of the untreated control, respectively (Fig. 1c and d and Table S1†). Based on the above findings, 0.2 mg mL−1 of TGS was chosen as the most effective dosage to treat the worms from day four of adulthood until death in all subsequent experiments unless otherwise stated.
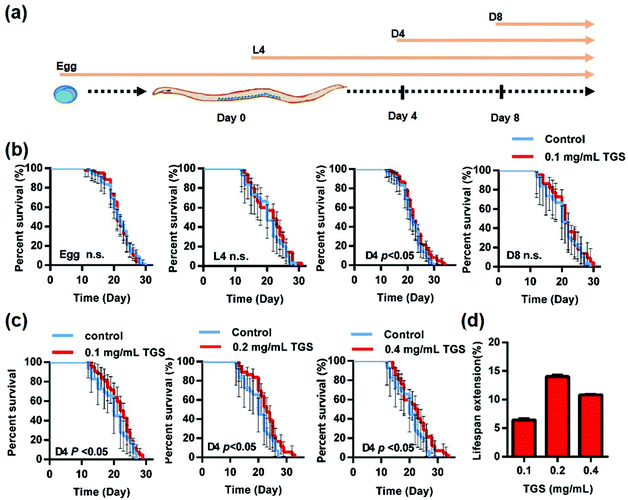 |
| Fig. 1 TGS extends the lifespan of C. elegans. (a) Scheme displaying the different timing stages of the TGS treatment on wild-type N2 worms; egg: from egg until death; L4: from young adult (larval) stage until death; D4: from day four of adulthood until death; D8: from day eight of adulthood until death; (b) the effect of TGS at 0.1 mg mL−1 on the lifespan of worms in the different stages; (c) the lifespans of the worms treated with 0.1, 0.2, and 0.4 mg mL−1 TGS and the corresponding concentrations of the control; (d) percentage changes in the lifespan of N2 worms treated with various concentrations of TGS are shown. For (b) and (c), the p value represents a comparison with the control calculated using the log rank test. Control: DMSO; the 95% confidence intervals are the error bars; n.s.: no significance. | |
As is well known, fasting is one of the mechanisms that can extend nematode lifespan, and this includes two methods: inhibiting bacterial growth to reduce the amount of food available and decreasing food intake as a repellent for the worms.3,22 To verify the first possibility, the effect of TGS on bacterial metabolism was measured using the growth of the E. coli strain OP50 after treatment with TGS. It was found that TGS had no effect on the growth of OP50 (Fig. 2a). For the second possibility, it was found that the worms did not avoid bacteria treated with TGS (Fig. 2b and c and S2†). These results indicated that TGS did not affect the fasting status of the worm. Additionally, reducing fertility is often associated with lifespan extension.23 As shown in Fig. 2d, the TGS treatment did not interfere with fertility, compared with the control group, which was consistent with the findings of the survival rate in worms treated with TGS from the egg or larval stage. Altogether, these data suggested that the TGS-mediated extension of lifespan worked as a direct effect on C. elegans, rather than indirectly influencing the bacteria.
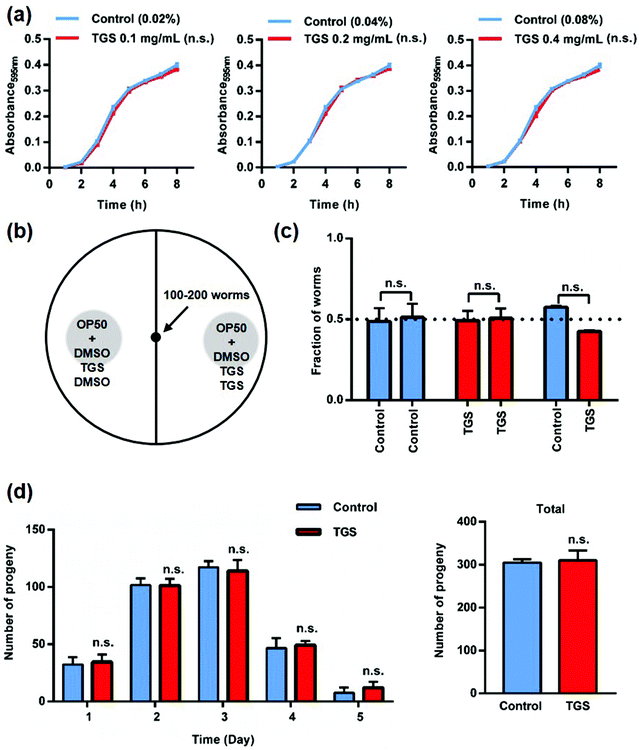 |
| Fig. 2 Bacterial metabolism and food intake are not necessary for the TGS-mediated longevity effect: (a) treatment with TGS at 0.1, 0.2, and 0.4 mg mL−1 for 8 h made no difference on the growth of OP50 bacteria compared to the equivalent DMSO concentration; (b) schematic representation of the food preference assay. Cpd1 and cpd2 represent the DMSO or TGS for both sides; (c) the worms did not have any preference between the TGS at 0.2 mg mL−1 and the control as measured during the bacterial avoidance assay. Mean ± SD. Control: DMSO; n.s.: no significance. (d) The TGS treatment had no impact on fertility during the five-day period, n.s.: no significance. | |
3.2. TGS improved the healthspan of C. elegans
To investigate whether TGS-mediated lifespan extension was related to enhanced health span, the effects of TGS on worm activity were evaluated by assessing the mobility and muscle health. The worms at fourth-day old were treated with TGS at 0.2 mg mL−1, and the activities of worms at 5-, 8-, and 12-days old from the control and TGS-treated groups were investigated. The results showed that the TGS resulted in a significant improvement in the mobilities of the young (5-days old, 9%, p < 0.01), middle (8-days old, 14.42%, p < 0.001), and old (12-days old, 13.75%, p < 0.001) ages of worms (Fig. 3a). Additionally, this improvement in the TGS-treated group was correlated with a better maintenance of muscle fiber organization of the worms expressing a full-length pmyo-3MYO-3::GFP reporter marking myosin heavy chain (Fig. 3b). These results suggested that a decline in body movement with aging was delayed by the TGS. Additionally, the auto-fluorescent lipofuscin accumulations in an aging model of C. elegans from the control and TGS-treated groups were measured. The results revealed that compared to the control worms, the TGS-treated worms on day 8 (28.7%, p < 0.001) and day 12 (25%, p < 0.05) of adult life exhibited significant decreases of the intensity of auto-fluorescent pigment (Fig. 3c and d). Collectively, TGS alleviated the features of age-related physical declines in C. elegans, which indicated an improvement in the fitness of the worms.
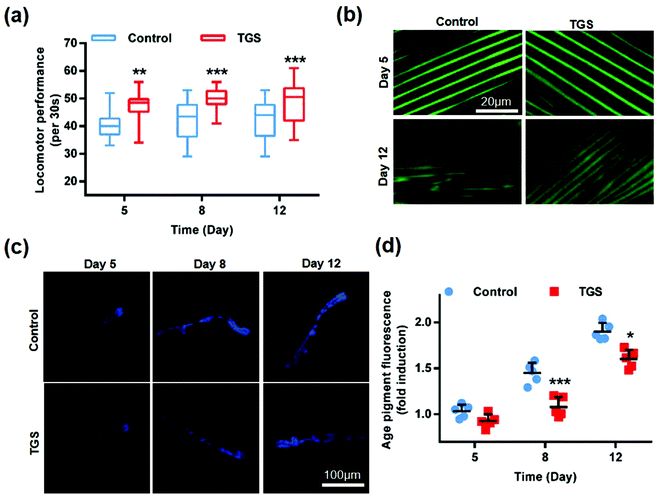 |
| Fig. 3 The TGS improved the healthspan of C. elegans. (a) Locomotor performances in the wild-type young (5-day), middle (8-day), and old (12-day) worms were measured after TGS treatment at the fourth day of adulthood. TGS (0.2 mg mL−1) and treated from day 4 prevented an age-related decline in the worm locomotion. ** p < 0.01, *** p < 0.001 (two-tailed Student's test), n = 75, mean ± SD; (b) representative images of the muscle morphologies at days 5 and 8 in adulthood of the pmyo-3MYO-3::GFP worms treated with 0.2 mg mL−1 of TGS and the control from day 4 of adulthood. Scale bar = 20 μm; (c) the lipofuscin fluorescence accumulation in C. elegans treated with the TGS (0.2 mg mL−1) on day 5, 8 and 12 was assessed. Scale bar = 100 μm; (d) the fluorescence intensities of the lipofuscin. An age pigment in the DMSO- and TGS-treated nematodes from (c) were quantified using Image J software. The graph depicts the mean percentage in arbitrary units relative to that of the control worms on day 5. Mean ± SD, * p < 0.05, *** p < 0.001, Student's t test. | |
3.3. TGS improved the resistance of C. elegans under oxidative stress
As worms age, the resistance to external stimuli declines sharply and increases reactive oxygen species (ROS), which further accelerates the process of aging, forming a vicious cycle.24,25 To confirm whether TGS could increase the stress resistance in C. elegans, the ability to response against oxidative stress was first examined using a t-butyl hydrogen peroxide (tBHP) resistance assay in the wild-type C. elegans. The survival rates of the worms treated with 0.2 mg mL−1 of TGS were measured under the condition induced by 7 mM of tBHP.19 It was found that treatment with 0.2 mg mL−1 of TGS significantly protected worms against oxidative stress to prolong the survival time (28.22%, p < 0.001), (Fig. 4a and Table S2†). When the stimuli were generated by an oxidant, continuously increased reactive oxygen species (ROS) were responsible for the process of aging, which was measured using 2′,7′-dichlorodihydro-fluorescein diacetate (H2DCF-DA), a free radical sensor. The level of ROS was detected in living and lysis nematodes. As shown in Fig. 4b, the results demonstrated that the ROS level in the lysed worms was typically higher during a stress response induced by 7 mM of tBHP, which was significantly decreased by treatment with the TGS (12.4%, p < 0.05) compared with the tBHP condition. Importantly, compared to the tBHP condition, this result was consistent with that detected in living worms, in which the TGS treatment obviously decreased the relative intensity of the ROS in living worms (9.18% p < 0.01), (Fig. 4c and d). In summary, TGS can increase the stress resistance of C. elegans by inhibiting oxidative stress.
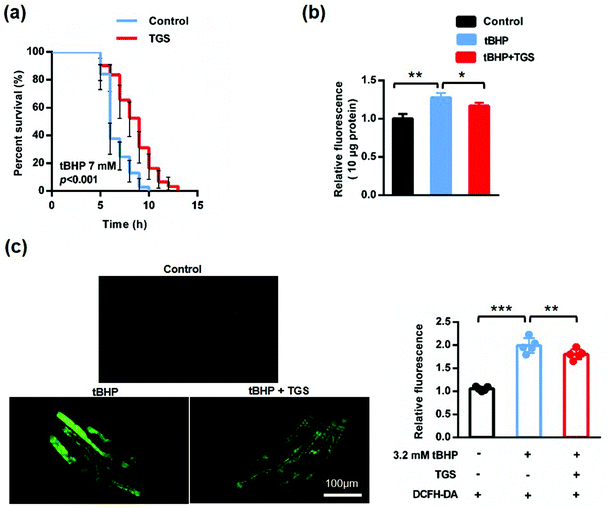 |
| Fig. 4 The TGS increased the resistance of oxidative stress of C. elegans. (a) The survival of worms treated with DMSO- or 0.2 mg mL−1 TGS under the exposure of 7 mM of tBHP, the 95% confidence intervals are the error bars; (b) the relative fluorescence quantitation of the ROS level in the lysed worms from the untreated control, the tBHP, and the tBHP combined with 0.2 mg mL−1 of TGS; (c) images and quantitation of the ROS levels in living worms from three groups above. Scale bar = 100 μm. Data are the means ± SD, *** p < 0.001, compared with control group (Student's t test); ** p < 0.01, compared with the tBHP group (Student's t test). Control: DMSO; tBHP: t-butyl hydrogen peroxide. | |
3.4. TGS required NRF2/SKN-1 activation to extend the lifespan of C. elegans
NRF-2 and its C. elegans ortholog, SKN-1 play a pivotal role in the oxidative stress response and organismal lifespan.26 The effect of TGS on various mutants defective for SKN-1 was examined, and it was found that SKN-1 in the intestine was essential for TGS-induced longevity. This was because the effect of longevity was completely lost in the skn-1 (zu135) mutant rather than in the skn-1 (zu67) mutant (increased 13.2%, p < 0.05) (Fig. 5a and b and Table S3†). Furthermore, the expression of glutathione S-transferase-4 (GST-4), a downstream target of SKN-1 isoform C in vehicle- and TGS-treated worms, was measured. Compared to the control, the TGS treatment caused a significant increase (p < 0.01) in the endogenous mRNA level in the wide-type C. elegans (Fig. 5c). Additionally, the gst-4p::GFP signal in a transgenic worm strain, CL2166 expressing GST-4 promoter driven GFP was enhanced by the TGS treatment for 24 h (p < 0.05) (Fig. 5d and f), compared with that of the control worms. These data indicated that the effect of TGS on extending lifespan depended on its activation of SKN-1.
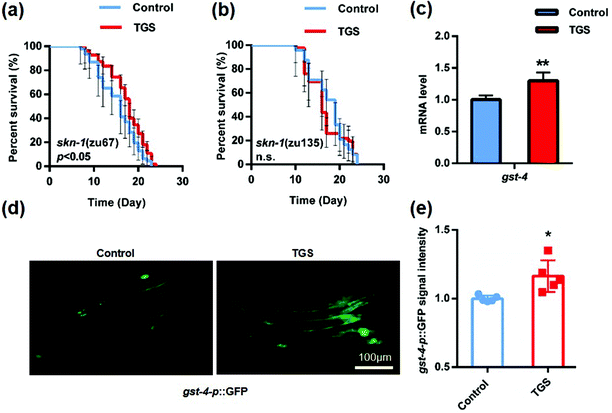 |
| Fig. 5 TGS extended the lifespan through SKN-1 activation. (a and b) The lifespan of the skn-1 (zu135) and skn-1 (zu67) mutants treated with DMSO or TGS (0.2 mg mL−1) from day 4 until death was assessed. The p value represents the comparison with control calculated using the log rank test, the 95% confidence intervals are the error bars; (c) the mRNA level of the gst-4 in the wild-type worms on day four treated with DMSO or TGS for 24 h was analyzed using real-time PCR analysis; (d and f) after treatment for 24 h, the images and quantitative analysis of the GFP fluorescence in the fifth-day worms expressing the GST-4 promoter from the control and the TGS-treated groups are shown. Scale bar = 100 μm, mean ± SD, * p < 0.05, ** p < 0.01, Student's t test. Control: DMSO. | |
3.5. TGS extended the lifespan of C. elegans through the activation of the SIRT1/SIR 2.1 signaling pathway
Sirtuins (SIRTs) are widely distributed across a range of organisms from bacteria to humans that belong to a class of highly conserved proteins. They play critical roles in regulating cellular metabolism, responses to oxidative stress, and lifespan through the deacetylation of histones and non-histones.27 One report validated that TGS enhanced the resistance to oxidative stress and improved the mitochondrial functions through the activation of SIRT 1 in vitro.28 SIR 2.1, the worm sirtuin homolog, is a protein deacetylase that depends on the cellular coenzyme nicotinamide adenine dinycleotied (NAD+).29 To determine whether TGS-induced lifespan expansion depended on the SIRT1/SIR 2.1 pathway, the content of NAD+ was measured, and it was found that it displayed a significant increase (43.7%, p < 0.01) in the worms after treatment with TGS for 24 h (Fig. 6a). Moreover, the mRNA level of sir-2.1 in the TGS-treated worms was higher than that of the control worms (p < 0.01) (Fig. 6b). To further understand the effects of TGS on SIR 2.1 activation, the survival of the sir-2.1-null mutant and sir-2.1 (ok434) nematodes treated with TGS was analyzed, and it was found that TGS failed to increase the lifespan of the sir-2.1 mutant (Fig. 6c and Table S3†). This indicated that the longevity-promoting effects of TGS was required for the SIR 2.1 signaling pathway. Previous studies have reported that activation of SIR 2.1 can improve mitochondrial homeostasis and prolong lifespan by regulating mitonuclear protein imbalance and activating the mitochondrial unfolded protein response (UPRmt).29 Therefore, the ratio of mtDNA/nDNA and the mRNA level of the UPRmt genes were further investigated using quantitive real-time PCR analysis. It was found that TGS altered the nDNA translation (p < 0.01) and induced mitonuclear protein imbalance (Fig. 6d). Moreover, increased mRNA levels of hsp-6 (p < 0.05) and hsp-60 (p < 0.01) were found in the TGS-treated worms compared with that of the control groups (Fig. 6e). In addition, the fluorescence intensity of the transgenic strain, SJ4100 expressing hsp-6::GFP, was increased (p < 0.05) when exposed to TGS treatment for 24 h (Fig. 6f and g). Together, these results indicate that TGS plays an important role in extending lifespan through the maintenance of SIRT1/SIR 2.1-mediated mitochondrial homeostasis.
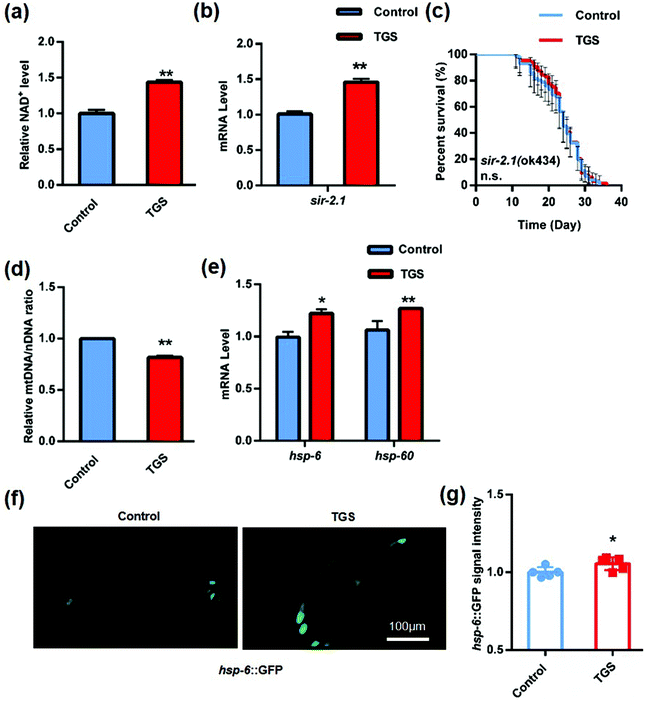 |
| Fig. 6 TGS extended the lifespan through its activation of the SIRT1/SIR 2.1 signalling pathway. (a) TGS (0.2 mg mL−1) treatment for 24 h from day 4 of adulthood increased the NAD+ level of C. elegans; (b) the mRNA expression of sir-2.1 in wild-type worms treated with TGS for 24 h from day 4 was upregulated compared to the control; (c) TGS treatment from day 4 until death did not extend lifespan in the sir-2.1 (ok434) mutant, the 95% confidence intervals are the error bars; (d) treatment with TGS for 24 h induced nuclear DNA translation in worms at the fourth-day age stage; (e) the mRNA levels of hsp-6 and hsp-60, two UPRmt genes in wild-type worms, were enhanced by treatment with TGS (0.2 mg mL−1) for 24 h from day 4 of adulthood; (f and g) images and quantitation of the GFP fluorescence in the transgenic strain, SJ4100 (hsp-6::GFP), treated with TGS (0.2 mg mL−1) and the control for 24 h from five different fields. Scale bar = 100 μm, mean ± SD, * p < 0.05, ** p < 0.01, Student's t test. | |
3.6. TGS prolonged the lifespan of C. elegans through the activation of the FOXO/DAF-16 signaling pathway
There is a FOXO transcription factor homolog in Caenorhabditis elegans that is encoded by the gene, daf-16, which is a central regulator for multiple signaling pathways, including longevity, development, stress resistance, and reproduction.30 Additionally, a few studies have confirmed that SIRT1 could deacetylate FOXO 1, enhance the activity of nuclear ectopic transcription, and increase the expression of antioxidant enzymes such as superoxide dismutase (sod-3) and catalases (ctl-1, ctl-2, and clt-3).31,32 Based on previous reports, it was further studied whether the longevity of TGS was mediated by the activation of the DAF-16 pathway. The lifespan in the mutant daf-16 (mu86) C. elegans treated with TGS from day four until death was similar to the control, indicating that DAF-16 was required for the longevity-promoting effects of TGS (Fig. 7a and Table S3†). Furthermore, the qPCR analysis showed that the mRNA levels of ctl-2 and hsp-16.2, a heat shock protein (HSP) as target genes of DAF-16/FOXO,33 were significantly increased by the TGS treatment (p < 0.01, p < 0.05), compared with control group (Fig. 7b). Additonally, it was detected that the GFP fluorescence intensity of the transgenic strain, sod-3::GFP, was obviously increased by the TGS treatment (p < 0.001), compared to that of the control group (Fig. 7c and d). The GFP signal analysis demonstrated that the expression of the small heat shock protein-16.2 (hsp-16.2) in the hsp-16.2::GFP transgenic strain, TJ375, was significantly higher than that of control group (p < 0.001) (Fig. 7e and f). Collectively, these data suggest that the DAF-16 signaling pathway is necessary for the lifespan extension induced by TGS.
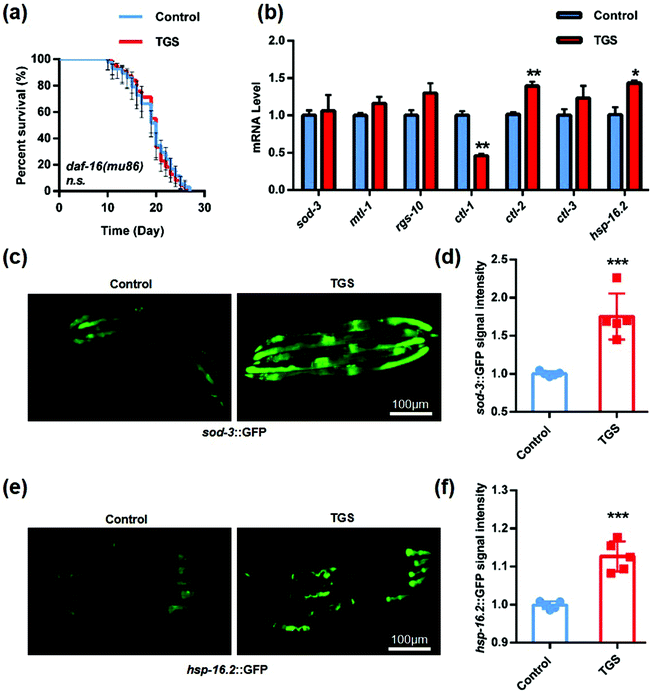 |
| Fig. 7 TGS extended the lifespan by activating the FOXO/DAF-16 signalling pathway. (a) TGS treatment from day 4 until death did not extend the lifespan of the daf-16 (mu86) mutant worms, the 95% confidence intervals are the error bars; (b) the mRNA levels of the key target genes of daf-16 were induced when the worms treated with TGS (0.2 mg mL−1) for 24 h from day four of adulthood; (c and d) after 24 h of treatment, images and quantitation of the GFP fluorescence in the transgenic strain, CF1553 (sod-3::GFP), treated with TGS (0.2 mg mL−1) and the control from day 4 of adulthood are shown; (e and f) the transgenic strain worms expressing hsp-16.2::GFP were treated with DMSO or TGS for 24 h (0.2 mg mL−1)from day 4 of adulthood and pictures were taken and quantified using GFP fluorescence. Scale bar = 100 μm, mean ± SD, * p < 0.05, ** p < 0.01, *** p < 0.001, Student's t test. | |
3.7. An analysis of the components of TGS and their effects on anti-oxidation and longevity
To clarify the active components in the TGS, the HPLC method was utilized to identify the total ginsenosides. As shown in Fig. 8, it was determined that TGS contained ginsenoside Rg1 (17.06%), Re (12.24%), Rf (4.29%), Rg2 (20.41%), Rh1 (R) (0.22%), Rb1 (9.87%), Rc (0.90%), F1 (0.48%), Rb2 (0.58%), Rb3 (13.25%), Rd (1.15%), F2 (0.63%), Rk3 (2.27%), Rg3 (0.20%), PPT (2.39%), CK (0.51%), Rh2 (S) (1.37%), and PPD (0.4%), with the concentration of 88.22%, according to the standard curve of the different ginsenosides. Subsequently, the ginsenoside monomers in TGS that might exhibit anti-oxidative activity and the longevity effect were evaluated (Rg2, 41 μg mL−1; Rg1, 34.0 μg mL−1; Re, 24.5 μg mL−1; Rb1, 20.0 μg mL−1; Rh2, 3.0 μg mL−1; Rd, 2.5 μg mL−1; Rc, 2.0 μg mL−1; Rh1, 0.5 μg mL−1; according to the concentration ratio of TGS at 0.2 mg mL−1). First, the survival ability of the worms that were treated with the different ginsenosides for 24 h against oxidative stress was analyzed. As shown in Fig. 9a and b and Table S5,† treatment with 0.2 mg mL−1 of TGS significantly protected the worms against oxidative stress to prolong the survival time (29.14%, p < 0.001), which was better than any other separate ginsenoside treatment. Furthermore, Rg1 at 34.0 μg mL−1, Re at 24.5 μg mL−1, and Rb1 at 20.0 μg mL−1 were confirmed to improve the pro-longevity effects of the worms under the condition of oxidative stress as 13.5% (p < 0.05), 11.3% (p < 0.05), and 12.61% (p < 0.05), respectively. Additionally, the auto-fluorescent lipofuscin accumulation in an aging model of C. elegans was measured for evaluating the anti-aging effect. The results revealed that these ginsenoside monomers had no effects on the decrease in the lipofuscin accumulation in worms at day five (Fig. 9c and d), compared to the control worms. Critically, TGS-treated worms at day 12 (33.3%, p < 0.01) of adult life exhibited significant decreases of the intensity of auto-fluorescent pigment (Fig. 9c and e). Additionally, the worms with the Rg1, Re, and Rb1 treatments showed great reductions in pigment accumulations (Rg1–27.71%, p < 0.05; Re-28.33%, p < 0.05 Rb1–28.5%, p < 0.05), which were similar to that of the TGS. Collectively, Rg1, Re, and Rb1 may be major effective components in TGS that improve the anti-oxidative activity and alleviate the features of aging-related physical decline in C. elegans.
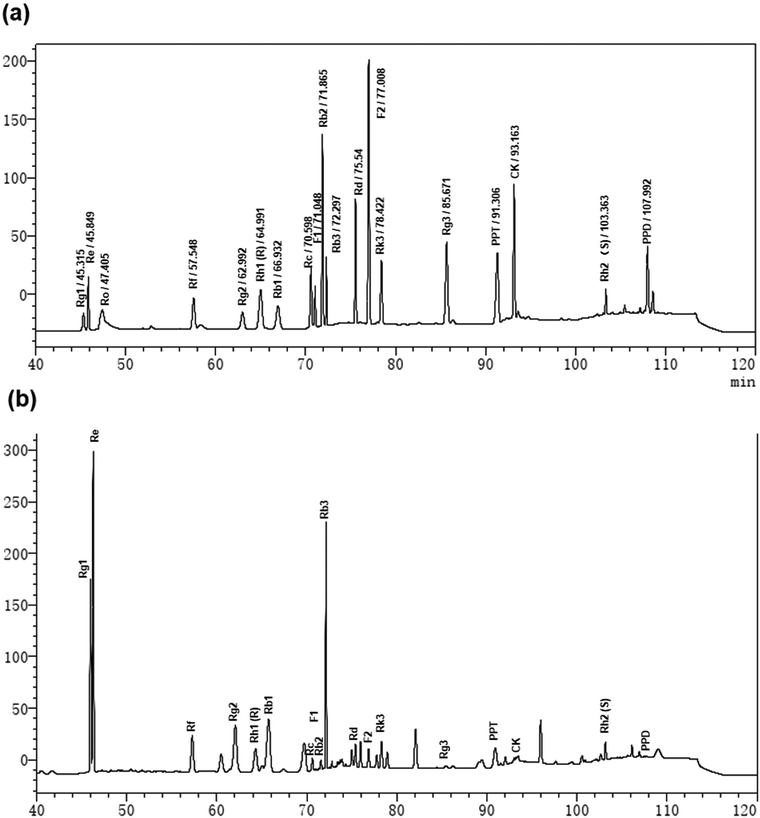 |
| Fig. 8 The HPLC chromatogram of TGS: (a) the HPLC analysis of the standard mixtures including Rg1, Re, Ro, Rf, Rg2, Rh1 (R), Rb1, Rc, F1, Rb2, Rb3, Rd, F2, Rk3, Rg3, PPT, CK, Rh2(S), and PPD; and (b) the HPLC chromatogram of TGS with UV detection at 203 nm. | |
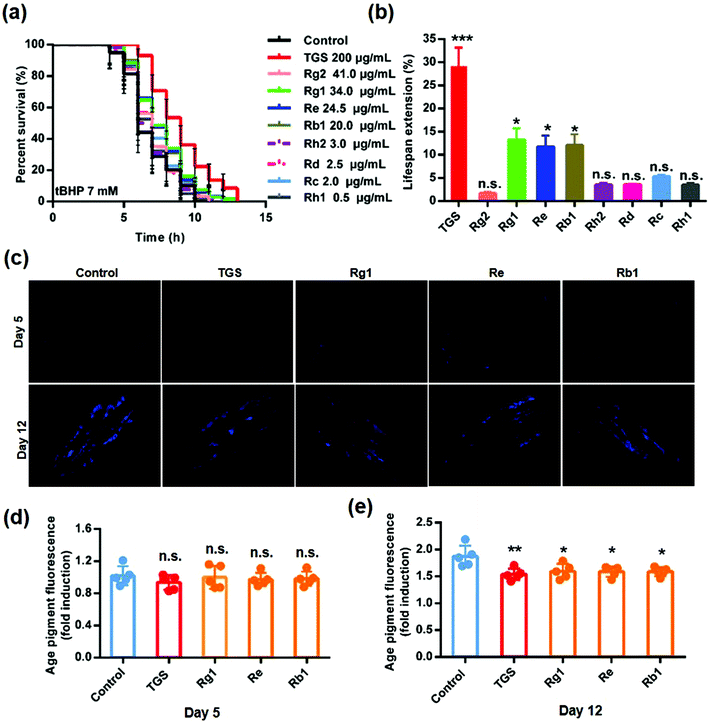 |
| Fig. 9 The ginsenoside components of the TGS had anti-oxidative and longevity effects on C. elegans. (a) Survival of the worms treated with DMSO-, 0.2 mg mL−1 TGS, or different ginsenosides under the exposure of 7 mM of tBHP, the 95% confidence intervals are the error bars; (b) percentage changes in the lifespan of the N2 worms treated with the different ginsenosides under 7 mM of tBHP are shown; (c) the lipofuscin fluorescence accumulation in C. elegans treated with TGS (0.2 mg mL−1) and the different ginsenosides (Rg1-34.0 μg mL−1, Re-24.5 μg mL−1, Rb1-20.0 μg mL−1) on days 5 and 12 were assessed. Scale bar = 100 μm; (d and e) the fluorescence intensities of the lipofuscin, an age pigment in DMSO-, TGS-, Rg1-, Re-, and Rb1-treated nematodes from (c) were quantified using Image J software. The graph depicts the mean percentage in arbitrary units relative to that of the control worms on day 5, mean ± SD, * p < 0.05, ** p < 0.01, *** p < 0.001, Student's t test. | |
4. Discussion
From these results, it was found that TGS had a direct effect on extending longevity to possess the maximum lifespan in C. elegans from day 4 until death without any effects on bacterial growth or food intake. Moreover, TGS significantly improved the aging-related decline in the physiological functions of nematodes. These functions of TGS were mediated by the SKN-1, SIR 2.1, and DAF-16 signaling pathways. This means that TGS as functional components not only prolong the lifespan of nematodes, but also delay entering the aging stage. Rg1, Re, and Rb1 may be the major ginsenoside monomers for prolonging lifespan, which should be further investigated and compared to explore their functions and molecular mechanisms.
As described previously, aging is a progressive deterioration of many physiological functions of an organism.34 In addition, the hallmarks contributing to the aging process can be caused by oxidative damage. Therefore, redox signaling is essential for host defense and in a diverse array of signaling pathways. SKN-1 during the postembryonic stages and the NRF2 ortholog regulate detoxification genes using constitutive and stress-inducible mechanisms in the intestine and the ASI chemosensory neurons, respectively.18 In addition, its target, the gst-4 gene, encodes glutathione-S-transferase-4, which is a phase II detoxification enzyme detoxifying products of oxidative stress.35 In this study, it was found that TGS did not extend the mean lifespan of skn-1(zu135) worms, indicating that SKN-1 signaling in the intestine was essential for this action of TGS. Moreover, TGS activated the GST-4 upregulation on both the mRNA and protein levels. This indicated that TGS improved the condition of the intestine to decrease the stress response and extend the lifespan of C. elegans by activating the SKN-1/GST-4 pathway. FOXO/DAF-16 is a family member of the Forkhead transcription factors that regulates growth, metabolism, the stress response, and longevity in many organisms.36 DAF-16 proteins are distributed in the cytoplasm. When the worms are stressed, the DAF-16 proteins are phosphorylated, activated, and accumulate in the nucleus; meanwhile, they regulate downstream target genes. Multiple downstream genes of DAF-16, including sod-3, mtl-1 (a expressed form of metallothionein), rgs-10 (a regulator of G-protein signaling), ctl-1, ctl-2, ctl-3, and hsp-16.2, are essential for free radical scavenging and the aging process.37 SOD-3 converts superoxide, the primary form among the ROS generated in the mitochondria, into hydrogen peroxide for anti-oxidant activity.38 HSP-16.2 is among the most highly induced HSPs during oxidative stress or thermal stress under physiological conditions.39 These results support that TGS activated DAF-16 signaling to promote the levels of target genes at the mRNA and protein levels can increase the stress resistance and longevity of worms. Overall, this data indicated that TGS can activate SKN-1 and the DAF-16 signaling pathways to reduce the accumulation of free radicals and increase the anti-oxidative ability during the aging process to extend lifespan.
Another hallmark of aging is the gradual decay of protein homeostasis.40 During the aging process, there is a progressive decline in mitochondrial function and maintenance correlated with the load of mtDNA mutations41 and mitochondrial stress response.42 UPRmt is activated to provide a protective ability when nDNA-encoded protein synthesis is not matched by that of mtDNA-encoded, which is a state of mitonuclear protein imbalance.32 Importantly, NAD+-dependent SIRT1/SIR 2.1 have been shown to play multiple roles in UPRmt, the activation of ROS defense, DAF-16 signaling, and mitochondrial energy homeostasis, which can prevent aged-induced metabolic decline and promote longevity in worms.43,44 In this study, it was found that TGS treatment can activate SIRT1/SIR-2.1 signaling to cause a marked induction of UPRmt for maintaining lifespan extension. Collectively, all of the data suggest that the role of TGS for extending longevity was primarily mediated by activating multiple signaling pathways. It is worthwhile to mention that the function of TGS targeting multiple targets might have been associated with the different ginsenosides of TGS, which should be further investigated in the future.
Interestingly, an important experiment found that the effect of TGS on prolonging the lifespan of nematodes was only effective when the nematodes were given TGS from the fourth day until death, rather than from the egg stage or L4. This finding was quite different from previous studies on other drugs that prolong life or delay aging, which demonstrated the best results from the egg or L4 stage.17,45 According to all of the results above, it was concluded in this study that this special time was associated with anti-oxidative activity. To further identify the possible mechanisms of TGS for prolonging longevity, based the intensity of the DCFH-DA probe, it was found that TGS treatment for 24 h either from L4 or D4 decreased ROS accumulation in lysed worms (L4-6.68%, p < 0.01; day 4-28.5%, p < 0.001) (Fig. S1a†). Additionally, TGS treatment for 24 h either from L4 or day 4 decreased ROS accumulation in the living worms (L4-11.19%, p < 0.001; day 4-11.79%, p < 0.01) (Fig. S1b†). In addition, the qPCR analysis showed a significant change in the anti-oxidant gene expression with TGS treatment both from L4 (gst-4-p < 0.001, mtl-1-p < 0.01, ctl-2-p < 0.01, hsp-16.2-p < 0.001) or day 4 (gst-4-p < 0.01, ctl-2-p < 0.01, hsp-16.2-p < 0.05) (Fig. S1c and d†). This indicated that effect of anti-oxidation was both exhibited in C. elegans after TGS treatment from L4 or day 4 rather than only day 4. So why maintaining antioxidant activity after day four could effectively extend lifespan of worms? Recent studies have demonstrated that “mitohormesis” is a concept that an adaptive and protective anti-oxidant response is activated in the mitochondria by an initial accumulation of moderate levels of potentially toxic ROS. These studies have suggested that day one is the first day of adulthood and coincides with a burst in ROS production, and day three is the late reproductive phase (but still pre-aging) with the characteristics of activated ROS defense.32,46,47 At present, many studies have provided direct evidence for this concept. For example, a transient increase in ROS occurred naturally during early development in synchronized C. elegans that put in motion an increase in stress resistance and extended the lifespan in those worms.48 Natural products, such as epigallocatechin-3-gallate, have been confirmed to extend the lifespan of nematodes by inducing the production of ROS from early-to-mid adulthood.49 However, the potent anti-oxidants, such as N-acetylcysteine and vitamins, as traditional antioxidants, did not prolong the life of nematodes treated from egg/L4 and even counteracted the effect of life extension, which was caused by the increase of ROS level in the early stage.49,50 These combined discoveries and results indicated the following two points. First, adequate ROS levels in the early life development of C. elegans can play an active regulatory role in lifespan as an important signaling regulator. TGS intervention before prematurity has an inhibitory effect on ROS levels, and therefore it does not affect lifespan extension. Second, the fourth day of C. elegans was physiologically a suitable time for anti-oxidative stress, and at that time the addition of TGS enhanced the anti-oxidant capacity, thereby exerting life-prolonging effects. Therefore, these experimental results provide an experimental basis for a suitable time choice to exert antioxidant effects.
To elucidate the components of the TGS that might be responsible for the anti-oxidative activity and longevity effects, the HPLC method was used to identify and quantify the ginsenoside compounds from the TGS. It was found that Rg1, Re, and Rb1 exhibited similar effects as TGS on the anti-oxidative activity and delayed the entry of the senescence stage in C. elegans. Surprisingly, individual treatments with Rg1, Re, and Rb1 were less effective than that of TGS, indicating that these ginsenosides might have the potential to synergistically exert anti-oxidant effects as well as anti-aging effects. Additionally, it remains unclear which ginsenoside at the same concentration is best for worm lifespan, and this should be the subject of further research.
5. Conclusions
In summary, it was discovered in this study that TGS extended the lifespan and healthspan by promoting anti-oxidation and maintaining mitochondrial homeostasis. Importantly, the molecular mechanism of TGS may depend on the SKN-1, SIR 2.1, and DAF-16 signal pathways. These findings provide solid evidence that TGS might exert its beneficial role from day 4 of adulthood, and Rg1, Re, and Rb1 may be major active components in TGS that have anti-oxidative and anti-aging effects. This study provides new insights for the further development and research of ginsenosides as active components in ginseng that can serve as functional foods for improving healthy aging and the oxidative response.
Abbreviations
TGS | Total ginsenoside |
tBHP |
t-Butyl hydrogen peroxide |
ROS | Reactive oxygen species |
UPRmt | Mitochondrial unfolded protein response |
NGM | Nematode growth medium |
Author contributions
Conceptualization, X. L., Z. W.; methodology, H. W., S. Z. and L. Z.; formal analysis, H. W., X. L.; investigation, H. W., S. Z. and L. Z.; data curation, H. W., X. L.; writing—original draft preparation, H. W., X. L.; writing—review and editing, H. W., X. L.; visualization, H. W., Z. W., X. L.; supervision, L. S., D. Z., Z. W., X. L.; project administration, D. Z., Z. W., X. L.; funding acquisition, D. Z., X. L. All authors have read and agreed to the published version of the manuscript.
Conflicts of interest
All authors declare that they have no conflict of interest.
Acknowledgements
This study was supported by the National Key Research and Development Program of China (2017YFC1702103), National Natural Science Foundation of China (U19A2013), the Science and Technology Development Plan Project of Jilin Province (20190101010JH, 202002053JC), the National Key Research and Development Program of China (2019YFC1709900) and the Administration of Traditional Chinese Medicine of Jilin Province (2020172).
References
- T. Niccoli and L. Partridge, Ageing as a risk factor for disease, Curr. Biol., 2012, 22, R741–R752 Search PubMed.
- C. López-Otín, M. Blasco, L. Partridge, M. Serrano and G. Kroemer, The hallmarks of aging, Cell, 2013, 153, 1194–1217 Search PubMed.
- C. Kenyon, The genetics of ageing, Nature, 2010, 464, 504–512 Search PubMed.
- J. Campisi, P. Kapahi, G. Lithgow, S. Melov, J. Newman and E. Verdin, From discoveries in ageing research to therapeutics for healthy ageing, Nature, 2019, 571, 183–192 Search PubMed.
- Z. Zhang, Y. Zhou, H. Fan, K. Billy, Y. Zhao, X. Zhan, L. Yang and Y. Jia, Effects of Lycium barbarum Polysaccharides on Health and Aging of C. elegans Depend on daf-12/daf-16, Oxid. Med. Cell. Longevity, 2019, 2019, 6379493 Search PubMed.
- Z. Yang, Y. Yu, H. Lin, D. Liao, X. Cui and H. Wang, Lonicera japonica extends lifespan and healthspan in Caenorhabditis elegans, Free Radical Biol. Med., 2018, 129, 310–322 Search PubMed.
- G. Huang, X. Mei and J. Hu, The Antioxidant Activities of Natural Polysaccharides, Curr. Drug Targets, 2017, 18, 1296–1300 Search PubMed.
- K. Kim, D. Lee, H. Lee, C. Kim, K. Jung and K. Kang, Beneficial effects of Panax ginseng for the treatment and prevention of neurodegenerative diseases: past findings and future directions, J. Ginseng Res., 2018, 42, 239–247 Search PubMed.
- G. Su, Z. Li, R. Wang, Y. Lu, J. Nan, Y. Wu and Y. Zhao, Signaling pathways involved in p38-ERK and inflammatory factors mediated the anti-fibrosis effect of AD-2 on thioacetamide-induced liver injury in mice, Food Funct., 2019, 10, 3992–4000 Search PubMed.
- A. Rajabian, M. Rameshrad and H. Hosseinzadeh, Therapeutic potential of Panax ginseng and its constituents, ginsenosides and gintonin, in neurological and neurodegenerative disorders: a patent review, Expert Opin. Ther. Pat., 2019, 29, 55–72 Search PubMed.
- W. Fan, Y. Huang, H. Zheng, S. Li, Z. Li, L. Yuan, X. Cheng, C. He and J. Sun, Ginsenosides for the treatment of metabolic syndrome and cardiovascular diseases: Pharmacology and mechanisms, Biomed. Pharmacother., 2020, 132, 110915 Search PubMed.
- J. Lee, S. Choi, O. Kwon, T. Shin, J. Lee, B. Lee, I. Yoon, M. Pyo, H. Rhim, Y. Lim, Y. Shim, J. Ahn, H. Kim, D. Chitwood, S. Lee and S. Nah, Effects of ginsenosides, active ingredients of Panax ginseng, on development, growth, and life span of Caenorhabditis elegans, Biol. Pharm. Bull., 2007, 30, 2126–2134 Search PubMed.
- M. Wang, J. Ren, X. Chen, J. Liu, X. Xu, X. Li, D. Zhao and L. Sun, 20(S)-ginsenoside Rg3 promotes myoblast differentiation and protects against myotube atrophy via regulation of the Akt/mTOR/FoxO3 pathway, Biochem. Pharmacol., 2020, 180, 114145 Search PubMed.
- G. Sandner, A. Mueller, X. Zhou, V. Stadlbauer, B. Schwarzinger, C. Schwarzinger, U. Wenzel, K. Maenner, J. van der Klis, S. Hirtenlehner, T. Aumiller and J. Weghuber, Ginseng Extract Ameliorates the Negative Physiological Effects of Heat Stress by Supporting Heat Shock Response and Improving Intestinal Barrier Integrity: Evidence from Studies with Heat-Stressed Caco-2 Cells, C. elegans, and Growing Broilers, Molecules, 2020, 25(4), 835 Search PubMed.
- H. Ahn, S. Hong, J. Kim and O. Kwon, Panax ginseng extract rich in ginsenoside protopanaxatriol offers combinatorial effects in nitric oxide production via multiple signaling pathways, SpringerPlus, 2013, 2, 96 Search PubMed.
- G. Sutphin and M. Kaeberlein, Measuring Caenorhabditis elegans life span on solid media, J. Visualized Exp., 2009, 27, 1152 Search PubMed.
- D. Ryu, L. Mouchiroud, P. Andreux, E. Katsyuba, N. Moullan, A. Nicolet-Dit-Félix, E. Williams, P. Jha, G. Lo Sasso, D. Huzard, P. Aebischer, C. Sandi, C. Rinsch and J. Auwerx, Urolithin A induces mitophagy and prolongs lifespan in C. elegans and increases muscle function in rodents, Nat. Med., 2016, 22, 879–888 Search PubMed.
- E. Dehghan, Y. Zhang, B. Saremi, S. Yadavali, A. Hakimi, M. Dehghani, M. Goodarzi, X. Tu, S. Robertson, R. Lin, A. Chudhuri and H. Mirzaei, Hydralazine induces stress resistance and extends C. elegans lifespan by activating the NRF2/SKN-1 signalling pathway, Nat. Commun., 2017, 8, 2223 Search PubMed.
- H. Li, X. Liu, D. Wang, L. Su, T. Zhao, Z. Li, C. Lin, Y. Zhang, B. Huang and J. Lu,
O-GlcNAcylation of SKN-1 modulates the lifespan and oxidative stress resistance in Caenorhabditis elegans, Sci. Rep., 2017, 7, 43601 Search PubMed.
- D. Yoon, M. Lee and D. Cha, Measurement of Intracellular ROS in Caenorhabditis elegans Using 2′,7′-Dichlorodihydrofluorescein Diacetate, Bio-Protocol, 2018, 8(6), e2774 Search PubMed.
- I. Bratic, J. Hench and A. Trifunovic,
Caenorhabditis elegans as a model system for mtDNA replication defects, Methods, 2010, 51, 437–443 Search PubMed.
- F. Cabreiro, C. Au, K.-Yi Leung and N. Vergara-lrigaray, Metformin Retards Aging in C. elegans by Altering Microbial Folate and Methionine Metabolism, Cell, 2013, 153, 228–239 Search PubMed.
- D. S. Wilkinson, R. C. Taylor and A. Dillin, Analysis of aging in Caenorhabditis elegans, Methods Cell Biol., 2012, 107, 353–381 Search PubMed.
- F. Monickaraj, S. Aravind, P. Nandhini, P. Prabu, C. Sathishkumar, V. Mohan and M. Balasubramanyam, Accelerated fat cell aging links oxidative stress and insulin resistance in adipocytes, J. Biosci., 2013, 38, 113–122 Search PubMed.
- R. Sohal and W. Orr, The redox stress hypothesis of aging, Free Radical Biol. Med., 2012, 52, 539–555 Search PubMed.
- M. McMahon, K. Itoh, M. Yamamoto, S. Chanas, C. Henderson, L. McLellan, C. Wolf, C. Cavin and J. Hayes, The Cap'n'Collar basic leucine zipper transcription factor Nrf2 (NF-E2 p45-related factor 2) controls both constitutive and inducible expression of intestinal detoxification and glutathione biosynthetic enzymes, Cancer Res., 2001, 61, 3299–3307 Search PubMed.
- J. Kim, I. Bang, Y. Noh, D. Kim, E. Bae and I. Hwang, Metabolites produced by the oral commensal bacterium Corynebacterium durum extend the lifespan of via SIR-2.1 overexpression, Int. J. Mol. Sci., 2020, 21, 2212 Search PubMed.
- Y. Wang, X. Liang, Y. Chen and X. Zhao, Screening SIRT1 Activators from Medicinal Plants as Bioactive Compounds against Oxidative Damage in Mitochondrial Function, Oxid. Med. Cell. Longevity, 2016, 2016, 4206392 Search PubMed.
- S. Imai, C. Armstrong, M. Kaeberlein and L. Guarente, Transcriptional silencing and longevity protein Sir2 is an NAD-dependent histone deacetylase, Nature, 2000, 403, 795–800 Search PubMed.
- H. Tissenbaum, DAF-16: FOXO in the Context of C. elegans, Curr. Top. Dev. Biol., 2018, 127, 1–21 Search PubMed.
- C. Shen, J. Jiang, L. Yang, D. Wang and W. Zhu, Anti-ageing active ingredients from herbs and nutraceuticals used in traditional Chinese medicine: pharmacological mechanisms and implications for drug discovery, Br. J. Pharmacol., 2017, 174, 1395–1425 Search PubMed.
- L. Mouchiroud, R. Houtkooper, N. Moullan, E. Katsyuba, D. Ryu, C. Cantó, A. Mottis, Y. Jo, M. Viswanathan, K. Schoonjans, L. Guarente and J. Auwerx, The NAD(+)/Sirtuin Pathway Modulates Longevity through Activation of Mitochondrial UPR and FOXO Signaling, Cell, 2013, 154, 430–441 Search PubMed.
- C. Murphy, S. McCarroll, C. Bargmann, A. Fraser, R. Kamath, J. Ahringer, H. Li and C. Kenyon, Genes that act downstream of DAF-16 to influence the lifespan of Caenorhabditis elegans, Nature, 2003, 424, 277–283 Search PubMed.
- D. C. Park and S. G. Yeo, Aging, Korean J. Audiol., 2013, 17, 39–44 Search PubMed.
- T. K. Blackwell, M. J. Steinbaugh, J. M. Hourihan, C. Y. Ewald and M. Isik, SKN-1/Nrf, stress responses, and aging in Caenorhabditis elegans, Free Radicals Biol. Med., 2015, 88, 290–301 Search PubMed.
- H. A. Tissenbaum, DAF-16: FOXO in the Context of C. elegans, Curr. Top. Dev. Biol., 2018, 127, 1–21 Search PubMed.
- C. T. Murphy, S. A. McCarroll, C. I. Bargmann, A. Fraser, R. S. Kamath, J. Ahringer, H. Li and C. Kenyon, Genes that act downstream of DAF-16 to influence the lifespan of Caenorhabditis elegans, Nature, 2003, 424, 277–283 Search PubMed.
- J. McElwee, K. Bubb and J. Thomas, Transcriptional outputs of the Caenorhabditis elegans forkhead protein DAF-16, Aging Cell, 2003, 2, 111–121 Search PubMed.
- A. Strayer, Z. Wu, Y. Christen, C. Link and Y. Luo, Expression of the small heat-shock protein Hsp16–2 in Caenorhabditis elegans is suppressed by Ginkgo biloba extract EGb 761, FASEB J., 2003, 17, 2305–2307 Search PubMed.
- H. Koga, S. Kaushik and A. M. Cuervo, Protein homeostasis and aging: The importance of exquisite quality control, Ageing Res. Rev., 2011, 10, 205–215 Search PubMed.
- A. Bratic and N. Larsson, The role of mitochondria in aging, J. Clin. Invest., 2013, 123, 951–957 Search PubMed.
- J. Tower, Mitochondrial maintenance failure in aging and role of sexual dimorphism, Arch. Biochem. Biophys., 2015, 576, 17–31 Search PubMed.
- L. Mouchiroud, R. H. Houtkooper, N. Moullan, E. Katsyuba, D. Ryu, C. Canto, A. Mottis, Y. S. Jo, M. Viswanathan, K. Schoonjans, L. Guarente and J. Auwerx, The NAD(+)/Sirtuin pathway modulates longevity through activation of mitochondrial UPR and FOXO signaling, Cell, 2013, 154, 430–441 Search PubMed.
- N. Moroz, J. J. Carmona, E. Anderson, A. C. Hart, D. A. Sinclair and T. K. Blackwell, Dietary restriction involves NAD(+) -dependent mechanisms and a shift toward oxidative metabolism, Aging Cell, 2014, 13, 1075–1085 Search PubMed.
- R. Chin, X. Fu, M. Pai, L. Vergnes, H. Hwang, G. Deng, S. Diep, B. Lomenick, V. Meli, G. Monsalve, E. Hu, S. Whelan, J. Wang, G. Jung, G. Solis, F. Fazlollahi, C. Kaweeteerawat, A. Quach, M. Nili, A. Krall, H. Godwin, H. Chang, K. Faull, F. Guo, M. Jiang, S. Trauger, A. Saghatelian, D. Braas, H. Christofk, C. Clarke, M. Teitell, M. Petrascheck, K. Reue, M. Jung, A. Frand and J. Huang, The metabolite α-ketoglutarate extends lifespan by inhibiting ATP synthase and TOR, Nature, 2014, 510, 397–401 Search PubMed.
- K. Zarse, S. Schmeisser, M. Groth, S. Priebe, G. Beuster, D. Kuhlow, R. Guthke, M. Platzer, C. R. Kahn and M. Ristow, Impaired insulin/IGF1 signaling extends life span by promoting mitochondrial L-Proline catabolism to Induce a transient ROS signal, Cell Metab., 2012, 15, 451–465 Search PubMed.
- G. Bjelakovic, D. Nikolova, L. Gluud, R. Simonetti and C. Gluud, Mortality in randomized trials of antioxidant supplements for primary and secondary prevention: systematic review and meta-analysis, J. Am. Med. Assoc., 2007, 297, 842–857 Search PubMed.
- D. Bazopoulou, D. Knoefler, Y. Zheng, K. Ulrich, B. Oleson, L. Xie, M. Kim, A. Kaufmann, Y. Lee, Y. Dou, Y. Chen, S. Quan and U. Jakob, Developmental ROS individualizes organismal stress resistance and lifespan, Nature, 2019, 576, 301–305 Search PubMed.
- L. Xiong, Y. Chen, J. Tong, Y. Gong, J. Huang and Z. Liu, Epigallocatechin-3-gallate promotes healthy lifespan through mitohormesis during early-to-mid adulthood in Caenorhabditis elegans, Redox Biol., 2018, 14, 305–315 Search PubMed.
- T. J. Schulz, K. Zarse, A. Voigt, N. Urban, M. Birringer and M. Ristow, Glucose Restriction Extends Caenorhabditis elegans Life Span by Inducing Mitochondrial Respiration and Increasing Oxidative Stress, Cell Metab., 2007, 6, 280–293 Search PubMed.
Footnote |
† Electronic supplementary information (ESI) available. See DOI: 10.1039/d1fo00576f |
|
This journal is © The Royal Society of Chemistry 2021 |