DOI:
10.1039/D1AY90094C
(AMC Technical Brief)
Anal. Methods, 2021,
13, 3731-3734
Hand-held X-ray fluorescence analysis of archaeological artefacts: challenges, advantages and limitations
Received
5th July 2021
First published on 12th August 2021
Abstract
Hand-held X-ray fluorescence spectroscopy has brought great advances in the study of cultural heritage materials, allowing fast, non-invasive and non-destructive analysis of artworks or works of art on-site, particularly in museums, conservation studios, and during archaeological excavations. This Technical Brief discusses the main advantages and limitations of the technique applied to archaeological artefacts, together with its potential to answer research questions for archaeologists and conservators.
X-ray fluorescence (XRF) spectroscopy is a technique used to determine the elemental compositions of a wide range of materials. Being non-destructive and non-invasive, XRF is ideal for applications in the cultural heritage sector. In the last decades, technological developments in X-ray generation and detection have led to the production and commercialisation of hand-held and other types of portable XRF instruments, which can perform fast and reliable analysis of objects without needing to collect samples. Studying artworks or works of art and collections in situ removes the need to transport delicate and valuable objects from a museum/conservation studio to the laboratory. Among the portable XRF instruments, hand-held devices are particularly useful as their small size and ease of handling facilitates their use in the analysis of large or immovable objects, even where access is difficult (e.g. temporary scaffolding in historical buildings), and at archaeological sites. They are also fast, as a single measurement can be obtained within a minute, and cost-effective compared with bench-top equipment or other techniques.
Applications
The results obtained from the analysis of archaeological artefacts by hand-held XRF can help to answer many of the research questions raised by archaeologists, curators and conservators:
(1) The general composition of a material can be quickly determined through qualitative analysis, taking into account the detection limits of each element. In archaeology, this allows rapid grouping of artefacts from the same class of material (identifying specific types of copper alloy, glass, stone, etc.), prior to carrying out typological studies or conservation.
(2) A small number of key elements may be enough to identify a material, such as a pigment in a paint layer, and therefore obtain information about date or provenance, or to recognise a hazard, such as lead contamination in soil.
(3) Hand-held XRF can help in the preliminary attribution of small pieces to a main object or different phases of repair, reconstructing the integrity of an artefact.
(4) Trace element and isotopic analyses are often required to fully answer questions about specific construction technology, provenance, dating, and studies of the state of preservation of the assemblage. However, they often require sampling. In these situations, hand-held XRF can help select the most suitable physical samples required for subsequent quantitative analysis.
The XRF method
A number of recently published AMC Technical Briefs (no. 77 and 89) have discussed hand-held XRF spectrometry in detail.1 In summary, using this technique the surface of an object is irradiated with an X-ray beam. This results in the displacement of some electrons from their atomic orbital positions. Electrons in outer orbitals fill the gaps created in the vacated lower orbitals, releasing X-rays in the process. This X-ray energy is characteristic for each specific element, and is detected and displayed as a spectrum of intensity against energy. In an XRF spectrum the position of the peaks and their intensity identify the elements present in the material and their abundance, respectively.
Elements with a low atomic number (Z) emit low-energy fluorescence, whilst higher Z elements can produce fluorescence at both high and low energies, according to their atomic structure. The intensity of the X-rays decreases as they exit the object (the matrix), and pass through the air between the object’s surface and the X-ray detector. To increase the sensitivity towards low Z elements and reduce the amount of air in the beam path, a portable vacuum pump or helium gas purge can be used, improving the detection efficiency. Nevertheless, low Z elements, such as hydrogen, oxygen and carbon, cannot be detected with hand-held XRF, and detection may be poor or unreliable for elements lighter than calcium, depending on the situation.
Portable XRF suppliers offer different types of stand, sample mounts and portable enclosed chambers for their instruments, so that they can be adapted to specialised uses, analysing liquids, powders, or fragile objects, and most provide technical guidance notes available through their websites.
Further information on using hand-held and portable XRF instruments within the cultural heritage sector is given in ref. 2 and 3.
Experimental aspects to consider
Despite the simple data acquisition process with hand-held XRF, specific knowledge of the basic principles of the technique and the phenomena occurring when a surface interacts with X-rays is essential. This will help the operator to select appropriate calibration procedures, and the instrument parameters for collecting and interpreting the spectra, with particular regard to matrix correction, the attribution of the peaks to specific elements (peaks from different elements may overlap) and the evaluation of instrumental artefacts and anomalies that give rise to potentially misleading results. The accuracy and repeatability precision of the results can be checked by analysing certified reference materials (for example the CHARM set for the analysis of historical copper alloys),4 with general composition and certified analyte concentration similar to that of the object being studied, and comparing the results. This is particularly important for quantitative analysis, which always requires specialist assessment.
XRF is a surface technique and the penetration depth of the X-rays varies according to: the composition of the material; the voltage used during the measurement; and, the particular analyte element. For this reason, misleading results can be obtained if the surface is not cleaned or polished, to remove soil, concretions, paint, or weathered layers (the majority of archaeological artefacts are characterised by altered surfaces).
The results will be affected if an object is not sufficiently thick to absorb all of the X-rays from the XRF beam. The necessary thickness will differ depending on the material, and will be greater for low density materials like plastics or paper. Objects made of different layers, like paintings or glazed ceramics, require particular attention, as the X-rays may penetrate several layers of material and the results will contain a mixture of chemical information from all of them. In ceramics, this could include a glaze, underglaze pigment, slip and ceramic fabric. In paintings, the spectrum of the canvas support can be overlaid with the spectrum of the paint layers in order to identify which elements in the spectrum of the paint have contributions from the background (canvas).
Most hand-held XRF instruments have a spot size of about 3–8 mm in diameter, which in some instruments can be tailored according to the object being studied. If the area of interest is smaller than the spot size of the instrument, the spectra will show the features of additional components. For this reason, a larger aperture is suitable for homogeneous materials, while a smaller aperture is more appropriate to study objects with fine details. The X-ray beam may also not be uniform across the area being analysed.
Other important parameters to consider are the distance between the instrument and the object, and the object’s geometry and topography. Most hand-held instruments need to be used in direct contact with the artefact, ideally on an area with a uniform, flat surface. However, sometimes this is not possible, and the increased distance results in a reduction of the collected signal, mainly due to attenuation of the low energy X-rays from low atomic number elements. If the object exhibits surface roughness or a complex shape, a similar reduction of the signal occurs.
It is essential that hand-held XRF operators are fully trained in the safe use of the instrument because the method makes use of ionising radiation. In the heritage sector, analytical work will often involve analysing different types of material in widely varying situations, and in each case the operator needs to assess and mitigate the risks to themselves and others, seek advice as necessary, and keep a record of the action taken.
Case studies in archaeology
Leather book cover from the London wreck
The London was a warship, which sank in March 1665, following an explosion. More than 700 objects were recovered from the seabed, among them a very fragile leather book cover with patches of surviving decoration (Fig. 1). Hand-held XRF was used to investigate the chemical composition of the decoration and identify specific gold and red pigments.5 The analysis confirmed the presence of gold (Au), and detected lead (Pb) and mercury (Hg) in the red paint, suggesting the use of cinnabar/vermilion (HgS natural and synthetic, respectively) and red lead (Pb3O4) pigments (Fig. 1). Red lead is often found together with other red pigments, and in this case the mixture is the result of the adulteration of expensive vermilion with a cheaper pigment. The presence of iron (Fe) is most probably due to post-burial contamination, as iron was also detected in the undecorated areas. The use of gold leaf and vermillion proves the high value of this artefact both in the past and present, as it is very rare to find residues of pigments on archaeological artefacts from Northern Europe.
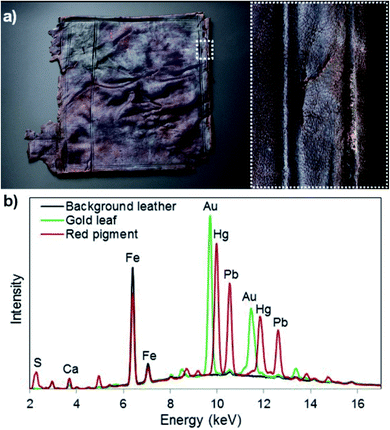 |
| Fig. 1 (a) Leather book cover from the London shipwreck; (b) XRF spectra from the background leather, the gold leaf and the red paint. All images © Historic England. | |
Copper alloy pan from Rooswijk shipwreck
The Rooswijk was an 18th-century Dutch East India Company ship, which was wrecked off the Kent coast in 1740. During the excavation of the shipwreck many objects were recovered, including intact metal artefacts that would not fit inside a benchtop XRF machine. So these were investigated with hand-held XRF to identify the manufacturing process, the alloy composition and the state of preservation, in order to design the most appropriate conservation strategies.6 A copper alloy pan recovered from the Rooswijk was analysed, prior to carrying out desalination and surface cleaning (Fig. 2). Three parts of the pan were investigated: the base, the rim, and the rivets. Where possible, small selected areas were gently abraded and cleaned to expose unaltered metal. The rim and the base are brass with a composition of about 66 g per 100 g copper (Cu), 27 g per 100 g zinc (Zn), 2.3 g per 100 g lead (Pb) and traces of arsenic (As) and nickel (Ni) (Fig. 2). Lead and arsenic are difficult to quantify when they occur together because their characteristic X-rays have overlapping energies. The rivets are mainly copper. Iron (Fe) was also detected in quite high concentrations on the surface because the object had been excavated from a concretion formed around a nearby corroded iron artefact.
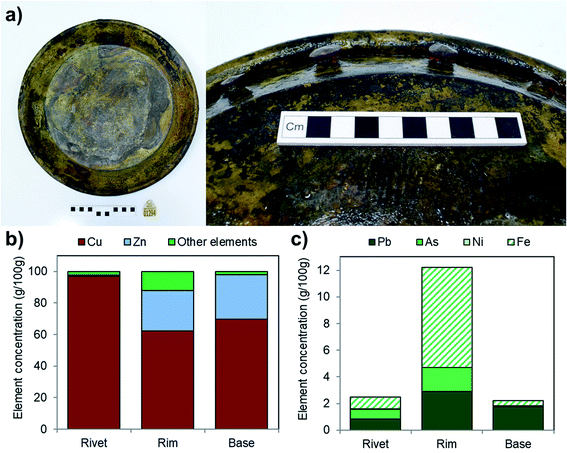 |
| Fig. 2 (a) Copper-alloy pan from the Rooswijk shipwreck; (b) and (c) concentration of the elements in the alloys of the rivets, rim and base of the pan. © Rooswijk1740 project. | |
Recent developments in portable XRF line and area scans
Hand-held XRF analysers can perform single point analysis, particularly suitable to investigate homogenous areas on artefacts. For larger, heterogeneous objects, portable instruments can be coupled with motorised xyz stages, which allow the collection of line or area maps. Spectra are collected at regular fixed intervals and the intensity of the peak for a specific element is evaluated at each interval against the distance along the line/area. This generates spatially resolved elemental information, detecting the presence of a specific element across a region of particular interest. Macro-XRF (MA-XRF) scanners are widely used to investigate artworks or works of art, as they can produce large-scale elemental maps, collecting compositional data from an entire surface in a completely non-destructive way.7 This method has been applied successfully in situ to detect the distribution of pigments in mural paintings, to evaluate the pictorial technique and state of preservation. On stained-glass windows, it was used to answer historical and structural questions about the glass composition, structural integrity of the windows, and to evaluate glass alteration.
An important advantage of this method is that it presents the chemical data in a visual way, rather than as a plot, so that the results can be communicated more easily to non-specialist audiences.
The #Rooswijk1740 project is funded and led by the Cultural Heritage Agency of the Netherlands (Ministry of Education, Science and Culture), in collaboration with project partner Historic England and UK-contractor MSDS Marine.
Francesca Gherardi (Investigative science, Historic England, Fort Cumberland, Portsmouth).
This Technical Brief was prepared for the Analytical Methods Committee (AMC), with contributions from members of the AMC Heritage Science Expert Working Group, and approved by the AMC on 19 May 2021.
Further reading
-
Analytical Methods Committee Technical Briefs, RSC Publishing Themed Collection, http://rsc.li/amctb Search PubMed.
-
A. Bezur, L. Lee, M. Loubser and K. Trentelman, Hand-held XRF in Cultural Heritage: A Practical Workbook for Conservators, Getty Conservation Institute, 2019 Search PubMed.
-
N. Shugar and J. L. Mass, Hand-held XRF for Art and Archaeology, Cornell University Press, 2012 Search PubMed.
-
A. Heginbotham and V. Armando Solé, Charmed Pymca, Part I : A Protocol for Improved Inter-laboratory Reproducibility in the Quantitative ED-XRF Analysis of Copper Alloys, http://www.getty.edu/museum/conservation/papers/pymca.html.
-
The London wreck – a kaleidoscope of specialists, materials and artefacts, Historic England Research Issue 16. July 2020, pp. 70–81. https://historicengland.org.uk/whats-new/research/the-london-wreck-a-kaleidoscope-of-specialists-materials-and-artefacts/ Search PubMed.
-
E. Kuiper, Conservation of copper alloy artefacts from the Dutch East Indiaman the Rooswijk, Research Department Reports, Historic England, 2018, vol. 36, https://research.historicengland.org.uk/Report.aspx?i=16039%26ru=%2FResults.aspx%3Fp%3D176 Search PubMed.
- M. Alfeld, J. V. Petroso, M. van Eikema Hommes, G. Van der Snickt, G. Tauber, J. Blaas, M. Haschke, K. Erler, D. Joris and K. Janssens, A mobile instrument for in situ scanning macro-XRF (MA-XRF) investigation of historical paintings, J. Anal. At. Spectrom., 2013, 28(5), 760–767 RSC.
|
This journal is © The Royal Society of Chemistry 2021 |