DOI:
10.1039/D0FO02232B
(Paper)
Food Funct., 2021,
12, 291-301
Polyphenol from millet bran increases the sensitivity of colorectal cancer cells to oxaliplatin by blocking the ganglioside GM3 catabolism
Received
24th August 2020
, Accepted 13th November 2020
First published on 20th November 2020
Abstract
Colorectal cancer (CRC) is an aggressive malignancy with very limited therapeutic approaches. Drug resistance develops as a frequent characteristic in many patients with CRC, which leads to a decrease in the therapeutic efficacy of anticancer agents. Our previous evidences showed that bound polyphenol from millet bran (BPIS) possesses the potential of inhibiting cancer cell proliferation, and its main anticancer components are ferulic acid (FA) and p-coumaric acid (p-CA). In the present study, we found that BPIS significantly increases the sensitivity of human drug-resistant CRC cell line to oxaliplatin (OXA), a commonly used chemotherapy drug against CRC. Mechanistically, we indicated that BPIS significantly impairs the expression of a gene encoding multidrug resistance protein 1 (MDR1), a well-known permeability glycoprotein (P-gp), by preventing ganglioside GM3 catabolism. Neuraminidase 3 (NEU3) is a key enzyme catalyzing the conversion of ganglioside GM3 to ceramide trihexosides (Gb3), whose expression is increased in drug-resistant HCT-116/L cells. BPIS treatment increased GM3 level, but reduced Gb3 and P-gp levels by inhibiting NEU3 expression, which subsequently boosted the chemotherapy sensitivity of drug-resistant HCT-116/L cells to OXA. These findings reveal that BPIS increases the chemo-sensitivity by remodeling NEU3-mediated ganglioside GM3 catabolism, and it may be applied as a novel drug for facilitating the effectiveness of chemotherapeutic agents in CRC.
1. Introduction
Colorectal cancer (CRC) is one of the most common malignancies of the digestive system worldwide, accounting for 9.6 million deaths in 2018.1 Drug resistance triggered in CRC patients still constitutes a severe problem that constantly weakens the efficacy of both conventional and novel molecular therapies, such as the therapy using oxaliplatin (OXA), a commonly used chemical medicine in CRC therapy.2 Therefore, it is necessary to develop novel drugs that exhibit anticancer activity and less toxic side effects. Foxtail millet (Setaria italica), one of the oldest cultivated and the most important cereal foods in China, includes abundant amount of glucose, lipoprotein, vitamin, polyphenol, etc.3 Foxtail millet bran is a by-product produced during the milling of foxtail millet and a remarkable source of polyphenols.4 An increasing amount of reports have shown that polyphenols derived from millets display significant anti-tumor activity. Hosoda et al. found that polyphenol from millets has the effect of inhibiting inflammation.5 Chandrasekara et al. indicated that polyphenol from whole millet grains possesses anti-proliferative and DNA scission potential in HT-29 cells.6 Our previous studies have shown that bound polyphenol from millet bran (BPIS) possesses an outstanding anti-tumor effect both in vitro and in vivo, compared to that from whole millet grains, but it has no effect on normal cell growth.7 Based on the preceding studies, we further investigated whether BPIS could have an effect on weakening cancer drug resistance. Excitedly, we found that BPIS dramatically reverses chemo-resistance in human drug-resistant CRC HCT-116/L cells to OXA. Subsequently, we elucidated its molecular mechanism of reversing drug resistance in-depth.
Since varied types of gangliosides typically play structural roles in cells, trafficking and communication occur across these lipid barriers; thus, glycolipid biology and chemistry have attracted increasing interest in relation to cancer.8,9 Nohara et al. found that ganglioside content is 4-fold higher in more aggressive MDA-MB-231 breast cancer cells than in MCF-7 cells, especially GM3 level is 18-fold higher in MDA-MB-231 cells.10 This data implied that GM3 may involve in the regulation of drug chemo-resistance. Neuraminidase 3 (NEU3) is a key enzyme for GM3 degradation and takes part in the process of changing ganglioside GM3 into ceramide trihexosides (Gb3).11,12 NEU3 is often markedly up-regulated in human cancers, which leads to the over-accumulation of Gb3. Liu et al. found that the rise in Gb3 in cancer cells increases the expression level of permeability glycoprotein (P-gp) through the recruitment of c-Src kinase, and P-gp is a well-documented and predominant phenotype impairing patients’ response to cancer chemotherapy.13–15 Other reports have also shown that gangliosides are directly related to the expression and activation of P-gp,16 while it is unclear whether the over-expression of NEU3 in cancers is directly connected to the expression of P-gp. Additionally, ceramide (Cer) as a precursor of ganglioside through inducing programmed cell death regulates the chemo-sensitivity of many chemotherapeutic drugs.17,18 These discoveries suggest that it is a new therapeutic strategy for drug resistance to intervene the ganglioside metabolism in cancer cells.
So far, there are no reports about the reversal effect of polyphenols on cancer drug resistance through remodeling ganglioside catabolism. In this study, results showed that BPIS derived from millet bran has the potential to reverse chemo-resistance of CRC to OXA both in vitro and vivo. Mechanically, we firstly found that BPIS remodels ganglioside GM3 catabolism in drug-resistant HCT-116/L cells by regulating the levels of NEU3 and its associated metabolites, resulting in the sharp decrease in P-gp and increase in chemo-sensitivity.
2. Materials and methods
2.1 Materials and reagents
Oxaliplatin (99%), p-coumaric acid (≥99%) and ferulic acid (≥99.5%) were purchased from Aladdin Group Corp (Shanghai, China). Azoxymethane (AOM) was purchased from Sigma Chemical Co., Ltd (St Louis, USA). Dextran sodium sulfate (DSS) was purchased from MP Biomedicals Co., Ltd (Santa Ana, USA). RPMI-1640 medium, DMEM/F-12 1
:
1 medium and fetal bovine serum (FBS) were purchased from GIBCO Co., Ltd (Grand Island, USA). Rhodamine-123 (Rho-123), MTT and dimethyl sulfoxide (DMSO) were obtained from Solarbio life sciences Co., Ltd (Beijing, China). Antibodies for P-gp and NEU3 were purchased from Proteintech Co., Ltd (Wuhan, China). GAPDH antibody was purchased from Abcam Co., Ltd (Cambridge, UK).
2.2 Extraction and analysis of BPIS
The procedures for the extraction of BPIS from foxtail millet bran were implemented as described previously.7 In previous studies, the analysis of the composition of BPIS was conducted.19 First, the sample of BPIS fraction was dissolved in 1 ml methyl alcohol, following which a UPLC-Triple-TOF/MS system (Waters Corp., Milford, MA) was used to detect the composition of BPIS. Subsequently, the data were visually analyzed using Waters Empower software (Waters Corp., Milford, MA). Twelve phenolic acid compounds were identified in BPIS, and the compounds were listed as follows:
Vanillic acid 4-O-β-D-glucopyranoside (C14H18O9), glucosyringic acid (C15H20O10), ferulic acid 4-O-β-D-glucopyranoside (C16H20O9), 4-hydroxybenzoic acid (C7H6O3), vanillic acid (C8H8O4), syringic acid (C9H10O5), p-coumaric acid (C9H8O3), vitexin (C21H20O10), ferulic acid (C10H10O4), isoferulic acid (C10H10O4), biferulic acid (C20H18O9), and 4,4′-dihydroxy-3,5′-dimethoxy,3′-bicinnamic acid (C20H18O8).
2.3 Cell culture
CRC HCT-116 cell line was obtained from the Chinese Type Culture Collection (Shanghai, China). Oxaliplatin drug-resistant HCT-116/L cell line was purchased from Bogoo Biotechnology Co., Ltd (Shanghai, China). HCT-116 cell line was cultured in DMEM/F-12 1
:
1 medium with 10% FBS. Drug-resistant HCT-116/L cell line was cultured in RPMI-1640 medium with 10% FBS. Drug-resistant HCT-116/L cells were routinely maintained in a medium containing 5000 ng ml−1 OXA. All cell lines were incubated in a humidified chamber with 5% CO2 at 37 °C.
2.4 MTT cytotoxicity assay
The drug resistance reversal effects of BPIS and FA combination p-CA on drug-resistant HCT-116/L cells were determined by MTT assay. Drug-resistant HCT-116/L cells were seeded in 96-well plates at a density of 6000 cells per well and treated with different concentrations of OXA or co-incubated with BPIS (0.3 mg ml−1) and ferulic acid (FA) combination p-coumaric acid (p-CA), respectively, for 24 h. Subsequently, 20 μl of MTT (5 mg ml−1) was added to each well and incubated at 37 °C for 4 h. Then, the culturing medium was removed and 150 μl DMSO was added in each well to measure the absorbance value at 570 nm.
2.5 Rhodamine-123 (Rho-123) intracellular accumulation assay
Drug-resistant HCT-116/L cells were plated into 6-well plates and allowed to grow for 24 h. Cells were treated with different concentrations of BPIS for 24 h. Subsequently, cells were washed twice with phosphate buffer saline (PBS), and then stained with Rho-123 (1 μM) for 20 min at 37 °C. The Rho-123 intracellular accumulation assay was conducted by flow cytometry.
2.6 Western blot assay
Drug-resistant HCT-116/L cells were plated into 60 mm dishes and treated with BPIS for 24 h. The total protein was extracted by lysing the cells in lysis buffer (WBIP
:
PMSF = 100
:
1) for 30 min at low temperature, and then centrifugating. Subsequently, 60 μg of cell lysates from each sample were separated by SDS-PAGE (10%), and then the protein was transferred to polyvinylidene fluoride (PVDF) membranes. The membranes were incubated with primary antibodies at 4 °C overnight, and then the PVDF membranes were washed with TBST buffer and incubated with the corresponding secondary antibodies at room temperature for 2 h. Then, the protein bands were visualized using enhanced chemoluminescence.
2.7 Quantitative reverse transcription PCR (RT-qPCR) assay
Drug-resistant HCT-116/L cells were plated into 12-well plates and incubated at 37 °C in 5% CO2 overnight. Then, cells were treated with different concentrations of BPIS for 24 h. The total RNA was extracted from cells using TRIzol (Takara, Japan) for mRNA expression analysis, and 500 ng mRNA was reversed into cDNA. RT-qPCR assay was performed using Trans Start Top Green qPCR Super Mix (TransGen Biotech, Beijing, China). GAPDH gene was used as mRNA gene internal control. The primes used in this study were as follows:
GAPDH-F: 5′-AAGGTCGGAGTCAACGGATTT-3′
GAPDH-R: 5′-CCTGGAAGATGGTGATGGGATT-3′
P-gp-F: 5′-GATTGCTCACCGCCTGTCCAC-3′
P-gp-R: 5′-CGTGCCATGCTCCTTGACTCTG-3′
NEU3-F: 5′-TACTCTGATCTGGCTGCTCTGGAG-3′
NEU3-R: 5′-ATCTGCTCACACTCTTGCTTGGTC-3′
2.8 Transient transfection assay
Drug-resistant HCT-116/L cells were plated into 60 mm dishes and incubated at 37 °C for 24 h, and the specific small interfering RNA (siRNA) of NEU3 (Gene Pharma, Shanghai) was transiently transfected using HiPerFect Transfection reagent (Qiagen) when cell density reached 50%–60% confluence. After 6 h, the medium was replaced with fresh PRMI-1640, and cells were incubated for 48 h prior to being harvested for the extraction of protein lysates.
2.9 Enzyme-linked immunosorbent assay (ELISA)
Drug-resistant HCT-116/L cells were plated into 60 mm dishes and incubated with different concentrations of BPIS for 24 h. Cell supernatants were harvested and centrifuged at 13
000 rpm at 4 °C for 5 min. The levels of Cer, GM3 and Gb3 in the supernatants were measured by ELISA kits according to manufacturer's instructions. The absorbance value was measured at 450 nm. The final values were normalized to total protein concentrations.
2.10 Xenograft model
All animal procedures were performed in accordance with the Guidelines for Care and Use of Laboratory Animals of China Institute for Radiation Protection, China, and approved by the Animal Ethics Committee of China Institute for Radiation Protection, China.
Five-week-old male BALB/c nude mice were purchased from Beijing Vital River Laboratory Animal Technology Co., Ltd. The nude mice were maintained under specified-pathogen free (SPF) conditions in the laboratory animal service center of the China Institute for Radiation Protection. Drug-resistant HCT-116/L (1.2 × 106) cells were injected into the mice subcutaneously in 150 μl of PBS, and the animals were monitored twice a week and then the tumor volume was calculated using the following formula: tumor volume (mm3) = 0.5 tumor length (mm) × tumor width2 (mm2). Two weeks later, the volume of the xenograft tumor reached 100 mm3, and the 21 nude mice were randomly divided into three groups (Control, OXA and OXA combination BPIS). Mice in the OXA group received an intraperitoneal injection of 5 mg OXA per kg body weight once a week, and the control group was injected with normal saline. Mice in the combination group received an intraperitoneal injection of 5 mg per kg body weight once a week and intragastric administration of BPIS with a dose of 75 mg kg−1 six times a week. After 2 weeks, all the animals were sacrificed according to ethical demands, and the tumors were removed to measure their weight and subsequently frozen at −80 °C for the next histological study.
2.11 Establishment of colitis-associated colorectal cancer (CAC) model in mice
All animal procedures were performed in accordance with the Guidelines for Care and Use of Laboratory Animals of China Institute for Radiation Protection, china, and approved by the Animal Ethics Committee of China Institute for Radiation Protection, China.
C57BL/6J male mice (5 weeks old) were purchased from Beijing Vital River Laboratory Animal Technology Co., Ltd. All mice were hosted in a specific pathogen-free environment at 26 ± 1 °C and 60% ± 5% relative humidity in the laboratory animal service center of the China Institute for Radiation Protection. These mice were randomly divided into two groups: Control (C); Model (M). C group was treated with normal saline as untreated control, and M group was intraperitoneally injected once with AOM (10 mg per kg body weight) and a week later given 2% DSS for three cycles (7 days with DSS, then 14 days without). At week 14, all mice were sacrificed, and intestines of mice were excised and stored in liquid nitrogen immediately, until being harvested for the extraction of protein lysates and RNA.
2.12 Immunohistochemistry (IHC) assays
The tumor samples were fixed in 4% buffered formalin overnight, and then these samples were fixed, embedded in paraffin. All sections obtained from 10% paraffin-embedded tissues were stained with antibody of NEU3 and P-gp according to the manufacturer's protocols. Then, these stained sections were photographed under an inverted microscope. Subsequently, IHC data were visualized and assessed by Image pro plus 6.0 software.
2.13 Patient sample
The CRC study protocol was approved by the Human Research Ethics Committee of the Third People's Hospital (Shanxi, China). The procedures were performed in accordance with the Guidelines for Care and Use of the Third People's Hospital (Shanxi, China). Written informed consent was obtained from all participants.
2.14 Statistical analysis
Statistical analyses were performed with the SPSS 17.0 software. Data were expressed as mean ± SD (standard deviation) of at least three independent experiments. Student's t-test was used for single variable comparisons, and one-way ANOVA analysis was used for multiple groups. The differences between the experimental groups were considered significant and highly significant if the P value was less than 0.05 (p < 0.05) and 0.01 (p < 0.01), respectively.
3. Results
3.1 BPIS and its active components inhibit the chemo-resistance of drug-resistant HCT-116/L cells to OXA
Our previous study found that bound polyphenol from millet bran (BPIS) possesses the potential of inhibiting cancer cell proliferation and 12 phenolic acid compounds (as listed above) were identified in BPIS, and FA and p-CA were confirmed as its main anticancer components.7,19 To further assess whether BPIS and its main components could reverse drug resistance in CRC to OXA, drug-resistant human CRC cell line (HCT-116/L) from the parental (HCT-116) cell line was established. As shown in Fig. 1A and B, the viability of HCT-116 and drug-resistant HCT-116/L cells was inhibited on treatment with different concentrations of OXA. The IC50 values of drug-resistant HCT-116/L cells and HCT-116 cells to OXA were 40.128 μg ml−1 and 3.245 μg ml−1, respectively, (resistance index = 12.3) (Table 1). These results indicated that drug-resistant HCT-116/L cells exhibit prominent resistance activity. Subsequently, HCT-116/L cells were treated with different concentrations of BPIS and FA combination p-CA at the natural proportion in BPIS. As shown in Fig. 1C and E, BPIS (IC50 = 0.386 mg ml−1) markedly inhibited the proliferation of drug-resistant HCT-116/L cells compared with FA combination p-CA (IC50 = 0.724 mg ml−1) at the same concentration. To further confirm the reversal effects of BPIS and FA combination p-CA on drug-resistant HCT-116/L cells, the cells were treated with BPIS (0.3 mg ml−1) combination with different concentration of OXA for 24 h. Data indicated that BPIS dramatically inhibited OXA chemo-resistance and the reversal fold was 11.52 (Fig. 1D and Table 2). Interestingly, as Fig. 1F and Table 3 show, at the same concentration of BPIS, the chemo-resistance reversal effect of FA combination p-CA on drug-resistant HCT-116/L cells was relatively lower and its reversal fold was 6.526. These results indicate that the inhibitory activity of FA combination p-CA on drug-resistant HCT-116/L cells is less than that of BPIS. We therefore speculate that synergistic effects of the two active components with other phenolic acids in BPIS may be required for inhibiting the growth of cells.
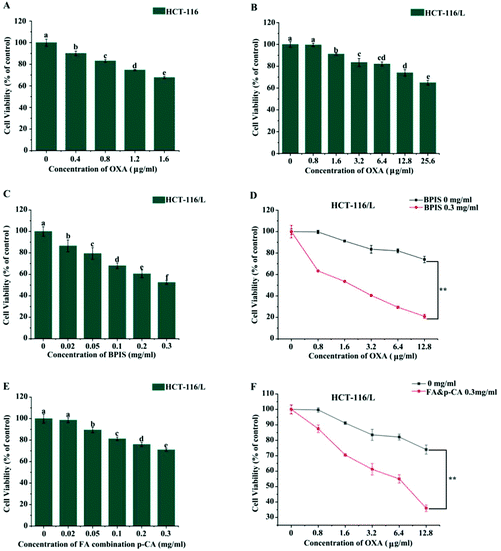 |
| Fig. 1 BPIS increases chemo-sensitivity in drug-resistant HCT-116/L cells to OXA. (A and B) MTT assay was carried out to detect viability of HCT-116 cells and drug-resistant HCT-116/L cells with OXA treatment, respectively. Columns with no letters in common were significantly different between two groups, p < 0.05. (C) Cell proliferation rate was assessed by MTT assay with BPIS treatment for 24 h. Columns with no letters in common were significantly different between two groups, p < 0.05. (D) The proliferation rate of drug-resistant HCT-116/L cells was measured by MTT assay with OXA alone and BPIS combination OXA treatment. Data represent mean ± SD (n = 3). *P < 0.05, **P < 0.01 compared with untreated cells. (E) The viability rate of drug-resistant HCT-116/L cells with FA combination p-CA treatment was tested by MTT assay. Columns with no letters in common were significantly different between two groups, p < 0.05. (F) Cell viability was measured by MTT assay in drug-resistant HCT-116/L cells co-treated with FA combination p-CA and OXA. Data represent mean ± SD (n = 3). *P < 0.05, **P < 0.01 compared with untreated cells (A–C: ANOVA test, D and F: Student's t-test). | |
Table 1 The IC50 values of OXA in drug-resistant HCT-116/L and HCT-116 cells
Chemotherapy drug |
IC50 (μg ml−1) |
Resistance index |
HCT-116 |
HCT-116/L |
OXA |
3.245 |
40.128 |
12.3 |
Table 2 BPIS increases chemo-sensitivity in drug-resistant HCT-116/L cells to OXA
Chemotherapy drug |
IC50 (μg ml−1) |
Reversal fold |
OXA |
40.128 |
11.52 |
OXA + BPIS |
3.482 |
Table 3 FA combination p-CA increases chemo-sensitivity in drug-resistant HCT-116/L cells to OXA
Chemotherapy drug |
IC50 (μg ml−1) |
Reversal fold |
OXA |
40.128 |
6.14 |
OXA + FA & p-CA |
6.526 |
3.2 BPIS increases the chemo-sensitivity in drug-resistant HCT-116/L cells to OXA by enhancing the intracellular accumulation of OXA
P-gp is a typical ATP-dependent membrane transporter, and its abnormal expression often leads to drug resistance of cancer cells to chemotherapeutic agents by inhibiting drug accumulation and increasing efflux.20–23 Hence, we wondered whether BPIS significantly enhances the chemo-sensitivity of human CRC to OXA by impairing the function of P-gp. It has been known that Rho-123 is commonly used as an indicator to evaluate the efflux function of P-gp.24 Therefore, we evaluated the efflux function of the ABC transporter via staining Rho-123. As expected, the mean fluorescence intensity was markedly enhanced in drug-resistant HCT-116/L cells with BPIS treatment. It implies that BPIS promotes the intracellular accumulation of chemotherapeutic agents (Fig. 2A and B). Furthermore, RT-qPCR and Western blot analysis showed that BPIS dramatically blocks the expression of P-gp in drug-resistant HCT-116/L cells at mRNA and protein levels (Fig. 2C–E). These results suggest that BPIS increases the sensitivity to OXA in drug-resistant HCT-116/L cells, which is attributed to the increased intracellular accumulation of OXA induced by impairing ABC transporters.
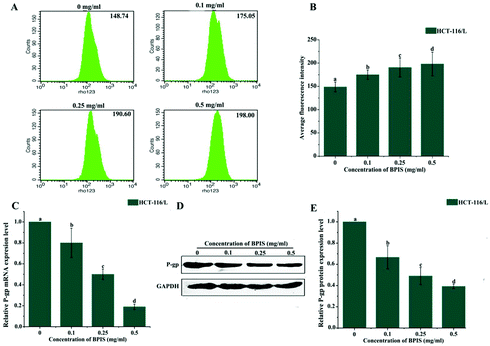 |
| Fig. 2 BPIS decreases OXA chemo-resistance in CRC by increasing intracellular accumulation of OXA in drug-resistant HCT-116/L cells. (A) The mean fluorescence intensity of intracellular accumulation of Rho-123 was detected by flow cytometry. (B) Bar charts display the average fluorescence intensity. Columns with no letters in common were significantly different between two groups, p < 0.05 (ANOVA test). (C and D) The expression level of P-gp was tested by RT-qPCR and Western blot assay. Columns with no letters in common were significantly different between two groups, p < 0.05 (ANOVA test). (E) The relative expression level of P-gp in drug-resistant HCT-116/L cells was analyzed using Image J software. Columns with no letters in common were significantly different between two groups, p < 0.05 (ANOVA test). | |
3.3 Ganglioside GM3 catabolism is involved in the development of CRC and its chemo-resistance
It has been reported that the levels of gangliosides are directly related to the expression of P-gp.16,25 Gangliosides are a class of lipids characterized by the presence of sialic acid residues in their carbohydrate moiety, which are synthesized from a common precursor (GM3).26 We therefore speculated that ganglioside metabolism is disordered in drug-resistant HCT-116/L cells, and these phenomena may promote the drug resistance of CRC to OXA. The levels of ganglioside-related metabolites were analyzed by ELISA in HCT-116 cells and drug-resistant HCT-116/L cells. Data showed that the levels of Cer and GM3 in drug-resistant HCT-116/L cells are reduced and the level of Gb3 is increased compared to that in HCT-116 cells (Fig. 3I). Additionally, ganglioside catabolism occurs at the plasma membrane through the action of membrane sialidase NEU3. So, the expression levels of NEU3 and P-gp in HCT-116 cells and drug-resistant HCT-116/L cells were examined by Western blot analysis. As shown in Fig. 3G and H, dramatically increased levels of NEU3 and P-gp were observed in drug-resistant HCT-116/L cells. These results suggest that abnormal ganglioside GM3 catabolism is an important factor for CRC chemo-resistance. Further, the AOM/DSS-induced CAC mice model was constructed to evaluate the expression of NEU3 in mice. As shown in Fig. 3A–C, NEU3 was over-expressed in intestines of M group mice compared with that in C group at mRNA and protein levels. Importantly, the expression level of NEU3 in patient intestinal tissues also showed a significant increase (Fig. 3D–F). Together, the data indicate that abnormal ganglioside GM3 catabolism regulates CRC development and its chemo-resistance.
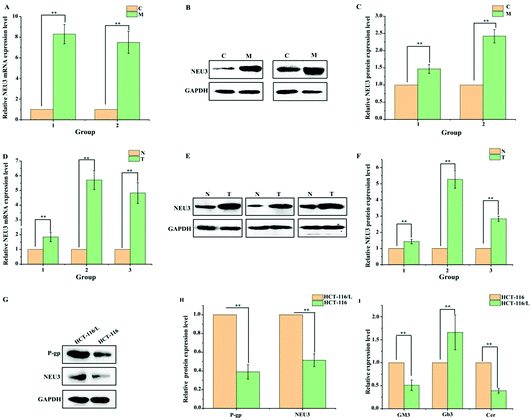 |
| Fig. 3 Ganglioside GM3 catabolism is involved in regulating the development of CRC and its chemo-sensitivity. (A and B) The expression level of NEU3 was detected in AOM/DSS-induced CAC mice model by RT-qPCR and Western blot assay. Data represent mean ± SD (n = 3). *P < 0.05, **P < 0.01 compared with C group. (C) The relative expression level of NEU3 in intestinal tissue samples of CAC mice model was analyzed using Image J software. *P < 0.05, **P < 0.01 compared with C group. (D and E) The expression level of NEU3 was measured in intestinal tissue samples of CRC patients by RT-qPCR and Western blot assay. Data represent mean ± SD (n = 3). *P < 0.05, **P < 0.01 compared with N group. (F) The relative expression level in CRC patient intestinal tissues was quantitatively analyzed by Image J software. Data represent mean ± SD (n = 3). *P < 0.05, **P < 0.01 compared with N group. (G) Western blot assay was performed to assess the expression levels of P-gp and NEU3 in drug-resistant HCT-116/L and HCT-116 cells. Data represent mean ± SD (n = 3). *P < 0.05, **P < 0.01 compared with HCT-116/L cells. (H) The relative expression levels of NEU3 and P-gp were analyzed using Image J software in HCT-116 and drug-resistant HCT-116/L cells. *P < 0.05, **P < 0.01 compared with HCT-116/L cells. (I) The levels of GM3, Gb3 and Cer were tested in HCT-116 and drug-resistant HCT-116/L cells by ELISA. Data represent mean ± SD (n = 3). *P < 0.05, **P < 0.01 compared with HCT-116 cells (A–I: Student's t-test). | |
3.4 BPIS restrains ganglioside GM3 catabolism through reducing the expression of NEU3 in drug-resistant HCT-116/L cells
In order to confirm whether ganglioside catabolism is involved in the reversal effect of drug resistance induced by BPIS, the consequence of BPIS on the expression of NEU3 was detected. RT-qPCR and Western blot analysis showed that the expression level of NEU3 is significantly inhibited in drug-resistant HCT-116/L cells treated with different concentrations of BPIS (Fig. 4A–C). Next, the levels of the key metabolites related to ganglioside GM3 catabolism were measured by ELISA. As expected, the decreased expression of NEU3 resulted in the intracellular accumulation of Cer and GM3. On the contrary, the content of Gb3 was decreased in drug-resistant HCT-116/L cells treated with BPIS (Fig. 4D). These results indicate that BPIS dramatically blocks the ganglioside GM3 catabolism through reducing the expression level of NEU3, a key enzyme of ganglioside catabolism.
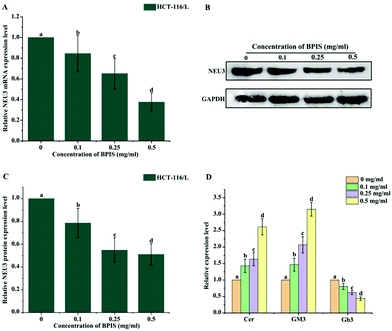 |
| Fig. 4 Ganglioside GM3 catabolism is restrained by BPIS treatment through reducing the expression level of NEU3 in drug-resistant HCT-116/L cells. (A and B) RT-qPCR and Western blot assay were carried out to analyze the expression level of NEU3 in drug-resistant HCT-116/L cells with BPIS treatment. Columns with no letters in common were significantly different between two groups, p < 0.05. (C) The relative expression level of NEU3 in drug-resistant HCT-116/L cells was analyzed using Image J software. Columns with no letters in common were significantly different between two groups, p < 0.05. (D) ELISA was conducted to detect the levels of Cer, GM3 and Gb3 in drug-resistant HCT-116/L cells incubated with different concentrations of BPIS. Columns with no letters in common were significantly different between two groups, p < 0.05 (A–D: ANOVA test). | |
3.5 NEU3 mediates the reversal effect of BPIS on resistance in drug-resistant HCT-116/L cells to OXA
To further determine the role of NEU3 in mediating the reversal effect of BPIS on resistance in drug-resistant HCT-116/L cells to OXA, the expression level of NEU3 was suppressed using a specific siRNA in drug-resistant HCT-116/L cells for 24 h, and it was followed by treatment with 0.5 mg ml−1 of BPIS at 37 °C for 24 h. As shown in Fig. 5A and B, BPIS suppressed the expression level of P-gp, which was augmented in drug-resistant HCT-116/L cells, after knocking-down NEU3. Meantime, down-regulating the expression of NEU3 by using siRNA resulted in a more significant accumulation of GM3 and sharp reduction in Gb3 compared with that under BPIS treatment (Fig. 5C). Furthermore, as demonstrated in Fig. 5D and Table 4, on knocking-down NEU3, cell viability rate dramatically decreased compared to that of NC, and the reversal effect of BPIS on resistance in drug-resistant HCT-116/L cells to OXA was intensified (RF = 14.65). These results hint that the over-expression of NEU3 is directly related to chemotherapy resistance of CRC, which plays a vital role in the process of the reversal effect of BPIS on drug resistance of HCT-116/L cells to OXA.
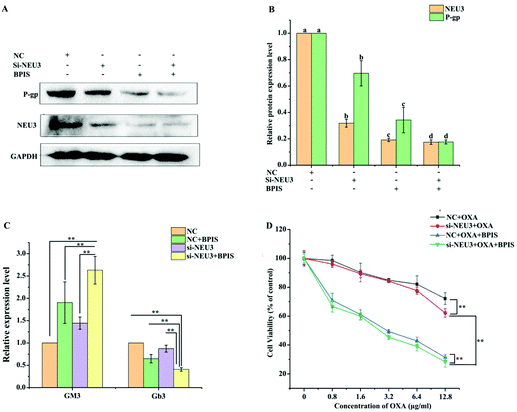 |
| Fig. 5 NEU3 mediates the reversal effect of BPIS on resistance in drug-resistant HCT-116/L cells to OXA. (A) The expression levels of NEU3 and P-gp were detected in drug-resistant HCT-116/L cells by Western blot assay. (B) The relative expression levels of NEU3 and P-gp in drug-resistant HCT-116/L cells were visually and quantitatively analyzed by Image J software. Columns with no letters in common were significantly different between two groups, p < 0.05. (C) The levels of GM3 and Gb3 were measured in drug-resistant HCT-116/L cells by ELISA. Data represent mean ± SD (n = 3). *P < 0.05, **P < 0.01 compared with NC. (D) Cell proliferation rate was assessed by MTT assay. *P < 0.05, **P < 0.01 compared with NC (B: ANOVA test, C and D: Student's t-test). | |
Table 4 Knocking-down NEU3 increases chemo-sensitivity in drug-resistant HCT-116/L cells to OXA
Chemotherapy drug |
IC50 (μg ml−1) |
Reversal fold |
NC + OXA |
40.153 |
— |
si-NEU3 + OXA |
23.621 |
1.70 |
NC + BPIS + OXA |
3.48 |
11.54 |
si-NEU3 + OXA + BPIS |
2.741 |
14.65 |
3.6 BPIS enhances the chemo-sensitivity of CRC to OXA in xenograft nude mice model
To confirm whether BPIS could have a synergistic effect with OXA in chemotherapy in vivo, the xenograft model in nude mice was constructed by subcutaneous injection with drug-resistant HCT-116/L cells. As shown in Fig. 6A–C, after treatment with OXA for 2 weeks, there was no significant change in volume and weight in OXA alone group compared to that in the control group. Excitedly, the growth of tumor was remarkably inhibited on treatment with BPIS combination OXA. Further, results from RT-qPCR and Western blot showed that the expression levels of NEU3 and P-gp are significantly reduced with BPIS combination OXA treatment compared to that with OXA alone treatment (Fig. 6F–H), and these phenomena were verified by IHC assay (Fig. 6D and E). These results illustrate that BPIS reverses the CRC chemo-resistance to OXA by suppressing the expression of NEU3, which mediates ganglioside GM3 catabolism, in xenograft nude mice model.
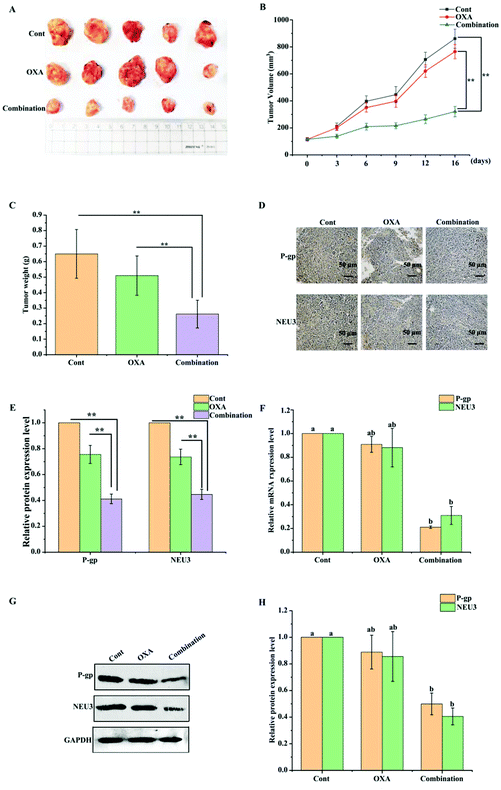 |
| Fig. 6 Effect of BPIS combination OXA in a nude mouse xenograft model of CRC. (A) Xenograft tumors in situ were isolated and photographs were taken. (B) Tumor volume was recorded. *P < 0.05, **P < 0.01 compared with control (Student's t-test). (C) Tumor weight was calculated. *P < 0.05, **P < 0.01 compared with control (Student's t-test). (D) Representative photomicrographs showed the expression levels of NEU3 and P-gp by IHC staining. (E) The relative expression levels of NEU3 and P-gp in tumor were analyzed using Image-Pro Plus 6.0 software. *P < 0.05, **P < 0.01 compared with control (Student's t-test). (F and G) RT-qPCR and Western blot assay were implemented to measure the expression levels of P-gp and NEU3 in mice tumor. Columns with no letters in common were significantly different between two groups, p < 0.05 (ANOVA test). (H) The relative expression levels of P-gp and NEU3 were quantified and calculated by Image J software. Columns with no letters in common were significantly different between two groups, p < 0.05 (ANOVA test). | |
4. Discussion
Despite the sequential improvement in CRC treatment in the last few decades, drug resistance to platinum agents is still recognized as one of the major impediments to CRC therapy.27 Increasing evidences have shown that some polyphenols extracted from natural products have the ability of reversing drug resistance. Meher et al. found that platinum drug resistance (cisplatin or oxaliplatin) can be promoted in ovarian cancer cells by resveratrol treatment.28 Daniela et al. showed that the combination of natural polyphenol chlorogenic acid (CGA) and cisplatin increases the sensitivity of human cervical cancer cells to cisplatin.29 Our data showed that bound polyphenol from millet bran (BPIS) has the excellent potential to reverse chemo-resistance in CRC to OXA in vitro (Fig. 1), and BPIS can also reverse OXA-resistance in vivo, which has not been shown before (Fig. 6). Meantime, the reversal effect of the main active components (FA and p-CA) in BPIS on chemo-resistance to OXA in CRC was evaluated. Interestingly, the present data showed that the inhibitory effects of FA combination p-CA at the natural proportion in BPIS on drug-resistant HCT-116/L cells were less than those of BPIS at the same concentration, which infers that the two active components with other phenolic acids in BPIS have synergistic effects on the inhibition of cell growth (Fig. 1C–F). In 2002, Liu et al. proposed a new concept of anticancer and antioxidant research, that is, the total nutrient components in plants and vegetables rather than the single components play an antioxidant role and have anti-cancer effects.30 Our finding is consistent with this opinion, and, hence, we used total polyphenols of millet bran (BPIS) rather than its main active anti-cancer components for further research.
The markedly up-regulated P-gp in cancer tissues is one of the main causes for the failure of chemotherapy in CRC, and the over-expression of P-gp in chemo-resistant CRC patients leads to abnormally active drug efflux.31 Despite the fact that several clinical trials to overcome P-gp-mediated drug efflux were conducted, the outcomes were not satisfactory due to the high toxicity of P-gp inhibitors.32 Thus, the screening of some safe and effective inhibitors that suppress the expression of P-gp has become a new scheme to reverse the drug resistance. Many studies have shown that the active components of natural polyphenols have the potential to reverse drug resistance by preventing the expression of P-gp. Elisabetta et al. showed that polyphenols from Mangifera indica could impair drug resistance by decreasing the expression of P-gp.33 Munthaj et al. found that gallic acid and ellagic acid increase the sensitivity of linagliptin in male Wistar albino rats by P-gp inhibition.34 In this study we found that BPIS sharply down-regulates the expression of P-gp in vitro and in vivo (Fig. 2C–E and 6F–H). At the same time, the mean fluorescence intensity of Rho-123, as an indicator for evaluating the efflux function of P-gp, was significantly increased in drug-resistant HCT-116/L cells treated with BPIS (Fig. 2A and B). These results indicate that BPIS promotes the sensitivity of CRC to OXA in drug-resistant HCT-116/L cells, which is attributed to the increased intracellular accumulation of OXA induced by the suppression of P-gp expression. Our results imply that polyphenol from millet bran (BPIS) has the potential to be used as a novel inhibitor of P-gp.
Studies have shown that drug sensitivities can be affected by disrupting cellular glycosphingolipid metabolism.35 For example, Liu et al. showed that the sensitivity to various chemotherapeutic drugs is increased 7 to 240-folds and Rho-123 uptake is enhanced by glucosylceramide synthase (GCS) antisense transfection in drug-resistant MCF-7 cells.36 Gouazé found that GCS blockade down-regulates P-gp and increases the chemo-sensitivity in drug-resistant MCF-7 cells to doxorubicin.37 Hence, the screening of some natural active molecules, which could target glycosphingolipid metabolism, may provide an alternative and efficacious approach to address the obstacle of drug resistance. Yoshinagaa et al. found that naringin inhibits the proliferation of tumor cells through suppressing the expression of NEU3, which induces the accumulation of ganglioside GM3.38 This evidence implies that polyphenols present the anticancer potential by regulating ganglioside metabolism. Our result showed that the cellular levels of ganglioside GM3 catabolism-related metabolites (Cer, GM3 and Gb3) are disordered in drug-resistant HCT-116/L cells compared with that in HCT-116 cells (Fig. 3I), and, excitedly, the abnormally decreased levels of Cer and GM3 and the enhanced level of Gb3 are remarkably reversed by BPIS treatment in drug-resistant HCT-116/L cells (Fig. 4D). At the same time, we found that BPIS dramatically down-regulates the expression level of NEU3, a key enzyme for ganglioside GM3 catabolism, in vitro and in vivo (Fig. 4A–C and 6). These evidences demonstrate an important role of BPIS in regulating the reversal effect on chemo-resistance in CRC to OXA by remolding NEU3-mediated ganglioside GM3 catabolism. We further found that the expression level of P-gp is significantly decreased after knocking-down NEU3 in drug-resistant HCT-116/L cells (Fig. 5A), and followed by the reduction of cell viability (Fig. 5D). Particularly, knock-downing NEU3 facilitated the reversal effect of BPIS by intensifying the reduction of P-gp and Gb3, as well as prompted a striking increase in GM3 in drug-resistant HCT-116/L cells incubated with BPIS (Fig. 5A–C). Our study identifies that NEU3-mediated ganglioside GM3 catabolism is one of the key elements that contributes to the chemo-resistance of CRC to OXA.
In summary, BPIS enhances the sensitivity of drug-resistant CRC cells to OXA by remodeling NEU3-mediated ganglioside GM3 catabolism. Our study showed that BPIS treatment increases the intracellular accumulation of GM3 and decreases the levels of Gb3 and P-gp by inhibiting NEU3 expression, which subsequently results in the increase in the chemotherapy sensitivity of drug-resistant CRC cells to OXA. This report emphasizes that BPIS from millet bran possesses the potential to be used as an effective chemosensitizer of CRC.
Funding
This study was supported by the National Natural Science Foundation of China (No. 31770382, 32072220, 81803238), “1331 Project” Key Innovation Center of Shanxi Province, Shanxi Province Science Foundation for Key Projects (No.201801D111001), Shanxi Key Laboratory for Research and Development of Regional Plants, and Higher Education Institution Project of Shanxi Province: Ecological Remediation of Soil Pollution Disciplines Group (No. 20181401)
Conflicts of interest
The authors declare that they have no conflict of interest.
References
- K. D. Miller, S. A. Goding and A. P. Ortiz,
et al., Global cancer statistics 2018: GLOBOCAN estimates of incidence and mortality worldwide for 36 cancers in 185 countries, CA Cancer J. Clin., 2018, 68, 425–445 CrossRef.
- Y. Liu, W. Wu and W. Hong,
et al., Raltitrexed based chemotherapy for advanced colorectal cancer, Clin. Res. Hepatol. Gastroenterol., 2014, 38, 219–225 CrossRef CAS.
- J. L. Dong, L. Wang, J. Lu and Y. Y. Zhu,
et al., Structural, antioxidant and adsorption properties of dietary fiber from foxtail millet (Setaria italica) bran, J. Sci. Food Agric., 2019, 99, 3886–3894 CrossRef CAS.
- K. K. Adom, M. E. Sorrells and R. H. Liu, Phytochemical profiles and antioxidant activity of wheat varieties, J. Agric. Food Chem., 2003, 51, 7825–7834 CrossRef CAS.
- A. Hosoda, Y. Okai and E. Kasahara,
et al., Potent immunomodulating effects of bran extracts of traditional Janpnese millets on nitric oxide and cytokine production of macrophages (RAW 264.7) induced by lipoplyscccaride, J. UOEH, 2012, 34, 285–296 CrossRef CAS.
- A. Chandrasekara and F. Shahidi, Antiprolifirative potential and DNA scission inhibitory activity of phenolics from whole millet grains, J. Funct. Foods, 2011, 3, 159–170 CrossRef CAS.
- Y. S. Jiang, H. S. Shu and W. L. Zong,
et al., Bound polyphenol from foxtail millet bran induces apoptosis in HCT-116 cell through ROS, J. Funct. Foods, 2015, 17, 958–968 CrossRef.
- H. H. Hsu, M. C. Chen and R. Baskaran, Oxaliplatin resistance in colorectal cancer cells is mediated via activation of ABCG2 to alleviate ER stress induced apoptosis, J. Cell Physiol., 2018, 233, 5458–5467 CrossRef CAS.
- V. G. Andersson and M. C. Cabot, Glycosphingolipids and drug resistance, Biochim. Biophys. Acta, 2006, 1758, 2096–2103 CrossRef.
- K. Nohara, F. Wang and S. Spiegel, Glycosphingolipid composition of MDA-MB-231 and MCF-7 human breast cancer cell lines, Breast Cancer Res. Treat., 1998, 2, 149–157 CrossRef.
- K. Takahashi, M. Hosono and I. Sato,
et al., Sialidase NEU3 contributes neoplastic potential on colon cancer cells as a key modulator of gangliosides by regulating Wnt signaling, Int. J. Cancer, 2015, 137, 1560–1573 CrossRef CAS.
- S. Morgan, M. Subathra and O. N. Julie,
et al., Glucose Availability and Glycolytic Metabolism Dictate Glycosphingolipid Levels, J. Cell. Biochem., 2015, 116, 67–80 CrossRef.
- Y. Y. Liu, V. Gupta and G. A. Patwardhan,
et al., Glucosylceramide synthase upregulates MDR1 expression in the regulation of cancer drug resistance through cSrc and β-catenin signaling, Mol. Cancer, 2010, 9, 145–160 CrossRef.
- X. D. Ma, M. Q. Hu and H. Wang,
et al., Discovery of traditional Chinese medicine monomers and their synthetic intermediates, analogs or derivatives for battling P-gp-mediated multi-drug resistance, Eur. J. Med. Chem., 2018, 159, 381–392 CrossRef CAS.
- C. E. Dunk, J. J. Pappas and P. Lye,
et al., P-Glycoprotein, (P-gp)/ABCB1 plays a functional role in extravillous trophoblast (EVT) invasion and is decreased in the pre-eclamptic placenta, J. Cell. Mol. Med., 2018, 22, 5378–5393 CrossRef CAS.
- A. J. Dijkhuis, J. Douwes and W. Kamps,
et al., Differential expression of sphingolipids in P-glycoprotein or multidrug resistance-related protein 1 expressing human neuroblastoma cell lines, FEBS Lett., 2003, 548, 28–32 CrossRef CAS.
- S. A. Morad and M. C. Cabot, Ceramide-orchestrated signalling in cancer cells, Nat. Rev. Cancer, 2013, 13, 51–65 CrossRef CAS.
- O. Cuvillier, G. Pirianov and B. Kleuser,
et al., Suppression of ceramide-mediated programmed cell death by sphingosine-1-phosphate, Nature, 1996, 381, 800–803 CrossRef CAS.
- L. Yang, H. S. Shu and Q. L. Han,
et al., Reversal Effects of Bound Polyphenol from Foxtail Millet Bran on Multidrug Resistance in Human HCT-8/Fu Colorectal Cancer Cell, J. Agric. Food Chem., 2018, 66, 5190–5199 CrossRef.
- J. Kong, Y. Qiu and Y. Li,
et al., TGF-β1 elevates P-gp and BCRP in hepatocellular carcinoma through HOTAIR/miR-145 axis, Biopharm. Drug Dispos., 2019, 40, 70–80 CrossRef CAS.
- C. E. Dunk, J. J. Pappas and P. Lye,
et al., P-Glycoprotein, (P-gp)/ABCB1 plays a functional role in extravillous trophoblast (EVT) invasion and is decreased in the pre-eclamptic placenta, J. Cell. Mol. Med., 2018, 22, 5378–5393 CrossRef CAS.
- M. Jayshree, S. Randall and K. Narendra, Intestinal breast cancer resistance protein (BCRP) requires Janus kinase 3 activity for drug efflux and barrier functions in obesity, J. Biol. Chem., 2019, 294, 18337–18348 CrossRef.
- C. E. Dunk, J. J. Pappas and P. Lye,
et al., P-Glycoprotein, (P-gp)/ABCB1 plays a functional role in extravillous trophoblast (EVT) invasion and is decreased in the pre-eclamptic placenta, J. Cell. Mol. Med., 2018, 22, 5378–5393 CrossRef CAS.
- L. He, H. Zhu and S. Zhou,
et al., Wnt pathway is involved in 5-FU drug resistance of colorectal cancer cells, Exp. Mol. Med., 2018, 50, 101–112 CrossRef.
- E. J. Salustiano, K. M. Costa and L. L. Freire,
et al., Inhibition of glycosphingolipid biosynthesis reverts multidrug resistance by differentially modulating ABC transporters in chronic myeloid leukemias, J. Biol. Chem., 2020, 19, 6457–6471 CrossRef.
- P. Giussani, C. Tringali and L. Riboni,
et al., Sphingolipids: Key Regulators of Apoptosis and Pivotal Players in Cancer Drug Resistance, Int. J. Mol. Sci., 2014, 15, 4356–4392 CrossRef.
- J. Ylenia, G. P. Beatriz and O. Raul,
et al., Oxaliplatin-Biomimetic Magnetic Nanoparticle Assemblies for Colon Cancer-Targeted Chemotherapy: An In Vitro Study, Pharmaceutics, 2019, 11, 395–414 CrossRef.
- N. Meher, B. Philip and C. Charles,
et al., Combinations of resveratrol, cisplatin and oxaliplatin applied to human ovarian cancer cells, Anticancer Res., 2012, 32, 53–59 Search PubMed.
- C. Daniela, F. Raffaella and V. Caterina,
et al., Chlorogenic Acid Interaction with Cisplatin and Oxaliplatin: Studies in Cervical Carcinoma Cells, Nat. Prod. Commun., 2016, 11, 499–502 Search PubMed.
- M. V. Eberhardt, C. Y. Lee and R. H. Liu, Antioxidant activity of fresh apples, Nature, 2000, 405, 903–904 CrossRef CAS.
- C. Daniela, F. Raffaella and V. Caterina,
et al., Chlorogenic Acid Interaction with Cisplatin and Oxaliplatin: Studies in Cervical Carcinoma Cells, Nat. Prod. Commun., 2016, 11, 499–502 Search PubMed.
- G. Y. Lee, J. Y. Joung and J. H. Cho,
et al., Overcoming P-Glycoprotein-Mediated Multidrug Resistance in Colorectal Cancer: Potential Reversal Agents among Herbal Medicines, J. Evidence-Based Complementary Altern. Med., 2018, 17, 1–9 Search PubMed.
- C. Elisabetta, R. Nadia and R. Idania,
et al., In vitro modulation of ABCB/P - gp expression by polyphenols from Mangifera indica, Chem.–Biol. Interact., 2010, 186, 287–294 CrossRef.
- S. Munthaj and R. V. Swaroopa, Enhanced oral bioavailability of linagliptin by the influence of gallic acid and ellagic acid in male Wistar albino rats: involvement of p-glycoprotein inhibition, Drug Metab. Pers. Ther., 2019, 34, 2363–2378 Search PubMed.
- S. F. Tan, W. Dunton and X. Liu,
et al., A Acid ceramidase promotes drug resistance in acute myeloid leukemia through NF-κB-dependent P-glycoprotein upregulation, J. Lipid Res., 2019, 60, 1078–1086 CrossRef CAS.
- Y. Y. Liu, T. Y. Han and A. E. Giuliano,
et al., Ceramide glycosylation potentiates cellular multidrug resistance, FASEB J., 2001, 15, 719–730 CrossRef CAS.
- V. Gouazé, Y. Y. Liu and C. S. Prickett,
et al., Glucosylceramide synthase blockade down regulates P-glycoprotein and resensitizes multidrug-resistant breast cancer cells to anticancer drugs, Cancer Res., 2005, 65, 3861–3867 CrossRef.
- A. Yoshinagaa, N. Kajiyaa and K. Oishi,
et al., NEU3 inhibitory effect of naringin suppresses cancer cell growth by attenuation of EGFR signaling through GM3 ganglioside accumulation, Eur. J. Pharmacol., 2016, 782, 21–29 CrossRef.
Footnote |
† These authors contributed equally to this work. |
|
This journal is © The Royal Society of Chemistry 2021 |