DOI:
10.1039/D0RA08441G
(Paper)
RSC Adv., 2020,
10, 41041-41046
The synthesis of N,N′-disulfanediyl-bis(N′-((E)-benzylidene)acetohydrazide) from (E)-N′-benzylideneacetohydrazide and S8†
Received
3rd October 2020
, Accepted 31st October 2020
First published on 11th November 2020
Abstract
Herein we report an oxidative coupling reaction for N–S/S–S bond formation from (E)-N′-benzylideneacetohydrazide and S8 to furnish substituted N,N′-disulfanediyl-bis(N′-((E)-benzylidene) acetohydrazide). It provides a direct approach for the synthesis of disulfides with good yields.
Introduction
Disulfide bonds are important structural units which were found prevalently in natural or endogenous peptides.1 They have been applied in digital light processing 3D printing,2 and as bioactive agrochemicals,3 antimicrobials,4 and synthetic intermediates.5 In addition, it is well-established that the disulfide linkage can be cleaved with the tripeptide glutathione (GSH),6 which is over-expressed in cancer cells associated with strong biomedical activities.7 Especially, a number of S,S′-bis(heterosubstituted) disulfides with N–S–S–N units exhibit a wide spectrum of biological activity (Fig. 1).8 Thus, developing an efficient and practical procedure for the synthesis of disulfides is highly desirable. Numerous strategies have been developed for the formation of disulfide bonds.9 Among these pathways, the most common approach involves the substitution of a sulfenyl derivative with a thiol or thiol derivative and these predecessor's work have been summarized by Witt (Scheme 1A).10 In recent decades, oxidative coupling of thiols has developed into an efficient approach for producing disulfides. Many oxidants such as oxygen or air,11,12 hydrogen peroxide,13 halogens,14 high-valent sulfur compounds15 and other agents16 were applied (Scheme 1B). In addition, methyl (E)-2-(2-hydroxybenzylidene)hydrazine-1-carbodithioate17 and N-phenacylbenzothiazolium bromides18 have also been used as starting materials to produce disulfides (Scheme 1C). Different from these C–S–S–C bonds, there are only a few reports in the literatures that describe N–S–S–N bond formation. In these cases, secondary amines reacted with disulfur dichloride to afford S,S′-bis(heterosubstituted) disulfides (Scheme 1D).8,19 As part of our continuing efforts into the development of the C–S bonds formation,20 herein we report an efficient method for generating N,N′-disulfanediyl bis(N′-((E)-benzylidene) acetohydrazide from (E)-N′-benzylideneacetohydrazide and S8. To the best of our knowledge, it is the first example of the formation of N–S–S–N bonds from S8 in moderate yields, and the reaction conditions are simple and mild (Scheme 1E).
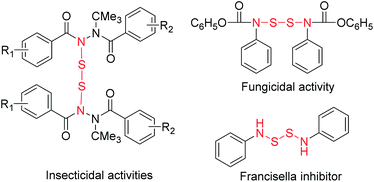 |
| Fig. 1 Selected biologically active pharmaceuticals derived from S,S′-bis(heterosubstituted) disulfides. | |
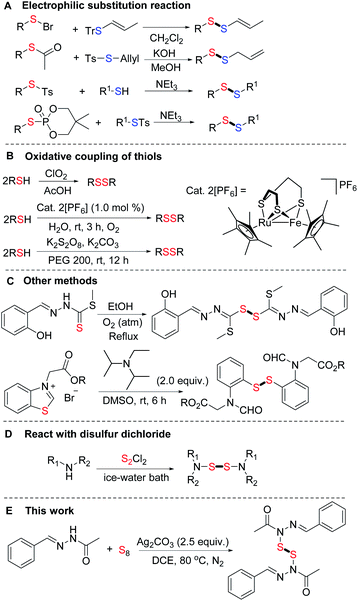 |
| Scheme 1 Strategies for the preparation of disulfides. | |
Results and discussion
As an initial experiment, we treated the model substrate (E)-N′-benzylideneacetohydrazide 1a and S8 using Ag2CO3 as the oxidant in 1,2-dichloroethane at 80 °C (Table 1). First, the screening of the loading of Ag2CO3 was carried out, and it was found that 2.5 equiv. of Ag2CO3 provided the best result (Table 1, entries 1–5). After this, we only obtained 32% yield when it added 2.5 equiv. of K2S2O8 and reduced the loading of Ag2CO3 to 0.5 equiv. (Table 1, entry 6). Meanwhile, we also evaluated the amount of S8, and the use of 0.3 equiv. of the material gave the highest yield (Table 1, entries 3, 7–9). The temperature also played an important role in the reaction. Various temperatures, such as 60, 90, 100, 110 and 120 °C were also screened, but the yields are poor (Table 1, entries 10–14). Finally, there was no obvious improvement on shortening the reaction time to 2 h or prolonging the reaction time to 4, 6, or 12 h (Table 1, entries 15–18). Impressively, control experiments revealed that the reactions performed under O2 and air atmosphere provided slightly decreased yields (Table 1, entry 19). And it has been shown that K2CO3 replaced Ag2CO3 has no reaction (Table 1, entry 20).
Table 1 Optimization of reaction conditionsa,b
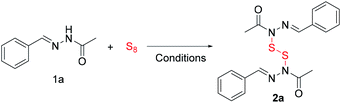
|
Entry |
Additive (equiv.) |
S8 (equiv.) |
Temp (°C) |
Time (h) |
Yieldb (%) |
Reaction conditions: 1a (0.2 mmol), S8 (0.3 mmol), Ag2CO3 (2.5 equiv.) in CH2ClCH2Cl (2.0 mL) was stirred at sealed tube, N2, 80 °C for 3 h. Isolated yields. Added K2S2O8 (2.5 equiv.). Air. O2. |
1 |
Ag2CO3 (1.0) |
S8 (0.3) |
80 |
3 |
46 |
2 |
Ag2CO3 (2.0) |
S8 (0.3) |
80 |
3 |
61 |
3 |
Ag2CO3 (2.5) |
S8 (0.3) |
80 |
3 |
71 |
4 |
Ag2CO3 (3.0) |
S8 (0.3) |
80 |
3 |
66 |
5 |
Ag2CO3 (4.0) |
S8 (0.3) |
80 |
3 |
64 |
6 |
Ag2CO3 (0.5) |
S8 (0.3) |
80 |
3 |
32c |
7 |
Ag2CO3 (2.5) |
S8 (0.2) |
80 |
3 |
42 |
8 |
Ag2CO3 (2.5) |
S8 (0.4) |
80 |
3 |
63 |
9 |
Ag2CO3 (2.5) |
S8 (0.6) |
80 |
3 |
60 |
10 |
Ag2CO3 (2.5) |
S8 (0.3) |
60 |
3 |
40 |
11 |
Ag2CO3 (2.5) |
S8 (0.3) |
90 |
3 |
49 |
12 |
Ag2CO3 (2.5) |
S8 (0.3) |
100 |
3 |
52 |
13 |
Ag2CO3 (2.5) |
S8 (0.3) |
110 |
3 |
53 |
14 |
Ag2CO3 (2.5) |
S8 (0.3) |
120 |
3 |
50 |
15 |
Ag2CO3 (2.5) |
S8 (0.3) |
80 |
2 |
41 |
16 |
Ag2CO3 (2.5) |
S8 (0.3) |
80 |
4 |
68 |
17 |
Ag2CO3 (2.5) |
S8 (0.3) |
80 |
6 |
61 |
18 |
Ag2CO3 (2.5) |
S8 (0.3) |
80 |
12 |
59 |
19 |
Ag2CO3 (2.5) |
S8 (0.3) |
80 |
3 |
46d, 48e |
20 |
K2CO3 (2.5) |
S8 (0.3) |
80 |
3 |
0 |
With the standard reaction conditions in hand (Table 1, entry 3), we subsequently investigated the scope of the benzoquinones (Table 2). Substrates derived from aromatic aldehydes with the para-substituted groups, such as 4-OMe (2b), 4-C(CH3)3 (2c), 4-C2H5 (2d), 4-Br (2e), 4-Cl (2f), afforded the corresponding products in good yields. Unfortunately, the product with an electron-withdrawing group such as 4-NO2 (2g) could not be detected. Gratifyingly, the substrates with meta-substituted groups such as electron-withdrawing 3-Br (2h) and 3-CF3 (2j) and electron-donating 3-OMe (2i) also give good yields. But, the product with a 3-NO2 group (2k) could not be observed. Subsequently, many components with the electron-donating groups such as 2-CH3 (2l) and 2-OC2H5 (2m) at the ortho position on the benzene ring gave good yields. However, the substrates with a halogen group such as 2-Br (2n) and electron-withdrawing groups such as 2-CF3 (2o), 2-NO2 (2p) at the ortho-position did not to provide the corresponding products. To our delight, many disubstituted substrates such as 2q and 2r also gave the related products in 62–65% yields. In addition, the structure of the product (2d) was confirmed by X-ray crystallography. Notably, the gram-scale synthesis was achieved under the standard conditions, giving the product 2a in 46% yield.
Table 2 Scope of various substituents on the benzene ring of the aromatic aldehyde 1a,b
Reaction conditions: 1a (0.2 mmol), S8 (0.3 mmol), Ag2CO3 (2.5 equiv.) in CH2ClCH2Cl (2.0 mL) was stirred at sealed tube, N2, 80 °C for 3 h. Isolated yields. |
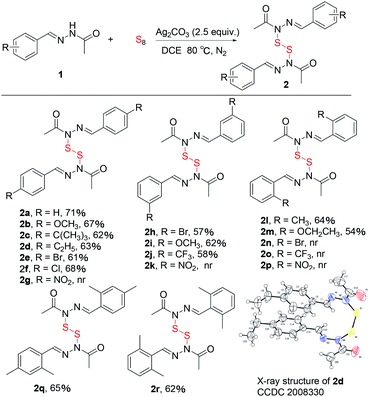 |
Various acyl hydrazides were also examined to explore the limits of the reaction. As shown in Table 3, benzohydrazide (2s), 2-methylbenzohydrazide (2t) all afforded good yields. Furthermore, the alkyl hydrazide such as formyl hydrazide (2u), valeryl hydrazide (2v) also afforded the corresponding products in 49–54% yields. Surprisingly, thiophene-2-carbohydrazide (2w) picolinohydrazide (2x) failed to produce the requisite disulfides.
Table 3 Scope of various substituents of caprylic hydrazide 1a,b
Reaction conditions: 1a (0.2 mmol), S8 (0.3 mmol), Ag2CO3 (2.5 equiv.) in CH2ClCH2Cl (2.0 mL) was stirred at sealed tube, N2, 80 °C for 3 h. Isolated yields. |
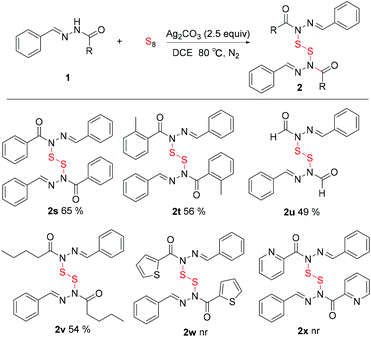 |
To understand the mechanism of the reaction, several control experiments were carried out (Scheme 2). First, product 2a was not obtained without Ag2CO3 (Scheme 2a), which indicated that Ag2CO3 was crucial to facilitate this reaction. Second, we found that the desired product 2a was only slightly decreased when the radical scavenger such as TEMPO was added to the reaction mixture (Scheme 2b). The result indictates that a free radical pathway might be ruled out in this transformation. Third, replacing (E)-N′-benzylideneacetohydrazide with (E)-N′-benzylidenebenzohydrazide produced the desired compound 2s in 65% yield under the standard conditions. Additionally, the product 4 was not observed when (E)-1-benzylidene-2-phenylhydrazine 3 was used as the substrate under the standard reaction conditions (Scheme 2c). Furthermore, when (E)-1-benzylidene-2-methylhydrazine was used in the reaction, the target product 5 was not detected (Scheme 2d). Based on these results, we knew that the acetyl group was essential for the reaction and an oxygen atom of acetyl group was also necessary. Additionally, the reactions carried out under O2 and air atmosphere have slightly decreased the yields of the product and the by-products increased (Table 1, entries 18 and 19).
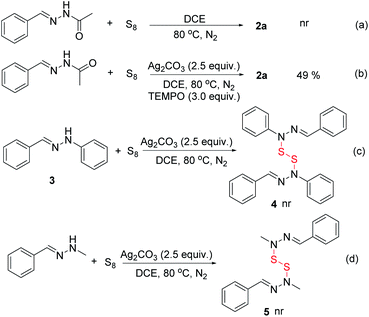 |
| Scheme 2 Mechanistic studies. | |
On basis of these preliminary studies and previous reports,21–28 a plausible pathway for the preparation of 2a from (E)-N′-benzylideneacetohydrazide is proposed in (Scheme 3). Initially, Ag2CO3 react with 1 to produce the intermediate (A). Next, A reacts with S8 to provide the intermediate B. The intermediate C is then produced through an electrophilic attack of elemental sulfur.21,22 Finally, 2a was obtained via facile oxidative coupling of the intermediate C.
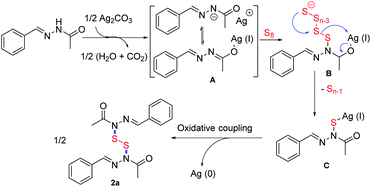 |
| Scheme 3 Postulated reaction mechanism. | |
Conclusions
In summary, we have developed a silver-mediated oxidative coupling reaction for N–S/S–S bond formation using (E)-N′-benzylideneacetohydrazide and S8 as the starting materials under neutral conditions. In these processes, N,N′-disulfanediyl bis(N′-((E)-benzylidene) acetohydrazides have been successfully synthesized. Moreover, this method provides a direct way to form the disulfides in moderate yields.
Conflicts of interest
There are no conflicts to declare.
Acknowledgements
We gratefully thank the Shandong Provincial Natural Science Foundation (ZR2017LB006) and Academic promotion program of Shandong First Medical University (no. 2019LJ003) for financial support.
Notes and references
- A. Taguchi, K. Kobayashi, Y. Cui, K. Takayama, A. Taniguchi and Y. Hayashi, Disulfide-driven cyclic peptide synthesis of human endothelin-2 with a solid-supported Npys-Cl, J. Org. Chem., 2020, 85, 1495 CrossRef CAS.
- X. P. Li, R. Yu, Y. Y. He, Y. Zhang, X. Yang, X. J. Zhao and W. Huang, Self-healing polyurethane elastomers based on a disulfide bond by digital light processing 3D printing, ACS Macro Lett., 2019, 8, 1511 CrossRef CAS.
- F. J. R. Mejías, S. M. Lopez-Haro, R. M. Varela, J. M. G. Molinillo, J. J. Calvino and F. A. Macías, In situ eco encapsulation of bioactive agrochemicals within fully organic nanotubes, ACS Appl. Mater. Interfaces, 2019, 11, 41925 CrossRef.
- Ş. D. Doğan, S. Buran, M. G. Gündüz, C. Özkul, V. S. Krishna and D. Sriramd, Synthesis of disulfide-bridged N-phenyl-N′-(alkyl/aryl/heteroaryl) urea derivatives and evaluation of their antimicrobial activities, Chem. Biodiversity, 2019, 16, e190046 Search PubMed.
-
(a) J. N. Zhao, F. Yang, Z. Y. Yu, X. F. Tang, Y. F. Wu, C. F. Ma and Q. W. Meng, Copper(I)-catalyzed sulfenylation of 1,3-dicarbonyl substrates with disulfides under mild conditions, Synlett, 2019, 30, 2295 CrossRef;
(b) J. N. Zhao, F. Yang, Z. Y. Yu, X. F. Tang, Y. F. Wu, C. F. Ma and Q. W. Meng, Visible light-mediated selective a-functionalization of 1,3-dicarbonyl compounds via disulfide induced aerobic oxidation, Chem. Commun., 2019, 55, 13008 RSC;
(c) Z. H. Shang, Q. Y. Chen, L. L. Xing, Y. L. Zhang, L. Wait and Y. F. Du, In situ formation of RSCl/ArSeCl and their oxidative coupling with enaminone derivatives under transition-metal free conditions, Adv. Synth. Catal., 2019, 361, 4926 CrossRef CAS;
(d) M. Mesgar, J. Nguyen-Le and O. Daugulis, 1,2-Bis(arylthio)arene synthesis via aryne intermediates, Chem. Commun., 2019, 55, 9467 RSC;
(e) C. C. Xia, Z. J. Wei, Y. Yang, W. B. Yu, H. X. Liao, C. Shen and P. F. Zhang, Palladium-catalyzed thioetherification of quinolone derivatives via decarboxylative C–S cross-couplings, Chem.–Asian J., 2016, 11, 360 CrossRef CAS.
-
(a) P. Kumar, B. Liu and G. A. Behl, A comprehensive outlook of synthetic strategies and applications of redox-responsive nanogels in drug delivery, Macromol. Biosci., 2019, 19, 1900071 CrossRef;
(b) B. Sui, C. Cheng and P. Xu, Pyridyl disulfide functionalized polymers as nanotherapeutic platforms, Adv. Ther., 2019, 2, 1900062 CrossRef CAS;
(c) J. F. Quinn, M. R. Whittaker and T. P. Davis, Glutathione responsive polymers and their application in drug delivery systems, Polym. Chem., 2017, 8, 97 RSC;
(d) M. H. Lee, Z. Yang, C. W. Lim, Y. H. Lee, S. Dongbang, C. Kang and J. S. Kim, Disulfide-cleavage-triggered chemosensors and their biological applications, Chem. Rev., 2013, 113, 5071 CrossRef CAS;
(e) J. K. Oh, Disassembly and tumor-targeting drug delivery of reduction-responsive degradable block copolymer nanoassemblies, Polym. Chem., 2019, 10, 1554 RSC;
(f) H. Mutlu, E. B. Ceper, X. Li, J. Yang, W. Dong, M. M. Ozmen and P. Theato, Sulfur chemistry in polymer and materials Science, Macromol. Rapid Commun., 2019, 40, 1800650 CrossRef;
(g) S. Pottanam-Chali and B. J. Ravoo, Polymer Nanocontainers for intracellular delivery, Angew. Chem., Int. Ed., 2020, 59, 2962 CrossRef CAS.
- R. Bej, P. Rajdev, R. Barmana and S. Ghosh, Hyperbranched polydisulfide, Polym. Chem., 2020, 11, 990 RSC.
-
(a) F. E. Adelowo, I. A. O. Ojo and O. S. Amuda, Synthesis and fungicidal activity of some sulphide derivatives of O-phenyl-N-substituted phenylcarbamates, Adv. Biol. Chem., 2011, 1, 122 CrossRef CAS;
(b) R. F. Sun, Y. L. Zhang, L. Chen, Y. Q. Li, Q. S. Li, H. B. Song, R. Q. Huang, F. C. Bi and Q. M. Wang, Design, synthesis and insecticidal activities of new N-benzoyl-N′-phenyl-N′-sulfenylureas, J. Agric. Food Chem., 2009, 57, 3661 CrossRef CAS;
(c) P. Ramaraju, D. Gergeres, E. Turos, S. Dickey, D. V. Lim, J. Thomas and B. Anderson, Synthesis and antimicrobial activities of structurally novel S,S′-bis(heterosubstituted) disulfides, Bioorg. Med. Chem. Lett., 2012, 22, 3623 CrossRef CAS.
-
(a) A. I. Uraev, S. E. Nefedov, K. A. Lyssenko, V. G. Vlasenko, V. N. Ikorskii, D. A. Garnovskii, N. I. Makarova, S. I. Levchenkov, I. N. Shcherbakov, M. R. Milenković and G. S. Borodkina, Synthesis, structure, spectroscopic studies and magnetic properties of Cu2N2O4-, Cu2N2O2(S2)-, Cu2N2S4-chromophores based on aminomethylene derivatives of pyrazole-5-one(thione), Polyhedron, 2020, 188, 114623 CrossRef CAS;
(b) L. Li, Q. Chen, H. H. Xu, X. H. Zhang and X. G. Zhang, DBU-promoted demethoxylative thioannulation of alkynyl oxime ethers with sulfur for the synthesis of bisisothiazole-4-yl disulfides, J. Org. Chem., 2020, 85(15), 10083 CrossRef CAS;
(c) H. X. Yue, J. H. Wang, Z. H. Xie, J. Tian, D. Y. Sang and S. P. Liu, 1,3-Diisopropylcarbodiimide-mediated synthesis of disulfides from thiols, ChemistrySelect, 2020, 5(14), 4273 CrossRef CAS;
(d) A. Parida, K. Choudhuri and P. Mal, Unsymmetrical disulfides synthesis via sulfenium ion, Chem.–Asian J., 2019, 14(15), 2579 CrossRef CAS.
- M. Musiejuk, J. Doroszuk, B. Jędrzejewski, G. O. Nieto, M. M. Navarro and D. Witt, Diastereoselective synthesis of Z-alkenyl disulfides from thiophosphorylated ketones and thiosulfonates, Adv. Synth. Catal., 2020, 362, 533 CrossRef.
-
(a) S. M. S. Chauhan, A. Kumar and K. A. Srinivas, Oxidation of thiols with molecular oxygen catalyzed by cobalt(II) phthalocyanines in ionic liquid, Chem. Commun., 2003, 18, 2348 RSC;
(b) J. A. Fernández-Salas, S. Manzini and S. P. Nolan, Efficient ruthenium-catalysed S–S, S–Si and S–B bond forming reactions, Chem. Commun., 2013, 49, 5829 RSC;
(c) M. Li and J. M. Hoover, Aerobic copper-catalyzed decarboxylative thiolation, Chem. Commun., 2016, 52, 8733 RSC;
(d) Y. Dou, X. Huang, H. Wang, L. Yang, H. Li, B. Yuan and G. Yang, Reusable cobalt-phthalocyanine in water: efficient catalytic aerobic oxidative coupling of thiols to construct S–N/S–S bonds, Green Chem., 2017, 19, 2491 RSC;
(e) W. P. Sohtun, A. Kannan, K. H. Krishna, D. Saravanan, M. S. Kumar and M. Velusamy, Synthesis, characterization, crystal structure and molecular docking studies of a S-methyldithiocarbazate derivative: bis[2-hydroxy-benzylidenehydrazono) (methylthio) methyl]disulfide, Acta Chim. Slov., 2018, 65, 621 CrossRef CAS;
(f) Y. H. Zhang, D. W. Yang, Y. Li, X. Y. Zhao, B. M. Wang and J. P. Qu, Biomimetic catalytic oxidative coupling of thiols using thiolate-bridged dinuclear metal complexes containing iron in water under mild conditions, Catal. Sci. Technol., 2019, 9, 6492 RSC.
-
(a) A. Dhakshinamoorthy, M. Alvaro and H. Garcia, Aerobic oxidation of thiols to disulfides using iron metal–organic frameworks as solid redox catalysts, Chem. Commun., 2010, 46, 6476 RSC;
(b) M. Oba, K. Tanaka, K. Nishiyama and W. Ando, Aerobic oxidation of thiols to disulfides catalyzed by diaryl tellurides under photosensitized conditions, J. Org. Chem., 2011, 76, 4173 CrossRef CAS;
(c) A. Corma, T. Ródenas and M. J. Sabater, Aerobic oxidation of thiols to disulfides by heterogeneous goldcatalysts, Chem. Sci., 2012, 3, 398 RSC;
(d) P. Das, S. Ray, A. Bhaumik, B. Banerjee and C. Mukhopadhyay, Cubic Ag2O nanoparticle incorporated mesoporous silica with large bottle-neck like mesopores for the aerobic oxidative synthesis of disulfide, RSC Adv., 2015, 5, 6323 RSC.
-
(a) A. Hatano, S. Makita and M. Kirihara, Synthesis and characterization of a DNA analogue stabilized by mercapto C-nucleoside induced disulfide bonding, Bioorg. Med. Chem. Lett., 2004, 14, 2459 CrossRef CAS;
(b) R. Dong, M. Pfeffermann, D. Skidin, F. Wang, Y. Fu, A. Narita, M. Tommasini, F. Moresco, G. Cuniberti, R. Berger, K. Müllen and X. Feng, Persulfurated coronene: a new generation of “Sulflower”, J. Am. Chem. Soc., 2017, 139, 2168 CrossRef CAS.
-
(a) R. Priefer, Y. J. Lee, F. Barrios, J. H. Wosnick, A. M. Lebuis, P. G. Farrell and D. N. Harpp, Dicubyl Disulfide, J. Am. Chem. Soc., 2002, 124, 5626 CrossRef CAS;
(b) M. H. Ali and M. McDermott, Oxidation of thiols to disulfides with molecular bromine on hydrated silica gel support, Tetrahedron Lett., 2002, 43, 6271 CrossRef CAS;
(c) E. Bourlès, R. A. Sousa, E. Galardon, M. Selkti, A. Tomas and I. Artaud, Synthesis of cyclic mono- and bis-disulfides and their selective conversion to mono- and bis-thiosulfinates, Tetrahedron, 2007, 63, 2466 CrossRef;
(d) Y. S. Izmest'ev, S. V. Pestova, O. M. Lezina, S. A. Rubtsova and A. V. Kutchin, Synthesis of novel chiral 18-sulfanyl and sulfonyl dehydroabietane derivatives, ChemistrySelect, 2019, 4, 11034 CrossRef.
-
(a) A. R. Hajipour, S. E. Mallakpour and H. Adibi, Selective and efficient oxidation of sulfides and thiols with benzyltriphenylphosphonium peroxymonosulfate in aprotic solvent, J. Org. Chem., 2002, 67, 8666 CrossRef CAS;
(b) R. Leino and J. E. Lönnqvist, A very simple method for the preparation of symmetrical disulfides, Tetrahedron Lett., 2004, 45, 8489 CrossRef CAS;
(c) A. D. Hudwekar, P. K. Verma, J. Kour, S. Balgotra and S. D. Sawant, Effect of sodium and fluorine Co-doping on the properties of fluorite-like rare-earth molybdates of Nd5Mo3O16 type, Eur. J. Org. Chem., 2019, 6, 1242 CrossRef.
-
(a) H. Firouzabadi, N. Iranpoor, H. Parham, A. Sardarian and J. Toofan, Oxidation of thiols to their disulfides with bis trinitratocerium(IV)l chromate [Ce(NO3)3]2CrO4 and pyridinum chlorochromate, Synth. Commun., 1984, 14, 717 CrossRef CAS;
(b) L. Delaude and P. Laszlo, A novel oxidizing reagent based on potassium ferrate(VI)1, J. Org. Chem., 1996, 61, 6360 CrossRef CAS;
(c) S. Patel and B. K. Mishra, Cetyltrimethylammonium dichromate: a mild oxidant for coupling amines and thiols, Tetrahedron Lett., 2004, 45, 1371 CrossRef CAS.
- W. P. Sohtun, A. Kannan, K. Hari Krishna, D. Saravanan, M. Suresh Kumar and M. Velusamy, Synthesis, characterization, crystal structure and molecular docking studies of a S-methyldithiocarbazate derivative: bis[2-hydroxy-benzylidenehydrazono) (methylthio) methyl] disulfide, Acta Chim. Slov., 2018, 65, 621 CrossRef CAS.
- S. C. Sahoo and S. C. Pan, Synthesis of N-formyl-2-benzoyl benzothiazolines, 2-substituted benzothiazoles, and symmetrical disulfides from N-phenacylbenzothiazolium bromides, Org. Lett., 2019, 21, 6208 CrossRef CAS.
-
(a) K. T. Koshy, M. D. Burdick and D. W. Knuth, Multiphase photodegradation of methyl N-[[[[[(l,l-dimethylethyl)(5,5-dimethyl-2-thioxo-l,3,2-dioxaphosphorinan-2-yl)-am-no]thio]methylamino]carbonyl]oxy]ethanimidothioate, J. Agric. Food Chem., 1983, 31, 625 CrossRef CAS;
(b) D. Raban, D. Noyd and L. Bermann, Mass spectral fragmentation of disulfenamides, Phosphorus Sulfur Relat. Elem., 1976, 1, 153 CrossRef.
-
(a) C. C. Xia, Z. J. Wei, Y. Yang, W. B. Yu, H. X. Liao, C. Shen and P. F. Zhang, Palladium-catalyzed thioetherification of quinolone derivatives via decarboxylative C–S cross-couplings, Chem.–Asian J., 2016, 11, 360 CrossRef CAS;
(b) C. . C. Xia, Z. J. Wei, C. Shen, J. Xu, Y. Yang, W. K. Su and P. F. Zhang, Palladium-catalyzed direct ortho-sulfonylation of azobenzenes with arylsulfonyl chlorides via C–H activation, RSC Adv., 2015, 5, 52588 RSC;
(c) C. C. Xia, K. Wang, J. Xu, Z. J. Wei, C. Shen, G. Y. Duan, Q. Zhu and P. F. Zhang, Copper(II)-catalyzed remote sulfonylation of aminoquinolines with sodium sulfinates via radical coupling, RSC Adv., 2016, 6, 37173 RSC;
(d) K. Wang, G. D. Wang, G. Y. Duan and C. C. Xia, Cobalt(II)-catalyzed remote C5-selective C–H sulfonylation of quinolines via insertion of sulfur dioxide, RSC Adv., 2017, 7, 51313 RSC.
- J. M. Liu, Y. Y. Zhang, Y. Y. Yue, Z. X. Wang, H. B. Shao, K. L. Zhuo, Q. Z. Lv and Z. G. Zhang, Metal-free dehydrogenative double C–H sulfuration to access thieno [2,3-b] indoles using elemental sulfur, J. Org. Chem., 2019, 84, 12946 CrossRef CAS.
- Z. W. Chen, P. Liang, F. Xu, R. L. Qiu, Q. Tan, L. P. Long and M. Ye, Lewis acid-catalyzed intermolecular annulation: three-component reaction toward imidazo [1,2-a] pyridine thiones, J. Org. Chem., 2019, 84, 9369 CrossRef CAS.
- V. Khakyzadeh, A. Rostami, H. Veisi, B. S. Shaghasemi, E. Reimhult, R. Luque, Y. Z. Xia and S. Darvishi, Direct C–S bond formation via C–O bond activation of phenols in a crossover Pd/Cu dual-metal catalysis system, Org. Biomol. Chem., 2019, 17, 4491 RSC.
- P. Q. Zhou, Y. B. Huang, W. Q. Wu, W. T. Yu, J. X. Li, Z. Z. Zhu and H. F. Jiang, Direct access to bis-S-heterocycles via copper-catalyzed three component tandem cyclization using S8 as a sulfur source, Org. Biomol. Chem., 2019, 17, 3424 RSC.
- T. B. Nguyen and P. Retailleau, DIPEA-promoted reaction of 2-nitrochalcones with elemental sulfur: an unusual approach to 2-benzoylbenzothiophenes, Org. Lett., 2017, 19, 4858 CrossRef CAS.
- T. B. Nguyen, Elemental sulfur and molecular iodine as efficient tools for carbon–nitrogen bond formation via redox reactions, Asian J. Org. Chem., 2017, 6, 477 CrossRef CAS.
- T. B. Nguyen, Recent Advances in the synthesis of heterocycles via reactions involving elemental sulfur, Adv. Synth. Catal., 2017, 359, 1106 Search PubMed.
- T. B. Nguyen, Recent Advances in the synthesis of heterocycles via reactions involving elemental sulfur, Adv. Synth. Catal., 2020, 362(17), 3448 CrossRef CAS.
Footnote |
† Electronic supplementary information (ESI) available. CCDC 2008330. For ESI and crystallographic data in CIF or other electronic format see DOI: 10.1039/d0ra08441g |
|
This journal is © The Royal Society of Chemistry 2020 |