DOI:
10.1039/D0RA07794A
(Paper)
RSC Adv., 2020,
10, 43975-43984
Spice fixed oils as a new source of γ-oryzanol: nutraceutical characterization of fixed oils from selected spices†
Received
11th September 2020
, Accepted 20th November 2020
First published on 11th December 2020
Abstract
γ-Oryzanol is an important group of nutraceuticals that play a key role in addressing metabolic disorders. This study, for the first time, examined volatile-free spice fixed oils (FOs) as an alternate plant source for γ-oryzanol and other nutraceuticals (phenolics, flavonoids, phytosterols, and tocopherols) using HPLC, HR-MS and NMR. The in vitro antioxidant activities of FOs were also analysed. The selected spices were Alpinia galanga, Cinnamomum zeylanicum, Trigonella foenum-graecum, Foeniculum vulgare and Myristica fragrans. The major polyphenols and flavonoids quantified were gallic, protocatechuic, vanillic, syringic, para-coumaric, ferulic, rutin, trans-cinnamic, and quercetin. T. foenum-graecum FOs recorded high levels of ergosterol (48.56 mg/100 g) and stigmasterol (247.36 mg/100 g). The fucosterol levels were high in A. galanga (268.31 mg/100 g) FOs, whereas C. zeylanicum FOs showed high content of β-sitosterols (7037.77 mg/100 g). C. zeylanicum and T. foenum-graecum FOs recorded high α-tocopherol content (47.55 and 15.96 mg/100 g respectively). C. zeylanicum FOs showed high levels of three ferulates, namely, cycloartenyl ferulate, 24-methylene cycloartenyl ferulate and β-sitosteryl ferulate, whose contents were 89.42, 170.23 and 50.23 mg/100 g respectively which was confirmed by HRMS with a molecular mass (m/z) of 601.45, 615.47, and 589.45 respectively. Further, γ-oryzanol ferulates in C. zeylanicum FOs were confirmed by 1H-NMR analysis. The acidified methanolic extractives of FOs showed high free radical scavenging activity and antioxidant potential. These spice FOs have excellent antioxidant activities, and are novel potential functional ingredients against lifestyle disorders.
1. Introduction
There is an emerging interest in the consumption of foods that are naturally rich in bioactive molecules due to their potential effects beneficial to human health.1 One such group of bioactive molecules that is attracting growing attention is γ-oryzanol. Rice bran (by-product) is a well-known and sole source for γ-oryzanol. γ-Oryzanol (a mixture of ferulic acid esters of triterpene alcohols and sterols) is a group of unique compounds with potent applications in nutraceutical, pharmaceutical, and cosmeceutical industries.2 An inhibitory effect of the four major components of γ-oryzanol, namely, cycloartenyl ferulate, 24-methylene cycloartenyl ferulate, campesteryl ferulate and sitosteryl ferulate, has been reported.3 γ-Oryzanol has been evaluated for its anti-obesity, anti-aging, and anti-cancer activities and also for its ability to lower blood cholesterol levels, aid in body building, provide relief from menopause symptoms and perform radical scavenging activities.4 The importance of γ-oryzanol in attenuating various metabolic disorders and its potential activities has been reviewed.5 Due to the increasing health consciousness among the human population, particularly towards high cholesterol levels, the market demand for γ-oryzanol is on rise.6 In 2018, the global γ-oryzanol market was 1.4 billion (USD), which is likely to be increased to 3.5 billion dollars (USD) by 2025 (Global market insights). Hence due to the increasing market demand, there is a need to find an alternative natural source.
Spices are the first-line natural source of bioactive and nutraceutical compounds.7 Spices are aromatic parts of the plants that have high flavour content, and are used to enhance the taste of food.8 All the spices originate from plant parts such as flowers, fruits, seeds, barks, leaves, and roots. They are considered either as food or as medicine to cure certain diseases, to maintain health, and to increase longevity.9 It has been shown that the spices contain natural bioactive and antioxidant compounds that combat chronic diseases such as cancer, diabetes, and immunological disorders.9,10
The volatile oils of spices and herbs provide the aroma/flavour to the fortified food products. Most of the spices and herbs are well known for their antimicrobial, anti-mutagenic and antioxidant properties due to the presence of nutraceuticals.8 These compounds provide various health benefits by intervening in the biological processes via nutrigenomics and immunomodulatory mechanisms.11,12 Vegetable oils are rich in nutraceutical compounds such as phenolics, flavonoids, phytosterols and tocopherols. The role of phytosterols, in particular β-sitosterol in sex hormones, cell membrane precursor and anti-obesity has been reported.4 As a result, there is a long list of spice nutraceutical compounds from crude extracts and new compounds are being added very often.10,13 The non-volatile oil of animal or plant origin (spices) is defined as fixed oil (FO). The scientific knowledge on various spice FO fatty acid profiles, antioxidant activities and nutraceutical compounds remains largely unexplored.
Alpinia galanga (Zingiberaceae) is one of the 230 species of Alpinia genus. The local names include greater galanga, lengkuas or Thai ginger. This is the most widely used medicinal rhizome that contains bioactives and volatile oils (VO). The extractives of this spice showed numerous pharmacological functions including antibacterial, antifungal, antioxidant, antidiabetic, immunomodulatory, and hypolipidemic activities.14 Cinnamomum zeylanicum (Lauraceae), commonly known as cinnamon, is one of the 300 known species. The polyphenols present in the cinnamon extracts have shown anti-inflammatory and antioxidant properties, particularly in their ability to reduce reactive oxygen species (ROS), to improve insulin sensitivity and carbohydrate metabolism.15 The reported total oil content in cinnamon bark is in the range of 0.2–1.6%.16 Trigonella foenum-graecum L. (Fabaceae), commonly known as fenugreek, is one spice among the 260 species in the family. The seeds and green leaves of the fenugreek are of medicinal importance. The digestive stimulant, antidiabetic, hypocholesterolemic, and hepatoprotective effects of fenugreek were reported.17 The total oil content (volatile oils and FOs) and fatty acid profiling of the fenugreek seeds were reported earlier.18 Foeniculum vulgare (Umbelliferae) is one of the important medicinal plants, commonly known as fennel, and used for the treatment of various diseases. The phytochemical and pharmacological properties such as hypolipidemic and antiatherogenic activities of the aqueous extract of F. vulgare were reported.19 Myristica fragrans (Myristicaceae) is commonly known as nutmeg. M. fragrans has stimulant, narcotic, carminative, astringent, aphrodisiac, hypolipidemic, antithrombotic, antiplatelet aggregation, antifungal, anti-dysenteric, and anti-inflammatory activities.10 The percentage of total oil and fatty acid composition of nutmeg was reported.20
In the present study, FOs of selected Indian spices were analysed for the presence of γ-oryzanol and other nutraceutical compounds (polyphenols, flavonoids, phytosterols, and tocopherols) that were characterized by high performance liquid chromatography (HPLC). We confirmed the presence of γ-oryzanol by nuclear magnetic resonance (NMR) and high-resolution mass spectrometry (HR-MS). The in vitro antioxidant activities of the spice FO were analysed using various in vitro assays. To the best of our knowledge, this is the first report describing the presence of γ-oryzanol in spice FOs. In addition, for the first time, we performed the confirmation, nutraceutical characterization, and estimation of antioxidant activities of these Indian spice FOs. The images of the selected Indian spices, namely, Alpinia galanga, Cinnamomum zeylanicum, Trigonella foenum-graecum, Foeniculum vulgare and Myristica fragrans are shown in Fig. 1.
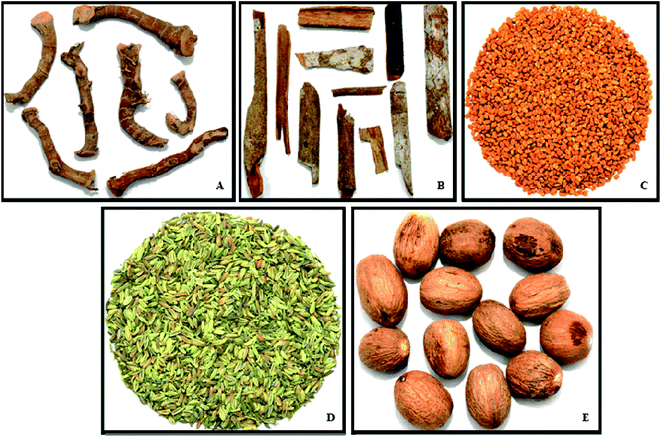 |
| Fig. 1 Images representing the authentic selected Indian spices (A). Alpinia galanga L., (greater galanga), (B). Cinnamomum zeylanicum (cinnamon), (C). Trigonella foenum-graecum L. (methi), (D). Foeniculum vulgare (fennel), and (E). Myristica fragrans (nutmeg). | |
2. Experimental
2.1. Chemicals
The chemicals used in the study such as heptadecanoic acid (C17:0), 2,2′-azino-bis(3-ethyl benzo thiazoline-6-sulphonic acid) (ABTS), 1,1-diphenyl-2-picryl hydrazyl (DPPH), 2,4,6-tripyridyl-triazine (TPTZ), and HPLC standards for polyphenols, phytosterols, tocopherols and oryzanol were purchased from Sigma-Aldrich, Bangalore, India. All the other chemicals and solvents used were of analytical grade. All the solvents used in nuclear magnetic resonance (NMR) work were purchased from Sigma Aldrich, Bangalore, India.
2.2. Source of the spice material
Five (5) selected Indian spices, namely, A. galanga, C. zeylanicum, T. foenum-graecum L., F. vulgare and M. fragrans were procured from an authentic supplier (Vriksha Vijnan Private Limited, Bangalore and Karnataka). The powder microscopy studies (Magnus MLXi-TR plus LED Trinocular microscope, Manufactured by M/S. Magnus Opto Systems India Pvt. Ltd) were performed to authenticate all the selected spices (ESI†). The spices were ground to powders using Mill-grinder (Multi Mill grinder developed by CFTRI) for 20 ± 2 min at high speed and the powders were stored in polythene air tight bags until further use.
2.3. Preparation of volatile-free spice powder and fixed oil extraction
The steam distillation process with a distillation flask maintained at 100 °C was employed until the complete removal of volatiles. The volatile-free spice powder was used for FO extraction using a Soxhlet apparatus at 68 ± 2 °C for 8 h. FAME of the selected spice FOs prepared using boron trifluoride (BF3) methanol method and GC-MS (Agilent Technologies, Milan, Italy) was reported.21
2.4. Fourier-transform infrared (FTIR) spectroscopy analysis
A High Throughput Screening eX-Tension II (Bruker, Germany) equipped with a globular mid-IR source and a DLATGS detector was used. The spice FO was loaded on optimal film thickness for FTIR transmission measurements. Spectra were recorded in the transmission mode in the spectral region from 4000 to 400 cm−1. All spectra were normalized by multiplicative signal correction (MSC) in the environment using OPUS software version 7.5.22
2.5. Separation of polyphenol and flavonoid compounds
Phenolics and flavonoids were separated from 70% aqueous methanol extractive containing 0.1% hydrochloric acid by reverse-phase HPLC (Shimadzu LC 20A; Shimadzu Corp.) with the C18 column (250 × 4.6, 5 μ, Waters Corporation, Milford, MA, USA), equipped with a UV detector at 280 and 320 nm and were quantified using standards. A binary solvent system, using water adjusted to pH 2.6 with acetic acid as solvent A and 80% acetonitrile as solvent B, was run at a flow rate of 1.2 mL min−1 for 60 min.23
2.6. Separation of phytosterols
The separation of phytosterols was performed on a LC-10A HPLC having a photodiode array detector (Shimadzu, Tokyo, Japan) connected with a Kromasil 100 C18-5 μ column (250 × 4.6 mm i.d. Supelco Inc, Bellefonte, PA, USA), at a temperature of 30 ± 0.1 °C with 30
:
70 (v/v) methanol
:
acetonitrile as the mobile phase at a flow rate of 1.2 mL min−1, and the detection wavelength was 205 nm.24
2.7. Separation of tocopherols
The composition of tocopherols in the extracted spice FO was determined by HPLC (Shimadzu Corporation, Tokyo, Japan) with an isocratic elution of the methanol/water (95:5 v/v) solvent system as the mobile phase connected with a C18 column (250 × 4.6 mm i.d.) at a flow rate of 1.5 mL min−1.25 Tocopherols were detected by fluorescence at excitation and emission wavelengths of 290 and 330 nm respectively.
2.8. Separation of γ-oryzanol
The γ-oryzanol content was determined by HPLC (Shimadzu Corporation, Tokyo, Japan) with an isocratic elution of acetonitrile
:
methanol
:
isopropanol (10
:
9
:
1, v/v) mobile phase with a C18 phenomenox column (150 × 4.6 mm) at a flow rate of 1.0 mL min−1.25
2.9. High-resolution-mass spectroscopy of oryzanol
Further confirmation of γ-oryzanol was performed using a HR-MS system (Hybrid Quadrupole-TOF AB Sciex Triple TOF 5600, Singapore). The standards and C. zeylanicum FO were directly infused with MS grade acetonitrile solvents at a flow rate of 30 μL min−1 for 3 min. An accurate mass measurement was carried out in the ESI negative mode from 50 to 800 m/z. The obtained data were compiled using the Peak View 2.1 Software (AB Sciex Triple TOF 5600, Singapore).
2.10. 1H NMR analysis for structural confirmation of oryzanol
The presence of γ-oryzanol was verified in the spice FO by NMR analysis.26 Solution-state NMR experiments were performed using a 400.23 MHz Brüker AVANCE spectrometer, equipped with a double resonance probe. The sample temperature was regulated to 298 ± 2 K. All the spectra were reference to TMS as the internal standard. The spectra were processed using the Brüker TOPSPIN 2.1 software. First, 100 mg of C. zeylanicum FO was dissolved in 0.6 mL of deuterated chloroform-CDCl3 (99.8%), and for acquiring the standard γ-oryzanol 1H-NMR spectrum, 4 mg of compound dissolved in 0.6 mL of CDCl3. The 1H spectra were acquired using a single pulse with a relaxation delay of 5 s, a spectral width of 8012 Hz and 48
076 complex data points for an acquisition time of 2.99 s. Selective 1D 1H-NOE spectra were collected using a spectral width of 8012 Hz and 48
076 complex data points for an acquisition time of 2.9 s and with mixing times of 500 ms and a recycle delay of 2 s. The 1D-DPFGSE NOE standard pulse sequence was used from Bruker library. Selective Gaussian pulses of 60 ms were used to invert the target resonance peaks to observe intra transfer of NOEs to the aromatic peak.
2.11. Estimation of in vitro antioxidant potentials
2.11.1. Sample preparation. First, 1 g spice FO samples was taken and extracted with 20 mL of 70% methanol containing 0.1% hydrochloric acid (HCl) by shaking continuously at room temperature for 1 h. The sample was centrifuged at 10
000 rpm at 10 °C for 10 min. The pellet was collected and re-extracted as described in the above procedure, and both the supernatants were pooled, filtered through a 0.45 μm filter, and stored at −20 °C until further use.27
2.11.2. Determination of total antioxidant content. A 0.3 mL spice FO extract was combined with 28 mM sodium phosphate and 4 mM ammonium molybdate, and the reaction mixture was incubated at 95 °C for 90 min. After cooling to room temperature, the absorbance of the solution was measured at 695 nm using a double beam spectrophotometer (UV-160 A, Shimadzu Corporation, Kyoto, Japan) against the blank.28 The total antioxidant activity was expressed as the number of gram equivalents of ascorbic acid (AAEq.) per 100 g of the spice FO.
2.11.3. Determination of DPPH radical scavenging activity. The DPPH free radical scavenging activity was determined, according to the method reported.29 The spice FO (1–10 mg) or standard (50–150 μL) was added to an equal volume of the DPPH reagent (0.1 mM in methanol) to a final reaction mixture of 2 mL, and vortexed vigorously. The reaction tubes were incubated in the darkness at room temperature for 20 min, and the discolouration of DPPH was measured against a reagent blank at 517 nm. The percentage inhibition (EC50) of the analysed samples was represented as ascorbic acid equivalents.
DPPH scavenging activity (EC50) = (OD control − OD sample)/OD control × 100 |
2.11.4. Determination of ferric reducing antioxidant power (FRAP). FRAP was performed in the spice FO extracts according to the method.30 This method is based on the ability of the sample to reduce Fe3+ to Fe2+ ions. Briefly, to a known quantity (200 μL) of sample extractives, 3.0 mL of working FRAP reagent was added. After incubation at room temperature for 6 min, the absorbance was measured at 593 nm against Trolox as the standard.
2.11.5. Determination of ABTS radical scavenging activity. The TEAC (Trolox Equivalent Antioxidant Capacity) assay measures the ability of a compound to eliminate or scavenge radicals against Trolox used as an antioxidant.31 The FO extractives at different concentrations (10–100 μL) were mixed with 2 mL of the diluted ABTS solution (13.2 mg potassium persulfate was mixed with 20 mL of 7.4 mM ABTS incubated in the darkness for 16 h) for 6 min, followed by the measurement of light absorbance at 734 nm. The percentage inhibition (EC50) of the analysed samples was represented as ascorbic acid equivalents.
2.12. Statistical analysis
All the results are expressed as mean ± S.D of three replicates. The EC50 values were calculated using the non-linear regression for determining DPPH and ABTS assays. The HR-MS peak integration was performed using the AB Sciex Triple TOF 5600, Peak View 2.1 Software (AB Sciex Triple TOF 5600, Singapore), and the NMR spectra were processed using the Brüker TOPSPIN 2.1 software.
3. Results and discussion
3.1. FTIR analysis
All the extracted FOs were subjected to the FTIR analysis to find the functional groups (Fig. 2). The major peak at 2922 cm−1 indicates the linear relationship between the numbers of cis-double bonds for a series of unsaturated fatty acid methyl esters. The absorption band at 1160 cm−1 in the extracted FO samples represented the saturated ester band (C–C(
O)–O). The absorbance at 2823 cm−1 indicated the stretching of the –C–H(CH2) groups. The 1743 cm−1 absorbance represented the –C
O stretching mode of lipids. The CH2 bending vibration of the acyl chains of lipids and the CH2 scissoring vibration of the acyl chains were seen at 1461 cm−1. The absorbance between 750 and 717 cm−1 indicated the presence of the overlapping methylene (–CH2) rocking vibration and the out-of-plane vibration of cis-disubstituted olefins. The FTIR analysis showed that the observed functional groups corroborate with the reported functional groups of other studied FOs.32 Recently, studies on the B. rubra seed oil functional groups have been confirmed by FTIR analysis, which were compared and correlated with the present study.22
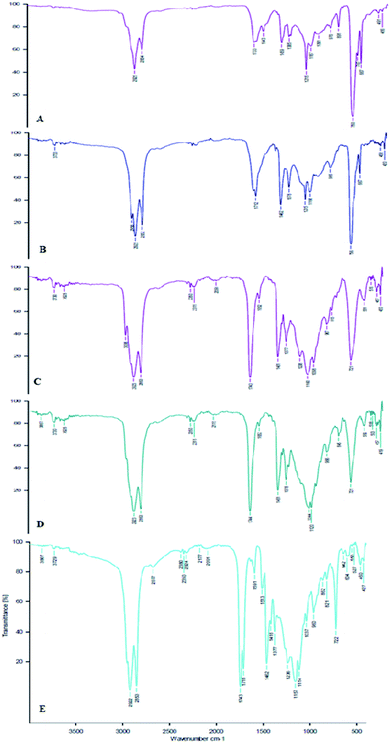 |
| Fig. 2 FTIR finger printing of the functional group representations in the selected spice FOs. (A) = A. galanga, (B) = C. zeylanicum, (C) = T. foenum-graecum, (D) = F. vulgare, and (E) = M. fragrans. | |
3.2. Separation of polyphenols and flavonoids
Phenolics are a class of nutraceutical compounds present in oils, and they provide antioxidant activities. The polyphenols have a positive correlation with the improvement of gut microbiota that plays an important role in various metabolic disorders such as cardiovascular disease, obesity and neurodegenerative disease.33,34 The content of phenolics may vary from oil to oil, and the content depends on the processing conditions employed. The identified polyphenols were gallic, protocatechuic, vanillic, syringic, para-coumaric, ferulic, rutin, trans-cinnamic, and quercetin respectively (Table 1). The A. galanga FO had 2.87 mg/100 g para-coumaric acid, C. zeylanicum FOs showed a high amount of trans-cinnamic acid (22.38 mg/100 g), whereas T. foenum-graecum FOs showed a high amount of para-coumaric acid (0.47 mg/100 g). The C. zeylanicum FO showed the presence of 14.64 mg/100 g of quercetin, and very low levels of rutin were detected in the T. foenum-graecum FO (0.36 mg/100 g), indicating maximum removal of volatiles from the spices.35 Similarly, the methanolic extracts of A. galanga and C. zeylanicum showed lipase inhibition and reduction of triglycerides, total cholesterol, low-density lipoprotein-c and an increase in high-density lipoprotein-c.36,37
Table 1 HPLC quantification profiles of phenolics, flavonoids, phytosterols, tocopherols and γ-oryzanola
|
A. galanga |
C. zeylanicum |
T. foenum-graecum |
F. vulgare |
M. fragrans |
All the values are expressed as mean ± SD of three replicates. ND = Not detected. |
Phenolics and flavonoids (mg/100 g) |
Gallic acid |
ND |
8.13 ± 0.69 |
ND |
1.65 ± 0.05 |
4.06 ± 0.36 |
Protocatechuic acid |
ND |
3.22 ± 0.03 |
ND |
ND |
ND |
Vanillic acid |
0.91 ± 0.02 |
0.56 ± 0.02 |
0.21 ± 0.01 |
ND |
ND |
Syringic acid |
ND |
ND |
ND |
ND |
0.14 ± 0.01 |
para Coumaric acid |
2.87 ± 0.04 |
0.43 ± 0.00 |
0.47 ± 0.02 |
0.10 ± 0.00 |
ND |
Ferullic acid |
ND |
0.79 ± 0.04 |
0.03 ± 0.00 |
ND |
ND |
Rutin |
ND |
ND |
0.36 ± 0.02 |
ND |
ND |
Transcinnamic acid |
ND |
22.38 ± 1.86 |
0.02 ± 0.00 |
ND |
ND |
Quercitin |
ND |
14.64 ± 1.24 |
0.02 ± 0.00 |
ND |
ND |
![[thin space (1/6-em)]](https://www.rsc.org/images/entities/char_2009.gif) |
Phytosterols (mg/100 g) |
Ergosterol |
34.55 ± 0.17 |
36.07 ± 1.16 |
48.56 ± 0.79 |
10.21 ± 0.74 |
3.58 ± 0.16 |
Fucosterol |
268.31 ± 4.09 |
191.62 ± 2.04 |
155.82 ± 11.68 |
78.20 ± 3.19 |
8.19 ± 0.22 |
Stigmasterol |
158.28 ± 3.72 |
145.86 ± 3.32 |
247.36 ± 22.48 |
110.59 ± 4.24 |
6.70 ± 0.23 |
β-Sitosterol |
5237.12 ± 23.31 |
7037.77 ± 204.08 |
4992.45 ± 160.49 |
924.90 ± 29.03 |
282.66 ± 6.72 |
Squalene |
9.83 ± 0.27 |
28.77 ± 0.53 |
6.57 ± 0.15 |
2.73 ± 0.08 |
1.20 ± 0.20 |
![[thin space (1/6-em)]](https://www.rsc.org/images/entities/char_2009.gif) |
Tocopherols (mg/100 g) |
α |
15.60 ± 0.01 |
47.55 ± 0.01 |
15.96 ± 0.85 |
1.08 ± 0.00 |
ND |
β + γ |
6.19 ± 0.00 |
2.31 ± 0.11 |
3.47 ± 0.34 |
0.70 ± 0.02 |
4.03 ± 0.67 |
Δ |
3.18 ± 0.00 |
ND |
2.12 ± 0.17 |
0.74 ± 0.01 |
2.46 ± 0.22 |
![[thin space (1/6-em)]](https://www.rsc.org/images/entities/char_2009.gif) |
Oryzanol (mg/100 g) |
Cycloartenyl ferulate |
49.68 ± 0.57 |
89.42 ± 14.44 |
3.56 ± 0.45 |
2.64 ± 0.07 |
4.07 ± 0.06 |
24-Methylene cycloartenyl ferulate |
10.88 ± 0.99 |
170.23 ± 25.35 |
0.79 ± 0.08 |
3.34 ± 0.07 |
2.90 ± 0.04 |
Campesteryl ferulate |
5.09 ± 0.36 |
ND |
3.10 ± 0.11 |
1.08 ± 0.05 |
9.73 ± 0.21 |
β-Sitosterylferulate |
6.06 ± 0.10 |
50.23 ± 3.73 |
3.74 ± 0.43 |
ND |
ND |
3.3. Separation of phytosterols
Phytosterols constitute the largest portion of the unsaponifiable matter of the oils. Phytosterols play an important role in cardiovascular diseases by the reduction of low-density lipoprotein (LDL) levels in the blood. According to the literature, phytosterols play a key role in the reduction of cholesterol since ancient days.38 The high levels of ergosterol and stigmasterol were present in T. foenum-graecum FOs (48.56 and 247.36 mg/100 g respectively), fucosterol levels were high in A. galanga (268.31 mg/100 g) FOs, whereas C. zeylanicum FOs showed a high content of β-sitosterols (7037.77 mg/100 g) (Table 1). Squalene is the intermediate component of cholesterol biosynthesis, which acts as an antioxidant in most of the oils. It is an effective singlet oxygen quencher, which prevents lipid peroxidation and protects skin from ultraviolet radiations. Among the extracted FOs, C. zeylanicum showed high squalene content (28.77 mg/100 g), whereas low squalene content (1.20 mg/100 g) was observed in M. fragrans. In general, the total phytosterol content in the vegetable oil is reported to be maximum with 600 mg/100 g of oil, which is very low when compared to that of the present spice FO with 12.35-folds increased content.39 It was shown that the daily consumption of phytosterols (2–3 g) reduces the blood LDL levels (10%) in humans.40 Recent studies have also shown that the phytosterols play a potential role in the treatment of dyslipidemic patients.41,42 Sitosterol prevents obesity-related chronic inflammation by reducing the levels of circulating interleukin 6 and tumour necrosis factor-alpha.43
3.4. Separation of tocopherols
The identification and quantification of tocopherols in the FO was performed by HPLC. A high α-tocopherol content was observed in C. zeylanicum FOs (47.55 mg/100 g) and T. foenum-graecum FOs (15.96 mg/100 g) (Table 1). A. galanga FOs were high in δ-tocopherol (3.18 mg/100 g). Compared to the present study, a high content of α and β + γ tocopherols was reported in nine varieties of T. foenum-graceum.17 The vegetable oils are the major source of tocopherols that act as excellent antioxidants and also have an ability to scavenge the free radicals.39 Tanaka et al. reported that the tocopherols have an anti-lipidemic activity, as they promote the thermogenic adipocyte differentiation in mammalian white adipose tissues by increasing the gene expression levels of PGC 1α, UCP 1, and CD 137.44
3.5. Separation of γ-oryzanol
γ-Oryzanol is a group of ferulates that are one of the important nutraceutical components present in rice bran oil, and it has several biological effects. The γ-oryzanol content varies for different rice bran sources used.45 γ-Oryzanol is shown to combat obesity, and it acts as an anti-aging and anti-dandruff agent. In the studied FO, mainly four types of ferulates, namely, cycloartenyl ferulate (C40H57O4), 24-methylene cycloartenyl ferulate (C41H59O4), campesteryl ferulate (C38H55O4), and β-sitosteryl ferulate (C39H57O4) were identified and quantified (Table 1). C. zeylanicum FOs showed high levels of three ferulate compounds, namely, cycloartenyl ferulate, 24-methylene cycloartenyl ferulate and β-sitosteryl ferulate, whose contents were 89.42, 170.23 and 50.23 mg/100 g respectively. A review on the importance of oryzanol in various metabolic disorders and its potential activity has been reported.5 Studies on the cholesterol lowering activity of oryzanol and its association with the reduction of aortic fatty streak formation were reported.46 Toorani et al. studied the antioxidant activity of oryzanol in lipid systems and different degrees of unsaturation. They showed that the greatest antioxidant activity of γ-oryzanol was observed in its TAG environment of the rice bran oil.47
3.6. High-resolution-mass spectrometry of oryzanol
The ferulates in the extracted spice FO were analysed by HR-MS (Fig. 3A and B). The total ion chromatogram (TIC) was performed in the negative mode, which showed that four base peaks corresponding to the four main compounds of standard γ-oryzanol were clearly detected with a molecular mass (m/z) of 601.45, 615.47, 575.43, and 589.45 respectively. When compared with the standards, the extracted FO showed all the ferulate compounds. The C. zeylanicum FO showed the presence of significant levels of all the major ferulates except campesteryl ferulate. Similar LC-MS/MS characterization and quantification studies of γ-oryzanol in grains of 16 Korean rice varieties were reported.48 The γ-oryzanol is employed as a dietary supplement to treat hyperlipidemia, and neurological symptoms such as menopausal syndrome and bowel syndrome. It is also shown to improve the muscle strength.49
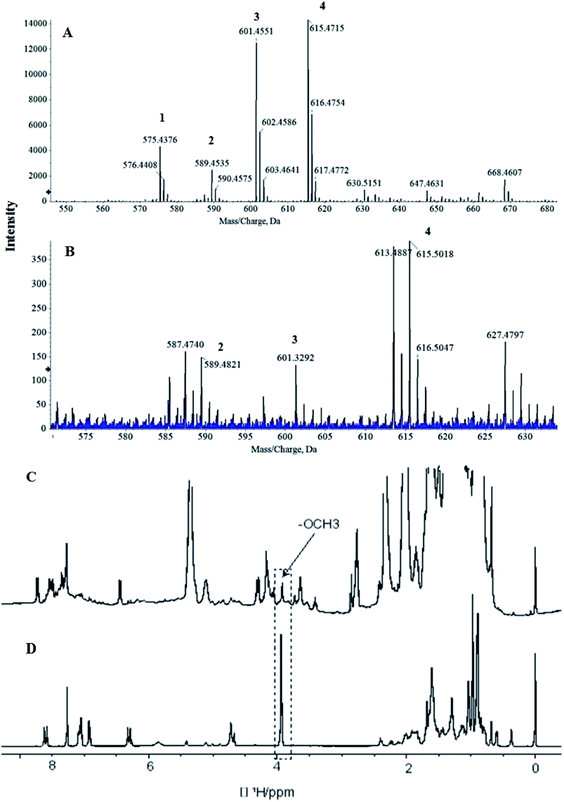 |
| Fig. 3 HRMS spectra showing the ferulates of γ-oryzanol standard (A) and C. zeylanicum fixed oil (B). 1H-NMR spectrum of C. zeylanicum fixed oil (C), and standard γ-oryzanol (D), dissolved in solvent CDCl3 and recorded at 400.23 MHz magnetic field. The –OCH3 peaks are highlighted in the dotted line box and with arrow as well. 1 = campesteryl ferulate (m/z – H+ = 575), 2 = sitosteryl ferulate (m/z – H+ = 589), 3 = cycloartenyl ferulate (m/z – H+ = 601) and 4 = 24-methylenecycloartanyl ferulate (m/z – H+ = 615). | |
3.7. 1H NMR analysis for structural confirmation of oryzanol
The presence of γ-oryzanol in C. zeylanicum FOs was confirmed by NMR analysis (Fig. 3C and D). A chemometric approach was used, where comparisons were made with the 1H-NMR spectrum of standard γ-oryzanol. The 1H-NMR spectrum of C. zeylanicum FOs showed all the 1H-NMR characteristics of γ-oryzanol, as observed in the standard. In the aliphatic region, 0 to 2 ppm showed a cycloartenol kind of pattern. Furthermore, ferulate skeleton patterns such as methoxy groups (–O–CH3), and aromatic resonances were also observed. In particular, a methoxy group (–O–CH3) can be used as the finger print region in the complex pattern that is observed in the composition of oil.26 Selective 1D-1H-NOE experiments were performed, as these are useful in the analysis of selective metabolites from a complex mixture. The overlay spectrum for standard and C. zeylanicum FOs is shown (Fig. 4). The observance of the NOE correlation between the OCH3 peak and the aromatic peak indicated that these peaks are in close proximity in a space. This can only be possible for ferulate kinds of skeleton, and hence, they provide the unambiguous assignment of the presence of γ-oryzanol (Fig. 4). The present study results were in concurrence with the study of γ-oryzanol in refined rice bran oil using methoxy groups (–O–CH3) by the NMR method.26 A recent study on the characterization of the γ-oryzanol species in rice bran oil has reported two additional compounds, cyclobranyl ferulate and cyclosadyl ferulate, in addition to the regular 24-methylenecycloartanyl ferulate.50
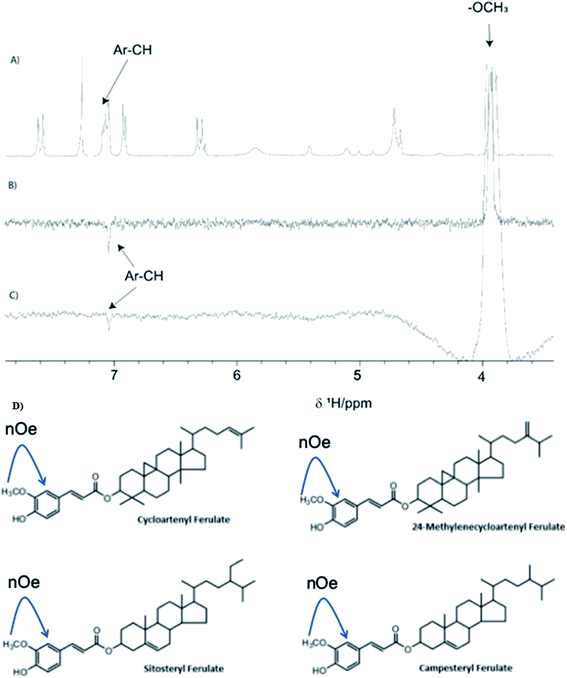 |
| Fig. 4 Stack plot of the 1D 1H-NMR spectrum of standard γ-oryzanol (A), selective 1D 1H-NOE spectrum of standard γ-oryzanol (B), and C. zeylanicum fixed oil (C) with mixing time of 500 ms. Negative NOEs can be seen with the aromatic resonances upon inversion of the OCH3 peak at 3.98 ppm. The spectra were recorded using a 400.23 MHz Bruker Avance instrument, and 2D structure of common γ-oryzanol ferulates (D). The NOE correlation was also indicated by a single-headed arrow between the –OCH3 group and the adjacent aromatic proton. | |
3.8. Estimation of in vitro antioxidant potentials
3.8.1. Total antioxidant content. The total antioxidant capacity of M. fragrans FOs was found to be the highest (0.67 g of AA eq./100 g), and that of T. foenum-graceum FOs (0.06 g of AA eq./100 g) was noted to be the least amongst the studied FOs (Table 2). The total antioxidant activity of some of the commercially available vegetable oils ranged from 34 to 130 μg of Trolox eq./100 g of oils,51 whereas the studied FO showed the total antioxidant activity ranging between 0.06 and 0.67 g of AA eq./100 g of oil. Hence, the studied spice FO showed better total antioxidant activity than vegetable oils. Similarly, the radical scavenging ability of these FOs was the highest, and the lowest activity was found in DPPH, FRAP, and ABTS assays. A similar kind of variation of radical scavenging activity with B. rubra seed oil has been reported recently.22
Table 2 In vitro antioxidant assays of the FOs extracted from the spicesa
Parameter |
A. galanga |
C. zeylanicum |
T. foenum-graecum |
F. vulgare |
M. fragrans |
All the values are expressed as mean ± SD of three replicates. |
Total antioxidant assay by phosphomolybdate method (g/100 g of oil ascorbic acid eq.) |
0.39 ± 0.01 |
0.10 ± 0.07 |
0.06 ± 0.00 |
0.10 ± 0.00 |
0.67 ± 0.01 |
DPPH (mg EC50/mL) |
3.75 ± 0.00 |
6.38 ± 0.18 |
8.75 ± 0.59 |
6.83 ± 0.21 |
2.89 ± 0.01 |
FRAP (M of trolox eq./100 g of oil) |
3.14 ± 0.13 |
2.58 ± 0.18 |
1.28 ± 0.01 |
1.11 ± 0.02 |
34.83 ± 0.63 |
ABTS (mg EC50/mL) |
10.77 ± 0.63 |
15.14 ± 0.25 |
41.42 ± 1.51 |
17.62 ± 1.45 |
0.77 ± 0.07 |
3.8.2. DPPH radical scavenging activity. The radical scavenging activity of FOs was studied by the DPPH method and expressed as the EC50 value. The lower EC50 value indicates a higher radical scavenging activity (RSA). Table 2 shows that M. fragrans FOs (2.89 mg EC50/mL) displayed better RSA than the other studied FOs. The FO showed the efficiency of RSA in the decreasing order of M. fragrans > A. galanga > C. zeylanicum > F. vulgare > T. foenum-graecum. When compared to the EC50 of refined palm oil (11.8 mg mL−1 of oil), the spice FO showed a high radical scavenging activity (2.89–8.75 mg mL−1 of oil) to combat free radicals.39
3.8.3. FRAP activity. The FOs were subjected to FRAP analysis to find out the activity towards free radical scavenging potentials (Table 2), wherein the ability of the extracted FO to reduce ferric ions can be analysed. The highest FRAP activity was observed in M. fragrans FOs (34.83 M of Trolox eq./100 g), whereas F. vulgare FOs (1.11 M of Trolox eq./100 g) showed the least FRAP activity compared with the other oils. The reported values are higher than the recently reported B. rubra seed oil extractive FRAP values of 80% methanol and 70% methanol + 0.1% hydrochloric acid (362 and 149 mM of Trolox/100 g of oil, respectively).22
3.8.4. ABTS radical scavenging activity. ABTS radical scavenging activity is one of the most important antioxidant methods widely used in food industries. The FOs were subjected to the ABTS assay to determine their efficiency. Table 2 shows that the M. fragrans FO showed highly effective 50% of inhibition at 0.77 mg mL−1 when compared with other studied oils. The T. foenum-graecum FO showed less activity (41.42 mg mL−1) of oil with 50% of inhibition. M. fragrans FOs showed the highest activity at low concentrations, whereas T. foenum-graecum FOs showed the lowest activity at high concentrations.
4. Conclusion
Spices have been consumed for a long time owing to their functional properties, especially in India and Asia. Till date, the researchers focused mostly on the extraction of essential oils from the spices and studied the health beneficial activities of the spice extractives in in vitro and in vivo systems. However, in the present study, for the first time, the presence of potent nutraceutical compounds such as γ-oryzanol, phenolics, flavonoids, phytosterols, and tocopherols was demonstrated in the residual mass of the spices after isolation of VO (essential oils). The C. zeylanicum FO showed the presence of substantial amounts of γ-oryzanol, which was confirmed by HPLC, NMR, and HRMS analysis. Similarly, the C. zeylanicum FO showed significant levels of β-sitosterol and α-tocopherol. This study presents a new and sustainable source of γ-oryzanol that is a potent nutraceutical compound for addressing lipid disorders and has a great global market demand. Further studies need to be performed for large-scale efficient extraction of γ-oryzanol from VO-free spices. This will enable the commercial availability of γ-oryzanol for food and pharmaceutical industries.
Conflicts of interest
The authors have declared that there is no conflict of interest.
Acknowledgements
VM is thankful to Indian Council of Medical Research, New Delhi for the award of SRF 3/1/2/69/2016(Nut.). The authors greatly acknowledge the Director, CSIR-CFTRI for his kind support. This work was supported by Science and Engineering Research Board (SERB)-Department of Science and Technology, New Delhi (ECR/2016/001548).
References
- C. Perez-Ternero, M. A. de Sotomayor and M. D. Herrera, J. Funct. Foods, 2017, 32, 58–71 CrossRef CAS.
- K. Srikaeo, Organic rice bran oils in health, in Wheat and Rice in Disease Prevention and Health, Academic Press, 2014, pp. 453–465 Search PubMed.
- K. Yasukawa, T. Akihisa, Y. Kimura, T. Tamura and M. Takido, Biol. Pharm. Bull., 1998, 21(10), 1072–1076 CrossRef CAS.
- M. J. Lerma-García, J. M. Herrero-Martínez, E. F. Simó-Alfonso, C. R. Mendonça and G. Ramis-Ramos, Food Chem., 2009, 115(2), 389–404 CrossRef.
- A. F. G. Cicero and A. Gaddi, Phytother. Res., 2001, 15(4), 277–289 CrossRef CAS.
- N. Rong, L. M. Ausman and R. J. Nicolosi, Lipids, 1997, 32(3), 303–309 CrossRef CAS.
- L. Diniz do Nascimento, A. A. B. D. Moraes, K. S. D. Costa, J. M. Pereira Galúcio, P. S. Taube, C. M. L. Costa and L. J. G. D. Faria, Biomolecules, 2020, 10(7), 988 CrossRef.
- D. J. Bound, P. S. Murthy, P. S. Negi and P. Srinivas, LWT--Food Sci. Technol., 2020, 122, 108987 CrossRef.
- A. K. De, and M. De, Functional and therapeutic applications of some important Spices, in The Role of Functional Food Security in Global Health, 2019, pp. 499–510 Search PubMed.
- S. Kiani, S. M. van Ruth, L. W. van Raamsdonk and S. Minaei, LWT--Food Sci. Technol., 2019, 104, 61–69 CrossRef CAS.
- M. Farràs, R. M. Valls, S. Fernández–Castillejo, M. Giralt, R. Solà and I. Subirana, J. Nutr. Biochem., 2013, 24, 1334–1339 CrossRef.
- P. Rosignoli, R. Fuccelli, R. Fabiani, M. Servili and G. Morozzi, J. Nutr. Biochem., 2013, 24, 1513–1519 CrossRef CAS.
- E. V. Arias-Rios, G. R. Acuff, A. Castillo, L. M. Lucia, S. E. Niebuhr and J. S. Dickson, LWT--Food Sci. Technol., 2019, 102, 136–141 CrossRef CAS.
- A. P. Jain, R. S. Pawar, S. Lodhi and A. K. Singhai, Pharmacogn. Commun., 2012, 2(3), 30–37 CrossRef CAS.
- J. W. Hong, G. E. Yang, Y. B. Kim, S. H. Eom, J. H. Lew and H. Kang, BMC Complementary Altern. Med., 2012, 12(1), 237 Search PubMed.
- J. S. Pruthi, Spices and condiments. National Book Trust, India, 1976 Search PubMed.
- O. N. Ciftci, R. Przybylski, M. Rudzinska and S. Acharya, J. Am. Oil Chem. Soc., 2011, 88(10), 1603–1610 CrossRef CAS.
- M. A. Al-Sebaeai, M. Alfawaz, A. K. Chauhan, A. AL-Farga and S. Fatma, Int. J. Food Sci. Nutr., 2017, 6, 52–55 Search PubMed.
- N. S. Jamwal, S. Kumar and A. C. Rana, Pharma Sci. Monit., 2013, 4(3), 327–341 Search PubMed.
- Z. Niyas, P. S. Variyar, A. S. Gholap and A. Sharma, J. Agric. Food Chem., 2003, 51, 6502–6504 CrossRef CAS.
- V. Manasa, S. R. Vaishnav and A. W. Tumaney, J. Food Sci. Technol., 2020 DOI:10.1007/s13197-020-04813-8.
- S. S. Kumar, V. Manasa, A. W. Tumaney, B. K. Bettadaiah, S. R. Chaudhari and P. Giridhar, RSC Adv., 2020, 10(53), 31863–31873 RSC.
- V. M. Burin, S. G. Arcari, A. M. T. Bordignon-Luiz and L. L. F. Costa, J. Chromatogr. Sci., 2011, 49(8), 647–651 CAS.
- D. I. Sánchez-Machado, J. López-Hernández, P. Paseiro-Losada and J. López-Cervantes, Biomed. Chromatogr., 2004, 18(3), 183–190 CrossRef.
- E. J. Rogers, S. M. Rice, R. J. Nicolosi, D. R. Carpenter, C. A. McClelland and L. J. RomanczykJr, J. Am. Oil Chem. Soc., 1993, 70(3), 301–307 CrossRef CAS.
- Y. Endo and Y. Aso, J. Nutr. Sci. Vitaminol., 2019, 65, 72–74 CrossRef.
- I. Arcan and A. Yemenicioglu, J. Food Compos. Anal., 2009, 22, 184–188 CrossRef CAS.
- P. Prieto, M. Pineda and M. Agulilar, Anal. Biochem., 1999, 269, 337–341 CrossRef CAS.
- S. S. Kumar, P. Manoj and P. Giridhar, LWT--Food Sci. Technol., 2015, 64, 468–474 CrossRef CAS.
- I. F. Benzie and J. J. Strain, Methods Enzymol., 1999, 299, 15–27 CAS.
- R. Re, N. Pellegrini, A. Proteggente, A. Pannala, M. Yang and C. Rice-Evans, Free Radic. Biol. Med., 1999, 26, 1231–1237 CrossRef CAS.
- Y. P. Timilsena, J. Vongsvivut, R. Adhikari and B. Adhikari, Food Chem., 2017, 228, 394–402 CrossRef CAS.
- P. I. Oteiza, C. G. Fraga, D. A. Mills and D. H. Taft, Mol. Aspect. Med., 2018, 61, 41–49 CrossRef CAS.
- F. A. Tomas-Barberan, M. V. Selma and J. C. Espin, Curr. Opin. Clin. Nutr. Metab. Care, 2016, 19, 471–476 CrossRef CAS.
- X. Lin, I. Zhang, A. Li, J. E. Manson, H. D. Sesso, L. Wang and S. Liu, J. Nutr., 2016, 146, 2325–2333 CrossRef CAS.
- X. N. Ma, C. L. Xie, Z. Miao, Q. Yang and X. W. Yang, RSC Adv., 2017, 7(23), 14114–14144 RSC.
- P. Nyadjeu, E. P. Nguelefack-Mbuyo, A. D. Atsamo, T. B. Nguelefack, A. B. Dongmo and A. Kamanyi, BMC Complementary Altern. Med., 2013, 13(1), 1–10 CrossRef.
- D. W. Peterson, Exp. Biol. Med., 1951, 78(1), 143–147 CrossRef CAS.
- P. K. Prasanth Kumar, V. Manasa, B. Matthaus, P. Vijayaraj, A. G. Gopala Krishna and R. Ram, Bev. Food World, 2017, 44(10), 21–27 Search PubMed.
- M. B. Katan, S. M. Grundy, P. Jones, M. Law, T. Miettinen, R. Paoletti and S. W. Participants, Mayo Clin. Proc., 2003, 78(8), 965–978 CrossRef CAS.
- J. A. B. Olivares, I. S. M. Martin, M. E. Calle, C. B. Valdes, E. P. Arruche, E. A. Delgado and L. C. Yurita, Nutr. Hosp., 2015, 32(3), 1056–1060 CAS.
- E. A. Trautwein, M. A. Vermeer, H. Hiemstra and R. T. Ras, Nutr, 2018, 10(9), e1262 Search PubMed.
- M. Kurano, K. Hasegawa, M. Kunimi, M. Hara, Y. Yatomi, T. Teramoto and K. Tsukamoto, Biochim. Biophys. Acta, Mol. Cell Biol. Lipids, 2018, 1863(2), 191–198 CrossRef CAS.
- R. Tanaka-Yachi, C. Takahashi-Muto, K. Adachi, Y. Tanimura, Y. Aoki, T. Koike and C. Kiyose, J. Oleo Sci., 2017, 66(2), 171–179 CrossRef CAS.
- A. G. Gopala Krishna, K. H. Hemakumar and S. Khatoon, J. Am. Oil Chem. Soc., 2006, 83(2), 117–120 CrossRef.
- N. Rong, L. M. Ausman and R. J. Nicolosi, Lipids, 1997, 32(3), 303–309 CrossRef CAS.
- M. R. Toorani, M. T. Golmakani and H. H. Gahruie, LWT--Food Sci. Technol., 2020, 133, 109930 CrossRef CAS.
- H. W. Kim, J. B. Kim, S. M. Cho, I. K. Cho, Q. X. Li, H. H. Jang and K. A. Hwang, Int. J. Food Sci. Nutr., 2015, 66(2), 166–174 CrossRef.
- S. H. Moon, D. Kim, N. Shimizu, T. Okada, S. Hitoe and H. Shimoda, Toxicol. Rep., 2017, 4, 9–18 CrossRef CAS.
- K. Sawada, T. Nakagami, H. Rahmania, M. Matsuki, J. Ito, T. Mohri and K. Nakagawa, Food Chem., 2020, 337, 127956 CrossRef.
- S. Dhavamani, Y. P. C. Rao and B. R. Lokesh, Food Chem., 2014, 164, 551–555 CrossRef CAS.
Footnote |
† Electronic supplementary information (ESI) available. See DOI: 10.1039/d0ra07794a |
|
This journal is © The Royal Society of Chemistry 2020 |
Click here to see how this site uses Cookies. View our privacy policy here.