DOI:
10.1039/D0SC03116J
(Edge Article)
Chem. Sci., 2020,
11, 7239-7243
N-Heterocyclic carbene based catalytic platform for Hauser–Kraus annulations†
Received
3rd June 2020
, Accepted 18th June 2020
First published on 30th June 2020
Abstract
The venerable Hauser–Kraus annulation is an effective and convergent method for generating oxygenated polycyclic aromatic compounds. Despite its application in complex molecule synthesis, the harsh and strongly basic conditions can limit its utility in more functionalized molecular settings. We have developed the first catalytic Hauser–Kraus annulation based on N-heterocyclic carbene catalysis that proceeds under milder conditions. We demonstrate the scope of the transformation in the presence of several functional groups. We also propose a concerted mechanism for the annulation that proceeds through a non-canonical Breslow intermediate.
Introduction
The Hauser–Kraus annulation is arguably one of the most effective strategies for synthesizing oxygenated polycyclic aromatic compounds (1, Scheme 1),1,2 which are ubiquitous in pharmaceutical agents and biologically active natural products.3 The power of this reaction manifold is the ability to generate a broad range of polyaromatic products in a convergent manner, which is best depicted by the union of synthons 4 and 5. Despite the widespread utility of Hauser–Kraus annulations, some drawbacks persist. First, the reaction requires stoichiometric strong bases such as LDA, LTB, or LHMDS (pKaH > 30 in DMSO),4 which limits the functional group tolerance of the reaction. Secondly, the substituted phthalide substrates 2 are accessed from simple precursors such as lactol 6 through multi-step syntheses, which often require toxic chemicals such as potassium and sodium cyanide.5
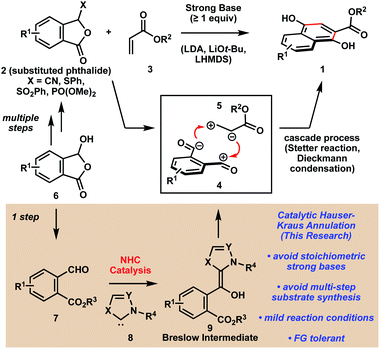 |
| Scheme 1 Design of a catalytic platform for Hauser–Kraus annulations. | |
We were interested in developing a catalytic platform for the synthesis of oxygenated polycyclic aromatic compounds that would maintain all of the advantages of traditional Hauser–Kraus annulations while also addressing the major drawbacks. Although this classical reaction has been known for more than 40 years, no catalytic versions have been reported in the literature. We envisioned that synthon 4 could be accessed catalytically by treating phthalaldehydates such as 7, which can be synthesized from lactols 6 in one step, with N-heterocyclic carbene (NHC) catalyst 8.6 A cascade of reactions would then furnish the desired polyaromatic product without the use of stoichiometric strong bases. In this Communication, we describe the development of the first catalytic Hauser–Kraus annulation system and we explore the generality of this method. We also provide mechanistic data to support a concerted mechanism for the transformation that proceeds through a non-canonical Breslow intermediate that is unprecedented for NHC-catalyzed annulations.
Results and discussion
Initial attempts to realize our proposal for a catalytic Hauser–Kraus annulation commenced with the examination of ethyl phthalaldehydate 10a and ethyl acrylate 11 (Table 1). In the presence of NHC precursors A or B and DBU as base (pKaH = 12 in DMSO),4 we did not observe any annulation product 12 (Table 1, entries 1–2). Gratifyingly, the NHC catalyst derived from imidazolium salt C, which is at least 103 times more nucleophilic than the carbene derived from thiazolium salt B,7 afforded the desired product in 40% yield (entry 3). An examination of other reaction solvents revealed that polar aprotic solvents such as DMF and DMSO were the best media for the reaction, with DMSO proving to be a slightly better solvent (entries 3–6). We measured the conversion of the reaction in the presence of DBU to be 95% (entry 6), suggesting that an alternate choice of base may lead to less decomposition and more product formation. Cs2CO3 (pKaH = 10 in DMSO)4 did not yield the desired product because of its diminished basicity (entry 7). In addition, product was not observed in the presence of the more basic NEt3 (pKaH = 9 in DMSO),4 presumably because of the nucleophilicity of the amine (entry 8). Ultimately, sterically hindered DIPEA (pKaH = 8.5 in DMSO)4 facilitated the formation of Hauser–Kraus annulation product 12 in 45% yield and only 60% conversion, suggesting considerably less decomposition (entry 9). The use of benzyl phthalaldehydate 10b increased the yield of product formation to 60% (entry 10). Finally, the addition of lithium salts improved the efficiency of the reaction (entries 11–13), with LiOAc proving to be the most effective additive.8 Under the optimal reaction conditions, including only 0.5 equivalents of DIPEA, the desired Hauser–Kraus annulation product 12 was obtained in 72% isolated yield (entry 13).
Table 1 Development of catalytic Hauser–Kraus annulationa
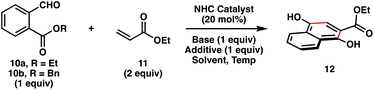
|
Entry |
NHC |
R |
Base |
Solvent |
Additive |
Temp. (°C) |
Time (h) |
Yieldb (%) |
Reaction conditions: 10 (0.25 mmol, 1 equiv.), 11 (0.5 mmol, 2 equiv.), NHC catalyst (0.05 mmol, 20 mol%), base (0.25 mmol, 1 equiv.), additive (0.25 mmol, 1 equiv.), solvent (2.5 ml, 0.1 M).
NMR yield (trimethoxybenzene as internal standard).
0.5 equiv. DIPEA.
Isolated yield in parentheses.
|
1 |
A |
Et |
DBU |
DMF |
— |
60 |
24 |
<5 |
2 |
B |
Et |
DBU |
DMF |
— |
60 |
24 |
<5 |
3 |
C |
Et |
DBU |
DMF |
— |
60 |
24 |
40 |
4 |
C |
Et |
DBU |
THF |
— |
60 |
24 |
<5 |
5 |
C |
Et |
DBU |
PhMe |
— |
60 |
24 |
<5 |
6 |
C |
Et |
DBU |
DMSO |
— |
60 |
24 |
45 |
7 |
C |
Et |
Cs2CO3 |
DMSO |
— |
60 |
24 |
<5 |
8 |
C |
Et |
NEt3 |
DMSO |
— |
60 |
24 |
<5 |
9 |
C |
Et |
DIPEA |
DMSO |
— |
80 |
100 |
45 |
10 |
C |
Bn |
DIPEA |
DMSO |
— |
80 |
90 |
60 |
11 |
C |
Bn |
DIPEA |
DMSO |
LiBr |
80 |
50 |
60 |
12 |
C |
Bn |
DIPEA |
DMSO |
LiCl |
80 |
50 |
75 |
13 |
C |
Bn |
DIPEA |
DMSO |
LiOAc |
80 |
12 |
80 (72)c,d |
|
With optimal conditions in hand, we explored the generality of the catalytic Hauser–Kraus annulation towards different benzyl phthalaldehydate substrates (Table 2A). Methyl substitution at various positions in the backbone of the phthalaldehydate were tolerated (15a–15c). Various halogens were also compatible with the reaction, including fluorine, chlorine, bromine, and iodine (15d–15g). The presence of halogens may be problematic with traditional Hauser–Kraus annulation protocols with strong lithium amides that could undergo lithium–halogen exchange. Products with an electron-donating methoxy substituent (15h) and electron-withdrawing benzyloxy substituent (15i) were also generated. Because of the mildness of the catalytic reaction conditions, the presence of an acidic proton of an aniline-based carbamate did not prevent the formation of the desired product 15j. Finally, we extended the catalytic chemistry to the synthesis of polycyclic heteroaromatic systems, such as isoquinoline 15k.
Table 2 Generality of catalytic Hauser–Kraus annulationa
Reaction conditions: 13 (0.25 mmol, 1 equiv.), 14 (0.5 mmol, 2 equiv.), NHC catalyst (0.05 mmol, 20 mol%), DIPEA (0.125 mmol, 0.5 equiv.), LiOAc (0.25 mmol, 1 equiv.), solvent (2.5 ml, 0.1 M).
|
|
Next, we examined the scope of electrophilic coupling partners (Table 2B). Several acrylates were employed in the reaction without affecting product yield (12, 15l–15o). Acrylonitrile was also a competent substrate for the reaction (15p). α,β-Unsaturated ketones furnished Hauser–Kraus annulation products (15q–15s) in slightly diminished yields. Although crotyl and cinnamyl esters did not yield the desired products (15t, 15u), internal alkenes activated by two electron-withdrawing groups proved to be effective substrates for the reaction (15v–15x). We were also pleased to observe the formation of benzoquinone products 15y and 15z with quaternary carbons when 1,1-disubstituted electron-deficient alkenes were used as substrates.
We performed a series of experiments to distinguish between a stepwise or concerted mechanism for the catalytic Hauser–Kraus annulation (Scheme 2). As discussed previously (Table 1, entry 13), ortho-aldehyde ester 10b yielded annulation product 12 (eqn (1)). Surprisingly, electronically similar para-aldehyde ester 16 did not yield Stetter reaction product 17 (eqn (2)), which was expected to form if these reaction conditions facilitated the formation of a traditional Breslow intermediate (9, Scheme 1). In addition, non-aromatic aldehyde ester 18 did not form the product that would have resulted from a Stetter reaction/Dieckmann condensation sequence (eqn (3)). These observations suggest that the traditional Breslow intermediate may not be operative under the catalytic Hauser–Kraus reaction conditions. Moreover, the results are more consistent with a concerted [4 + 2] cycloaddition mechanism than a stepwise Stetter reaction/Dieckmann condensation mechanism (vide infra).
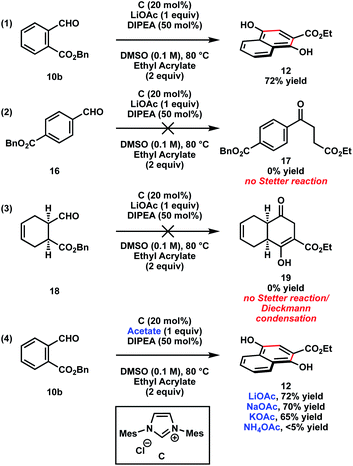 |
| Scheme 2 Mechanistic studies. | |
Although multiple lithium salts with different counter anions improved the efficiency of the reaction, LiOAc proved to be the optimal additive (Table 1, entry 13). We were therefore interested in probing the role of various counter cations of acetate salts (eqn (4)). Whereas lithium, sodium, and potassium acetate salts yielded the desired product 12, non-Lewis acidic ammonium acetate did not furnish the product, suggesting the importance of a Lewis acidic counter cation in activating the substrates (vide infra).
Based on these observations, we propose the mechanism depicted in Scheme 3. Deprotonation of imidazolium salt C with DIPEA results in the formation of the catalytically active NHC 20. Coupling of the catalyst with benzyl phthalaldehydate 10b and concomitant liberation of benzyl alcohol, which can be detected by 1H NMR,9 results in the formation of non-canonical Breslow intermediate 21. To the best of our knowledge, the formation of this type of O-acylated intermediate from the coupling of an aldehyde and an NHC catalyst is unprecedented,10,11 and its reactivity may be distinct from that of a traditional Breslow intermediate. Catalytically generated dienes such as 21 are not accessible from aldehyde esters 16 and 18, which accounts for the lack of product formation with these substrates (eqn (2) and (3), Scheme 2). Subsequent concerted [4 + 2] cycloaddition of intermediate 21 with acrylate 11 results in the formation of cycloadduct 23. The quaternary complex depicted in transition structure 22 is consistent with the experimentally determined rate orders,9 and it suggests the cooperative roles of the NHC catalyst 20 and Lewis acidic LiOAc.8,12 Decomposition of cycloadduct 23 reforms the active catalytic NHC 20 and unveils coupled product 24, which spontaneously tautomerizes to the observed Hauser–Kraus annulation product 12. Interestingly, when non-aromatic aldehyde ester 18 was subjected to the optimized reaction conditions in the absence of ethyl acrylate, liberation of benzyl alcohol was detected by 1H NMR, suggesting the formation of an O-acylated Breslow intermediate. The lack of Hauser–Kraus product formation with substrate 18 under optimized conditions (Scheme 2, eqn (3)) and the inability of this substrate to form a diene analogous to non-canonical Breslow intermediate 21 are consistent with a concerted [4 + 2] cycloaddition mechanism in the catalytic Hauser–Kraus annulation.
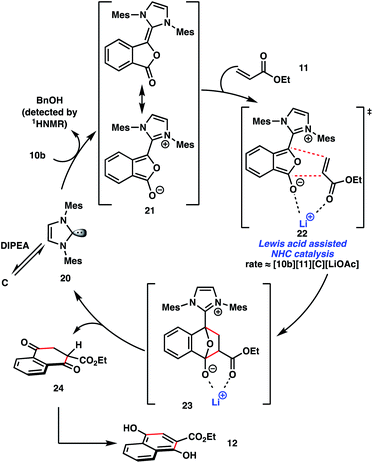 |
| Scheme 3 Proposed mechanism with non-canonical Breslow intermediate. | |
Conclusions
We have developed the first catalytic platform for Hauser–Kraus annulations. Given the mildness of the reaction conditions, we are able to generate a broad range of functionalized products in synthetically useful yields. Mechanistic studies are consistent with a concerted [4 + 2] cycloaddition that proceeds via Lewis acid assisted NHC catalysis. The application of this catalytic platform for the synthesis of complex functionalized molecules and the development of an enantioselective reaction are areas of ongoing interest in our laboratory.
Conflicts of interest
There are no conflicts to declare.
Acknowledgements
Financial support was provided by W. W. Caruth, Jr. Endowed Scholarship, Welch Foundation (I-1748), National Institutes of Health (R01GM102604), American Chemical Society Petroleum Research Fund (59177-ND1), Teva Pharmaceuticals Marc A. Goshko Memorial Grant (60011-TEV), and Sloan Research Fellowship. We also thank our diverse collection of lab members for creating an environment that supported the success of this project.
Notes and references
-
(a) F. M. Hauser and R. P. Rhee, J. Org. Chem., 1978, 43, 178–180 CrossRef CAS;
(b) G. A. Kraus and H. Sugimoto, Tetrahedron Lett., 1978, 19, 2263–2266 CrossRef.
-
(a) A. S. Mitchell and R. A. Russell, Tetrahedron, 1995, 51, 5207–5236 CrossRef CAS;
(b) D. Mal and P. Pahari, Chem. Rev., 2007, 107, 1892–1918 CrossRef CAS PubMed;
(c) K. Rathwell and M. A. Brimble, Synthesis, 2007, 643–662 CAS.
- For recent examples of Hauser–Kraus annulations in complex molecule synthesis, see:
(a) K. C. Nicolaou, H. Zhang, J. S. Chen, J. J. Crawford and L. Pasunoori, Angew. Chem., Int. Ed., 2007, 46, 4704–4707 CrossRef CAS PubMed;
(b) D. Mal and S. R. De, Org. Lett., 2009, 11, 4398–4401 CrossRef CAS PubMed;
(c) J. Li and D. R. Mootoo, Synthesis, 2013, 45, 2287–2293 CrossRef CAS;
(d) M. A. Brimble, N. P. S. Hassan, B. J. Naysmith and J. Sperry, J. Org. Chem., 2014, 79, 7169–7178 CrossRef CAS PubMed;
(e) M. Dischmann, T. Frassetto, M. A. Breuning and U. Koert, Chem.–Eur. J., 2014, 20, 11300–11302 CrossRef CAS PubMed;
(f) C. L. Hugelshofer and T. Magauer, Angew. Chem., Int. Ed., 2014, 53, 11351–11355 CrossRef CAS PubMed;
(g) K. Tatsuta and S. Hosokawa, Chem. Rec., 2014, 14, 28–40 CrossRef CAS PubMed;
(h) N. P. S. Hassan, B. J. Naysmith, J. Sperry and M. A. Brimble, Tetrahedron, 2015, 71, 7137–7143 CrossRef CAS;
(i) T. Kim, K. H. Jeong, K. S. Kang, M. Nakata and J. Ham, Eur. J. Org. Chem., 2017, 1704–1712 CrossRef CAS;
(j) R. Peng and M. S. VanNieuwenhze, J. Org. Chem., 2019, 84, 760–768 CrossRef CAS PubMed.
- F. G. Bordwell, Acc. Chem. Res., 1988, 21, 456–463 CrossRef CAS.
-
Hydrogen cyanide, potassium cyanide and sodium cyanide, The MAK-Collection for Occupational Health and Safety: Occupational Toxicants, 2003, vol. 19, pp. 190–210 Search PubMed.
- For seminal reviews of N-heterocyclic carbene catalysis, see:
(a) A. T. Biju, N. Kuhl and F. Glorius, Acc. Chem. Res., 2011, 44, 1182–1195 CrossRef CAS PubMed;
(b) A. Grossmann and D. Enders, Angew. Chem., Int. Ed., 2012, 51, 314–325 CrossRef CAS PubMed;
(c) S. J. Ryan, L. Candish and D. W. Lupton, Chem. Soc. Rev., 2013, 42, 4906–4917 RSC;
(d) J. Mahatthananchai and J. W. Bode, Acc. Chem. Res., 2014, 47, 696–707 CrossRef CAS PubMed;
(e) D. M. Flanigan, F. Romanov-Michailidis, N. A. White and T. Rovis, Chem. Rev., 2015, 115, 9307–9387 CrossRef CAS PubMed;
(f) S. R. Yetra, A. Patra and A. T. Biju, Synthesis, 2015, 47, 1357–1378 CrossRef CAS;
(g)
A. T. Davies and A. D. Smith, In Recent advances in N-heterocyclic carbene organocatalysis, Georg Thieme Verlag, 2017, pp. 395–442 Search PubMed.
- B. Maji, M. Breugst and H. Mayr, Angew. Chem., Int. Ed., 2011, 50, 6915–6919 CrossRef CAS PubMed.
- For early discussions of dual activation with N-heterocyclic carbenes and Lewis acids, see:
(a) D. T. Cohen and K. A. Scheidt, Chem. Sci., 2012, 3, 53–57 RSC;
(b) M. H. Wang and K. A. Scheidt, Angew. Chem., Int. Ed., 2016, 55, 14912–14922 CrossRef CAS PubMed.
- See ESI† for details.
- For examples of O-acylated Breslow intermediates derived from 1,2-diketones, see:
(a) C. R. Sinu, E. Suresh and V. Nair, Org. Lett., 2013, 15, 6230–6233 CrossRef CAS;
(b) K. Takaki, A. Ohno, M. Hino, T. Shitaoka, K. Komeyama and H. Yoshida, Chem. Commun., 2014, 50, 12285–12288 RSC;
(c) X. W. Kong, G. X. Zhang, S. Yang, X. Z. Liu and X. Q. Fang, Adv. Synth. Catal., 2017, 359, 2729–2734 CrossRef CAS;
(d) K. Takaki, M. Hino, A. Ohno, K. Komeyama, H. Yoshida and H. Fukuoka, Beilstein J. Org. Chem., 2017, 13, 1816–1822 CrossRef CAS;
(e) J. Liu, D. K. Das, G. Zhang, S. Yang, H. Zhang and X. Fang, Org. Lett., 2018, 20, 64–67 CrossRef CAS PubMed;
(f) L. Ping, J. Bak, Y. Kim and J. Bouffard, J. Org. Chem., 2018, 83, 9240–9249 CrossRef CAS PubMed.
- For other examples of dearomatized Breslow intermediates, see:
(a) X. Chen, S. Yang, B.-A. Song and Y. R. Chi, Angew. Chem., Int. Ed., 2013, 52, 11134–11137 CrossRef CAS PubMed;
(b) D. Janssen-Müller, S. Singha, T. Olyschläger, C. G. Daniliuc and F. Glorius, Org. Lett., 2016, 18, 4444–4447 CrossRef PubMed;
(c) J. Xu, S. Yuan and M. Miao, Org. Lett., 2016, 18, 3822–3825 CrossRef CAS;
(d) D.-F. Chen and T. Rovis, Synthesis, 2017, 49, 293–298 CrossRef CAS;
(e) X. Chen, H. Wang, K. Doitomi, C. Y. Ooi, P. Zheng, W. Liu, H. Guo, S. Yang, B.-A. Song, H. Hirao and Y. R. Chi, Nat. Commun., 2017, 8, 15598 CrossRef PubMed;
(f) H. Wang, X. Chen, Y. Li, J. Wang, S. Wu, W. Xue, S. Yang and Y. R. Chi, Org. Lett., 2018, 20, 333–336 CrossRef CAS PubMed;
(g) X. Yang, G. Luo, L. Zhou, B. Liu, X. Zhang, H. Gao, Z. Jin and Y. R. Chi, ACS Catal., 2019, 9, 10971–10976 CrossRef CAS;
(h) K. Balanna, K. Madica, S. Mukherjee, A. Ghosh, T. Poisson, T. Besset, G. Jindal and A. T. Biju, Chem.–Eur. J., 2020, 26, 818–822 CrossRef CAS PubMed;
(i) Y. Liu, G. Luo, X. Yang, S. Jiang, W. Xue, Y. R. Chi and Z. Jin, Angew. Chem., Int. Ed., 2020, 59, 442–448 CrossRef CAS PubMed.
- The dual activation of annulations with NHC catalysts and Lewis acids is an established mode of reactivity:
(a) B. Cardinal-David, D. E. A. Raup and K. A. Scheidt, J. Am. Chem. Soc., 2010, 132, 5345–5347 CrossRef CAS PubMed;
(b) D. E. A. Raup, B. Cardinal-David, D. Holte and K. A. Scheidt, Nat. Chem., 2010, 2, 766–771 CrossRef CAS;
(c) J. Dugal-Tessier, E. A. O'Bryan, T. B. H. Schroeder, D. T. Cohen and K. A. Scheidt, Angew. Chem., Int. Ed., 2012, 51, 4963–4967 CrossRef CAS;
(d) J. Mo, X. Chen and Y. R. Chi, J. Am. Chem. Soc., 2012, 134, 8810–8813 CrossRef CAS PubMed;
(e) A. M. ElSohly, D. A. Wespe, T. J. Poore and S. A. Snyder, Angew. Chem., Int. Ed., 2013, 52, 5789–5794 CrossRef CAS PubMed;
(f) J. Qi, X. Xie, R. Han, D. Ma, J. Yang and X. She, Chem.–Eur. J., 2013, 19, 4146–4150 CrossRef CAS PubMed;
(g) S. R. Yetra, A. Bhunia, A. Patra, M. V. Mane, K. Vanka and A. T. Biju, Adv. Synth. Catal., 2013, 355, 1089–1097 CrossRef CAS;
(h) S. Bera, R. C. Samanta, C. G. Daniliuc and A. Studer, Adv. Synth. Catal., 2014, 53, 9622–9626 CAS;
(i) S. Mukherjee, S. Joseph, A. Bhunia, R. G. Gonnade, S. R. Yetra and A. T. Biju, Org. Biomol. Chem., 2017, 15, 2013–2019 RSC;
(j) K. J. R. Murauski, D. M. Walden, P. H.-Y. Cheong and K. A. Scheidt, Adv. Synth. Catal., 2017, 359, 3713–3719 CrossRef CAS.
Footnote |
† Electronic supplementary information (ESI) available. See DOI: 10.1039/d0sc03116j |
|
This journal is © The Royal Society of Chemistry 2020 |