DOI:
10.1039/D0AN01949F
(Paper)
Analyst, 2021,
146, 296-304
Quantitative and ultrasensitive detection of brombuterol by a surface-enhanced Raman scattering (SERS)-based lateral flow immunochromatographic assay (FLIA) using AgMBA@Au–Ab as an immunoprobe
Received
30th September 2020
, Accepted 12th October 2020
First published on 26th October 2020
Abstract
Brombuterol is a new emerging β-adrenergic agonist that has been used as an additive in animal feed to enhance the lean meat-to-fat ratio. Due to its potential harm to consumers, it is urgent to develop sensitive, simple and rapid analytical methods to monitor brombuterol residue. In this study, a competitive lateral flow immunochromatographic assay (FLIA) based on surface-enhanced Raman scattering (SERS) was developed for ultrasensitive quantitative determination of brombuterol in swine liver, pork and feed samples. Ag@Au core–shell bimetallic nanoparticles with the highest SERS enhancement were synthesized, characterized and used as the substrate for preparation of the immunoprobe AgMBA@Au–Ab, in which the Raman reporter mercaptobenzoic acid (MBA) was embedded between the core–shell layers and monoclonal antibodies against brombuterol were immobilized on the surfaces of nanoparticles. The presence of brombuterol was identified through a color change on testing lines. In addition, quantitative detection of brombuterol was achieved by measuring the characteristic Raman peak intensity of MBA in the immunoprobes captured by the coating antigen. The IC50 and limit of detection (LOD) of the SERS-based FLIA for brombuterol were 45 pg mL−1 and 0.11 pg mL−1, respectively. The recoveries of brombuterol from spiked samples were in the range of 87.27–100.16% with relative standard deviations of 1.29%–6.99% (n = 3). The proposed SERS-based LFIA was proven to be a feasible method for ultrasensitive and rapid detection of brombuterol and might be a platform for sensitive and rapid detection of a broad range of analytes in clinical, environmental and food analyses.
Introduction
β-Adrenergic agonists, a family of synthetic phenethanolamine compounds mainly including clenbuterol, salbutamol and ractopamine (Table 1), are therapeutically administered to treat asthma and bronchial diseases in humans and animals.1,2 However, due to their potential roles in reducing animal fat levels and increasing the amount of muscle in livestock, β-agonists have been illegally used as animal growth promoters to increase daily weight gain.3 β-Agonist compounds are easily stored in human tissues after meat consumption and result in many serious health problems in consumers, with symptoms such as cardiac palpitations, tachycardia, nervousness, muscle tremors and confusion. Poisoning incidents resulting from high concentrations of β-agonist residue in edible tissue have occurred in some countries, such as Portugal, Australia, America and China.4,5 Therefore, β-agonists were prohibited as feed additives for livestock in many countries.6 Recently a new kind of β-agonist called brombuterol (Table 1) has emerged as a substitute for clenbuterol, salbutamol and ractopamine to evade detection by routine screening methods.7 To protect food safety and safeguard human health, it is very important and urgent to develop sensitive, simple, rapid and effective methods for monitoring brombuterol residue.
Table 1 The cross-reactivity (CR) values of the SERS-LFIA with the tested compounds
Compound |
Chemical structure |
IC50 (ng mL−1) |
CR (%) |
Brombuterol |
|
0.045 |
100 |
Clenbuterol |
|
0.129 |
34.88 |
Salbutamol |
|
9.182 |
0.49 |
|
Ractopamine |
|
19.09 |
0.24 |
Phenylethylamine A |
|
>100 |
<0.01 |
Phenylephrine |
|
>100 |
<0.01 |
Isoprenaline |
|
>100 |
<0.01 |
The main methods used for the detection of brombuterol in biological and food samples include gas chromatography-mass spectrometry,8 liquid chromatography-tandem mass spectrometry (LC-MS/MS),9 liquid chromatography with electrospray ionization tandem mass spectrometry (LC-ESI MS),10 ultra-high performance liquid chromatography with tandem mass spectrometry (UHPLC-LTQ Orbitrap MS),11 multiple signal amplified electrochemiluminescent immunoassay,12,13 and enzyme-linked immunosorbent assays (ELISAs).14 Although the chromatographic methods mentioned above exhibit the advantages of high accuracy and high sensitivity, they also have disadvantages, such as complicated performance, expensive instruments and the need for specialized technicians. ELISA shows high selectivity and sensitivity, but its operation is time-consuming and laborious. The electrochemical methods are disturbed by many interferents.
Over the past few decades, there has been an increasing demand for the development of point-of-care (POC) testing devices with high sensitivity and specificity for diagnostic purposes and other applications. Among various POC testing platforms, membrane-based lateral flow immunochromatographic assay (LFIA) has emerged as a popular technology and a powerful tool for rapid screening due to its simplicity, short time to obtain test results, user-friendly format, low cost, and long-term stability.15,16 Colloidal gold nanoparticles are the most commonly used labels in LFIAs because they are red in colour due to localized surface plasmon resonance. However, because of their low sensitivity, colloidal gold based LFIAs are frequently associated with subjective judgment errors.17,18 Therefore, improving the sensitivity and quantification capabilities of LFIAs could have a significant impact on the application of this technology.
In the last years, attempts to achieve sufficient sensitivity in LFIAs have included the integration of various nanomaterials, such as magnetic particles,19 carbon nano-particles,20 fluorescent microspheres,21 quantum dots,22 up-conversion fluorescence23 and lanthanide nanoparticles.24 When combined with a portable test strip reader for optical, magnetic, and electrochemical signals, LFIAs can accomplish rapid quantitative detection of the targets. As the concentration of target analytes is usually very low, most of the existing LFIAs described above are still troubled in some way by poor sensitivity and low accuracy.25
LFIA in combination with surface-enhanced Raman scattering (SERS) is a new and highly promising technology platform.26–29 SERS-based LFIAs using antibodies (Ab) conjugated to molecularly functionalized plasmonic nanoparticles (NPs) with narrow Raman fingerprints can overcome the above-mentioned problems faced in conventional LFIAs as they combine high sensitivity, quantification, and multiplexing.30–33 Synthesizing SERS substrates with higher Raman enhancement is a key factor in improving the sensitivity and quantification capabilities of SERS-LFIAs. The major contribution to SERS enhancement is attributed to the enhanced local electromagnetic field around the noble metal surface caused by localized surface plasmon resonance under the excitation of incident light. In particular, the redistribution of the local electromagnetic field around rough metal surfaces, nanogaps between close nanoparticles, and the sharp tips of nanostructures, termed “hot spots”, lead to extraordinarily enhanced SERS signals.
In SERS-LFIAs, Au and Ag NPs are the most widely used optical enhancement substrates.26–33 Generally, Au NPs display the advantages of easy preparation, homogeneity and biocompatibility with biomolecules such as proteins, antibodies and DNA. However, compared to Au NPs, Ag NPs exhibit 10–100 times higher plasmon resonance activity, but there are few reports of using Ag NPs in SERS-FLIA due to their lower biocompatibility and stability. It would be desirable to prepare Ag@Au core–shell bimetallic NPs as the substrate for the SERS immunoprobe. In this case, the Au shell can not only greatly increase the stability of Ag NPs without masking the plasmon resonance activity of Ag NPs, but can also further improve the SERS enhancement because gold–silver core–shell bimetallic NPs have higher SERS activity mainly due to the electronic ligand effect and localized electric field enhancement in core–shell NPs.34
In this study, Ag@Au core–shell bimetallic NPs were synthesized, characterized and used as the substrate in SERS-FLIA. The immunoprobe (e.g. AgMBA@Au–Ab) was prepared by sandwiching the Raman reporter mercaptobenzoic acid (MBA) between the core–shell layers and immobilizing the monoclonal antibody (mAb) against brombuterol on the surface of AgMBA@Au NPs. MBA trapped between the core and shell possesses intense electromagnetic field enhancement, greatly heightening the Raman signal of MBA. Moreover, the embedded MBA can be shielded by the Au shell from leakage, degradation and uncontrolled aggregation-induced enhancement. After competitive LFIA procedures, a color change on the test line (T line) effectively indicates the presence of target analytes for naked-eye detection. Additionally, quantitative detection of brombuterol was achieved by measuring the specific Raman scattering intensity of MBA on the T line (Fig. 1). Moreover, the embedded Raman reporter molecules were shielded by the metal shell from leakage, degradation and uncontrolled aggregation-induced enhancement.
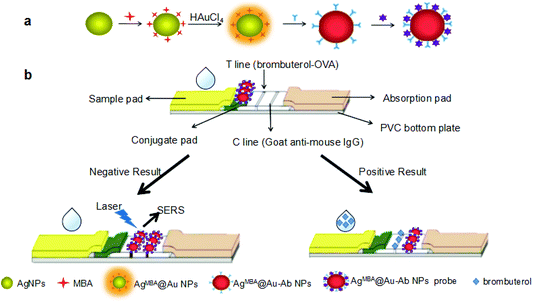 |
| Fig. 1 (a) Schematic illustration of the preparation of immunoprobe AgMBA@Au–Ab; (b) assembly of LFIA strip and the principle of competitive SERS-LFIA for brombuterol. | |
Materials and methods
Materials, chemicals and apparatus
Polyvinylpyrrolidone (PVP, 98%) was purchased from Sangon Biotech (Shanghai, China). L-Ascorbic acid was purchased from Sigma-Aldrich (Shanghai, China). Silver nitrate (AgNO3), sodium borohydride (NaBH4, 96%), sodium hydroxide, sodium sulfite (Na2SO3) and ethanol absolute (C2H5OH) were obtained from the Sinopharm Chemical Reagent Co., Ltd (Shanghai, China). Chloroauric acid (HAuCl4), bovine serum albumin (BSA), ovalbumin (OVA), sodium citrate tribasic dihydrate (Na3C6H5O7·2H2O, 99.5%), and 4-mercaptobenzoic acid (4-MBA) were purchased from Sigma (St Louis, MO, USA). All other chemicals were analytical grade. The mAb against brombuterol and coating antigen (brombuterol-OVA conjugate) were prepared by our group. The processes of antibody production and the development of ELISA for brombuterol with high sensitivity (0.56 ng mL−1) were described in detail in our published paper.14
Nitrocellulose (NC) membranes were purchased from Whatman (Shanghai, China). PVC sheets, adhesive tape and filter paper were purchased from Jieyi Biotechnology Co. Ltd (Shanghai, China). The deionized-RO water supply system (Dura 12FV) was purchased from THE LAB Com. (Dover, DE, USA). The UV-2300 spectrophotometer was bought from Techcom (Shanghai, China). The digital photographs of the samples were taken with an iPhone 8 (Apple, USA). Transmission electron microscopy (TEM) photographs were taken on a Tecnai G220 from USA FEI Company. The portable Raman Analyser RamTracer-200-HS was obtained from Opto Trace Technologies, Inc. (Suzhou, China).
Preparation of the immunoprobe (AgMBA@Au–Ab)
All glassware used in the following procedures was washed with freshly prepared aqua regia (HNO3/HCl, 1
:
3, v/v) followed by rinsing thoroughly with tap water and ultrapure water at least five times.
The preparation of the AgMBA@Au–Ab is illustrated in Fig. 1(a). Ag NPs were first prepared as the core, then the Raman reporter (MBA) was adsorbed onto the surface of the Ag NPs by a thiol group. AgMBA@Au NPs were obtained by reducing HAuCl4 around Ag NPs in the presence of ascorbic acid. The Ab against brombuterol was then immobilized on the surface of the AgMBA@Au NPs to form the final immunoprobe.
The spheroidal Ag NPs were prepared according to the literature with a slight modification.35 AgNO3 (17 mg) was dissolved in 100 mL of ultrapure water and the solution was heated to boiling under vigorous stirring, then 2 mL of 1% (wt) Na3C6H5O7·2H2O was rapidly injected into the solution. After boiling for 40 minutes to ensure complete reduction, the solution was naturally cooled to room temperature. The color of the final solution containing Ag NPs was greenish yellow. Then, 200 μL of MBA (0.1 mmol L−1) was added to the final solution under vigorous stirring for 5 hours to form the AgMBA NPs, which were stored at 4 °C until use.
To prepare Au growth solution, 222 μL of 2% (wt) HAuCl4, 240 μL of NaOH (0.2 mol L−1) and 3.00 mL of Na2SO3 (0.01 mol L−1) were added to a 20 mL glass bottle with 4.538 mL of water. The solution was left undisturbed overnight before use.
The spheroidal AgMBA@Au NPs were prepared by seed growth method with slight modification.36 2–18 mL of AgMBA NPs solution was centrifuged. The precipitate was redispersed in 2 mL of H2O and added to a 25 mL glass vial containing a mixture of 2.55 mL of H2O, 1 mL of PVP (5 wt%, Mw 40
000), 200 μL of ascorbic acid (0.5 mol L−1), 200 μL of NaOH (0.5 mol L−1), 50 μL of Na2SO3 (0.1 mol L−1) and 4 mL of Au growth solution. The reaction was kept at room temperature overnight to produce AgMBA@Au NPs, which were collected by centrifugation and washed with H2O at least three times. The AgMBA@Au NPs with the highest SERS intensity were used to bind with the antibody.
The pH value of the selected AgMBA@Au NPs solution was adjusted to 8.0 with 0.1 mol L−1 K2CO3. 5 mL of the AgMBA@Au NPs solution was mixed with 10 μL of mAb against brombuterol (8.345 mg mL−1) at 4 °C for 4 h. Finally, 200 μL of 5% BSA was added to the mixture and gently stirred for 3 h to block the unspecific binding sites on the surfaces of the particles. The resulting solution was centrifuged at 5000 rpm for 5 minutes at 4 °C and the sediment containing AgMBA@Au–Ab immunoprobes was diluted with pure water (1
:
1) and stored at 4 °C until use. The color of the final immunoprobe solution was a dark brown-red.
Preparation of LFIA strips
As shown in Fig. 1(b), the LFIA strip consists of five parts: a PVC bottom plate with a length of 6 cm and width of 4 mm, a sample pad, a conjugate pad on which 2.5 μL of AgMBA@Au–Ab was added, a NC membrane pasted in the middle of the PVC bottom plate with a T line and a C line (the T line and a C line were formed by evenly distributing 5 μL of brombuterol-OVA (2 mg mL−1) and 5 μL of goat anti-mouse IgG at 1
:
50 dilution, respectively), and an absorbent pad (100% pure cellulose fiber) with high absorption capacity serving as the chromatographic moving force. The sample pad and the absorbent pad were pasted at separate ends of the NC membrane with an overlap of about 1–2 mm. After naturally drying at room temperature for at least 30 min, the LFIA strips were sealed into glass bottles in the presence of desiccant gel and nitrogen gas and stored at 4 °C until use.
Competitive LFIA procedures and SERS signal measurement
Brombuterol standard or sample solution (200 μL) was dropped on the sample pad. Under capillary action, the brombuterol solution migrates toward the end of absorbent pad. The solution first mixes with the immunoprobes on the conjugate pad, then flows through the T line and C line, and finally reaches the absorption pad. The whole process can be completed in 15 minutes.
As illustrated in Fig. 1(b), in a negative situation (the concentration of brombuterol is zero), the AgMBA@Au–Ab on the conjugate pad moves to the T line and specifically binds with brombuterol-OVA; then, the superfluous immunoprobe continuously flows to the C line and is captured by the goat anti-mouse IgG. Two dark-brown-red bands will appear on the T line and C line. In contrast, in a positive situation (the concentration of brombuterol is high), the AgMBA@Au–Ab on the conjugate pad first binds with brombuterol, leaving no free antibody binding sites for brombuterol-OVA, leading to no immunoprobe captured on the T line. But AgMBA@Au–Ab-brombuterol can flow continuously to the C line and be captured by goat anti-mouse IgG. Thus, only one dark brown-red band will appear on the C line. Therefore, the degree of intensity of immunoprobe color on the T line is indirectly proportional to the concentration of brombuterol. Apparently, the presence of brombuterol can be semi-quantitatively evaluated with the naked-eye by the color intensity on the T line. Furthermore, the SERS intensity generated at 1575 cm−1 by MBA in the immunoprobes captured by brombuterol-OVA along the T line can be measured for quantitative determination of brombuterol.
A portable Raman analyser coupled with a microscope (Eplan, 40 × 0.6) was used for the measurement of SERS intensity. The excitation source was tuned to 785 nm with a laser power of 50 mW. The typical integration time was 10 s, with an average of 2 s and a boxcar of 1 s.
Detection of brombuterol in spiked samples
In order to test the accuracy and precision of the assay, three samples (swine liver, pork and feed) collected from a local market were used for the spiking experiment. The samples were cut or ground and then intensively homogenized. 2.0 g of homogenized samples were put into four separate polypropylene centrifuge tubes and then 0, 6, 60, and 600 μL of brombuterol standard solution (1 ng mL−1) were added to separate tubes. To each tube, 6 mL of methanol/water (1
:
1, v/v) was added. The tubes were shaken vigorously for 20 min and centrifuged at 7000 rpm for 10 min at 4 °C. The upper liquid was discarded. Then, 4 mL of HCl (0.1 mol L−1) was added to each tube to dissolve the precipitate and the tubes were kept at 4 °C overnight. On the next day, 800 μL of K2HPO4 (0.1 mol L−1) was added to each tube and the pH was adjusted to 7.0 with K2CO3 (0.1 mol L−1). The tubes were centrifuged at 7000 rpm for 10 min and the supernatant was loaded onto C18 SPE cartridges preconditioned with 3 mL ethyl acetate, 3 mL methanol and 5 mL water. The cartridges were subsequently rinsed with 3 mL of ultrapure water, dried under nitrogen and then eluted with 600 μL of ethyl acetate. The residual eluent was evaporated to dryness in a 50 °C water bath under nitrogen and the product was redissolved in 600 μL PBST. Theoretically, the final concentrations of brombuterol in the extracted solutions were 0, 0.01, 0.1 and 1 ng mL−1. Then, 200 μL of each extracted sample was applied in SERS-FLIA procedures.
Results and discussion
Preparation and characterization of Ag NPs and AgMBA@Au NPs
The SERS intensity of AgMBA@Au NPs is critical to the performance of the SERS-LFIA. Embedding MBA inside the Ag@Au as built-in hot spots allows high SERS enhancement and stability. In the proposed study, MBA embedded AgMBA@Au NPs were synthesized through a seed growth method. Ag NPs were prepared by the reduction of AgNO3 with sodium citrate acid under boiling conditions. Then, Raman reporter MBA molecules were adsorbed onto the Ag NP surface. To deposit the Au shell over the Ag core, ascorbic acid reacted with NaOH to form sodium ascorbate, the reducing reagent to reduce Au3+.
The TEM image and UV-vis spectrum of the Ag NPs are illustrated in Fig. 2(a) and 3(a), respectively. The TEM image reveals that the Ag NPs are homogeneous, with a size of about 39 nm. The UV-vis spectrum of the Ag NPs displays an absorption peak at 412 nm, which is typical of Ag NPs.
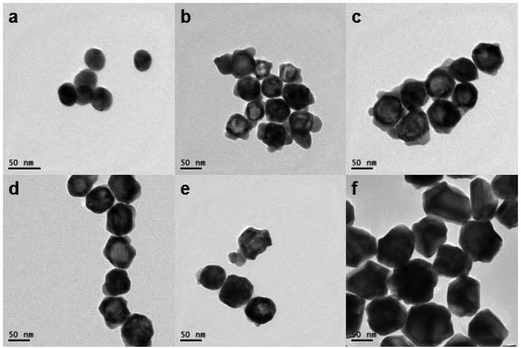 |
| Fig. 2 TEM images of Ag NPs (39 nm) (a) and AgMBA@Au NPs of different sizes (nm): (b) 55, (c) 60, (d) 72, (e) 81 and (f) 99. | |
MBA can be easily attached to Ag NPs via the thiol group in the MBA molecule. When 10.0 mL of Ag NPs was mixed with different amounts of MBA, greater MBA addition to the Ag NPs solution made the Ag NPs aggregate and even precipitate. It was found that 200 μL of MBA (0.1 m mol L−1) was the appropriate amount of MBA required to mix with 10.0 mL of the Ag NPs to form AgMBA NPs.
TEM images of the AgMBA@Au NPs with different Au shell thicknesses are shown in Fig. 2(b–f). During the preparation of AgMBA@Au NPs, it was observed that with increasing volumes of AgMBA NPs solution (2, 6, 10, 14 and 18 mL) added to the same volume (4 mL) of Au growth solution, the size of the prepared AgMBA@Au NPs gradually decreased (99, 81, 72, 60 and 55 nm). The reason is that, with the amount of AgMBA NPs increasing, less of the Au will be deposited on the surface of the AgMBA NPs, leading to a thinner Au shell. As the size of the Ag NPs is about 39 nm, the thicknesses of the Au shells can be estimated to be 30, 21, 16.5, 10.5 and 8 nm, respectively.
The UV-vis spectra of AgMBA@Au NPs with different Au shell thicknesses are displayed in Fig. 3(a). It can be seen that with the thickness of the Au shell increasing from 8 nm to 30 nm, the absorption peaks of AgMBA@Au NPs exhibit a red-shift from 510 nm to 542 nm, which fits well with the traditional Mie scattering theory and the support of dielectric data.37,38 In addition, the UV–vis absorption spectra are symmetric, which indicate that the AgMBA@Au NPs have good monodispersity and uniformity.
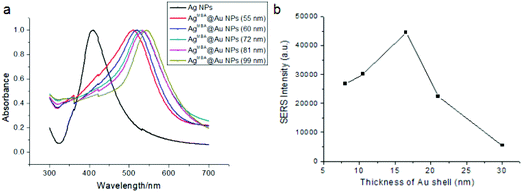 |
| Fig. 3 UV-vis spectra of Ag NPs and AgMBA@Au NPs of different sizes (a); the effect of Au shell thickness of AgMBA@Au NPs on SERS intensity (b). | |
The Raman intensities of AgMBA@Au NPs with different Au shell thicknesses at 1575 cm−1 are illustrated in Fig. 3(b). Because MBA's Raman signal comes from the gap of the electromagnetic field between Ag and Au, as the Au shell thickness increased, the Raman intensities gradually increased and reached a maximum at 16.5 nm. However, further increasing the Au shell thickness results in rapid signal decline. The thicker shell may hinder the output of Raman signal, influence its electromagnetic field coupling with the Ag core, and decrease the effect of electromagnetic enhancement. Therefore, the AgMBA@Au NP with 16.5 nm shell thickness was chosen as the substrate for the SERS-LFIA.
Testing of specific binding of immunoprobe
Before the detection of brombuterol, the specific binding of the immunoprobe to the coating antigen on the LFIA strip should be tested. Four LFIA procedures were performed with a zero concentration of analyte under the following conditions: (a) AgMBA@Au–Ab as probe, brombuterol-OVA coated on the T line; (b) AgMBA@Au-BSA as probe, brombuterol-OVA coated on the T line; (c) AgMBA@Au–Ab as probe, OVA spread on the T line; (d) AgMBA@Au–Ab as probe, Na2CO3-NaHCO3 buffer solution dispersed on the T line. The SERS spectra in the above four cases are shown in Fig. 4. From SERS spectrum (a) in Fig. 4, it can be seen that the specific Raman scattering peaks of MBA appeared at 1074 cm−1 (with a signal value of 6450 a.u.) and 1575 cm−1 (with a signal value of 11
500 a.u.), clearly demonstrating that the immunoprobe was specifically bound with brombuterol-OVA at the T line on the NC membrane. In contrast, in the SERS spectra (b–d) in Fig. 4, almost no SERS signals appear at 1074 cm−1 or 1575 cm−1, indicating that there is no nonspecific adsorption.
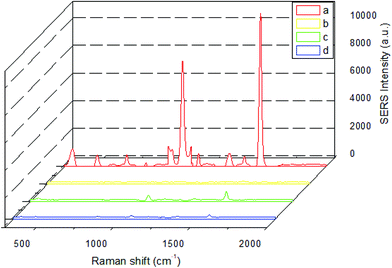 |
| Fig. 4 SERS spectra of SERS-LFIA at T line with 0 ng mL−1 of analyte in different situations: (a) AgMBA@Au–Ab NPs as probe, brombuterol-OVA coated on T line; (b) AgMBA@Au-BSA NPs as probe, brombuterol-OVA coated on T line; (c) AgMBA@Au–Ab NPs as probe, OVA spread on T line; (d) AgMBA@Au–Ab NPs as probe, Na2CO3-NaHCO3 buffer solution dispersed on T line. | |
Optimization of experimental conditions
The sensitivity of the SERS-LFIA can be improved by optimizing experimental conditions, such as the amount of Ab used for the preparation of immunoprobe, the amount of coating antigen and immunoprobe applied on the strip, etc. In this study, to evaluate the optimization we define a parameter called the inhibition ratio (B0/B0.1) in which B0 and B0.1 refer to the SERS intensities of MBA at 1575 cm−1 when brombuterol concentrations are at 0 ng mL−1 and 0.1 ng mL−1, respectively. Higher values of B0/B0.1 indicate higher sensitivity of the SERS-LFIA.
Different amounts of Ab against brombuterol were consecutively coupled to 1.0 mL of AgMBA@Au NPs and the effect of the amount of Ab on B0/B0.1 was investigated [Fig. 5(a)]. It is observed that when different volumes (1–3.5 μL) of Ab at a concentration of 8.345 mg mL−1 are used, the highest value of B0/B0.1 is achieved at 2.0 μL. Thus, the optimal amount of Ab for immunoprobe preparation is 2.0 μL.
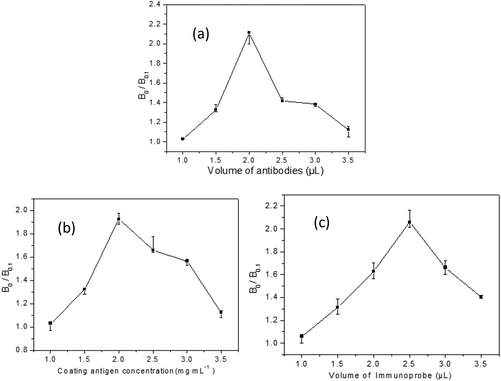 |
| Fig. 5 Optimization of the assay conditions: (a) the effect on B0/B0.1 of antibody volume in the preparation of immunoprobe; (b) the effect on B0/B0.1 of coating antigen; (c) the effect on B0/B0.1 of the amount of immunoprobe. | |
The amounts of the brombuterol-OVA spread on the T line and the immunoprobe dropped on the conjugate pad also influence the sensitivity of the assay. As shown in Fig. 5(b), when 5 μL of brombuterol-OVA at concentrations in the range of 1.0–3.5 mg mL−1 is loaded on the T line, the highest value of B0/B0.1 is obtained at 2.0 mg mL−1. Similarly, as shown in Fig. 5(c), when different volumes (0.5–3.5 μL) of immunoprobe are dropped on the conjugate pad, B0/B0.1 reaches its maximum at 2.5 μL of immunoprobe. Thus, the best conditions for SERS-LFIA are 5 μL brombuterol-OVA at 2.0 mg mL−1 and 2.5 μL of immunoprobes.
Sensitivity of the assay
Under the optimal conditions, brombuterol standard solutions at concentrations of 0, 10−4, 10−3, 10−2, 10−1, 1.0, 10 and 100 ng mL−1 were prepared by diluting brombuterol stock solution (1 mg mL−1) with PBS and applied for SERS-LFIA procedures. After the addition of 200 μL of brombuterol solution to the sample pad, the solution migrates toward the end of absorption pad. As shown in Fig. 6(a), the colors on the T line and C line gradually appear and can be seen directly by the naked eye. The color intensities of the T lines declined as the brombuterol concentration increased, which can allow semi-quantitative detection. The SERS spectra of MBA from the immunoprobes captured on T lines as measured by SERS analyzer are shown in Fig. 6(b). It is clear that the SERS intensity at 1575 cm−1 generated from MBA gradually decreased with the increase of brombuterol concentration, which was used as a basis to construct a standard curve for quantitative detection of brombuterol. The standard curve is plotted in the form of B/B0 × 100% versus log
C, where B and B0 are the SERS intensities of MBA at the standard point and zero concentration, respectively [Fig. 6(c)]. The sensitivity (expressed by the IC50 value, e.g. the concentration of brombuterol producing 50% signal inhibition) is calculated to be 0.045 ng mL−1. As three times the standard deviation (SD), the LOD is estimated to be 0.11 pg mL−1. These results demonstrated the very high sensitivity of the proposed SERS-LFIA for brombuterol.
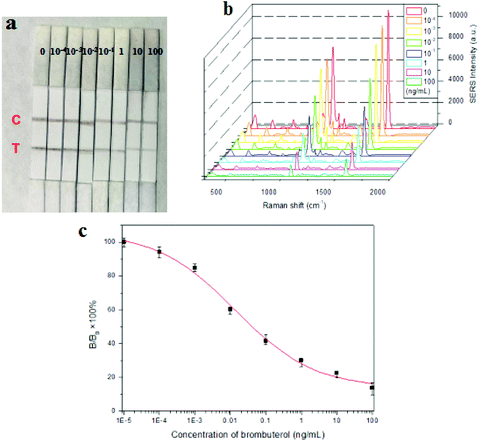 |
| Fig. 6 (a) Digital photograph of T lines on NC membranes after the assay procedure. The numbers above the T lines are the analyte standard concentrations (ng mL−1). (b) The SERS spectra arising from MBA on T lines after assay procedures under optimal experimental conditions. (c) The calibration curve of the SERS-LFIA for brombuterol, where B and B0 were the SERS intensities of MBA at 1575 cm−1 when the brombuterol solutions were at the standard points and zero concentration, respectively. | |
Reproducibility of SERS intensities
The reproducibility of the SERS intensities was examined by comparing the signals at 1575 cm−1 generated from MBA at ten different spots in the center sections of the T lines when the concentrations of brombuterol standard solutions were 0, 0.1 and 1.0 ng mL−1, respectively. As illustrated in Fig. 7, in each strip, the relative standard deviation (RSD) value of the SERS intensities obtained from 10 different points were 1.95%, 3.03% and 7.26%, respectively, suggesting the high precision of the SERS signal.
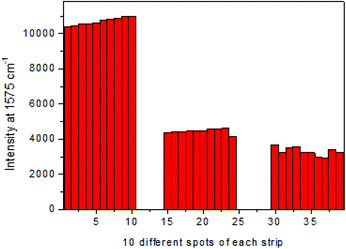 |
| Fig. 7 The SERS intensities of MBA at 1575 cm−1 from 10 different spots along the middle of the T lines on the strips when the concentrations were at 0, 0.1 and 1.0 ng mL−1. The RSD values of the SERS intensities were 1.95%, 3.03% and 7.26%, respectively. | |
Specificity of the assay
Generally the cross-reactivity (CR) values can be utilized to characterize the specificity of the immunoassay. Six β-adrenergic agonists, including clenbuterol, salbutamol, ractopamine, phenylethanolamine A, isoproterenol and phenylephrine, were selected to evaluate the specificity of the SERS-LFIA for brombuterol. All compounds including brombuterol were prepared in concentration ranges of 10−2–104 ng mL−1 and applied in SERS-LFIA procedures. The CR values are calculated according to the equation CR (%) = [IC50 of brombuterol]/[IC50 of tested compound] × 100%. The obtained CR values are displayed in Table 1. It can be seen that the CR values of the assay with salbutamol, ractopamine, phenylethanolamine A, isoproterenol and phenylephrine are less than 0.5%, which is negligible. However, the assay exhibits 34.88% cross-reactivity with clenbuterol because the molecular structure of clenbuterol is highly similar to that of brombuterol. As clenbuterol is a main β-adrenergic agonist illegally used as an additive in animal feed for growth promotion, the proposed LFIA might be used as a screening method for the detection of both brombuterol and clenbuterol.
Detection of brombuterol in spiked samples
In order to verify the practicality of the SERS-LFIA, three samples (swine liver, pork, feed) were spiked with different amounts of brombuterol. After pretreatment of the spiked samples, the extraction solutions were subjected to SERS-LFIA procedures. The contents of brombuterol in spiked samples were calculated according to the standard curve obtained on the same day. The results of the spiking experiment are summarized in Table 2. The recoveries of brombuterol from the spiked samples are 87.27–100.16% with relative standard deviation (RSD) values in the range of 1.26%–6.99% (n = 3), revealing that this novel strategy is able to effectively detect brombuterol in food and feed samples.
Table 2 The recoveries of brombuterol from spiked samples measured by SERS-LFIA
Samples |
Conc. spiked (ng mL−1) |
Conc. measured (ng mL−1) (mean ± SD, n = 3) |
RSD (%) |
Recovery (%) |
Swine liver |
0.01 |
(0.957±0.02)×10−2 |
2.09 |
95.67 |
0.1 |
(9.67±0.14)×10−2 |
1.45 |
96.76 |
1 |
0.985±0.023 |
2.34 |
98.54 |
Swine pork |
0.01 |
(0.93±0.012)×10−2 |
1.29 |
92.82 |
0.1 |
(9.47±0.034)×10−2 |
3.59 |
94.65 |
1 |
0.93±0.065 |
6.99 |
92.9 |
Swine feed |
0.01 |
(8.73±0.45)×10−3 |
5.15 |
87.27 |
0.1 |
(0.95±0.018)×10−2 |
1.89 |
95.1 |
1 |
1.002±0.057 |
5.69 |
100.16 |
Conclusions
A quantitatively ultrasensitive LFIA based on SERS for the detection of brombuterol in swine liver, pork and feed samples was developed. Ag@Au core–shell bimetallic nanoparticles with the highest SERS enhancement, good biocompatibility and high stability were synthesized, characterized and applied as the substrate for the preparation of the immunoprobe. The assay was completed within 15 min. The color changes observable by naked eye on the T lines were used for semi-quantitative detection. Furthermore, the specific Raman scattering intensities of MBA in the immunoprobes captured on the T lines were used for quantification. Under optimal conditions, the IC50 and LOD values of this approach for brombuterol were 45 pg mL−1 and 0.11 pg mL−1, respectively, indicating the ultra-sensitivity of the assay. The recoveries of brombuterol from spiked food samples detected by SERS-LFIA were 87.27%–100.16% with RSD values in the range of 1.29%–6.99% (n = 3). The proposed assay was proven to be a rapid, simple and ultrasensitive analytical method for brombuterol detection and might be an alternative analytical method for application in point-of-care clinical testing, environmental monitoring and food analysis.
Conflicts of interest
There is no conflict to declare.
Acknowledgements
The authors thank the National Natural Science Foundation of China (NSFC, contact no. 21175097 and 31772053) and a Project Funded by the Priority Academic Program Development of Jiangsu Higher Education Institutions (No. YX10900212) for financial support of this study.
References
- E. M. Snyder and B. D. Johnson, Am. J. Respir. Crit. Care Med., 2014, 189, 1445–1447 CrossRef
.
- Y. L. Xiong, M. J. Gower, C. Li, C. A. Elmore, G. L. Cromwell and M. D. Lindemann, Meat Sci., 2006, 73, 600–604 CrossRef CAS
.
- H. A. Kuiper, M. Y. Noordam, M. M. H. Dooren-Filpsen, R. Schilt and A. H. Roos, J. Anim. Sci., 1998, 76, 195–207 CrossRef CAS
.
- J. Barbosa, C. Cruz, J. Martins, J. M. Silva, C. Neves, C. Alves, F. Ramos and M. I. N. D. Silveira, Food Addit. Contam., 2005, 22, 563–566 CrossRef CAS
.
- Z. H. Gu and L. J. Zheng, Chin. J. Food Hygiene, 2007, 19, 10–12 Search PubMed
.
- J. Serratosa, A. Blass, B. Rigau, B. Mongrell, T. Rigau, M. Tortadès, E. Tolosa, C. Aguilar, O. Ribó and J. Balagué, Rev. Sci. Tech. Off. Int. Epiz., 2006, 25, 637–653 CrossRef CAS
.
- Bulletin of the Ministry of Agriculture of the People's Republic of China, no. 1519, 2010.
- J. Cheng, S. Wang and X. O. Su, PLoS One, 2013, 8, e76400 CrossRef CAS
.
- C. Juan, C. Igualada, F. Moragues, N. León and J. Mañes, J. Chromatogr., A, 2010, 1217, 6061–6068 CrossRef CAS
.
- A. González-Antuña, J. C. Domínguez-Romero, J. F. García-Reyes, P. Rodríguez-González, G. Centineo, J. I. García Alonso and A. Molina-Díaz, J. Chromatogr., A, 2013, 1288, 40–47 CrossRef
.
- Z. H. Zhang, H. Yan, F. Y. Cui, H. Yun, X. H. Chang, J. H. Li, X. Liu, L. J. Yang and Q. R. Hu, Food Anal. Methods, 2016, 9, 915–924 CrossRef
.
- T. T. Dong, L. Y. Hu, K. Zhao, A. P. Deng and J. G. Li, Anal. Chim. Acta, 2016, 945, 85–94 CrossRef CAS
.
- Q. Zhu, F. D. Cai, J. Zhang, K. Zhao, A. P. Deng and J. G. Li, Biosens. Bioelectron., 2016, 86, 899–906 CrossRef CAS
.
- Y. Y. Zhao, D. N. Jiang, K. Wu, H. Yang, H. J. Du, K. Zhao, J. G. Li and A. P. Deng, Anal. Methods, 2016, 8, 6941–6948 RSC
.
- B. Ngom, Y. Guo, X. Wang and D. Bi, Anal. Bioanal. Chem., 2010, 397, 1113–1135 CrossRef CAS
.
- X. J. Xiong, W. B. Rao, X. F. Guo, H. Wang and H. S. Zhang, J. Agric. Food Chem., 2012, 60, 5107–5111 CrossRef CAS
.
- S. C. Razo, V. G. Panferov, I. V. Safenkova, Y. A. Varitsev, A. V. Zherdev and B. B. Dzantiev, Anal. Chim. Acta, 2018, 1007, 50–60 CrossRef CAS
.
- Z. Rong, Q. Wang, N. Sun, X. Jia, K. Wang, R. Xiao and S. Wang, Anal. Chim. Acta, 2019, 1055, 140–147 CrossRef CAS
.
- J. Yan, Y. Liu, Y. Wang, X. Xu, Y. Lu, Y. Pan, F. Guo and D. Shi, Sens. Actuators, B, 2014, 197, 129–136 CrossRef CAS
.
- G. Posthuma-Trumpie, J. Wichers, M. Koets, L. J. M. Berendsen and A. van Amerongen, Anal. Bioanal. Chem., 2012, 402, 593–600 CrossRef CAS
.
- Q. Y. Xie, Y. H. Wu, Q. R. Xiong, H. Y. Xu, Y. H. Xiong, K. Liu, Y. Jin and W. H. Lai, Biosens. Bioelectron., 2014, 54, 262–265 CrossRef CAS
.
- N. A. Taranova, A. N. Berlina, A. V. Zherdev and B. B. Dzantiev, Biosens. Bioelectron., 2015, 63, 255–261 CrossRef CAS
.
- K. Bobosha, E. M. Tjon Kon Fat, S. J. F. van den Eeden, Y. Bekele, J. J. van der Ploeg-van Schip, C. J. de Dood, K. Dijkman, K. L. M. C. Franken, L. Wilson, A. Aseffa, J. S. Spencer, T. H. M. Ottenhoff, P. L. A. M. Corstjens and A. Geluk, PLoS Neglected Trop. Dis., 2014, 8, e2845 CrossRef
.
- E. Juntunen, T. Myyryläinen, T. Salminen, T. Soukka and K. Pettersson, Anal. Biochem., 2012, 428, 31–38 CrossRef CAS
.
- H. A. Joung, Y. K. Oh and M. G. Kim, Biosens. Bioelectron., 2014, 53, 330 CrossRef CAS
.
- M. X. Li, H. Yang, S. Q. Li, K. Zhao, J. G. Li, D. N. Jiang, L. L. Sun and A. P. Deng, J. Agric. Food Chem., 2014, 62, 10896–10902 CrossRef CAS
.
- V. Tran, B. Walkenfort, M. Kçnig, M. Salehi and S. Schlcker, Angew. Chem., Int. Ed., 2019, 58, 442–446 CrossRef CAS
.
- X. L. Fu, Z. Y. Cheng, J. M. Yu, P. Choo, L. X. Chen and J. Choo, Biosens. Bioelectron., 2016, 78, 530–537 CrossRef CAS
.
- M. Eryılmaz, E. A. Soykut, D. Çetin, İ. H. Boyacı, Z. Suludere and U. Tamer, Analyst, 2019, 144, 3573–3580 RSC
.
- X. L. Fu, J. H. Wen, J. W. Li, H. Lin, Y. M. Liu, X. M. Zhuang, C. Y. Tian and L. X. Chen, Nanoscale, 2019, 11, 15530–15536 RSC
.
- C. W. Wang, C. G. Wang, X. L. Wang, K. L. Wang, Y. H. Zhu, Z. Rong, W. Y. Wang, R. Xiao and S. Q. Wang, ACS Appl. Mater. Interfaces, 2019, 11, 19495–19505 CrossRef CAS
.
- H. B. Liu, X. J. Du, Y. X. Zang, P. Li and S. Wang, J. Agric. Food Chem., 2017, 65, 10290–10299 CrossRef CAS
.
- Q. Q. Shi, J. Huang, Y. N. Sun, R. G. Deng, M. Teng, Q. M. Li, Y. Y. Yang, X. F. Hu, Z. J. Zhang and G. P. Zhang, Microchim. Acta, 2018, 185, 84 CrossRef
.
- Y. Huang, Y. Yang, Z. Chen, X. Li and M. Nogami, J. Mater. Sci., 2008, 43, 5390–5393 CrossRef CAS
.
- P. C. Lee and D. Meisel, J. Phys. Chem., 1982, 86, 3391–3395 CrossRef CAS
.
- H. Liu, T. Liu, L. Zhang, L. Han, C. Gao and Y. Yin, Adv. Funct. Mater., 2015, 25, 5435–5443 CrossRef CAS
.
- M. Moskovits, I. Srnová-Šloufová and B. Vlčková, J. Chem. Phys., 2002, 116, 10435–10446 CrossRef CAS
.
- S. Lee, S. Kim, J. Choo, S. Y. Shin, Y. H. Lee, H. Y. Choi, S. Ha, K. Kang and C. H. Oh, Anal. Chem., 2007, 79, 916–922 CrossRef CAS
.
|
This journal is © The Royal Society of Chemistry 2021 |
Click here to see how this site uses Cookies. View our privacy policy here.