DOI:
10.1039/D0FO01724H
(Paper)
Food Funct., 2021,
12, 340-350
Alleviation of DSS-induced colitis via Lactobacillus acidophilus treatment in mice†
Received
2nd July 2020
, Accepted 24th November 2020
First published on 16th December 2020
Abstract
Gut microbiota play a major role in host physiology and immunity. Inflammatory bowel diseases (IBDs), the important immune-related diseases, can occur through immune system malfunction originating due to dysregulation of the gut microbiota. The aim of this study was to investigate the capabilities and mechanisms of Lactobacillus acidophilus (L. acidophilus) KBL402 and KBL409 treatment in the alleviation of colitis using the in vivo dextran sodium sulfate (DSS)-induced colitis mice model. Various colitis symptoms of mice, including disease activity index score [4.55 ± 0.99 (P < 0.001) and 5.12 ± 0.94 (P < 0.001), respectively], colon length [6.18 ± 0.43 mm (P < 0.001) and 6.62 ± 0.47 mm (P < 0.001), respectively], and colon histological score [(5.33 ± 1.03 (P < 0.001) and 4.00 ± 0.89 (P < 0.01), respectively)], were significantly restored with L. acidophilus KBL402 or KBL409 administration (1 × 109 colony-forming units) for 8 days. Moreover, inflammatory cytokines, chemokines, and myeloperoxidase were downregulated in mice with L. acidophilus treatment. Upregulation of anti-inflammatory cytokine IL-10 or regulatory T cells were discovered with L. acidophilus KBL402 (12.90 ± 7.87 pg mL−1) (P < 0.05) or L. acidophilus KBL409 treatment (10.63 ± 2.70%) (P < 0.05), respectively. Expressions of inflammation-related micro-RNAs (miRs) were also significantly altered in mice with L. acidophilus. Finally, L. acidophilus treatment could restore the diversity of the gut microbiota. Mice with L. acidophilus KBL402 treatment showed a high relative abundance of the genus Akkermansia (0.022 ± 0.017) and Prevotella (0.010 ± 0.006) (P < 0.01). Butyrate and propionate, the major short-chain fatty acids, in the ceca of DSS + KBL402-treated mice were significantly higher than in that of the mice with DSS-induced colitis (0.03 ± 0.02 ng mg−1 and 0.03 ± 0.01 ng mg−1, respectively) (P < 0.05). Our study suggests that L. acidophilus KBL402 and KBL409 could be useful for the prevention or treatment of IBDs in various ways including the modulation of immune responses and miR expression, restoration of the gut microbiota, and production of metabolites.
Introduction
Gut microbiota are strongly related to important host physiologies and immunities.1,2 The commensal homeostasis of the gut microbiota is favored by a balance of the gut microbiota and their metabolites.3 Therefore, various immune-related diseases, such as inflammatory bowel diseases (IBDs), can be caused by immune system malfunctions originating due to dysregulation of the gut microbiota.4,5 Gut microbiota can synthesize essential amino acids and short-chain fatty acids (SCFAs). Amino acids are major components of proteins, and can be used for the production of SCFAs.6,7 Generally, anaerobic bacteria in the intestine produce SCFAs as end-point metabolites and SCFAs can play a key role in adjusting the host's metabolism and regulating the immune response.8
Moreover, the role of micro-RNAs (miRs) as important regulators in innate and adaptive immune pathways is examined.9 miRs are small non-coding RNA species with 19 to 25 nucleotides and can contribute to the development of an inflammatory state in the gut because they are potent gene regulators for controlling various events related to immune cells such as differentiation, proliferation, signal transduction and apoptosis.9,10 miR-107, miR-146, miR-155 and miR-223 showed a strong association with the regulation of innate or adaptive immune responses in local gut inflammation.11–15
Various research suggested that certain types of diets, including the specific carbohydrate diet, the paleolithic diet, and the anti-inflammatory diet, are suitable for the prevention and treatment of IBDs.16,17 Administration of important nutrients and dietary supplements can alleviate IBD and prevent recurrence effectively via various mechanisms such as mucosal healing, gut permeability and microbiota restoration.18Lactobacillus spp. is a major probiotic strain that is highly effective for alleviating IBDs via various mechanisms including modulation of intestinal inflammatory mechanisms and recovery of intestinal microbiota.19–22 Moreover, previous studies demonstrated that administration of various Lactobacillus strains showed modulation in cytokines, restoration of gut tight junction and mucus thickness, and alteration in the gut bacterial diversity from the in vivo dextran sodium sulfate (DSS)-induced colitis model.22–24
Therefore, the aim of this study was to investigate the effects and mechanisms of Lactobacillus acidophilus (L. acidophilus) strains (KBL402 and KBL409) using the in vivo dextran sodium sulfate (DSS)-induced colitis mice model.
Materials and methods
Preparation of L. acidophilus strains
Two L. acidophilus strains (KBL402 and KBL409) were isolated from the feces of healthy Koreans and cultured as described in our previous studies.23–25 Both strains were identified by 16S ribosomal RNA (rRNA) gene sequencing and displayed strong resistance to high concentrations of bile salt (2%) and low pH (4). Then, the L. acidophilus KBL402 and KBL409 strains were cultured in Lactobacilli MRS Agar (BD Difco, Sparks, MD, USA) supplemented with 0.05% L-cysteine hydrochloride at 37 °C under anaerobic conditions for 24 h. Bacterial cells were collected by centrifugation at 3000 rpm and washed twice with 1× phosphate-buffered saline (PBS) before use. Bacterial concentration was measured by the cultivation method and the bacterial stock, which contained 1× PBS with 20% glycerol, was stored at −80 °C until use.
Development of the in vivo colitis model
All experimental procedures for the DSS-induced colitis models were approved by the Institutional Animal Care and Use Committee (IACUC: SNU-160602-9-3) of Seoul National University, Republic of Korea. Seven to eight week-old female C57BL/6J mice (Central Lab Animals Inc., Seoul, Republic of Korea) were used in this study. Eight mice were used for each experimental condition. Mice were kept in air-conditioned cages with a 12 h light–dark cycle and could freely access tap water and food. To induce colitis, water with 2% DSS (MW 36
000–50
000 Da; MP Biomedicals, LLC, Santa Ana, CA, USA) was supplied to mice for 7 days as previously described.23 An approximately 1 × 109 colony-forming units of L. acidophilus KBL402 or KBL409 with 200 μL 1× PBS were simultaneously administered by daily oral gavage.20–22 The body weights and disease activity indices (DAIs) of mice, including body weight loss (%), stool consistency, and blood in feces, were measured in triplicate for each time point as described previously (Table S1†).23,24,26 After 8 days of L. acidophilus treatment, all mice were sacrificed and cecum weights and colon lengths of mice were measured. Colon, stool and cecum samples were immediately stored at −80 °C until further use.
Histological analysis
Collected colons were divided into two segments, the proximal and distal colon. Distal segments were fixed in 10% formaldehyde and stained with hematoxylin and eosin as described previously.23,24,27 Histological scores of distal segments were examined using a Panoramic Viewer (3DHISTECH, Ltd, Budapest, Hungary). Histological scores were recorded as described previously on a scale from 0 to 12 following four categories: (1) loss of epithelium, (2) crypt damage, (3) depletion of goblet cells, and (4) inflammatory cell infiltration (Table S2†).23,24,28
Analyses of cytokine, chemokine, and myeloperoxidase (MPO) levels in colon samples
Weighed colon samples were homogenized using a MM 400 Mixer Mill homogenizer (Retsch, GmbH, Haan, Germany) in a 1× RIPA buffer (Thermo Fisher Scientific, Inc., Waltham, MA, USA) and a Halt Protease Inhibitor Cocktail (Thermo Fisher Scientific), as described previously.23 The supernatant was collected using centrifugation (at 4 °C, for 10 min at 15
000g). Cytokines including interferon (IFN)-γ, interleukin (IL)-4, IL-6, IL-10, IL-17A and tumor necrosis factor (TNF) were measured using a BD cytometric bead array mouse T helper cell (Th)1/Th2/Th17 cytokine kit (BD Biosciences, San Jose, CA, USA) according to the manufacturer's instructions. Cytokine IL-1β in the colon samples were measured using a Murine IL-1β Mini ABTS ELISA development kit (#900-M47; PeproTech, RockyHill, NJ, USA).24 C–C motif chemokine ligand 2 (CCL2) and C–X–C motif chemokine ligand 1 (CXCL1) in colon samples were measured using a JE/MCP-1 (CCL2) and a CXCL1 Mini ABTS ELISA development kit (#900-M126 and #900-M127; PeproTech), respectively.24 Mouse MPO ELISA (Hycult Biotech, Uden, the Netherlands) were used for MPO detection in the colon samples.23
Flow cytometry analysis
Flow cytometry analysis of T regulatory cells (Tregs) was performed as described previously with some modification.23,24 First, to decrease friction, the mesenteric lymph node (MLN) samples were carefully crushed in wet slides and filtered using a cell strainer (70 μm pore size, SPL Life Science Co., Ltd, Pocheon-Si, Gyeonggi-do, Republic of Korea). To confirm cell viability, T cells were stained using a fixable viability stain 510 (FVS510; BD Biosciences). Cell surfaces were stained using anti-CD3 fluorescein isothiocyanate (145-2C11; BD Biosciences), anti-CD4 PerCP-Cyanine5.5 (RM4-5; BD Biosciences), and anti-CD25 phycoerythrin (PC61; BD Biosciences), following the manufacturer's instructions. A fixation/permeabilization kit (eBioscience, San Diego, CA, USA) was used for cell permeabilization. Then, intracellular staining was performed using an Alexa Fluor 647 anti-Foxp3 antibody (MF23; BD Biosciences). The CD4 + CD25 + Foxp3+ Tregs were measured using a BD FACSVerse™ flow cytometer (BD Biosciences) and immunoglobulin G isotypes (BD Biosciences) were used as a control.
Analyses for miR expressions in colon samples
Small RNA (<200 nucleotides) fractions were extracted from distal colon samples using a miRNeasy mini kit (Qiagen, Hilden, Germany), following the manufacturer's instructions. Then, RNA samples were quantified using a Nanodrop® ND 1000 (Thermo Fisher Scientific) and 500 ng RNA samples were reverse-transcribed using a miScript II RT kit (Qiagen). Complementary DNA (2 ng) was amplified using a QuantiTects SYBR green PCR master mix (Qiagen), a miScript universal primer (Qiagen), and miScript primer assays (Qiagen): miR-107 (MS00023961), miR-146 (MS00001638), miR-155 (MS00001701) and miR-223 (MS00001960). PCR reactions were performed using a real-time PCR system (Applied Biosystems) with the following conditions: an initial denaturation at 95 °C, for 15 min, followed by 40 cycles of 94 °C, for 15 s, 55 °C, for 30 s, and 72 °C, for 30 s. miR expressions were normalized using a small nuclear RNA, C/D box 95 (Qiagen).
Analyses of cecal microbiota
Cecal microbiota were analyzed as described previously with some modifications.23,24 First, total DNA of cecum samples was extracted using a QIAamp fast DNA stool mini kit (Qiagen), following the manufacturer's instructions. Universal primer 515F and 926R were used for the amplification of the V4-5 region in the 16S rRNA gene.29 PCR amplicons were purified using a QIAquick PCR purification kit (Qiagen) and a MiSeq platform (Illumina, Inc., San Diego, CA, USA) was used for next-generation sequencing. Data processing was performed using a Quantitative Insights into Microbial Ecology 1.8.0 (QIIME) software (QIIME Development Team; http://qiime.org) and a Greengenes ver. 13_5 database (http://greengene.secondgenome.com). Briefly, sequences were clustered into operational taxonomic units (OTUs) using the OTU picking protocol with at least 97% nucleotide identity and a non-rarefied OTU table was generated for the relative abundance of microbial taxa. Alpha-diversity was demonstrated as PD whole tree indices. Beta-diversity was demonstrated as UniFrac distance, which was visualized in three-dimensional plots following the weighted principle coordinate analysis. Linear discriminant analysis (LDA) effect size (LEfSe) analysis was performed using Galaxy ver. 2.1.1.30–32
Quantification of amino acids in cecum samples
Amino acids in the cecal samples were measured as previously described.24 Concentrated cecum extracts (1 mL) in liquid chromatography-grade methanol (20 mg mL−1) were derivatized using a 70 μL AccQ·Tag ultra borate buffer (Waters Corporation., Milford, MA, USA) and a 20 μL AccQ·Tag ultra reagent (Waters Corporation). After incubating for 10 min at 55 °C, the amino acids in the derivatized samples were measured using an Acquity ultra-performance liquid chromatograph (Waters Corporation) and a SYNAPT G2-Si mass spectrometer (Waters Corporation) with an ESI probe under the following conditions: 1.5 kV capillary voltage, 600 L h−1 desolvation gas flow, 50 L h−1 cone gas flow, and 250 °C desolvation temperature. Amino acids were identified using an AA-S-19 analytical standard mixture (Sigma-Aldrich, St Louis, MO, USA). Data acquisition and quantitation were carried out using a MassLynx software 4.1 (Waters Corporation).33
Quantification of SCFAs in cecum samples
First, cecum samples were homogenized in distilled water and centrifuged at 13
000g for 5 min. The supernatant was collected and 1% 2-methylpentanoic acid was added to the supernatants as an internal standard. Ethyl ether was used as an extraction solvent. The organic layers of samples were measured using an Agilent 7890A gas chromatograph (Agilent Technologies, Santa Clara, CA, USA) under the following conditions: 1.5 kV capillary voltage, 600 L h−1 desolvation gas flow, 50 L h−1 cone gas flow, 170 °C oven temperature and 225 °C for a flame ionization detector and an injection port temperature. Nitrogen was used as the carrier gas. The retention times and peak areas of samples were confirmed using a standard mixture.34
Statistical analysis
Data are expressed as means ± standard deviation, maximum, median, and minimum values of the experimental groups in three independent experiments. When appropriate, GraphPad Prism ver. 5.04 (GraphPad Software, Inc., La Jolla, CA, USA) was used for statistical analyses such as the Shapiro–Wilk test and analysis of variance (ANOVA) with Dunnett's post hoc test and visualizations. P-values (P) <0.05 were considered statistically significant. In addition, Spearman's nonparametric correlation coefficients between the relative abundances of genus level taxa and specific metabolites were calculated using GraphPad Prism ver. 5.04 and visualized using pheatmap (ver. 1.0.12) package in R (ver. 3.5.0).
Results
Improvements of symptoms in mice with DSS-induced colitis using L. acidophilus treatment
Table 1 and Fig. S1† show the effects of L. acidophilus treatment on the colitis symptoms in mice. Compared to DSS + PBS-treated mice, DAI scores were reduced in DSS + KBL402-treated and DSS + KBL409-treated mice after 8 days of treatment [4.55 ± 0.99 (P < 0.001) and 5.12 ± 0.94 (P < 0.001), respectively]. Moreover, DSS + KBL402-treated and DSS + KBL409-treated mice also showed significantly longer colon lengths [6.18 ± 0.43 mm (P < 0.001) and 6.62 ± 0.47 mm (P < 0.001), respectively] and lower histological scores [(5.33 ± 1.03 (P < 0.001) and 4.00 ± 0.89 (P < 0.01), respectively)] compared to DSS + PBS-treated mice [(5.45 ± 0.42 and 9.50 ± 3.02, respectively)].
Table 1 Improvement of colitis symptoms after 8 days of Lactobacillus acidophilus (L. acidophilus) administration
Measurement |
Experimental groupa |
Water + PBS |
DSSb + PBS |
DSS + KBL402 |
DSS + KBL409 |
Data are expressed as mean ± standard deviation (SD) (range, median).
Dextran sodium sulfate.
Asterisks indicate statistical significance compared to the DSS + PBS-treated group as a positive control [*, P < 0.05;**, P < 0.01;***, P < 0.001; analysis of variance (ANOVA) with Dunnett's post hoc test].
Disease activity index (DAI).
|
Weight change (%) |
103.69 ± 2.34***c (99.89–112.36, 104.64) |
85.60 ± 4.89 (74.75–89.44, 88.29) |
90.10 ± 7.03 (75.91–101.87, 88.68) |
91.02 ± 8.05 (74.42–101.74, 92.53) |
DAI scored |
0.00 ± 0.00*** (0.00–0.00, 0.00) |
6.99 ± 1.13 (5.30–9.30, 6.80) |
4.55 ± 0.99*** (3.20–6.20, 4.40) |
5.12 ± 0.94*** (3.70–7.70, 5.20) |
Colon length (mm) |
7.31 ± 0.50*** (5.30–7.70, 7.5) |
5.45 ± 0.42 (4.90–6.20, 5.35) |
6.18 ± 0.43*** (5.90–7.40, 6.55) |
6.62 ± 0.47*** (5.90–7.40, 6.55) |
Histological score |
0.83 ± 0.41*** (0.50–1.50, 0.75) |
9.50 ± 3.02 (4.00–12.00, 11) |
5.33 ± 1.03** (4.00–7.00, 5) |
4.00 ± 0.89*** (3.00–5.00, 4.00) |
Differences in cytokine levels in mice with DSS-induced colitis using L. acidophilus treatment
Table 2 exhibits the effect of L. acidophilus treatment on the cytokine levels of mice with DSS-induced colitis. Overall, L. acidophilus treatment significantly affected the downregulation of Th1-, 2- and 17-related cytokines in mice with DSS-induced colitis (Table 2). Moreover, the colons from DSS + KBL402-treated mice showed significantly higher levels of anti-inflammatory cytokine IL-10 (12.90 ± 7.87 pg mL−1) than DSS + PBS-treated mice (5.12 ± 2.47 pg mL−1; P < 0.05).
Table 2 Differences in the cytokine levels of colons using L. acidophilus administration
Cytokine (pg mL−1) |
Experimental groupa |
Water + PBS |
DSS + PBS |
DSS + KBL402 |
DSS + KBL409 |
Data are expressed as mean ± SD (range, median).
Interferon.
Asterisks indicate statistical significance compared to the DSS + PBS-treated group as a positive control (*, P < 0.05; **, P < 0.01; ***, P < 0.001; ANOVA with Dunnett's post hoc test).
Interleukin.
Tumor necrosis factor.
|
IFNb-γ |
7.67 ± 0.83***c (6.55–8.64, 7.37) |
86.87 ± 7.62 (10.25–99.38, 83.04) |
60.60 ± 51.43 (16.9–86.85, 60.11) |
23.21 ± 3.85** (14.01–26.61, 19.18) |
ILd-1β |
34.50 ± 12.75** (21.85–46.00, 35.08) |
221.73 ± 122.12 (80.42–379.73, 211.93) |
69.64 ± 100.90** (10.92–278.24, 23.98) |
60.40 ± 48.49** (8.55–164.36, 46.15) |
IL-4 |
3.92 ± 0.23 (3.52–4.87, 4.09) |
4.50 ± 1.09 (3.32–7.97, 4.35) |
3.52 ± 0.34* (2.87–3.91, 3.47) |
3.60 ± 0.49* (2.98–4.54, 3.52) |
IL-6 |
5.92 ± 0.92*** (4.55–7.23, 5.97) |
972.28 ± 720.18c (524.43–2713.49, 689.52) |
446.02 ± 206.05 (251.14–767.23, 429.48) |
316.98 ± 192.14* (94.45–613.36, 280.16) |
IL-10 |
1.29 ± 1.79 (0.02–4.35, 0.41) |
5.12 ± 2.47 (2.63–8.21, 4.83) |
12.90 ± 7.87* (4.78–23.91, 8.87) |
9.26 ± 2.51 (5.75–12.66, 9.31) |
IL-17A |
7.15 ± 1.06*** (5.77–12.41, 7.93) |
17.50 ± 2.98 (10.77–22.49, 16.43) |
13.35 ± 3.23** (9.49–17.66, 12.23) |
8.71 ± 0.94*** (5.98–9.48, 7.25) |
TNFe |
33.68 ± 3.33*** (29.36–38.64, 33.24) |
255.96 ± 90.51 (72.26–446.59, 257.04) |
201.55 ± 54.79 (128.74–297.79, 192.63) |
101.03 ± 38.51*** (58.84–163.11, 96.29) |
Differences in chemokine and MPO levels in mice with DSS-induced colitis using L. acidophilus treatment
The levels of chemokines in colons from DSS + KBL402-treated or DSS + KBL409-treated mice [677.97 ± 446.86 pg mL−1 (P < 0.01) and 443.94 ± 384.85 pg mL−1 (P < 0.001), respectively, for CCL2; 146.29 ± 63.65 pg mL−1 (P < 0.05) and 135.55 ± 51.38 pg mL−1 (P < 0.005), respectively, for CXCL1] were significantly lower than DSS + PBS-treated mice (1616.02 ± 1040.83 pg mL−1 and 369.41 ± 302.91 pg mL−1, respectively) (Table 3). Moreover, L. acidophilus KBL409 treatment showed a significant reduction in MPO levels (4.66 ± 2.75 ng mg−1 tissue) in mice with DSS-induced colitis (P < 0.05) (Table 3).
Table 3 Differences in chemokine and myeloperoxidase (MPO) levels of colons using L. acidophilus administration
Target |
Experimental groupa |
Water + PBS |
DSS + PBS |
DSS + KBL402 |
DSS + KBL409 |
Data are expressed as mean ± SD (range, median).
C–C motif chemokine ligand 2.
Asterisks indicate statistical significance compared to the DSS + PBS-treated group as a positive control (*, P < 0.05;**, P < 0.01; ***, P < 0.001; ANOVA with Dunnett's post hoc test).
C–X–C motif chemokine ligand 1.
|
CCL2b (pg mL−1) |
59.27 ± 39.01***c (10.26–120.79, 47.59) |
1616.02 ± 1040.83 (157.93–3942.78, 1764.20) |
677.97 ± 446.86** (149.93–1244.43, 856.60) |
443.94 ± 384.85*** (54.71–1417.13, 367.81) |
CXCL1d (pg mL−1) |
51.94 ± 19.04* (38.03–79.05, 45.36) |
369.41 ± 302.91 (143.9–1252.47, 245.37) |
146.29 ± 63.65* (76.13–268.43, 129.55) |
135.55 ± 51.38* (41.08–211.35, 150.65) |
MPO (ng mg−1 tissue) |
0.16 ± 0.10*** (0.05–0.24, 0.19) |
9.59 ± 1.90 (6.80–11.09, 10.24) |
5.62 ± 2.12 (3.83–8.63, 5.01) |
4.66 ± 2.75* (1.63–8.56, 4.17) |
Differences in Tregs in mice with DSS-induced colitis using L. acidophilus treatment
Table 4 shows the effects of L. acidophilus treatment on Tregs in MLNs from mice with DSS-induced colitis. In particular, CD4 + CD25 + Foxp3+ Tregs were significantly higher in MLNs (10.63 ± 2.70%) from DSS + KBL409-treated mice than DSS + PBS-treated mice (5.97 ± 1.80%) (P < 0.05).
Table 4 Differences in CD4 + CD25 + Foxp3+ T regulatory cells (Tregs) from mesenteric lymph nodes using L. acidophilus administration
Target |
Experimental groupa |
Water + PBS |
DSS + PBS |
DSS + KBL402 |
DSS + KBL409 |
Data are expressed as mean ± SD (range, median).
Asterisks indicate statistical significance compared to the DSS + PBS-treated group as a positive control (*, P < 0.05; ANOVA with Dunnett's post hoc test).
|
CD4 + CD25 + Foxp3+ population (%) |
3.15 ± 0.45 (2.77–3.68, 3.08) |
5.97 ± 1.80 (3.74–7.76, 6.78) |
8.35 ± 4.77 (4.71–15.40, 5.06) |
10.63 ± 2.70*b (7.61–14.50, 10.45) |
Alterations of miRs in mice with DSS-induced colitis using L. acidophilus treatment
Table 5 shows the differences in miR expressions in mice with DSS-induced colitis due to L. acidophilus treatment. miR-107 was significantly higher in DSS + KBL409-treated mice (0.52 ± 0.20) compared to DSS + PBS-treated mice (0.15 ± 0.15) (P < 0.05). The lower level of miR-146a was also discovered in DSS + KBL409-treated mice (1.18 ± 0.75) than DSS + PBS-treated mice (2.44 ± 1.52) (P < 0.05). Moreover, miR-155 and miR-223 were significantly lower in DSS + KBL402-treated mice (8.42 ± 9.87 and 1.90 ± 0.91, respectively) compared to DSS + PBS-treated mice (30.09 ± 17.35 and 3.40 ± 1.29, respectively) (P < 0.05).
Table 5 Differences in micro-RNAs (mIRs) from mesenteric lymph nodes using L. acidophilus administration
mIRs (relative miRNA level) |
Experimental groupa |
Water + PBS |
DSS + PBS |
DSS + KBL402 |
DSS + KBL409 |
Data are expressed as mean ± SD (range, median).
Asterisks indicate statistical significance compared to the DSS + PBS-treated group as a positive control (*, P < 0.05;**, P < 0.01;***, P < 0.001; ANOVA with Dunnett's post hoc test).
|
miR-107 |
1.00 ± 0.49***b (0.40–1.73, 0.84) |
0.15 ± 0.15 (0.01–0.47, 0.12) |
0.39 ± 0.19 (0.21–0.68, 0.36) |
0.52 ± 0.20* (0.22–0.73, 0.57) |
miR-146a |
1.00 ± 0.41* (0.28–1.60, 1) |
2.44 ± 1.52 (1.10–6.40, 1.85) |
1.41 ± 0.76 (0.47–2.84, 1.18) |
1.18 ± 0.75* (0.38–2.49, 0.96) |
miR-155 |
1.00 ± 0.53** (0.44–1.72, 0.93) |
30.09 ± 17.35 (16.97–59.78, 21.53) |
8.42 ± 9.87* (0.95–26.86, 4.04) |
10.35 ± 11.06* (1.27–29.09, 8.49) |
miR-223 |
1.00 ± 0.35** (0.61–1.40, 1.01) |
3.40 ± 1.29 (1.97–5.56, 3.07) |
1.90 ± 0.91* (1.14–3.18, 1.47) |
2.10 ± 0.82 (0.94–3.10, 1.95) |
Alterations of cecal microbiota in mice with DSS-induced colitis using L. acidophilus treatment
Fig. 1 and Table 6 summarize the differences in cecal microbiota in mice with DSS-induced colitis due to L. acidophilus treatment. Compared to DSS + PBS-treated mice, the microbial communities in DSS + KBL402-treated and DSS + KBL409-treated mice exhibited abundant bacterial diversities and were distinctly clustered (Fig. 1A and B). A notable bacterial community in water + PBS-treated mice was the family S24-7_unclassified (50.1%) (Fig. 1C and D). However, in DSS + PBS-treated mice, a significant lower level of the family S24-7_unclassified was observed (4.6%) and the genus Bacteroides (34.4%) occupied the largest proportion. Clear upward tendency of the family S24-7_unclassified and downward trends of Bacteroides were discovered in DSS + KBL402-treated and DSS + KBL409-treated mice (Fig. 1C and D). Moreover, DSS + KBL402-treated mice showed significantly higher levels in genus Akkermansia (0.022 ± 0.017) compared to mice with DSS-induced colitis (0.007 ± 0.006) (P < 0.01) (Table 6). The relative abundance of genus Bacteroides and Mucispirillum were also clearly reduced in DSS + KBL402-treated [0.120 ± 0.082 (P < 0.001) and 0.027 ± 0.029 (P < 0.01), respectively] and DSS + KBL409-treated mice [0.103 ± 0.071 (P < 0.001) and 0.040 ± 0.040 (P < 0.05), respectively]. Finally, the genus Prevotella was significantly restored in DSS + KBL402-treated and DSS + KBL409-treated mice (0.010 ± 0.006 and 0.009 ± 0.006) (P < 0.01).
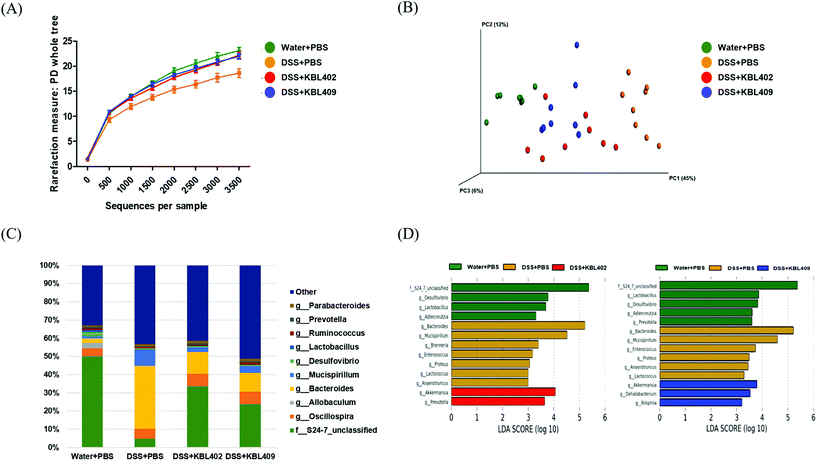 |
| Fig. 1 Differences in cecal microbiota using L. acidophilus administration. (A) Rarefaction plot. (B) Principal coordinate analysis plot with weighted UniFrac distances. (C) Taxonomic structures of cecal microbiota in the experimental groups. (D) Significantly different microbial taxa elucidated using the linear discriminant analysis (LDA) effect size (LEfSe) analysis (threshold >3.0). | |
Table 6 Differences in relative abundances of significantly different microbial taxa in cecal microbiota using L. acidophilus administration
Genus |
Experimental groupa |
Water + PBS |
DSS + PBS |
DSS + KBL402 |
DSS + KBL409 |
Data are expressed as mean ± SD (range, median).
Asterisks indicate statistical significance compared to the DSS + PBS-treated group as a positive control (*, P < 0.05; **, P < 0.01; ***, P < 0.001; ANOVA with Dunnett's post hoc test).
|
Akkermansia
|
0.001 ± 0.000 (0–0.001, 0.001) |
0.007 ± 0.006 (0.002–0.017, 0.005) |
0.022 ± 0.017**b (0.000–0.048, 0.025) |
0.012 ± 0.009 (0.003–0.028, 0.012) |
Bacteroides
|
0.026 ± 0.014*** (0.009–0.052, 0.024) |
0.345 ± 0.061 (0.224–0.526, 0.34) |
0.120 ± 0.082*** (0.034–0.277, 0.114) |
0.103 ± 0.071*** (0.021–0.263, 0.08) |
Mucispirillum
|
0.018 ± 0.013*** (0.006–0.016, 0.007) |
0.091 ± 0.061 (0.039–0.219, 0.066) |
0.027 ± 0.029** (0.003–0.069, 0.014) |
0.040 ± 0.040* (0.006–0.118, 0.019) |
Prevotella
|
0.009 ± 0.005** (0.001–0.016, 0.007) |
0.001 ± 0.001 (0.000–0.004, 0.001) |
0.010 ± 0.006** (0.002–0.016, 0.01) |
0.009 ± 0.006** (0.002–0.019, 0.006) |
Alterations of metabolites in mice with DSS-induced colitis using L. acidophilus treatment
Table 7 shows metabolites including amino acids and SCFAs in the ceca of mice with DSS-induced colitis. Overall, significantly lower concentrations of various amino acids including aspartic acid, cysteine, glutamine, glycine and serine were discovered in DSS + KBL402-treated and DSS + KBL409-treated mice compared to DSS + PBS-treated mice. Moreover, a significant reduction in the threonine concentrations was exhibited in mice with DSS-treated colitis after KBL409 treatment (100.24 ± 67.65 ng mg−1) (P < 0.05). Conversely, SCFAs including butyrate and propionate in the ceca from DSS + KBL402-treated mice (0.03 ± 0.02 ng mg−1 and 0.03 ± 0.01 ng mg−1, respectively) were significantly higher than DSS + PBS-treated mice (0.01 ± 0.01 ng mg−1 and 0.01 ± 0.01 ng mg−1, respectively) (P < 0.05). In DSS + KBL402-treated mice, the genus Lactobacillus showed significant correlations with acetate (P < 0.05) (Fig. 2). In DSS + KBL409-treated mice, the genus Prevotella also showed negative correlations with glycine and aspartic acid (P < 0.01 and P < 0.01, respectively) (Fig. 2).
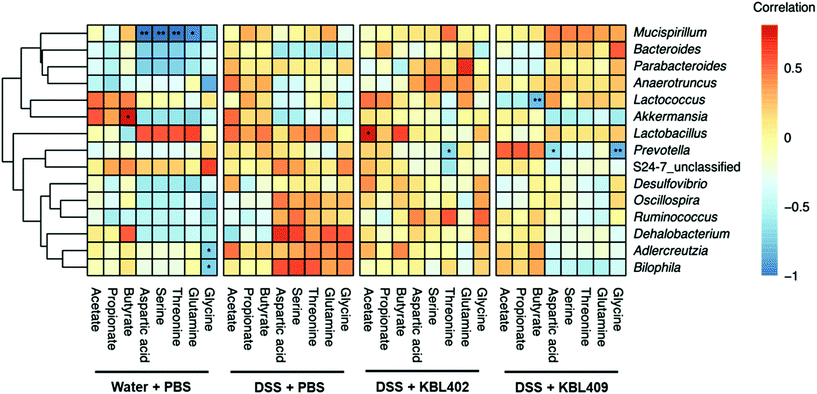 |
| Fig. 2 Heatmap of the Spearman's correlations between the relative abundances of genus level taxa and specific metabolites. The color of the matrix denotes the degree of correlation between the taxa and metadata variables. Asterisks indicate statistical significance (*, P < 0.05; **, P < 0.01). | |
Table 7 Differences in various amino acids and short chain fatty acids (SCFAs) using L. acidophilus administration
Metabolite (ng mg−1) |
Experimental groupa |
Water + PBS |
DSS + PBS |
DSS + KBL402 |
DSS + KBL409 |
Data are expressed as mean ± SD (range, median).
Asterisks indicate statistical significance compared to the DSS + PBS-treated group as a positive control (*, P < 0.05; **, P < 0.01; ***, P < 0.001; ANOVA with Dunnett's post hoc test).
|
Amino acid |
Aspartic acid |
64.69 ± 35.38**b (17.54–132.79, 68.71) |
282.30 ± 208.10 (13.7–824.73, 281.33) |
127.42 ± 89.00* (12.2–280.33, 105.62) |
104.79 ± 55.16** (30.06–199.89, 99.45) |
Cysteine |
0.00 ± 0.000* (0–0.01, 0.00) |
2.50 ± 3.41 (0.00–11.03, 1.34) |
0.14 ± 0.27* (0.00–0.79, 0.00) |
0.11 ± 0.35* (0.00–1.18, 0.00) |
Glutamine |
17.83 ± 11.56* (3.91–28.32, 19.72) |
95.52 ± 79.87 (0.00–348.81, 85.76) |
34.39 ± 29.94* (0.00–103.86, 27.82) |
39.36 ± 34.48* (3.50–123.55, 29.06) |
Glycine |
72.68 ± 42.41*** (32.35–152.66, 54.11) |
211.02 ± 108.95 (0.00–452.06, 222.52) |
85.26 ± 40.06*** (22.61–157.06, 76.85) |
88.03 ± 29.24*** (38.16–131.57, 87.81) |
Serine |
83.04 ± 34.41* (21.51–121.55, 90.35) |
184.97 ± 113.60 (0.00–434.35, 192.06) |
98.45 ± 27.16* (35.61–124.73, 105.24) |
106.49 ± 69.23* (33.1–226.86, 77.12) |
Threonine |
89.57 ± 32.09* (40.17–141.67, 91.16) |
184.25 ± 101.69 (0.00–388.43, 162.74) |
120.28 ± 57.64 (33.34–221.67, 96.52) |
100.24 ± 67.65* (35.78–247.37, 63.02) |
|
SCFA |
Acetate |
0.07 ± 0.00 (0.07–0.08, 0.07) |
0.03 ± 0.04 (0.00–0.08, 0.00) |
0.05 ± 0.04 (0.00–0.10, 0.08) |
0.05 ± 0.04 (0.00–0.10, 0.08) |
Butyrate |
0.02 ± 0.00 (0.02–0.02, 0.02) |
0.01 ± 0.01 (0.00–0.02, 0.01) |
0.03 ± 0.02** (0.02–0.07, 0.03) |
0.02 ± 0.01 (0.00–0.051, 0.02) |
Propionate |
0.03 ± 0.00 (0.02–0.03, 0.03) |
0.01 ± 0.01 (0.00–0.02, 0.02) |
0.03 ± 0.01** (0.00–0.04, 0.03) |
0.02 ± 0.01 (0.00–0.03, 0.02) |
Discussion
In this study, we demonstrated that L. acidophilus KBL402 and KBL409 treatment have a strong anti-inflammatory effect in mice with DSS-induced colitis. The mouse colitis model using DSS is a useful tool to elucidate the efficacy and mechanisms of probiotics for the alleviation of acute IBDs.23,24 After 7 days of DSS treatment, mice had severe damage in their colon with clinical symptoms including weight loss, diarrhea, and rectal bleeding.23,24,35 However, the symptoms of colitis in mice with L. acidophilus KBL402 or KBL409 treatment were significantly reduced (Table 1 and Fig. S1†).
Upregulation of pro- or inflammatory cytokines is a clear feature of IBDs.23,24 CCL2 is an important pro-inflammatory chemokine mainly produced by macrophages.36 CXCL1 is the main chemokine related to recruitment and activation of neutrophils.37 MPO is an important indicator of the infiltration of neutrophils.38 Moreover, the high levels of CCL2, CXCL1 and MPO were highly correlated with the increase in leukocyte infiltration.23,24 Therefore, L. acidophilus treatment can alleviate IBDs by modulating the secretion of inflammatory cytokines and inflammation-related molecules.
Moreover, a previous study reported that upregulation of IL-10 is closely related to the induction of Tregs.39 Induced Tregs in MLN via L. acidophilus treatment could successfully suppress acute colitis. However, further longitudinal studies using various in vivo IBD models and normal mice to elucidate fully the mechanisms of L. acidophilus treatments in a host should be performed.
Previous reports have suggested that miR expressions are highly associated with various important biological processes such as cell differentiation, proliferation, apoptosis, tumorigenesis, cell death and others.40,41 Moreover, miR expressions could be regulated in the microbial compositions of the host.40,41 A previous study suggested that miR-107 is strongly related with the downregulation of IL-6, IFN-γ and TNF-α and TGF-β promotion.12 It has been reported that miR-146a can suppress various gut barrier or inflammatory genes within the immune-related signaling pathways. In particular, the miR-146a knock-out mice have shown resistance to ulcerative colitis.14 miR-155 or miR-223 have been known as strong regulators in various immune cells including macrophage, Th1, and Th17 or modulators of the inflammasome complex and IL-1β production, respectively.13,15 Both miRs could increase in inflammatory conditions such as IBDs. Our results suggested the potential of L. acidophilus treatment for the improvement of colitis through modulation of miRs that are closely related to host inflammation.
Differences in the gut microbiota, including diversity and composition, are important for the progress of IBDs.23,24,42L. acidophilus KBL402 and KBL409 treatment can restore the microbial diversities of mice with DSS-induced colitis. The relative abundance of the family S24-7_unclassified was significantly higher and genus Bacteroides and Mucisprillum were clearly lower in L. acidophilus treated mice. Moreover, with L. acidophilus treatment, the higher relative abundance of genus Akkermansia and Prevotella was also discovered. Previous studies have suggested that genus Akkermansia can induce Foxp3+ Tregs and IL-10.24,43 Specific species in genus Prevotella have been reported to suppress inflammatory demyelinating diseases and multiple sclerosis successfully because they can induce Tregs and regulate cytokines.44,45
Furthermore, the higher levels of important SCFAs and several positive or negative correlations between metabolites and the relative abundances of genus level taxa, such as genus Lactobacillus and Prevotella, were discovered in mice with L. acidophilus treatment. Amino acids are important precursors of SCFAs, mainly produced by the gut microbiota.46 SCFAs such as acetate, butyrate, and propionate can play a role in the prevention and treatment of IBDs.3 Previous studies have reported that SCFAs contribute to various processes, including epithelial cell differentiation, Treg stimulation, and amelioration of mucosal inflammation, related to gut homeostasis.47–49 Butyrate can also exert beneficial effects in ulcerative colitis because it is the major energy source of the epithelium and can induce Tregs broadly.50L. acidophilus treatment clearly exhibits protective effects against IBDs via its clear effect on boosting SCFA production.
Therefore, we suggest that L. acidophilus treatment has various effects in mice with DSS-induced colitis, including the modulation of immune responses, control of the miR levels and restoration of the gut microbiota. However, this study only confirmed the short-term effects of L. acidophilus treatment for IBDs using the single mouse model. In particular, the extensive daily sampling of specimens, including stool and cecum, are needed to track the changes in the gut microbial compositions and their metabolites precisely. Therefore, to apply L. acidophilus treatment for the prevention and treatment of IBDs, further longitudinal studies using various cohorts of mice or different animal models should be performed with strong multi-omics tools to suggest the potential effects and secure mechanisms of L. acidophilus on various hosts.
Conclusion
In conclusion, L. acidophilus KBL402 and KBL409 treatment can significantly alleviate IBDs in various ways including the modulation of immune responses and miR expression, restoration of gut microbiota, and production of metabolites in the mouse model with DSS-induced colitis. Our results suggest the possibilities of L. acidophilus KBL402 and KBL409 as the useful tools for the prevention or treatment of IBDs and further longitudinal studies should be performed before the application of L. acidophilus KBL402 and KBL409 in various IBDs.
Conflicts of interest
GK is the founder of KoBioLabs, Inc., and SP and SJJ are the employees of KoBioLabs, Inc. The remaining authors declare that the research was conducted in the absence of any commercial or financial relationships that could be construed as a potential conflict of interest.
Acknowledgements
This work was supported by the National Research Foundation of Korea (NRF) grant funded by the Korean government (MSIP) (NRF-2015R1A2A1A10054078 and 2018R1A2A1A05078258).
References
- E. Thursby and N. Juge, Introduction to the human gut microbiota, Biochem. J., 2017, 474, 1823–1836 CrossRef CAS PubMed.
- J. M. M. Natividad and E. F. Verdu, Modulation of intestinal barrier by intestinal microbiota: Pathological and therapeutic implications, Pharmacol. Res., 2013, 69, 42–51 CrossRef CAS PubMed.
- G. Den Besten, K. Van Eunen, A. K. Groen, K. Venema, D. J. Reijngoud and B. M. Bakker, The role of short-chain fatty acids in the interplay between diet, gut microbiota, and host energy metabolism, J. Lipid Res., 2013, 54, 2325–2340 CrossRef CAS PubMed.
- H. J. Wu and E. Wu, The role of gut microbiota in immune homeostasis and autoimmunity, Gut Microbes, 2012, 3, 4–14 CrossRef PubMed.
- T. T. MacDonald and G. Monteleone, Immunity, inflammation, and allergy in the gut, Science, 2005, 307, 1920–1925 CrossRef CAS PubMed.
- Z. L. Dai, G. Wu and W. Y. Zhu, Amino acid metabolism in intestinal bacteria: Links between gut ecology and host health, Front. Biosci., 2011, 1, 1768–1786 CrossRef PubMed.
- E. P. J. G. Neis, C. H. C. Dejong and S. S. Rensen, The role of microbial amino acid metabolism in host metabolism, Nutrients, 2015, 7, 2930–2946 CrossRef CAS PubMed.
- R. J. Vonk, M. Priebe, K. Meijer, K. Venema and H. Roelofsen, The interaction of short-chain fatty acids (SCFA) with adipose tissue; relevance for systemic inflammation, Gastroenterology, 2011, 140, S860 CrossRef.
- K. L. Wallace, L. B. Zheng, Y. Kanazawa and D. Q. Shih, Immunopathology of inflammatory bowel disease, World J. Gastroenterol., 2014, 20, 6–21 CrossRef CAS PubMed.
- X. M. Xu and H. J. Zhang, MiRNAs as new molecular insights into inflammatory bowel disease: Crucial regulators in autoimmunity and inflammation, World J. Gastroenterol., 2016, 22, 2206–2218 CrossRef CAS PubMed.
- A. T. Filip, C. Marian, A. Anghel and O. Balacescu, Microbiota small RNAs in inflammatory bowel disease, J. Gastrointest. Liver Dis., 2016, 25, 509–516 CrossRef PubMed.
- X. Xue, A. T. Cao, X. Cao, S. Yao, E. D. Carlsen, L. Soong, C. G. Liu, X. Liu, Z. Liu, L. W. Duck, C. O. Elson and Y. Cong, Downregulation of microRNA-107 in intestinal CD11c+ myeloid cells in response to microbiota and proinflammatory cytokines increases IL-23p19 expression, Eur. J. Immunol., 2014, 44, 673–682 CrossRef CAS PubMed.
- U. P. Singh, A. E. Murphy, R. T. Enos, H. A. Shamran, N. P. Singh, H. Guan, V. L. Hegde, D. Fan, R. L. Price, D. D. Taub, M. K. Mishra, M. Nagarkatti and P. S. Nagarkatti, miR-155 deficiency protects mice from experimental colitis by reducing T helper type 1/type 17 responses, Immunology, 2014, 143, 478–489 CrossRef CAS PubMed.
- M. C. Runtsch, R. Hu, M. Alexander, J. Wallace, D. Kagele, C. Petersen, J. F. Valentine, N. C. Welker, M. P. Bronner, X. Chen, D. P. Smith, N. J. Ajami, J. F. Petrosino, J. L. Round and R. M. O'Connell, MicroRNA-146a constrains multiple parameters of intestinal immunity and increases susceptibility to DSS colitis, Oncotarget, 2015, 6, 28556–28572 CrossRef PubMed.
- M. Haneklaus, M. Gerlic, M. Kurowska-Stolarska, A.-A. Rainey, D. Pich, I. B. McInnes, W. Hammerschmidt, L. A. J. O'Neill and S. L. Masters, Cutting Edge: miR-223 and EBV miR-BART15 Regulate the NLRP3 Inflammasome and IL-1 Production, J. Immunol., 2012, 189, 3795–3799 CrossRef CAS PubMed.
- K. Knight-sepulveda, S. Kais, R. Santaolalla and M. T. Abreu, Diet and Inflammatory Bowel Disease, Gastroenterol. Hepatol., 2015, 11, 511–520 Search PubMed.
- D. Owczarek, T. Rodacki, R. Domagała-rodacka, D. Cibor, T. Mach, D. Owczarek and R. Domagała-rodacka, Diet and nutritional factors in inflammatory bowel diseases, World J. Gastroenterol., 2016, 22, 895–905 CrossRef CAS PubMed.
- F. Durchschein, W. Petritsch, H. F. Hammer, F. Durchschein, W. Petritsch and F. Heinz, Diet therapy for inflammatory bowel diseases: the established and the new, World J. Gastroenterol., 2016, 22, 2179–2194 CrossRef CAS PubMed.
- S. De Roock, M. Van Elk, M. E. A. Van Dijk, H. M. Timmerman, G. T. Rijkers, B. J. Prakken, M. O. Hoekstra and I. M. De Kleer, Lactic acid bacteria differ in their ability to induce functional regulatory T cells in humans, Clin. Exp. Allergy, 2009, 40, 103–110 Search PubMed.
- M. C. Hua, T. Y. Lin, M. W. Lai, M. S. Kong, H. J. Chang and C. C. Chen, Probiotic Bio-Three induces Th1 and anti-inflammatory effects in PBMC and dendritic cells, World J. Gastroenterol., 2010, 16, 3529–3540 CrossRef CAS PubMed.
- K. M. Lammers, P. Brigidi, B. Vitali, P. Gionchetti, F. Rizzello, E. Caramelli, D. Matteuzzi and M. Campieri, Immunomodulatory effects of probiotic bacteria DNA: IL-1 and IL-10 response in human peripheral blood mononuclear cells, FEMS Immunol. Med. Microbiol., 2003, 38, 165–172 CrossRef CAS PubMed.
- J. Park, I. Joe, P. D. Rhee, C. Jeong and G. Jeong, A lactic acid bacterium isolated from kimchi ameliorates intestinal inflammation in DSS-induced colitis, J. Microbiol., 2017, 55, 1–7 CrossRef PubMed.
- W. K. Kim, Y. J. Jang, B. Seo, D. H. Han, S. J. Park and G. P. Ko, Administration of Lactobacillus paracasei, strains improves immunomodulation and changes the composition of gut microbiota leading to improvement of colitis in mice, J. Funct. Foods, 2019, 6, 92 CAS.
- Y. J. Jang, W. K. Kim, D. H. Han, K. Lee and G. Ko,
Lactobacillus fermentum species ameliorate dextran sulfate sodium-induced colitis by regulating the immune response and altering gut microbiota, Gut Microbes, 2019, 10, 696–711 CrossRef CAS PubMed.
- W. K. Kim, Y. J. Jang, D. H. Han, B. Seo, S. Park, C. H. Lee and G. Ko, Administration of lactobacillus fermentum KBL375 causes taxonomic and functional changes in gut microbiota leading to improvement of atopic dermatitis, Front. Mol. Biosci., 2019, 6, 1 CrossRef PubMed.
- P. Alex, N. C. Zachos, T. Nguyen, L. Gonzales, T. E. Chen, L. S. Conklin, M. Centola and X. Li, Distinct cytokine patterns identified from multiplex profiles of murine DSS and TNBS-induced colitis, Inflamm. Bowel Dis., 2009, 15, 341–352 CrossRef PubMed.
- A. M. Ghaleb, B. B. McConnell, K. H. Kaestner and V. W. Yang, Altered intestinal epithelial homeostasis in mice with intestine-specific deletion of the Krüppel-like factor 4 gene, Dev. Biol., 2011, 349, 310–320 CrossRef CAS PubMed.
- E. Akgun, C. Çaliskan, H. A. Celik, A. O. Ozutemiz, M. Tuncyurek and H. H. Aydin, Effects of N-acetylcysteine treatment on oxidative stress in acetic acid-induced experimental colitis in rats, J. Int. Med. Res., 2005, 33, 196–206 CrossRef CAS PubMed.
- W. Walters, E. R. Hyde, D. Berg-Lyons, G. Ackermann, G. Humphrey, A. Parada, J. A. Gilbert, J. K. Jansson, J. G. Caporaso, J. A. Fuhrman, A. Apprill and R. Knight, Improved Bacterial 16S rRNA Gene (V4 and V4-5) and Fungal Internal Transcribed Spacer Marker Gene Primers for Microbial Community Surveys, m Systems, 2015, 1, e00009–e00015 Search PubMed.
- J. G. Caporaso, J. Kuczynski, J. Stombaugh, K. Bittinger, F. D. Bushman, E. K. Costello, N. Fierer, A. G. Pẽa, J. K. Goodrich, J. I. Gordon, G. A. Huttley, S. T. Kelley, D. Knights, J. E. Koenig, R. E. Ley, C. A. Lozupone, D. McDonald, B. D. Muegge, M. Pirrung, J. Reeder, J. R. Sevinsky, P. J. Turnbaugh, W. A. Walters, J. Widmann, T. Yatsunenko, J. Zaneveld and R. Knight, QIIME allows analysis of high-throughput community sequencing data, Nat. Methods, 2010, 7, 335–336 CrossRef CAS PubMed.
- M. G. I. Langille, J. Zaneveld, J. G. Caporaso, D. McDonald, D. Knights, J. A. Reyes, J. C. Clemente, D. E. Burkepile, R. L. Vega Thurber, R. Knight, R. G. Beiko and C. Huttenhower, Predictive functional profiling of microbial communities using 16S rRNA marker gene sequences, Nat. Biotechnol., 2013, 31, 814–821 CrossRef CAS PubMed.
- N. Segata, J. Izard, L. Waldron, D. Gevers, L. Miropolsky, W. S. Garrett and C. Huttenhower, Metagenomic biomarker discovery and explanation, Genome Biol., 2011, 12, R60 CrossRef PubMed.
- V. F. Roucher, E. Desnots, C. Naël, A. M. Agnoux, M. C. Alexandre-Gouabau, D. Darmaun and C. Y. Boquien, Use of UPLC-ESI-MS/MS to quantitate free amino acid concentrations in micro-samples of mammalian milk, SpringerPlus, 2013, 2, 622 CrossRef PubMed.
- L. A. David, C. F. Maurice, R. N. Carmody, D. B. Gootenberg, J. E. Button, B. E. Wolfe, A. V. Ling, A. S. Devlin, Y. Varma, M. A. Fischbach, S. B. Biddinger, R. J. Dutton and P. J. Turnbaugh, Diet rapidly and reproducibly alters the human gut microbiome, Nature, 2014, 505, 559–563 CrossRef CAS PubMed.
- W. K. Kim, J. S. Park, O. J. Sul, J. H. Seo, B. K. Choi, H. Y. Park, A. M. Latour, B. H. Koller, B. S. Kwon and C. S. Jeong, Role of TNFR-related 2 mediated immune responses in dextran sulfate sodium-induced inflammatory bowel disease, Mol. Cells, 2011, 31, 99–104 CrossRef CAS PubMed.
- E. T. Wong and V. Tergaonkar, Roles of NF-κB in health and disease: mechanisms and therapeutic potential, Clin. Sci., 2009, 116, 451–465 CrossRef CAS PubMed.
- K. V. Sawant, K. M. Poluri, A. K. Dutta, K. M. Sepuru, A. Troshkina, R. P. Garofalo and K. Rajarathnam, Chemokine CXCL1 mediated neutrophil recruitment: Role of glycosaminoglycan interactions, Sci. Rep., 2016, 6, 33123 CrossRef CAS PubMed.
- Y. Yan, V. Kolachala, G. Dalmasso, H. Nguyen, H. Laroui, S. V. Sitaraman and D. Merlin, Temporal and spatial analysis of clinical and molecular parameters in dextran sodium sulfate induced colitis, PLoS One, 2009, 4, e6073 CrossRef PubMed.
- H. H. Smits, A. Engering, D. Van Der Kleij, E. C. De Jong, K. Schipper, T. M. M. Van Capel, B. A. J. Zaat, M. Yazdanbakhsh, E. A. Wierenga, Y. Van Kooyk and M. L. Kapsenberg, Selective probiotic bacteria induce IL-10-producing regulatory T cells in vitro by modulating dendritic cell function through dendritic cell-specific intercellular adhesion molecule 3-grabbing nonintegrin, J. Allergy Clin. Immunol., 2005, 115, 1260–1267 CrossRef CAS PubMed.
- E. A. Miska, How microRNAs control cell division, differentiation and death, Curr. Opin. Genet. Dev., 2005, 15, 563–568 CrossRef CAS PubMed.
- R. Schickel, B. Boyerinas, S. M. Park and M. E. Peter, MicroRNAs: Key players in the immune system, differentiation, tumorigenesis and cell death, Oncogene, 2008, 27, 5959–5974 CrossRef CAS PubMed.
- J. Ni, G. D. Wu, L. Albenberg and V. T. Tomov, Gut microbiota and IBD: Causation or correlation?, Nat. Rev. Gastroenterol. Hepatol., 2017, 14, 573–584 CrossRef PubMed.
- N. R. Shin, J. C. Lee, H. Y. Lee, M. S. Kim, T. W. Whon, M. S. Lee and J. W. Bae, An increase in the Akkermansia spp. population induced by metformin treatment improves glucose homeostasis in diet-induced obese mice, Gut, 2014, 63, 727–735 CrossRef CAS PubMed.
- A. Mangalam, S. K. Shahi, D. Luckey, M. Karau, E. Marietta, N. Luo, R. S. Choung, J. Ju, R. Sompallae, K. Gibson-Corley, R. Patel, M. Rodriguez, C. David, V. Taneja and J. Murray, Human Gut-Derived Commensal Bacteria Suppress CNS Inflammatory and Demyelinating Disease, Cell Rep., 2017, 20, 1269–1277 CrossRef CAS PubMed.
- S. K. Shahi, S. N. Freedman, A. C. Murra, K. Zarei, R. Sompallae, K. N. Gibson-Corley, N. J. Karandikar, J. A. Murray and A. K. Mangalam,
Prevotella histicola, a human gut commensal, is as potent as COPAXONE® in an animal model of multiple sclerosis, Front. Immunol., 2019, 10, 462 CrossRef CAS PubMed.
- A. Basson, A. Trotter, A. Rodriguez-Palacios and F. Cominelli, Mucosal interactions between genetics, diet, and microbiome in inflammatory bowel disease, Front. Immunol., 2016, 7, 290 Search PubMed.
- A. Hartog, F. N. Belle, J. Bastiaans, P. De Graaff, J. Garssen, L. F. Harthoorn and A. P. Vos, A potential role for regulatory T-cells in the amelioration of DSS induced colitis by dietary non-digestible polysaccharides, J. Nutr. Biochem., 2015, 26, 227–233 CrossRef CAS PubMed.
- K. Machiels, M. Joossens, J. Sabino, V. De Preter, I. Arijs, V. Eeckhaut, V. Ballet, K. Claes, F. Van Immerseel, K. Verbeke, M. Ferrante, J. Verhaegen, P. Rutgeerts and S. Vermeire, A decrease of the butyrate-producing species roseburia hominis, and faecalibacterium prausnitzii defines dysbiosis in patients with ulcerative colitis, Gut, 2014, 63, 1275–1283 CrossRef CAS PubMed.
- L. P. Smits, K. E. C. Bouter, W. M. De Vos, T. J. Borody and M. Nieuwdorp, Therapeutic potential of fecal microbiota transplantation, Gastroenterology, 2013, 145, 946–953 CrossRef PubMed.
- Y. Furusawa, Y. Obata, S. Fukuda, T. A. Endo, G. Nakato, D. Takahashi, Y. Nakanishi, C. Uetake, K. Kato, T. Kato, M. Takahashi, N. N. Fukuda, S. Murakami, E. Miyauchi, S. Hino, K. Atarashi, S. Onawa, Y. Fujimura, T. Lockett, J. M. Clarke, D. L. Topping, M. Tomita, S. Hori, O. Ohara, T. Morita, H. Koseki, J. Kikuchi, K. Honda, K. Hase and H. Ohno, Commensal microbe-derived butyrate induces the differentiation of colonic regulatory T cells, Nature, 2013, 504, 446–450 CrossRef CAS PubMed.
Footnotes |
† Electronic supplementary information (ESI) available. See DOI: 10.1039/d0fo01724h |
‡ These authors contributed equally to this work. |
|
This journal is © The Royal Society of Chemistry 2021 |