DOI:
10.1039/D0SC01515F
(Edge Article)
Chem. Sci., 2020,
11, 10764-10769
Regioselective B(3,4)–H arylation of o-carboranes by weak amide coordination at room temperature†
Received
12th March 2020
, Accepted 3rd May 2020
First published on 5th May 2020
Abstract
Palladium-catalyzed regioselective di- or mono-arylation of o-carboranes was achieved using weakly coordinating amides at room temperature. Therefore, a series of B(3,4)-diarylated and B(3)-monoarylated o-carboranes anchored with valuable functional groups were accessed for the first time. This strategy provided an efficient approach for the selective activation of B(3,4)–H bonds for regioselective functionalizations of o-carboranes.
o-Carboranes, icosahedral carboranes – three-dimensional arene analogues – represent an important class of carbon–boron molecular clusters.1 The regioselective functionalization of o-carboranes has attracted growing interest due to its potential applications in supramolecular design,2 medicine,3 optoelectronics,4 nanomaterials,5 boron neutron capture therapy agents6 and organometallic/coordination chemistry.7 In recent years, transition metal-catalyzed cage B–H activation for the regioselective boron functionalization of o-carboranes has emerged as a powerful tool for molecular syntheses. However, the 10 B–H bonds of o-carboranes are not equal, and the unique structural motif renders their selective functionalization difficult, since the charge differences are very small and the electrophilic reactivity in unfunctionalized o-carboranes reduces in the following order: B(9,12) > B(8,10) > B(4,5,7,11) > B(3,6).8 Therefore, efficient and selective boron substitution of o-carboranes continues to be a major challenge.
Recently, transition metal-catalyzed carboxylic acid or formyl-directed B(4,5)–H functionalization of o-carboranes has drawn increasing interest, since it provides an efficient approach for direct regioselective boron–carbon and boron–heteroatom bond formations (Scheme 1a),9 with major contributions by the groups of Xie,10 and Yan,11 among others.12 Likewise, pyridyl-directed B(3,6)–H acyloxylations (Scheme 1b),13 and amide-assisted B(4,7,8)–H arylations14 (Scheme 1c) have been enabled by rhodium or palladium catalysis, respectively.15,16 Despite indisputable progress, efficient approaches for complementary site-selective functionalizations of o-carboranes are hence in high demand.17 Hence, metal-catalyzed position-selective B(3,4)–H functionalizations of o-carboranes have thus far not been reported.
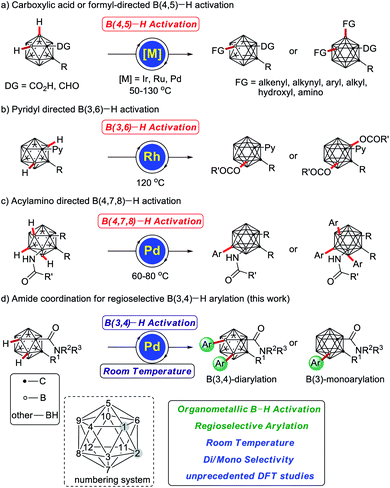 |
| Scheme 1 Chelation-assisted transition metal-catalyzed cage B–H activation of o-carboranes. | |
Arylated compounds represent key structural motifs in inter alia functional materials, biologically active compounds, and natural products.18 In recent years, transition metal-catalyzed chelation-assisted arylations have received significant attention as environmentally benign and economically superior alternatives to traditional cross-coupling reactions.19 Within our program on sustainable C–H activation,20 we have now devised a protocol for unprecedented cage B–H arylations of o-carboranes with weak amide assistance, on which we report herein. Notable features of our findings include (a) transition metal-catalyzed room temperature B–H functionalization, (b) high levels of positional control, delivering B(3,4)-diarylated and B(3)-monoarylated o-carboranes, and (c) mechanistic insights from DFT computation providing strong support for selective B–H arylation (Scheme 1d).
We initiated our studies by probing various reaction conditions for the envisioned palladium-catalyzed B–H arylation of o-carborane amide 1a with 1-iodo-4-methylbenzene (2a) at room temperature (Tables 1 and S1†). We were delighted to observe that the unexpected B(3,4)-di-arylated product 3aa was obtained in 59% yield in the presence of 10 mol% Pd(OAc)2 and 2 equiv. of AgTFA, when HFIP was employed as the solvent, which proved to be the optimal choice (entries 1–5).21 Control experiments confirmed the essential role of the palladium catalyst and silver additive (entries 6–7). Further optimization revealed that AgOAc, Ag2O, K2HPO4, and Na2CO3 failed to show any beneficial effect (entries 8–11). Increasing the reaction temperature fell short in improving the performance (entries 12 and 13). The replacement of the amide group in substrate 1a with a carboxylic acid, aldehyde, ketone, or ester group failed to afford the desired arylation product (see the ESI†). We were pleased to find that the use of 1.0 equiv. of trifluoroacetic acid (TFA) as an additive improved the yield to 71% (entry 14). To our delight, replacing the silver additive with Ag2CO3 resulted in the formation of B(3)–H mono-arylation product 4aa as the major product (entries 15–16).
Table 1 Optimization of reaction conditionsa
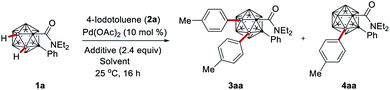
|
Entry |
Additive |
Solvent |
Yield of 3aa/% |
Yield of 4aa/% |
Reaction conditions: 1a (0.20 mmol), 2 (0.48 mmol), Pd(OAc)2 (10 mol%), additive (0.48 mmol), solvent (0.50 mL), 25 °C, 16 h, and isolated yield.
Without Pd(OAc)2.
At 40 °C.
At 60 °C.
TFA (0.2 mmol) was added.
1a (0.20 mmol), 2a (0.24 mmol), Pd(OAc)2 (5.0 mol%), and Ag2CO3 (0.24 mmol).
2a was added in three portions every 4 h. DCE = dichloroethane, TFE = 2,2,2-trifluoroethanol, HFIP = hexafluoroisopropanol, and TFA = trifluoroacetic acid.
|
1 |
AgTFA |
PhMe |
0 |
0 |
2 |
AgTFA |
DCE |
0 |
0 |
3 |
AgTFA |
1,4-Dioxane |
0 |
0 |
4 |
AgTFA |
TFE |
21 |
3 |
5 |
AgTFA |
HFIP |
59 |
4 |
6 |
AgTFA |
HFIP |
0 |
0b |
7 |
— |
HFIP |
0 |
0 |
8 |
AgOAc |
HFIP |
5 |
<3 |
9 |
Ag2O |
HFIP |
<3 |
<3 |
10 |
K2HPO4 |
HFIP |
0 |
0 |
11 |
Na2CO3 |
HFIP |
0 |
0 |
12 |
AgTFA |
HFIP |
53 |
4c |
13 |
AgTFA |
HFIP |
42 |
3d |
14
|
AgTFA
|
HFIP
|
71
|
<3
|
15 |
Ag2CO3 |
HFIP |
9 |
34f |
16
|
Ag
2
CO
3
|
HFIP
|
5
|
55
,
|
With the optimized reaction conditions in hand, we probed the scope of the B–H di-arylation of o-carboranes 1a with different aryl iodides 2 (Scheme 2). The versatility of the room temperature B(3,4)–H di-arylation was reflected by tolerating valuable functional groups, including bromo, chloro, and enolizable ketone substituents. The connectivity of the products 3aa and 3ab was unambiguously verified by X-ray single crystal diffraction analysis.22
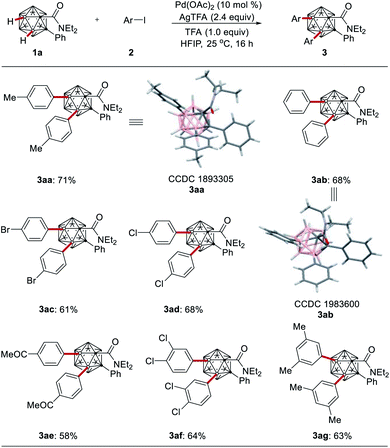 |
| Scheme 2 Cage B(3,4)–H di-arylation of o-carboranes. | |
Next, we explored the effect exerted by the N-substituent at the amide moiety (Scheme 3). Tertiary amides 1b–1f proved to be suitable substrates with optimal results being accomplished with substrate 1a. The effect of varying the cage carbon substituents R1 on the reaction's outcome was also probed, and both aryl and alkyl substituents gave the B–H arylation products and the molecular structures of the products 3dd, 3ea and 3fa were fully established by single-crystal X-ray diffraction.
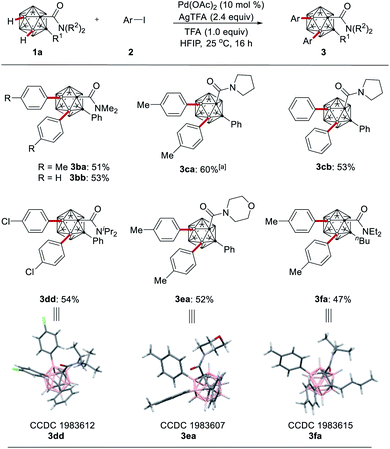 |
| Scheme 3 Effect of substituents on B–H diarylation. aAt 50 °C. | |
The robustness of the palladium-catalyzed B–H functionalization was subsequently investigated for the challenging catalytic B–H monoarylation of o-carboranes (Scheme 4). The B(3)–H monoarylation, as confirmed by single-crystal X-ray diffraction analysis of products 4aa and 4ai, proceeded smoothly with valuable functional groups, featuring aldehyde and nitro substituents, which should prove invaluable for further late-stage manipulation.
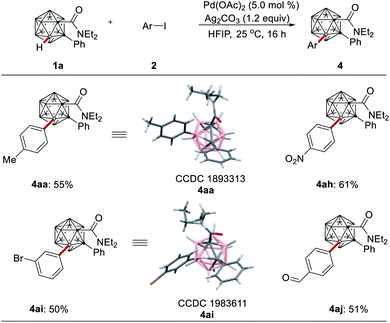 |
| Scheme 4 Cage B(3)–H mono-arylation of o-carboranes. | |
To elucidate the palladium catalysts' working mode, a series of experiments was performed. The reactions in the presence of TEMPO or 1,4-cyclohexadiene produced the desired product 3aa, which indicates that the present B–H arylation is less likely to operate via radical intermediates (Scheme 5a). The palladium catalysis carried out in the dark performed efficiently (Scheme 5b). Compound 4aa could be converted to di-arylation product 3aa with high efficiency, indicating that 4aa is an intermediate for the formation of the diarylated cage 3aa (Scheme 5c).
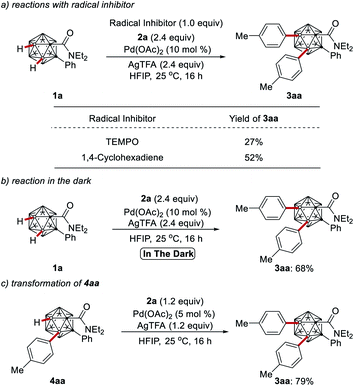 |
| Scheme 5 Control experiments. | |
To further understand the catalyst mode of action, we studied the site-selectivity of the o-carborane B–H activation for the first B–H activation at the B3 versus B4 position and for the second B–H activation at the B4 versus B6 position using density functional theory (DFT) at the PBE0-D3(BJ)/def2-TZVP+SMD(HFIP)//TPSS-D3(BJ)/def2-SVP level of theory (Fig. 1). Our computational studies show that the B3 position is 5.8 kcal mol−1 more favorable than the B4 position for the first B–H activation, while the B4 position is 3.4 kcal mol−1 more favorable than the B6 position for the second B–H activation. It is noteworthy that here the interaction between AgTFA and a cationic palladium(II) complex was the key to success, being in good agreement with our experimental results (for more details, see the ESI†).
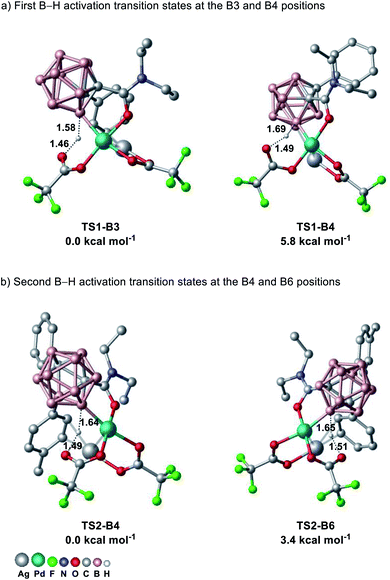 |
| Fig. 1 Computed relative Gibbs free energies in kcal mol−1 and the optimized geometries of the transition states involved in the B–H activation at the PBE0-D3(BJ)/def2-TZVP+SMD(HFIP)//TPSS-D3(BJ)/def2-SVP level of theory. (a) First B–H activation transition states at the B3 and B4 positions. (b) Second B–H activation transition states at the B4 and B6 positions. Irrelevant hydrogen atoms in the transition states are omitted for clarity and the bond lengths are given in Å. | |
A plausible reaction mechanism is proposed which commences with an organometallic B(3)–H activation of 1a with weak assistance of the amide group and assistance by AgTFA to form the cationic intermediate I (Scheme 6). Oxidative addition with the aryl iodide 2 affords the proposed cationic palladium(IV) intermediate II, followed by reductive elimination to give the B(3)-mono-arylation product 4aa. Subsequent B(4)-arylation occurs assisted by the weakly coordinating amide to generate the B(3,4)-di-arylation product 3aa. Due to the innate higher reactivity of the B(4)–H bond in intermediate 4aa – which is inherently higher than that of the B(6)–H bond – the B(3,6)-di-arylation product is not formed.
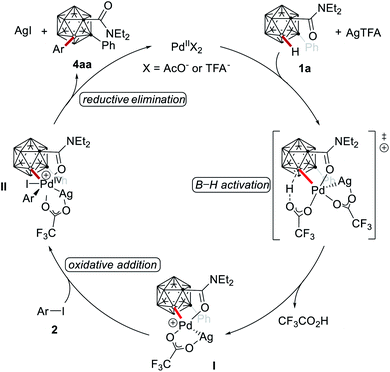 |
| Scheme 6 Proposed reaction mechanism. | |
In summary, room temperature palladium-catalyzed direct arylations at cage B(3,4) positions in o-carboranes have been achieved with the aid of weakly coordinating, synthetically useful amides. Thus, palladium-catalyzed B–H activations enable the assembly of a wealth of arylated o-carboranes. This method features high site-selectivity, high tolerance for functional groups, and mild reaction conditions, thereby offering a platform for the design and synthesis of boron-substituted o-carboranes. Our findings offer a facile strategy for selective activations of B(3,4)–H bonds, which will be instrumental for future design of optoelectronics, nanomaterials, and boron neutron capture therapy agents.
Conflicts of interest
There are no conflicts to declare.
Acknowledgements
Generous support by the Alexander von Humboldt Foundation (fellowship to Y. L.), the CSC (fellowship to L. Y.), the DAAD (fellowship to B. B. J.) and the DFG (Gottfried-Wilhelm-Leibniz prize to L. A.) is gratefully acknowledged. We also thank Dr J. Li for donation of chemicals and Dr Christopher Golz (University Göttingen) for support with the X-ray diffraction analysis.
Notes and references
-
(a)
R. N. Grimes, Carboranes, Academic Press, Amsterdam, 3rd edn, 2016 Search PubMed;
(b) Z. Xie and G.-X. Jin, Dalton Trans., 2014, 43, 4924–5133 RSC;
(c) J. Poater, M. Solà, C. Viñas and F. Teixidor, Angew. Chem., Int. Ed., 2014, 53, 12191–12195 CrossRef CAS PubMed;
(d)
N. S. Hosmane, Boron Science: New Technologies and Applications, Taylor & Francis Books/CRC Press, Boca Raton, FL, 2012 Search PubMed.
-
(a) J. J. Schwartz, A. M. Mendoza, N. Wattanatorn, Y. Zhao, V. T. Nguyen, A. M. Spokoyny, C. A. Mirkin, T. Baše and P. S. Weiss, J. Am. Chem. Soc., 2016, 138, 5957–5967 CrossRef CAS PubMed;
(b) R. N. Grimes, Dalton Trans., 2015, 44, 5939–5956 RSC;
(c) D. Brusselle, P. Bauduin, L. Girard, A. Zaulet, C. Viñas, F. Teixidor, I. Ly and O. Diat, Angew. Chem., Int. Ed., 2013, 52, 12114–12118 CrossRef CAS PubMed;
(d) A. M. Cioran, A. D. Musteti, F. Teixidor, Ž. Krpetić, I. A. Prior, Q. He, C. J. Kiely, M. Brust and C. Viñas, J. Am. Chem. Soc., 2012, 134, 212–221 CrossRef CAS PubMed;
(e) P. Bauduin, S. Prevost, P. Farràs, F. Teixidor, O. Diat and T. Zemb, Angew. Chem., Int. Ed., 2011, 50, 5298–5300 CrossRef CAS PubMed;
(f) B. P. Dash, R. Satapathy, E. R. Gaillard, J. A. Maguire and N. S. Hosmane, J. Am. Chem. Soc., 2010, 132, 6578–6587 CrossRef CAS PubMed.
-
(a) Z. J. Leśnikowski, J. Med. Chem., 2016, 59, 7738–7758 CrossRef PubMed;
(b) F. Issa, M. Kassiou and L. M. Rendina, Chem. Rev., 2011, 111, 5701–5722 CrossRef CAS PubMed;
(c) M. Scholz and E. Hey-Hawkins, Chem. Rev., 2011, 111, 7035–7062 CrossRef CAS PubMed.
-
(a) S. Mukherjee and P. Thilagar, Chem. Commun., 2016, 52, 1070–1093 RSC;
(b) R. Núñez, M. Tarrés, A. Ferrer-Ugalde, F. F. de Biani and F. Teixidor, Chem. Rev., 2016, 116, 14307–14378 CrossRef PubMed;
(c) X. Li, H. Yan and Q. Zhao, Chem.–Eur. J., 2016, 22, 1888–1898 CrossRef CAS PubMed.
-
(a) E. Q. Qian, A. I. Wixtrom, J. C. Axtell, A. Saebi, P. Rehak, Y. Han, E. H. Moully, D. Mosallaei, S. Chow, M. Messina, J.-Y. Wang, A. T. Royappa, A. L. Rheingold, H. D. Maynard, P. Kral and A. M. Spokoyny, Nat. Chem., 2017, 9, 333–340 CrossRef CAS PubMed;
(b) A. Saha, E. Oleshkevich, C. Viñas and F. Teixidor, Adv. Mater., 2017, 29, 1704238–1704245 CrossRef PubMed;
(c) A. C. Serino, M. E. Anderson, L. M. A. Saleh, R. M. Dziedzic, H. Mills, L. K. Heidenreich, A. M. Spokoyny and P. S. Weiss, ACS Appl. Mater. Interfaces, 2017, 9, 34592–34596 CrossRef CAS PubMed;
(d) C. J. Villagómez, T. Sasaki, J. M. Tour and L. Grill, J. Am. Chem. Soc., 2010, 132, 16848–16854 CrossRef PubMed.
-
(a) S. P. Fisher, A. W. Tomich, S. O. Lovera, J. F. Kleinsasser, J. Guo, M. J. Asay, H. M. Nelson and V. Lavallo, Chem. Rev., 2019, 119, 8262–8290 CrossRef CAS PubMed;
(b) A. F. Armstrong and J. F. Valliant, Dalton Trans., 2007, 4240–4251 RSC.
-
(a) S. P. Fisher, A. W. Tomich, J. Guo and V. Lavallo, Chem. Commun., 2019, 55, 1684–1701 RSC;
(b) Y.-P. Zhou, S. Raoufmoghaddam, T. Szilvási and M. Driess, Angew. Chem., Int. Ed., 2016, 55, 12868–12872 CrossRef CAS PubMed;
(c) M. Hailmann, N. Wolf, R. Renner, T. C. Schäfer, B. Hupp, A. Steffen and M. Finze, Angew. Chem., Int. Ed., 2016, 55, 10507–10511 CrossRef CAS PubMed;
(d) R. D. Adams, J. Kiprotich, D. V. Peryshkov and Y. O. Wong, Chem.–Eur. J., 2016, 22, 6501–6504 CrossRef CAS PubMed;
(e) A. El-Hellani and V. Lavallo, Angew. Chem., Int. Ed., 2014, 53, 4489–4493 CrossRef CAS PubMed;
(f) M. Joost, A. Zeineddine, L. Estévez, S. Mallet-Ladeira, K. Miqueu, A. Amgoune and D. Bourissou, J. Am. Chem. Soc., 2014, 136, 14654–14657 CrossRef CAS PubMed;
(g) Z.-J. Yao and G.-X. Jin, Coord. Chem. Rev., 2013, 257, 2522–2535 CrossRef CAS;
(h) Z. Qiu, S. Ren and Z. Xie, Acc. Chem. Res., 2011, 44, 299–309 CrossRef CAS PubMed;
(i)
N. S. Hosmane and J. A. Maguire in Comprehensive Organometallic Chemistry III, ed. R. H. Crabtree and D. M. P. Mingos, Elsevier, Oxford, 2007, vol. 3, pp. 175–264 Search PubMed.
- F. Teixidor, G. Barberà, A. Vaca, R. Kivekäs, R. Sillanpää, J. Oliva and C. Viñas, J. Am. Chem. Soc., 2005, 127, 10158–10159 CrossRef CAS PubMed.
-
(a) Y. Quan and Z. Xie, Chem. Soc. Rev., 2019, 48, 3660–3673 RSC;
(b) X. Zhang and H. Yan, Coord. Chem. Rev., 2019, 378, 466–482 CrossRef CAS;
(c) Y. Quan, C. Tang and Z. Xie, Dalton Trans., 2019, 48, 7494–7498 RSC;
(d) R. M. Dziedzic and A. M. Spokoyny, Chem. Commun., 2019, 55, 430–442 RSC;
(e) Y. Quan, Z. Qiu and Z. Xie, Chem.–Eur. J., 2018, 24, 2795–2805 CrossRef CAS PubMed;
(f) W.-B. Yu, P.-F. Cui, W.-X. Gao and G.-X. Jin, Coord. Chem. Rev., 2017, 350, 300–319 CrossRef CAS;
(g) D. Olid, R. Núñez, C. Viñas and F. Teixidor, Chem. Soc. Rev., 2013, 42, 3318–3336 RSC.
-
(a) Y. K. Au, H. Lyu, Y. Quan and Z. Xie, J. Am. Chem. Soc., 2019, 141, 12855–12862 CrossRef CAS PubMed;
(b) Y. Chen, Y. K. Au, Y. Quan and Z. Xie, Sci. China: Chem., 2019, 62, 74–79 CrossRef CAS;
(c) H. Lyu, Y. Quan and Z. Xie, Chem. Sci., 2018, 9, 6390–6394 RSC;
(d) H. Lyu, Y. Quan and Z. Xie, Chem.–Eur. J., 2017, 23, 14866–14871 CrossRef CAS PubMed;
(e) Y. Quan, H. Lyu and Z. Xie, Chem. Commun., 2017, 53, 4818–4821 RSC;
(f) R. Cheng, Z. Qiu and Z. Xie, Nat. Commun., 2017, 8, 14827 CrossRef CAS PubMed;
(g) H. Lyu, Y. Quan and Z. Xie, J. Am. Chem. Soc., 2016, 138, 12727–12730 CrossRef CAS PubMed;
(h) H. Lyu, Y. Quan and Z. Xie, Angew. Chem., Int. Ed., 2016, 55, 11840–11844 CrossRef CAS PubMed;
(i) Y. Quan and Z. Xie, Angew. Chem., Int. Ed., 2016, 55, 1295–1298 CrossRef CAS PubMed;
(j) Y. Quan, C. Tang and Z. Xie, Chem. Sci., 2016, 7, 5838–5845 RSC;
(k) H. Lyu, Y. Quan and Z. Xie, Angew. Chem., Int. Ed., 2015, 54, 10623–10626 CrossRef CAS PubMed;
(l) Y. Quan and Z. Xie, J. Am. Chem. Soc., 2014, 136, 15513–15516 CrossRef CAS PubMed.
-
(a) X. Zhang and H. Yan, Chem. Sci., 2018, 9, 3964–3969 RSC;
(b) X. Zhang, H. Zheng, J. Li, F. Xu, J. Zhao and H. Yan, J. Am. Chem. Soc., 2017, 139, 14511–14517 CrossRef CAS PubMed;
(c) H. Li, F. Bai, H. Yan, C. Lu and V. I. Bregadze, Eur. J. Org. Chem., 2017, 1343–1352 CrossRef.
-
(a) Y. Shen, K. Zhang, X. Liang, R. Dontha and S. Duttwyler, Chem. Sci., 2019, 10, 4177–4184 RSC;
(b) Q. Wang, S. Tian, C. Zhang, J. Li, Z. Wang, Y. Du, L. Zhou and J. Lu, Org. Lett., 2019, 21, 8018–8021 CrossRef CAS PubMed;
(c) F. Lin, J.-L. Yu, Y. Shen, S.-Q. Zhang, B. Spingler, J. Liu, X. Hong and S. Duttwyler, J. Am. Chem. Soc., 2018, 140, 13798–13807 CrossRef CAS PubMed;
(d) T.-T. Xu, K. Cao, J. Wu, C.-Y. Zhang and J. Yang, Inorg. Chem., 2018, 57, 2925–2932 CrossRef CAS PubMed;
(e) Y. Shen, Y. Pan, K. Zhang, X. Liang, J. Liu, B. Spingler and S. Duttwyler, Dalton Trans., 2017, 46, 3135–3140 RSC;
(f) K. Cao, T.-T. Xu, J. Wu, L. Jiang and J. Yang, Chem. Commun., 2016, 52, 11446–11449 RSC;
(g) Y. Zhang, Y. Sun, F. Lin, J. Liu and S. Duttwyler, Angew. Chem., Int. Ed., 2016, 55, 15609–15614 CrossRef CAS PubMed;
(h) K. Cao, Y. Huang, J. Yang and J. Wu, Chem. Commun., 2015, 51, 7257–7260 RSC.
- C.-X. Li, H.-Y. Zhang, T.-Y. Wong, H.-J. Cao, H. Yan and C.-S. Lu, Org. Lett., 2017, 19, 5178–5181 CrossRef CAS PubMed.
- H. Lyu, J. Zhang, J. Yang, Y. Quan and Z. Xie, J. Am. Chem. Soc., 2019, 141, 4219–4224 CrossRef CAS PubMed.
- For the preparation of functionalized o-carboranes via boron cluster synthesis, see:
(a) A. V. Safronov, N. I. Shlyakhtina and M. F. Hawthorne, Organometallics, 2012, 31, 2764–2769 CrossRef CAS;
(b) O. Bondarev, Y. V. Sevryugina, S. S. Jalisatgi and M. F. Hawthorne, Inorg. Chem., 2012, 51, 9935–9942 CrossRef CAS PubMed.
- For the synthesis of functionalized o-carboranes by iodonium and diazonium chemistry, see:
(a) D. Zhao and Z. Xie, Chem. Sci., 2016, 7, 5635–5639 RSC;
(b) D. Zhao and Z. Xie, Angew. Chem., Int. Ed., 2016, 55, 3166–3170 CrossRef CAS PubMed;
(c) V. V. Grushin, Acc. Chem. Res., 1992, 25, 529–536 CrossRef CAS;
(d) V. V. Grushin and T. M. Shcherbina, J. Organomet. Chem., 1985, 292, 105–117 CrossRef CAS.
-
(a) Y. Ge, J. Zhang, Z. Qiu and Z. Xie, Angew. Chem., Int. Ed., 2020, 59, 4851–4855 CrossRef CAS PubMed;
(b) C.-X. Cui, J. Zhang, Z. Qiu and Z. Xie, Dalton Trans., 2020, 49, 1380–1383 RSC;
(c) Y. K. Au, H. Lyu, Y. Quan and Z. Xie, Chin. J. Chem., 2020, 38, 383–388 CrossRef CAS;
(d) R. Cheng, Z. Qiu and Z. Xie, Chem.–Eur. J., 2020 DOI:10.1002/chem.202000549;
(e) Z. Yang, W. Zhao, W. Liu, X. Wei, M. Chen, X. Zhang, X. Zhang, Y. Liang, C. Lu and H. Yan, Angew. Chem., Int. Ed., 2019, 58, 11886–11892 CrossRef CAS PubMed;
(f) X. Wei, M.-J. Zhu, Z. Cheng, M. Lee, H. Yan, C. Lu and J.-J. Xu, Angew. Chem., Int. Ed., 2019, 58, 3162–3166 CrossRef CAS PubMed;
(g) T.-T. Xu, K. Cao, C.-Y. Zhang, J. Wu, L.-F. Ding and J. Yang, Org. Lett., 2019, 21, 9276–9279 CrossRef CAS;
(h) J. Wu, K. Cao, C.-Y. Zhang, T.-T. Xu, L.-F. Ding, B. Li and J. Yang, Org. Lett., 2019, 21, 5986–5989 CrossRef CAS PubMed;
(i) T.-T. Xu, K. Cao, C.-Y. Zhang, J. Wu, L. Jiang and J. Yang, Chem. Commun., 2018, 54, 13603–13606 RSC;
(j) C. Tang, J. Zhang, J. Zhang and Z. Xie, J. Am. Chem. Soc., 2018, 140, 16423–16427 CrossRef CAS PubMed;
(k) R. Cheng, B. Li, J. Wu, J. Zhang, Z. Qiu, W. Tang, S.-L. You, Y. Tang and Z. Xie, J. Am. Chem. Soc., 2018, 140, 4508–4511 CrossRef CAS PubMed;
(l) R. M. Dziedzic, J. L. Martin, J. C. Axtell, L. M. A. Saleh, T.-C. Ong, Y.-F. Yang, M. S. Messina, A. L. Rheingold, K. N. Houk and A. M. Spokoyny, J. Am. Chem. Soc., 2017, 139, 7729–7732 CrossRef CAS PubMed;
(m) Y. Quan and Z. Xie, J. Am. Chem. Soc., 2015, 137, 3502–3505 CrossRef CAS PubMed;
(n) J. Wu, K. Cao, T.-T. Xu, X.-J. Zhang, L. Jiang, J. Yang and Y. Huang, RSC Adv., 2015, 5, 91683–91685 RSC;
(o) Y. Quan, Z. Qiu and Z. Xie, J. Am. Chem. Soc., 2014, 136, 7599–7602 CrossRef CAS PubMed;
(p) Z. Qiu, Y. Quan and Z. Xie, J. Am. Chem. Soc., 2013, 135, 12192–12195 CrossRef CAS PubMed.
-
(a) M. Seki, Org. Process Res. Dev., 2016, 20, 867–877 CrossRef CAS;
(b) J. Hubrich, T. Himmler, L. Rodefeld and L. Ackermann, ACS Catal., 2015, 5, 4089–4093 CrossRef CAS;
(c) D. A. Horton, G. T. Bourne and M. L. Smythe, Chem. Rev., 2003, 103, 893–930 CrossRef CAS PubMed.
- Selected reviews:
(a) S. Rej, Y. Ano and N. Chatani, Chem. Rev., 2020, 120, 1710–1787 CrossRef PubMed;
(b) P. Gandeepan, T. Muller, D. Zell, G. Cera, S. Warratz and L. Ackermann, Chem. Rev., 2019, 119, 2192–2452 CrossRef CAS PubMed;
(c) J. C. K. Chu and T. Rovis, Angew. Chem., Int. Ed., 2018, 57, 62–101 CrossRef CAS PubMed;
(d) P. Gandeepan and L. Ackermann, Chem, 2018, 4, 199–222 CrossRef CAS;
(e) Y. Park, Y. Kim and S. Chang, Chem. Rev., 2017, 117, 9247–9301 CrossRef CAS PubMed;
(f) D.-S. Kim, W.-J. Park and C.-H. Jun, Chem. Rev., 2017, 117, 8977–9015 CrossRef CAS PubMed;
(g) Z. Dong, Z. Ren, S. J. Thompson, Y. Xu and G. Dong, Chem. Rev., 2017, 117, 9333–9403 CrossRef CAS PubMed;
(h) R.-Y. Zhu, M. E. Farmer, Y.-Q. Chen and J.-Q. Yu, Angew. Chem., Int. Ed., 2016, 55, 10578–10599 CrossRef CAS PubMed;
(i) T. Gensch, M. N. Hopkinson, F. Glorius and J. Wencel-Delord, Chem. Soc. Rev., 2016, 45, 2900–2936 RSC;
(j) K. Hirano and M. Miura, Chem. Lett., 2015, 44, 868–873 CrossRef CAS;
(k) D. A. Colby, A. S. Tsai, R. G. Bergman and J. A. Ellman, Acc. Chem. Res., 2012, 45, 814–825 CrossRef CAS PubMed;
(l) T. Satoh and M. Miura, Chem.–Eur. J., 2010, 16, 11212–11222 CrossRef CAS PubMed.
-
(a) L. Ackermann, Acc. Chem. Res., 2020, 53, 84–104 CrossRef CAS PubMed;
(b) J. Loup, U. Dhawa, F. Pesciaioli, J. Wencel-Delord and L. Ackermann, Angew. Chem., Int. Ed., 2019, 58, 12803–12818 CrossRef CAS PubMed;
(c) L. Ackermann, Acc. Chem. Res., 2014, 47, 281–295 CrossRef CAS PubMed.
- J. Wencel-Delord and F. Glorius, Nat. Chem., 2013, 5, 369–375 CrossRef CAS PubMed.
- CCDC 1893305 (3aa), 1983600 (3ab), 1983612 (3dd), 1983607 (3ea), 1983615 (3fa), 1893313 (4aa) and 1983611 (4ai) contain the supplementary crystallographic data.
Footnotes |
† Electronic supplementary information (ESI) available. See DOI: 10.1039/d0sc01515f |
‡ These authors contributed equally to this work. |
|
This journal is © The Royal Society of Chemistry 2020 |
Click here to see how this site uses Cookies. View our privacy policy here.