DOI:
10.1039/D0SC00542H
(Edge Article)
Chem. Sci., 2020,
11, 3862-3867
A chiral cobalt(II) complex catalyzed enantioselective aza-Piancatelli rearrangement/Diels–Alder cascade reaction†
Received
29th January 2020
, Accepted 11th March 2020
First published on 11th March 2020
Abstract
A chiral N,N′-dioxide/cobalt(II) complex catalyzed highly diastereoselective and enantioselective tandem aza-Piancatelli rearrangement/intramolecular Diels–Alder reaction has been disclosed. Various valuable hexahydro-2a,5-epoxycyclopenta[cd]isoindoles bearing six contiguous stereocenters have been obtained in good yields with excellent diastereo- and enantio-selectivities from a wide range of both readily available 2-furylcarbinols and N-(furan-2-ylmethyl)anilines.
Introduction
The Piancatelli reaction and its variants,1 a kind of acid-catalyzed rearrangement of 2-furylcarbinols, allow straightforward access to a large array of 2-substituted cyclopentenones and other structurally complex molecules. Due to the prevalence of cyclopentane structures in major natural product families, as well as in a wealth of biologically active molecules,2 this reaction has gained great interest in the past decade.3–7 Although a range of catalytic aza-Piancatelli reactions along with related cascade processes have been developed,4–6 only a few examples are catalytic enantioselective versions, which are dominated by chiral Brønsted acid catalysts (Scheme 1a). For instance, Rueping's group and Sun's group reported the first catalytic asymmetric aza-Piancatelli reaction with aniline derivatives by using a chiral N-triflyl phosphoramide catalyst6a and chiral phosphoric acid catalyst,6b respectively. Recently, Jiang and coworkers employed chiral phosphoramide to promote an enantioselective aza-Piancatelli rearrangement/Friedel–Crafts alkylation cascade reaction.6d In these cases, the chiral Brønsted acids enabled dehydration and regioselective nucleophilic addition, and then the resulting bifunctional chiral anion ensured stereoselection in the key 4π conrotatory electrocyclization of the pentadienyl cation (Scheme 1a). Piancatelli-type reactions are mechanistic analogous to Nazarov cyclization.8 Although Lewis acids are efficient to accelerate those rearrangements and the following cascade reactions as well,5 the chiral metal complex catalyzed asymmetric version is still void. Even the well-developed enantioselective Nazarov reaction is usually limited to strong-coordinated bidentate substrates.9 The major challenge in the asymmetric Piancatelli rearrangement might come from the elusive interaction between chiral metal/ligand and cyclopentadienyl cation, which makes it hard to define the stereochemistry.6b
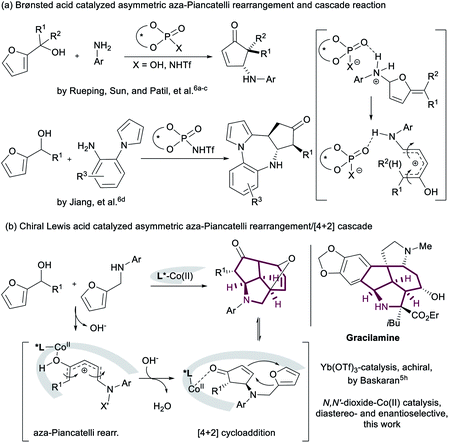 |
| Scheme 1 Asymmetric catalytic aza-Piancatelli rearrangement and cascade reaction. | |
Given the difficulties of aza-Piancatelli rearrangement and its initiated cascade reaction in the synthesis of multicyclic cores, and in connection with our long endeavor in chiral Lewis acid catalysis, we attempt to develop chiral metal complex catalysts to expand the enantioselective aza-Piancatelli-initiated cascade reactions. Chiral N,N′-dioxide–metal complexes are able to promote a number of asymmetric transformations with high efficiency and stereoselectivity,10 and are tolerant to water,11 which is generated in the dehydration step of Piancatelli rearrangement. Very recently, Baskaran disclosed an interesting Yb(OTf)3-catalyzed aza-Piancatelli rearrangement/[4+2] cycloaddition cascade to construct an octahydro-1H-cyclopenat[cd]isoindole structure, an aza-tricyclic core of a gracilamine alkaloid.5h We proposed that the well-characterized chiral pocket of N,N′-dioxide–metal complex might be able to adopt the hydrocyclopentadienyl cation from 2-furylcarbinol, delivering 3-amino substituted cyclopentenone, which undergoes a Lewis acid-promoted diastereoselective intramolecular Diels–Alder reaction (Scheme 1b). Herein, we report a new chiral N,N′-dioxide-cobalt(II) complex12 catalyzed diastereo- and enantioselective aza-Piancatelli rearrangement/Diels–Alder reaction, allowing efficient and direct construction of a number of hexahydro-2a,5-epoxycyclopenta[cd]isoindole derivatives. The metal salt and the ligand framework were found to be critical for the realization of enantiocontrol.
Results and discussion
In our preliminary investigation, we chose furan-2-yl(phenyl)methanol 1a and N-(furan-2-ylmethyl)aniline 2a as the model substrates to optimize the reaction conditions. Various metal salts, including Yb(OTf)3, Dy(OTf)3, and In(OTf)3 as well, which were proven effective for aza-Piancatelli rearrangement, were screened in toluene at 80 °C upon coordination with the chiral N,N′-dioxide ligand L3-PrPr2. Disappointingly, the corresponding product 3a was obtained as a nearly racemate with poor to moderate yields, lower reactivity and a longer reaction time than the achiral version4b,5a,c,h (Table 1, entries 1–3). We proposed that rare earth metal salts bear large ionic radii and multiple-coordination manners,13 which might be disadvantageous to create a tight pocket to confine the hydrocyclopentadienyl cation to deliver enantiocontrol in the competition of amine 2.14 The existence of water generated in the rearrangement step will accelerate the formation of a poor-enantioselective OH-bridged dimer when indium(III) salt was used.15 After examining other metal salts, we found that the reaction could be achieved with medium yield and enantioselectivity (36% yield, >19
:
1 dr, 65% ee; Table 1, entry 5) in the presence of Co(BF4)2·6H2O, where the center metal ion Co(II) is soft and bears a small ionic radius, reducing the bonding of the amine nucleophile and forming a suitable chiral pocket with the ligand to define the stereochemistry in the rearrangement of the hydrocyclopentadienyl intermediate. Encouraged by this result, the structure of N,N′-dioxide ligands was evaluated. It was revealed that the L-proline derived ligand L3-PrPr2 gives better enantioselectivity than other amino acid derived ligands (see the ESI† for details). The steric hindrance of the aniline subunits of the ligand has a dramatic influence on the enantioselectivity (Table 1, entries 5–9). Decreasing the steric hindrance, such as L3-PrEt2 containing 2,6-diethyl aniline substituent (Table 1, entry 6).
Table 1 Optimization of the reaction conditionsa
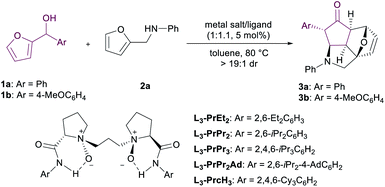
|
Entry |
Metal salt |
Ligand |
Yieldb (%) |
eec (%) |
Unless otherwise noted, the reactions were performed with metal salt/ligand (1 : 1.1, 5 mol%), 1a (0.10 mmol), and 2a (1.0 equiv.) in toluene (1.0 mL) at 80 °C for 16 h.
Isolated yield, and >19/1 dr was obtained.
Determined by HPLC analysis on a chiral stationary phase.
Co(II)/ligand (1 : 1.1, 10 mol%).
o-Xylene (1.0 mL) was used instead of toluene.
65 °C for 16 h.
1b was used instead of 1a.
|
1 |
Yb(OTf)3 |
L3-PrPr2
|
14 |
0 |
2 |
Dy(OTf)3 |
L3-PrPr2
|
<10 |
−3 |
3 |
In(OTf)3 |
L3-PrPr2
|
47 |
4 |
4 |
Co(OTf)2 |
L3-PrPr2
|
10 |
57 |
5 |
Co(BF4)2·6H2O |
L3-PrPr2
|
36 |
65 |
6 |
Co(BF4)2·6H2O |
L3-PrEt2
|
60 |
8 |
7 |
Co(BF4)2·6H2O |
L3-PrPr3
|
14 |
81 |
8 |
Co(BF4)2·6H2O |
L3-PrPr2Ad
|
42 |
82 |
9d |
Co(BF4)2·6H2O |
L3-PrcH3
|
55 |
83 |
10d,e |
Co(BF4)2·6H2O |
L3-PrcH3
|
63 |
85 |
11d,e,f |
Co(BF4)2·6H2O |
L3-PrcH3
|
20 |
92 |
12d,e,f,g |
Co(BF4)2·6H2O |
L3-PrcH3
|
88 |
90 |
Bulkier 2,4,6-substitutions, such as L3-PrcH3, bearing a 2,4,6-cyclohexyl aniline moiety, improved the yield to 55% and the ee value to 83% with 10 mol% catalyst loading (Table 1, entry 9). After careful investigation of the solvents, o-xylene was proven to be the best choice, and the desired product could be obtained in 63% yield with 85% ee (Table 1, entry 10). When the reaction temperature was reduced to 65 °C, the ee value had an obvious increase (92% ee), but the reaction activity was depressed (Table 1, entry 11). When furan-2-yl(4-methoxyphenyl)methanol 1b was used as the model substrate instead of 1a, the related product 3b could be obtained in 88% yield with 90% ee (Table 1, entry 12). The reason of dramatically increased reactivity might be that the para-methoxy group on phenyl of 2-furylcarbinol makes the cyclopentadienyl cation more stable. It is noteworthy that there is a dynamic equilibrium between the desired products 3 and the uncyclized intermediates in the solvent (see the ESI† for details).5h Finally, we established the optimized reaction conditions as Co(BF4)2·6H2O/L3-PrcH3 (1
:
1.1, 10 mol%) in o-xylene at 65 °C for 16 h.
With the optimized conditions in hand, we turned our attention to the scope of 2-furylcarbinols (Table 2). The 2-furylcarbinols containing electron-withdrawing or electron-neutral groups on the aryl moiety had lower reactivity than the standard substrate 1b in the reaction. The corresponding products 3c–3e were only achieved in medium yields with excellent ee values after the reaction time was prolonged. The phenyl ring of 2-furylcarbinols bearing electron-donating groups at the para, meta, or ortho position were converted to the desired products 3f–3i with good yields and enantioselectivities (58–79% yields and 90–93% ee). We were also pleased to find that the reaction was compatible with 2-naphthyl, 2-furyl, and 2-thienyl groups to furnish 3j–3l in 31–61% yields with 87–94% ee. Moreover, the absolute configuration of the product 3m was determined to be (2aR,2a1R,5S,5aR,7S,7aR) by X-ray crystallography analysis.16 It is noteworthy that only one diastereoisomer of the epoxycyclopenta[cd]isoindole products was isolated in all cases. The absolute configuration of the other products was determined to be uniform by comparing the circular-dichroism spectra with that of 3m.
Table 2 Substrate scope of 2-furylcarbinolsa
Unless otherwise noted, the reactions were performed with Co(BF4)2·6H2O/L3-PrcH3 (1 : 1.1, 10 mol%), 1 (0.10 mmol), and 2 (1.0 equiv.), in o-xylene (1.0 mL) at 65 °C. >19/1 dr.
|
|
Subsequently, we assessed the scope of N-(furan-2-ylmethyl)anilines in the reaction with furan-2-yl(4-methoxyphenyl)methanol 1b, and the results are summarized in Table 3. The electronic properties or steric hindrance of the substituents on the aromatic ring had a little effect on the stereoselectivity of the tandem reaction, and the desired epoxycyclopenta[cd]isoindoles 3n–3w were obtained in good to excellent yields (62–88% yields) with excellent enantioselectivities (82–95% ee). Of note, a 2-naphthyl containing amine was also a suitable substrate, giving the product 3x in 56% yield with 91% ee. Furthermore, N-((5-methylfuran-2-yl)methyl)aniline was converted to the corresponding product 3y with 90% yield and 89% ee as well.
Table 3 Substrate scope of N-(furan-2-ylmethyl)anilinesa
Unless otherwise noted, the reactions were performed with Co(BF4)2·6H2O/L3-PrcH3 (1 : 1.1, 10 mol%), 1b (0.10 mmol), and 2 (1.0 equiv.) in o-xylene (1.0 mL) at 65 °C. >19/1 dr.
|
|
To show the practicability of this methodology, a gram scale synthesis of the product 3b was carried out. In the presence of 10 mol% of Co(BF4)2·6H2O/L3-PrcH3, 2-furylcarbinol 1b (4 mmol) reacted with N-(furan-2-ylmethyl)aniline 2a (4 mmol) smoothly, and delivered 3b in 79% yield, >19
:
1 dr with 91% ee (Scheme 2a). We also carried out some product derivatization (Scheme 2b–d). For example, the compound 3b could undergo diastereoselective 1,2-addition with an allyl Grignard reagent or reduction of the carbonyl group, affording the corresponding alcohols in 91% yield with 94% ee (5) and 95% yield with 97% ee (7). Moreover, phenyloctahydro-2a,5-epoxycyclopenta[cd]isoindole 6 could be isolated in 90% yield with 95% ee through hydrogenation.
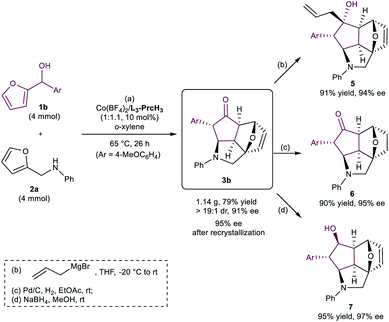 |
| Scheme 2 Gram scale experiment and product derivatization. | |
In addition, we tested other amine-type nucleophiles to probe the aza-Piancatelli rearrangement process (Scheme 3). When the substrate N-(thiophen-2-ylmethyl)aniline 2n was used, only the uncyclized product 4bn could be achieved in 95% yield with 89% ee. It indicates that the high diastereo- and enantio-selectivity come from the 4π conrotatory electrocyclization step. If amine 2o embodying a 2-furyl substituent with a longer chain was subjected to the reaction, the corresponding aza-Piancatelli rearrangement product 4bo was isolated in high yield with a medium ee value. Other secondary amines as the nucleophiles yielded the trans-substituted cyclopentenone derivatives 4bp and 4bq with moderate enantioselectivity, implying that hetero-atoms in furyl or thienyl might also participate in cooperation with the metal center to enhance the enantioselection.
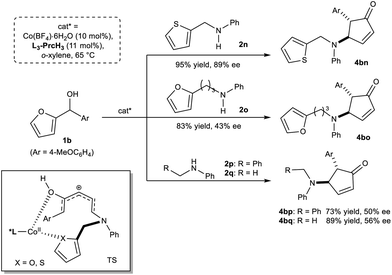 |
| Scheme 3 Other amine-type nucleophiles. | |
Conclusions
We have presented an efficient chiral Lewis acid catalyzed asymmetric aza-Piancatelli rearrangement/Diels–Alder cascade reaction of 2-furylcarbinols with N-(furan-2-ylmethyl)anilines. The use of a chiral N,N′-dioxide-Co(BF4)2·6H2O complex enabled the construction of a series of hexahydro-2a,5-epoxycyclopenta[cd]isoindoles bearing six contiguous stereocenters in a wonderful diastereo- and enantio-selective manner. Further investigations on chiral Lewis acid catalyzed other types of rearrangement and electrocyclization reactions as well as the study to probe the secret of enantiocontrol are underway.
Conflicts of interest
There are no conflicts to declare.
Acknowledgements
We thank the National Natural Science Foundation of China (U19A2014) for financial support.
Notes and references
-
(a) G. Piancatelli, A. Scettri and S. Barbadoro, Tetrahedron Lett., 1976, 17, 3555 CrossRef;
(b) G. Piancatelli, A. Scettri, G. David and M. D'auria, Tetrahedron, 1978, 34, 2775 CrossRef CAS;
(c) G. Piancatelli, M. D'auria and F. D'Onofrio, Synthesis, 1994, 867 CrossRef CAS;
(d) C. Piutti and F. Quartieri, Molecules, 2013, 18, 12290 CrossRef CAS PubMed;
(e) L. I. Palmer and J. R. de Alaniz, Synlett, 2014, 25, 8 CAS;
(f) R. F. A. Gomes, J. A. S. Coelho and C. A. M. Afonso, Chem.–Eur. J., 2018, 24, 9170 CrossRef CAS PubMed;
(g) C. Verrier, S. M. Sanchez, Y. Queneau and F. Popowycz, Org. Biomol. Chem., 2018, 16, 676 RSC;
(h) H. Wu, Q. Wang and J. P. Zhu, Eur. J. Org. Chem., 2019, 1964 CrossRef CAS.
-
(a) G. Liu, M. E. Shirley, K. N. Van, R. L. Mcfarlin and D. Romo, Nat. Chem., 2013, 5, 1049 CrossRef CAS;
(b) B. Heasley, Curr. Org. Chem., 2014, 18, 641 CrossRef CAS.
- For some selected examples of oxa-Piancatelli rearrangement:
(a) M. D'auria, Heterocycles, 2000, 52, 185 CrossRef;
(b) B. L. Yin, Y. L. Wu and J. Q. Lai, Eur. J. Org. Chem., 2009, 2695 CrossRef CAS;
(c) K. Ulbrich, P. Kreitmeier and O. Reiser, Synlett, 2010, 2037 CAS;
(d) L. I. Palmer, G. K. Veits and J. R. de Alaniz, Eur. J. Org. Chem., 2013, 6237 CrossRef CAS;
(e) L. I. Palmer and J. R. de Alaniz, Org. Lett., 2013, 15, 476 CrossRef CAS PubMed;
(f) D. Fisher, L. I. Palmer, J. E. Cook, J. E. Davis and J. R. de Alaniz, Tetrahedron, 2014, 70, 4105 CrossRef CAS;
(g) K. Michalak and J. Wicha, Tetrahedron, 2014, 70, 5073 CrossRef CAS;
(h) A. Bredihhin, S. Luiga and L. Vares, Synthesis, 2016, 48, 4181 CrossRef CAS;
(i) G. L. Goff, E. Adelin, G. Arcile and J. Ouazzani, Tetrahedron Lett., 2017, 58, 2337 CrossRef.
- For some selected examples of aza-Piancatelli rearrangement:
(a) S. W. Li and R. Batey, Chem. Commun., 2007, 3759 RSC;
(b) G. K. Veits, D. R. Wenz and J. R. de Alaniz, Angew. Chem., Int. Ed., 2010, 49, 9484 CrossRef CAS PubMed;
(c) L. I. Palmer and J. R. de Alaniz, Angew. Chem., Int. Ed., 2011, 50, 7167 CrossRef CAS PubMed;
(d) B. V. Reddy, G. Narasimhulu, P. S. Lakshumma, Y. V. Reddy and J. S. Yadav, Tetrahedron Lett., 2012, 53, 1776 CrossRef;
(e) D. R. Wenz and J. R. de Alaniz, Org. Lett., 2013, 15, 3250 CrossRef CAS PubMed;
(f) D. Leboeuf, E. Schulz and V. Gandon, Org. Lett., 2014, 16, 6464 CrossRef CAS PubMed;
(g) G. K. Veits, D. R. Wenz, L. I. Palmer, A. H. S. Amant, J. E. Hein and J. R. de Alaniz, Org. Biomol. Chem., 2015, 13, 8465 RSC;
(h) D. Leboeuf, L. Marin, B. Michelet, A. Perez-Luna, R. Guillot, E. Schulz and V. Gandon, Chem.–Eur. J., 2016, 22, 16165 CrossRef CAS PubMed;
(i) M. S. Estevão and C. A. M. Afonso, Tetrahedron Lett., 2017, 58, 302 CrossRef;
(j) Z. L. Xu, P. Xing and B. Jiang, Org. Lett., 2017, 19, 1028 CrossRef CAS PubMed;
(k) R. F. A. Gomes, N. R. Esteves, J. A. S. Coelho and C. A. M. Afonso, J. Org. Chem., 2018, 83, 7509 CrossRef CAS PubMed.
- For some examples of aza-Piancatelli-initiated cascade reactions:
(a) B. V. S. Reddy, Y. V. Reddy, P. S. Lakshumma, G. Narasimhulu, J. S. Yadav, B. Sridhar, P. P. Reddy and A. C. Kunwar, RSC Adv., 2012, 2, 10661 RSC;
(b) J. Q. Liu, Q. Shen, J. J. Yu, M. Y. Zhu, J. W. Han and L. M. Wang, Eur. J. Org. Chem., 2012, 6933 CrossRef CAS;
(c) B. V. S. Reddy, Y. V. Reddy and K. K. Singarapu, Org. Biomol. Chem., 2016, 14, 1111 RSC;
(d) L. Marin, V. Gandon, E. Schulz and D. Leboeuf, Adv. Synth. Catal., 2017, 359, 1157 CrossRef CAS;
(e) K. Nayani, R. Cinsani, A. Hussaini, P. S. Mainkar and S. Chandrasekhar, Eur. J. Org. Chem., 2017, 5671 CrossRef CAS;
(f) L. Marin, R. Guillot, V. Gandon, E. Schulz and D. Leboeuf, Org. Chem. Front., 2018, 5, 640 RSC;
(g) B. Baldé, G. Force, L. Marin, R. Guillot, E. Schulz, V. Gandon and D. Leboeuf, Org. Lett., 2018, 20, 7405 CrossRef PubMed;
(h) S. Gouse, N. R. Reddy and S. Baskaran, Org. Lett., 2019, 21, 3822 CrossRef CAS PubMed;
(i) S. D. Wang, R. Guillot, J. F. Carpentier, Y. Sarazin, C. Bour, V. Gandon and D. Leboeuf, Angew. Chem., Int. Ed., 2020, 59, 1134 CrossRef CAS PubMed.
- For some examples of asymmetric aza-Piancatelli rearrangement:
(a) Y. F. Cai, Y. R. Tang, I. Atodiresei and M. Rueping, Angew. Chem., Int. Ed., 2016, 55, 14126 CrossRef CAS PubMed;
(b) H. L. Li, R. B. Tong and J. W. Sun, Angew. Chem., Int. Ed., 2016, 55, 15125 CrossRef CAS PubMed;
(c) A. B. Gade and N. T. Patil, Synlett, 2017, 28, 1096 CrossRef CAS;
(d) Z. Wei, J. L. Zhang, H. M. Yang and G. X. Jiang, Org. Lett., 2019, 21, 2790 CrossRef CAS PubMed;
(e) G. R. Hammersley, M. F. Nichol, H. C. Steffens, J. M. Delgado, G. K. Veits and J. R. de Alaniz, Beilstein J. Org. Chem., 2019, 15, 1569 CrossRef CAS PubMed.
- For some selected examples of carbo-Piancatelli rearrangement:
(a) B. L. Yin, L. Huang, X. Y. Zhang, F. H. Ji and H. F. Jiang, J. Org. Chem., 2012, 77, 6365 CrossRef CAS PubMed;
(b) B. L. Yin, L. Huang, X. J. Wang, J. C. Liu and H. F. Jiang, Adv. Synth. Catal., 2013, 355, 370 CrossRef CAS;
(c) L. Huang, X. Y. Zhang, J. Li, K. Ding, X. H. Li, X. W. Zheng and B. L. Yin, Eur. J. Org. Chem., 2014, 338 CrossRef CAS;
(d) C. Y. Wang, C. Y. Dong, L. K. Kong, Y. L. Li and Y. Z. Li, Chem. Commun., 2014, 50, 2164 RSC;
(e) J. F. Xu, Y. Luo, H. P. Xu, Z. K. Chen, M. Z. Miao and H. J. Ren, J. Org. Chem., 2017, 82, 3561 CrossRef CAS PubMed.
-
(a) O. N. Faza, C. S. López, R. Álvarez and Á. R. de Lera, Chem.–Eur. J., 2004, 10, 4324 CrossRef PubMed;
(b) A. B. G. Pérez, P. Villar and Á. R. de Lera, Eur. J. Org. Chem., 2019, 2539 CrossRef.
- For some selected asymmetric Nazarov reactions:
(a) V. K. Aggarwal and A. J. Belfield, Org. Lett., 2003, 5, 5075 CrossRef CAS PubMed;
(b) I. Walz and A. Togni, Chem. Commun., 2008, 4315 RSC;
(c) P. Cao, C. Deng, Y. Y. Zhou, X. L. Sun, J. C. Zheng, Z. W. Xie and Y. Tang, Angew. Chem., Int. Ed., 2010, 49, 4463 CrossRef CAS PubMed;
(d) S. Raja, M. Nakajima and M. Rueping, Angew. Chem., Int. Ed., 2015, 54, 2762 CrossRef CAS PubMed;
(e) H. Y. Zhang and Z. Lu, Org. Chem. Front., 2018, 5, 1763 RSC;
(f) H. Y. Zhang and Z. Lu, Org. Lett., 2018, 20, 5709 CrossRef CAS PubMed;
(g) H. Y. Zhang, B. Cheng and Z. Lu, Org. Lett., 2018, 20, 4028 CrossRef CAS PubMed.
-
(a) X. H. Liu, L. L. Lin and X. M. Feng, Acc. Chem. Res., 2011, 44, 574 CrossRef CAS PubMed;
(b) X. H. Liu, L. L. Lin and X. M. Feng, Org. Chem. Front., 2014, 1, 298 RSC;
(c) X. H. Liu, H. F. Zheng, Y. Xia, L. L. Lin and X. M. Feng, Acc. Chem. Res., 2017, 50, 2621 CrossRef CAS PubMed;
(d) X. H. Liu, S. X. Dong, L. L. Lin and X. M. Feng, Chin. J. Chem., 2018, 36, 791 CrossRef CAS;
(e) X. M. Feng, Z. Wang and X. H. Liu, Top. Organomet. Chem., 2018, 62, 147 CrossRef CAS.
-
(a) Y. Xia, X. H. Liu, H. F. Zheng, L. L. Lin and X. M. Feng, Angew. Chem., Int. Ed., 2015, 54, 227 CrossRef CAS PubMed;
(b) Y. B. Liu, X. H. Liu, H. P. Hu, J. Guo, Y. Xia, L. L. Lin and X. M. Feng, Angew. Chem., Int. Ed., 2016, 55, 4054 CrossRef CAS PubMed;
(c) Y. Xia, L. L. Lin, F. Z. Chang, Y. T. Liao, X. H. Liu and X. M. Feng, Angew. Chem., Int. Ed., 2016, 55, 12228 CrossRef CAS PubMed;
(d) J. F. Zheng, L. L. Liu, L. Dai, X. Yuan, X. H. Liu and X. M. Feng, Chem.–Eur. J., 2016, 22, 18254 CrossRef CAS PubMed;
(e) H. Zhang, Q. Yao, L. L. Lin, C. R. Xu, X. H. Liu and X. M. Feng, Adv. Synth. Catal., 2017, 359, 3454 CrossRef CAS;
(f) L. Dai, L. L. Lin, J. F. Zheng, D. Zhang, X. H. Liu and X. M. Feng, Org. Lett., 2018, 20, 5314 CrossRef CAS PubMed;
(g) Y. L. Xu, F. Z. Chang, W. D. Cao, X. H. Liu and X. M. Feng, ACS Catal., 2018, 8, 10261 CrossRef CAS;
(h) J. X. Xu, L. F. Hu, H. P. Hu, S. L. Ge, X. H. Liu and X. M. Feng, Org. Lett., 2019, 21, 1632 CrossRef CAS PubMed.
-
(a) Z. L. Zhang, X. H. Liu, Z. Wang, X. H. Zhao, L. L. Lin and X. M. Feng, Tetrahedron Lett., 2014, 55, 3797 CrossRef CAS;
(b) Y. L. Kuang, Y. Lu, Y. Tang, X. H. Liu, L. L. Lin and X. M. Feng, Org. Lett., 2014, 16, 4244 CrossRef CAS PubMed;
(c) B. W. Ma, W. W. Luo, L. L. Lin, X. H. Liu and X. M. Feng, Chem. Commun., 2017, 53, 4077 RSC;
(d) D. Zhang, C. K. Yin, Y. H. Zhou, Y. L. Xu, L. L. Lin, X. H. Liu and X. M. Feng, Chem. Commun., 2017, 53, 7925 RSC;
(e) C. R. Xu, H. F. Zheng, B. W. Hu, X. H. Liu, L. L. Lin and X. M. Feng, Chem. Commun., 2017, 53, 9741 RSC.
- Y. Zhang, F. C. Zhang, L. Chen, J. Xu, X. H. Liu and X. M. Feng, ACS Catal., 2019, 9, 4834 CrossRef CAS.
- D. Yu, V. T. Thai, L. I. Palmer, G. K. Veits, J. E. Cook, J. R. de Alaniz and J. E. Hein, J. Org. Chem., 2013, 78, 12784 CrossRef CAS PubMed.
- Y. S. Chen, S. X. Dong, X. Xu, X. H. Liu and X. M. Feng, Angew. Chem., Int. Ed., 2018, 57, 16554 CrossRef CAS PubMed.
- CCDC 1971783 (3m) contains the ESI.†.
Footnote |
† Electronic supplementary information (ESI) available: 1H, 13C{1H} and 19F{1H} NMR, HPLC spectra, and CD spectra (PDF). X-ray crystallographic data for 3m (CIF). CCDC 1971783. For ESI and crystallographic data in CIF or other electronic format see DOI: 10.1039/d0sc00542h |
|
This journal is © The Royal Society of Chemistry 2020 |
Click here to see how this site uses Cookies. View our privacy policy here.