DOI:
10.1039/D0SC02689A
(Edge Article)
Chem. Sci., 2020,
11, 10517-10522
Base-catalyzed aryl halide isomerization enables the 4-selective substitution of 3-bromopyridines†
Received
11th May 2020
, Accepted 6th September 2020
First published on 9th September 2020
Abstract
The base-catalyzed isomerization of simple aryl halides is presented and utilized to achieve the 4-selective etherification, hydroxylation and amination of 3-bromopyridines. Mechanistic studies support isomerization of 3-bromopyridines to 4-bromopyridines proceeds via pyridyne intermediates and that 4-substitution selectivity is driven by a facile aromatic substitution reaction. Useful features of a tandem aryl halide isomerization/selective interception approach to aromatic functionalization are demonstrated. Example benefits include the use of readily available and stable 3-bromopyridines in place of less available and stable 4-halogenated congeners and the ability to converge mixtures of 3- and 5-bromopyridines to a single 4-substituted product.
Introduction
The synthetic value of aryl halides derives from their thoroughly studied reactivity that allows reliable and predictable access to functionalized aromatic compounds.1 The utility of these widely available substrates can be significantly increased as new reactivity modes are discovered and applied. In this regard, catalytic aryl halide isomerization drew our attention as a relatively underdeveloped yet potentially useful process (Scheme 1).2
 |
| Scheme 1 Concept of catalytic aryl halide isomerization. | |
The rearrangement of halogenated arenes under basic conditions has been extensively studied in the context of “halogen dance” chemistry.3 Early studies by Bunnett on the base-catalyzed isomerization of 1,2,4-tribromobenzene into 1,3,5-tribromobenzene revealed rearrangement occurs via intermolecular halogen transfer, resulting in regioisomeric mixtures and disproportionated side products.4 This catalytic rearrangement requires an acidic arene that can generate electrophilic halogen transfer intermediates (e.g. tetrabromobenzenes); thus, isomerization is observed for tribromobenzenes but not for simple aryl halides.5 This insight guided decades of development of modern “halogen dance” methodology, wherein stoichiometric and irreversible metalation of haloarenes can lead to rearrangement through intermolecular metal–halogen transposition.6 In addition to typically requiring stoichiometric lithium bases under cryogenic conditions, a synthetically useful dance requires a thermodynamic gradient in order to drive a selective rearrangement.3 In this regard, important achievements have been made in identifying specific classes of metalated haloarenes that rearrange as a strategy for electrophilic functionalization.7
We sought to identify more mild and general conditions for aryl halide isomerization as an entry to developing new arene functionalization methods. Inspired by sporadic reports of rearranged aryl halide side products8 in reactions involving aryne9,10 intermediates, we hypothesized that non-nucleophilic bases could enable reversible HX elimination/addition as an additional isomerization pathway (Fig. 1a). We further proposed that pairing isomerization with a tandem substitution reaction could provide a driving force for nontraditional selectivity in aromatic substitution reactions (Fig. 1b).11 We herein describe initial studies on a general approach to catalytic aryl halide isomerization and demonstrate its utility as a new route to 4-functionalized pyridines.12
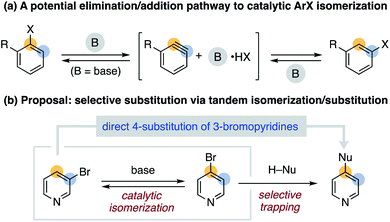 |
| Fig. 1 A general approach to aryl halide isomerization and its application to a new selective substitution reaction. | |
Results and discussion
We speculated bases that reversibly deprotonate aryl C–H bonds may create conditions capable of isomerizing aryl halides.13 This led us to investigate the non-nucleophilic organic superbase P4-t-Bu (pKBH+ 30.2 in DMSO) as a potential isomerization catalyst.14 In 1,4-dioxane, we discovered P4-t-Bu catalyzes the isomerization of 2-bromobenzotrifluoride (1) into all possible regioisomers (Scheme 2a). Under these conditions, 3-bromobenzotrifluoride (2) and 4-bromobenzotrifluoride (3) interconvert but do not form 2-bromobenzotrifluoride (Scheme 2b). No protodehalogenated or polyhalogenated side products are observed, and we note isomerization occurs to a lesser extent for 4-iodobenzotrifluoride (4).15 A variety of other bromoarenes (5, 6 and 7) also isomerize, including the formation of 4-bromopyridine from 3-bromopyridine (8).16 Although further studies on the scope of this process are ongoing, these observations suggest P4-t-Bu-catalyzed aryl halide isomerization is a general and reversible process.
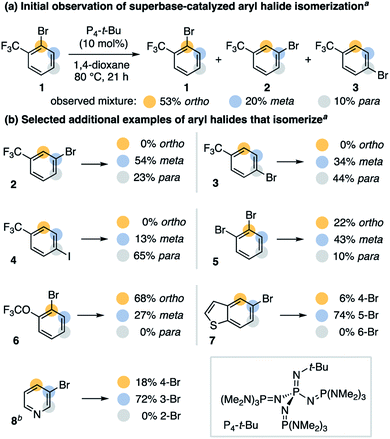 |
| Scheme 2 P4-t-Bu-catalyzed aryl halide isomerization. a Yields determined by 1H NMR spectroscopy; the mass balance is less than 100% with no observed haloarene side products; conditions for (b) are as shown in (a). b Reaction performed in cyclohexane for 14 h. | |
As a broader objective, we questioned if aryl halide isomerization could be utilized to address current challenges in aromatic functionalization. As a halogen migrates around an arene, we reasoned that differing electronic properties of isomeric C–X bonds could provide a source to differentiate interconverting isomers and drive an overall selective transformation.17 A mechanistic outline for the application of this concept to the 4-substitution of 3-bromopyridines is shown in Scheme 3. This pathway exploits the inherent preference for 4-bromopyridines to undergo nucleophilic aromatic substitution (SNAr) over 3-bromopyridines.18 However, a likely challenge is avoiding nucleophilic addition to the proposed 3,4-pyridyne intermediate, as this could decrease the desired reaction's yield and regioselectivity.19 Successful development of this protocol would offer an attractive route to 4-functionalized pyridines from 3-bromopyridines, which are more commercially available20 and stable21 than 4-halogenated congeners. This method would also complement other recently developed methods for 4-selective nucleophilic pyridine C–H functionalization, including McNally's powerful heterocyclic phosphonium salt approach.22,23
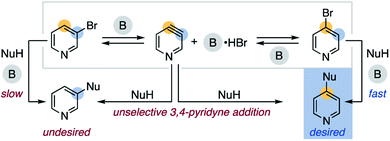 |
| Scheme 3 Proposed pathway for the 4-selective substitution of 3-bromopyridine (B = base, NuH = nucleophile). | |
As the proposed process requires stoichiometric base, we first investigated the use of hydroxide bases as more practical reagents for promoting aryl halide isomerization. Hydroxide bases are known to generate and be compatible with aryne intermediates, and we identified that 3-bromopyridines isomerize in the presence of 18-crown-6-ligated KOH in N,N-dimethylacetamide (see ESI†).24 Using these conditions, we then employed two separate strategies for optimizing a 4-selective etherification reaction of 3-bromopyridine (Table 1). When 1 equivalent of 3-bromopyridine (8) reacts with 4 equivalents of alcohol 9, a 2.4
:
1 ratio of 4:3-substituted product (10
:
11) is obtained in 54% overall yield (entry 1). This ratio is comparable to reported selectivities for nucleophilic additions to 3,4-pyridyne, which typically range from 1
:
1 to 3
:
1 for 4
:
3-addition selectivity.25 The 4-selectivity increases as higher ratios of pyridine
:
alcohol (8
:
9) are used, a result perhaps explainable by less alcohol intercepting a 3,4-pyridyne intermediate (entries 2–5). Based on this observation, we hypothesized that added bromide salts may enable more efficient isomerization and prevent undesired side reactions.26 When 50 mol% KBr is added to a reaction using a 1.5
:
1 pyridine
:
alcohol (8
:
9) ratio, the yield increases to 76% with >14
:
1 4-selectivity compared to 67% yield and 8.6
:
1 4-selectivity in the absence of bromide salt (entries 6–10).
Table 1 Optimization of 4-selective etherification of 3-bromopyridinea
A reaction profile with the optimized conditions shows the rapid formation of a low concentration of 4-bromopyridine (approximately 5%) that decreases as the reaction reaches completion (Scheme 4a). Subjection of the 3-substituted ether product (11) to the reaction conditions does not result in mass balance loss, indicating the high 4-selectivity is not a result of selective decomposition or product rearrangement.27 To test for the generation of 3,4-pyridyne under these conditions, when the alcohol is replaced with an excess of furan (12) the corresponding cycloadduct 13 forms in 42% yield (Scheme 4b).28 We also subjected 3-iodopyridine (14) to the reaction conditions in the absence of alcohol; in the presence of furan (12) cycloadduct 13 forms and in the presence of KBr a mixture of 3- and 4-bromopyridine form (8 and 15, Scheme 4c). The observed mixture of 3- and 4-bromopyridine supports the proposal of bromide addition to 3,4-pyridyne. Overall, these results are consistent with an isomerization pathway via 3,4-pyridyne and 4-substitution selectivity driven by a facile SNAr reaction.
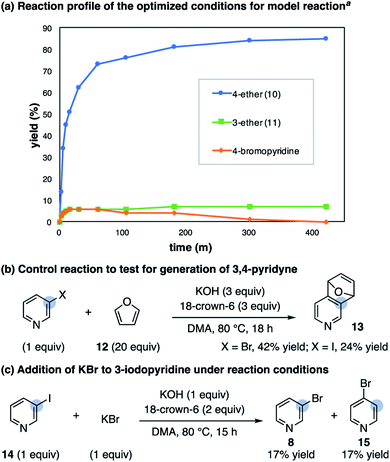 |
| Scheme 4 Mechanistic studies on the 4-selective etherification of 3-bromopyridine. a Conditions as shown in Table 1 using 1.5 : 1 ratio of 8 : 9 with 50 mol% KBr additive; see ESI† for details. | |
A substrate scope for the 4-selective etherification of 3-bromopyridines is provided in Table 2.29 A range of primary and secondary alcohols are first shown using 1.5 equiv. of simple bromopyridines. Sterically hindered alcohols (16) and those containing amino groups (17, 23, and 24), a terminal alkene (18) and a protected sugar (22) are suitable nucleophiles. Pyridines methylated in all positions react in high yield and selectivity (19, 20, 21 and 24), indicating that steric hindrance and acidic C–H bonds are tolerated on the arene. Pyridine biaryl substrates also selectively couple in the 4-position (25 and 26). Both 2-alkoxy (23) and 2-amino (28) substituents on the pyridine are tolerated, although we note the high selectivity observed for these substrates could originate from alcohol addition to a distorted 3,4-pyridyne intermediate.19 Pyridine substrates with more electron-withdrawing groups undergo direct 3-substitution under the current reaction conditions (e.g. 3-bromo-2-(trifluoromethyl)pyridine).30
Table 2 Scope of the 4-etherification of 3-bromopyridinesa
Yields are of purified 4-ether product; regioselectivities determined by 1H NMR spectroscopy of the crude reaction mixture.
Selectivity > 6 : 1; see ESI for details.
P4-t-Bu (1.3 equiv.) used as base in place of KOH.
|
|
An advantage of this functionalization strategy is demonstrated with substrates 29–33 in Table 2, where the bromopyridine substrate is obtained from commercial pyridines while a 4-halogenated isomer is either not available or significantly more expensive (see ESI† for a discussion). Using 1–1.2 equiv. of these bromopyridines, 4-substituted products featuring a carbamate (29), an acidic amide (30), a 2,6-disubstituted pyridine (31), a nicotine isomer (32) and a ketal (33) can be rapidly accessed.
This strategy can also utilize an arene's innate halogenation position as an entry to functionalizing more difficult to access C–H bonds. This is demonstrated in Scheme 5, where the 2,6-disubstituted pyridine 34 undergoes facile but unselective bromination in the 3- and 5-positions (35a and 35b). Application of conditions from Table 2 provides access to the 4-ether 36 through convergence of the regioisomeric bromopyridine mixture, highlighting an additional benefit of this methodology.31
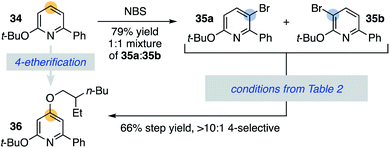 |
| Scheme 5 4-selective etherification of a 2,6-disubstituted pyridine. | |
We next examined if other nucleophiles can participate in the 4-selective substitution of 3-bromopyridines. We found that indolines are effective coupling partners and this route provides straightforward access to 4-aminopyridines from readily available 3-bromopyridines (37–40, Scheme 6a). The 4-amination of 3-bromopyridine with indoline proceeds on gram scale with excellent selectivity (37). A single isomer of the 5-bromoindoline product 38 is obtained, demonstrating the chemoselectivity of aryl halide isomerization.
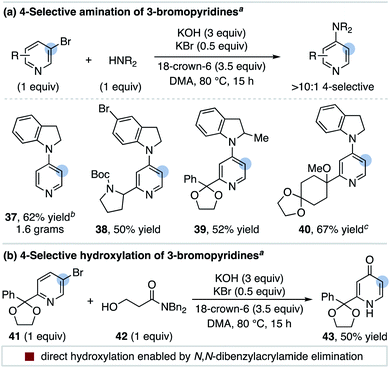 |
| Scheme 6 The 4-substitution of 3-bromopyridines with additional nucleophiles. a Isolated yield of purified 4-substituted products; selectivities determined by 1H NMR spectroscopy of crude reaction mixtures; b 1.5 equiv. of 3-bromopyridine used; c selectivity 9 : 1. | |
It is interesting to note that 4-hydroxypyridine side products are not typically observed for the reactions in Table 2 even though KOH is used as a base.32 To develop a 4-hydroxylation protocol, we instead hypothesized tandem isomerization/substitution could be further sequenced with a base-promoted elimination step. This is demonstrated in Scheme 6b, where the use of β-hydroxyamide 42 as a nucleophile directly delivers the 4-hydroxylated product 43 in 50% yield with >10
:
1 selectivity. We speculate this reaction proceeds through the standard 4-substitution pathway followed by a facile base-promoted acrylamide elimination reaction.33
Conclusions
This work demonstrates base-catalyzed aryl halide isomerization can be paired with SNAr reactivity to achieve unconventional substitution selectivity. In contrast, established “halogen dance” methodology relies on the controlled rearrangement of specific classes of stoichiometrically metalated haloarenes prior to treatment with electrophiles.3 Thus, tandem isomerization/selective interception may be a complementary and general strategy for achieving nontraditional selectivities in aryl halide functionalization chemistry.
Conflicts of interest
There are no conflicts to declare.
Acknowledgements
This work was supported by startup funds from Colorado State University. Acknowledgment is made to the Donors of the American Chemical Society Petroleum Research Fund for support of this research (ACS PRF #60171-DNI1). We thank Professors Andrew McNally (CSU) and Yiming Wang (Pittsburgh) for input on this manuscript.
Notes and references
- For selected modes of reactivity, see:
(a)
F. Terrier, Modern Nucleophilic Aromatic Substitution, Wiley-VCH, Weinheim, 2013 Search PubMed;
(b) J. Magano and J. R. Dunetz, Chem. Rev., 2011, 111, 2177–2250 Search PubMed;
(c) N. Zhang, S. R. Samanta, B. M. Rosen and V. Percec, Chem. Rev., 2014, 114, 5848–5958 Search PubMed;
(d) D. Seyferth, Organometallics, 2009, 28, 1598–1605 Search PubMed;
(e) J. Twilton, C. Le, P. Zhang, M. H. Shaw, R. W. Evans and D. W. C. MacMillan, Nat. Rev. Chem., 2017, 1, 0052 Search PubMed;
(f) J. F. Bunnett, J. Chem. Educ., 1974, 51, 312–315 Search PubMed.
- For selected examples of acid-catalyzed rearrangement and disproportionation of haloarenes, see:
(a) T. L. Jacobs, S. Winstein, J. W. Ralls and J. H. Robson, J. Org. Chem., 1946, 11, 27–33 Search PubMed;
(b) G. A. Olah, W. S. Tolgyesi and R. E. A. Dear, J. Org. Chem., 1962, 27, 3455–3464 Search PubMed;
(c) G. A. Olah and M. W. Meyer, J. Org. Chem., 1962, 27, 3464–3469 Search PubMed;
(d) J.-C. Jacquesy and M.-P. Jouannetaud, Tetrahedron Lett., 1982, 23, 1673–1676 Search PubMed.
-
(a) M. Schnürch, M. Spina, A. F. Khan, M. D. Mihovilovic and P. Stanetty, Chem. Soc. Rev., 2007, 36, 1046–1057 Search PubMed;
(b) M. Schlosser, Angew. Chem., Int. Ed., 2005, 44, 376–393 Search PubMed;
(c) W. Erb and F. Mongin, Tetrahedron, 2016, 72, 4973–4988 Search PubMed.
-
(a) J. F. Bunnett, Acc. Chem. Res., 1972, 5, 139–147 Search PubMed;
(b) C. E. Moyer Jr. and J. F. Bunnett, J. Am. Chem. Soc., 1963, 85, 1891–1893 Search PubMed;
(c) J. F. Bunnett and G. Scorrano, J. Am. Chem. Soc., 1971, 93, 1190–1198 Search PubMed.
-
(a) J. F. Bunnett and C. E. Moyer Jr., J. Am. Chem. Soc., 1971, 93, 1183–1190 Search PubMed;
(b) J. F. Bunnett and F. J. Kearley Jr., J. Org. Chem., 1971, 36, 184–186 Search PubMed.
- For selected applications in target-oriented synthesis, see:
(a) T. Sammakia, E. L. Stangeland and M. C. Whitcomb, Org. Lett., 2002, 4, 2385–2388 Search PubMed;
(b) F. Guillier, F. Nivoliers, A. Godard, F. Marsais and G. Quéguiner, Tetrahedron Lett., 1994, 35, 6489–6492 Search PubMed;
(c) D. L. Contins and J. K. Saha, Tetrahedron Lett., 1995, 36, 7995–7998 Search PubMed.
- For examples of the halogen dance on halopyridines, see:
(a) M. Mallet and G. Quéguiner, Tetrahedron, 1985, 41, 3433–3440 Search PubMed;
(b) E. Marzi, C. Bobbio, F. Cottet and M. Schlosser, Eur. J. Org. Chem., 2005, 2005, 2116–2123 Search PubMed;
(c) E. Marzi, A. Bigi and M. Schlosser, Eur. J. Org. Chem., 2001, 2001, 1371–1376 Search PubMed.
- For examples of reports that describe a rearranged aryl halide as a side product potentially derived from an aryne intermediate, see:
(a) G. W. Dalman and F. W. Neumann, J. Am. Chem. Soc., 1968, 90, 1601–1605 Search PubMed;
(b) M. Zoratti and J. F. Bunnett, J. Org. Chem., 1980, 45, 1769–1776 Search PubMed;
(c) S. Blanchard, I. Rodriguez, C. Kuehm-Caubère, P. Renard, B. Pfeiffer, G. Guillaumet and P. Caubère, Tetrahedron, 2002, 58, 3513–3524 Search PubMed.
- For reviews of aryne reactivity, see:
(a) H. Pellissier and M. Santelli, Tetrahedron, 2003, 59, 701–730 Search PubMed;
(b) M. G. Reinecke, Tetrahedron, 1982, 38, 427–498 Search PubMed;
(c) A. E. Goetz and N. K. Garg, J. Org. Chem., 2014, 79, 846–851 Search PubMed;
(d) P. M. Tadross and B. M. Stoltz, Chem. Rev., 2012, 112, 3550–3577 Search PubMed.
- For examples of halide addition to aryne intermediates, see:
(a) G. Wittig and R. W. Hoffmann, Chem. Ber., 1962, 95, 2729–2734 Search PubMed;
(b) T. Hamura, Y. Chuda, Y. Nakatsuji and K. Suzuki, Angew. Chem., Int. Ed., 2012, 51, 3368–3372 Search PubMed;
(c) V. V. Grushin and W. J. Marshall, Organometallics, 2008, 27, 4825–4828 Search PubMed;
(d) S. Bhattacharjee, A. Guin, R. N. Gaykar and A. T. Biju, Org. Lett., 2019, 21, 4383–4387 Search PubMed.
- For reviews of vicarious and cine-substitution reactions, see:
(a) J. Suwiński and K. Świerczek, Tetrahedron, 2001, 57, 1639–1662 Search PubMed;
(b) M. M
kosza and K. Wojciechowski, Chem. Rev., 2004, 104, 2631–2666 Search PubMed;
(c) M. M
kosza and J. Winiarski, Acc. Chem. Res., 1987, 20, 282–289 Search PubMed;
(d) J. Wang and G. Dong, Chem. Rev., 2019, 119, 7478–7528 Search PubMed.
- For examples of cine-substitution reactions on 3-bromopyridines, see:
(a) K. Vinter-Pasquier, B. Jamart-Grégoire and P. Caubère, Heterocycles, 1997, 45, 2113–2129 Search PubMed;
(b) K. B. Smith, Y. Huang and M. K. Brown, Angew. Chem., Int. Ed., 2018, 57, 6146–6149 Search PubMed;
(c) Z. Dong, G. Lu, J. Wang, P. Liu and G. Dong, J. Am. Chem. Soc., 2018, 140, 8551–8562 Search PubMed.
-
(a) I. Popov, H.-Q. Do and O. Daugulis, J. Org. Chem., 2009, 74, 8309–8313 Search PubMed;
(b) T. Imahori and Y. Kondo, J. Am. Chem. Soc., 2003, 125, 8082–8083 Search PubMed;
(c) J. A. Zoltewicz and C. L. Smith, J. Am. Chem. Soc., 1966, 88, 4766–4767 Search PubMed.
-
(a) R. Schwesinger and H. Schlemper, Angew. Chem., Int. Ed., 1987, 26, 1167–1169 Search PubMed;
(b)
Superbases for Organic Synthesis: Guanidines, Amidines, Phosphazenes and Related Organocatalysts, ed. T. Ishikawa, John Wiley & Sons, Ltd, Chichester, UK, 2009 Search PubMed.
-
(a) This is consistent with known rates of formation of benzyne and selectivities for benzyne additions although we cannot rule out other pathways at this time for these substrates, see ref. 9 for reviews;
(b) For examples of classical halogen dance on bromobenzotrifluorides, see: F. Mongin, O. Desponds and M. Schlosser, Tetrahedron Lett., 1996, 37, 2767–2770 Search PubMed.
- M. Mallet and G. Quénguiner, Tetrahedron, 1982, 38, 3035–3042 Search PubMed.
-
(a) S. Rohrbach, A. J. Smith, J. H. Pang, D. L. Poole, T. Tuttle, S. Chiba and J. A. Murphy, Angew. Chem., Int. Ed., 2019, 58, 16368–16388 Search PubMed;
(b) S. Schröter, C. Stock and T. Bach, Tetrahedron, 2005, 61, 2245–2267 Search PubMed.
- M. Lloung, A. Loupy, S. Marque and A. Petit, Heterocycles, 2004, 63, 297–308 Search PubMed.
- For discussions on 3,4-pyridynes, see:
(a) A. E. Goetz and N. K. Garg, Nat. Chem., 2013, 5, 54–60 Search PubMed;
(b) A. E. Goetz, S. M. Bronner, J. D. Cisneros, J. M. Melamed, R. S. Paton, K. N. Houk and N. K. Garg, Angew. Chem., Int. Ed., 2012, 51, 2758–2762 Search PubMed.
- 3-Bromopyridines are substantially more available in chemical catalogs of various suppliers, including the online database E-mail: https://www.emolecules.com (accessed March 2, 2020); for a discussion and examples of the 3-halogenation of pyridines, see
(a)
J. A. Joule and K. Mills, Heterocyclic Chemistry, Wiley, West Sussex, 5th edn, 2011 Search PubMed;
(b) V. Cañibano, J. F. Rodríguez, M. Santos, M. A. Sanz-Tejedor, M. C. Carreño, G. González and J. L. García-Ruano, Synthesis, 2001, 14, 2175–2179 Search PubMed;
(c) B. Das, K. Venkateswarlu, A. Majhi, V. Siddaiah and K. R. Reddy, J. Mol. Catal. A: Chem., 2007, 267, 30–33 Search PubMedfor a recent 4-selective pyridine halogenation method, see:
(d) J. N. Levy, J. V. Alegre-Requena, R. Liu, R. S. Paton and A. McNally, J. Am. Chem. Soc., 2020, 142, 11295–11305 Search PubMed.
-
(a) W. J. Feast and J. Tsibouklis, Polym. Int., 1994, 35, 67–74 Search PubMed;
(b) J. P. Wibaut and F. W. Broekman, Recl. Trav. Chim. Pays-Bas, 1959, 78, 593–603 Search PubMed;
(c) E. L. Fisher, C. W. am Ende and J. M. Humphrey, J. Org. Chem., 2019, 84, 4904–4909 Search PubMed.
- For reviews and examples of 4-pyridine C–H functionalization to C–O and C–N bonds, see:
(a) D. E. Stephens and O. V. Larionov, Tetrahedron, 2015, 71, 8683–8716 Search PubMed;
(b) K. Murakami, S. Yamada, T. Kaneda and K. Itami, Chem. Rev., 2017, 117, 9302–9332 Search PubMed;
(c) R. J. Sundberg and S. Jiang, Org. Prep. Proced. Int., 1997, 29, 117–122 Search PubMed;
(d) Y. Gu, Y. Shen, C. Zarate and R. Martin, J. Am. Chem. Soc., 2019, 141, 127–132 Search PubMed;
(e) J.-Y. Cho, M. K. Tse, D. Holmes, R. E. Maleczka Jr and M. R. Smith, III, Science, 2002, 295, 305–308 Search PubMed;
(f) L. Yang, K. Semba and Y. Nakao, Angew. Chem., Int. Ed., 2017, 56, 4853–4857 Search PubMed.
-
(a) R. D. Dolewski, M. C. Hilton and A. McNally, Synlett, 2018, 29, 8–14 Search PubMed;
(b) M. C. Hilton, R. D. Dolewski and A. McNally, J. Am. Chem. Soc., 2016, 138, 13806–13809 Search PubMed;
(c) R. G. Anderson, B. M. Jett and A. McNally, Angew. Chem., Int. Ed., 2018, 57, 12514–12518 Search PubMed;
(d) C. Patel, M. Mohnike, M. C. Hilton and A. McNally, Org. Lett., 2018, 20, 2607–2610 Search PubMed.
- For examples and discussions of using alkoxide and hydroxide bases in aryne chemistry, see:
(a) Y. Apeloig, D. Arad, B. Halton and C. J. Randall, J. Am. Chem. Soc., 1986, 108, 4932–4937 Search PubMed;
(b) Y. Dong, M. I. Lipschutz and T. D. Tilley, Org. Lett., 2016, 18, 1530–1533 Search PubMed;
(c) Y. Yuan, I. Thomé, S. H. Kim, D. Chen, A. Beyer, J. Bonnamour, E. Zuidema, S. Chang and C. Bolm, Adv. Synth. Catal., 2010, 352, 2892–2898 Search PubMed;
(d) P. H. Willoughby, D. Niu, T. Wang, M. K. Haj, C. J. Cramer and T. R. Hoye, J. Am. Chem. Soc., 2014, 136, 13657–13665 Search PubMed.
- For examples of low selectivities for addition to unbiased 3,4-pyridynes, see ref. 9, 19 and
(a) J. A. Zoltewicz and C. Nisi, J. Org. Chem., 1969, 34, 765–766 Search PubMed;
(b) B. Jamart-Gregoire, C. Leger and P. Caubère, Tetrahedron Lett., 1990, 31, 7599–7602 Search PubMed. For changes in selectivities of alcohol addition to arynes depending on conditions used, see:
(c) J. F. Bunnett, D. A. R. Happer, M. Patsch, C. Pyun and H. Takayama, J. Am. Chem. Soc., 1966, 88, 5250–5254 Search PubMed;
(d) M. Stiles, R. G. Miller and U. Burckhardt, J. Am. Chem. Soc., 1963, 85, 1792–1797 Search PubMed . It is also possible that direct 3-substitution of 8 contributes to the low 4:3-substitution regioselectivity.
- A similar strategy was tested to no effect by Bunnett to rule out a benzyne intermediate in tribromobenzene isomerization, see ref. 5.
- We note that 3,4-dibromopyridine is not observed during the course of the reaction, nor does the subjection of the 3-brominated derivative of 10 to the reaction conditions result in debromination to give 10. These results suggest an intermolecular halogen transfer pathway is not involved in the 4-etherification reaction.
- S. J. Connon and A. F. Hegarty, J. Chem. Soc., Perkin Trans. 1, 2000, 1245–1249 Search PubMed.
- A variety of substrates from Table 2 were subjected to the reaction conditions in the absence of alcohol; 4-bromopyridines are observed, indicating the likely involvement of aryl halide isomerization; see ESI† for details.
- For a similar SNAr reaction, see: C. E. Basséne, F. Suzenet, N. Hennuyer, B. Staels, D.-H. Caignard, C. Dacquet, P. Renard and G. Guillaumet, Bioorg. Med. Chem. Lett., 2006, 16, 4528–4532 Search PubMed.
- See ref. 12c for a similar convergence example in a Pd/norbornene co-catalyzed 4-selective amination reaction using N-hydroxylamine ester electrophiles.
- In the reactions reported in Table 2, less than 5% of 4-hydroxypyridine side products are typically observed. Attempts to perform the reaction in the absence of alcohol did not significantly increase the yield of hydroxylation products.
-
N,N-Dibenzyl acrylamide is observed as the major byproduct in this reaction. For related hydroxylation strategies, see:
(a) A. Revuelto, M. Ruiz-Santaquiteria, H. de Lucio, A. Gamo, A. A. Carriles, K. J. Gutiérrez, P. A. Sánchez-Murcia, J. A. Hermoso, F. Gago, M.-J. Camarasa, A. Jiménez-Ruiz and S. Velázquez, ACS Infect. Dis., 2019, 5, 873–891 Search PubMed;
(b) J. F. Rogers and D. M. Green, Tetrahedron Lett., 2002, 43, 3585–3587 Search PubMed.
Footnote |
† Electronic supplementary information (ESI) available. See DOI: 10.1039/d0sc02689a |
|
This journal is © The Royal Society of Chemistry 2020 |