DOI:
10.1039/D0RA07756A
(Paper)
RSC Adv., 2020,
10, 39700-39707
Formulation and characterization of oil-in-water nanoemulsions stabilized by crude saponins isolated from onion skin waste
Received
10th September 2020
, Accepted 15th October 2020
First published on 30th October 2020
Abstract
In the current work, a detailed study of crude saponins isolated from onion skin waste (OSW) was carried out to minimize the interfacial tension (γ) at oil–water interfaces, and to evaluate important factors on the formulation and characterization of nanoemulsions (NEs) stabilized by OSW saponins as a new natural emulsifier. It was found that OSW saponins are moderately strong surface-active agents. The droplet size of OSW saponin-stabilized NEs formulated by a high-pressure homogenizer was significantly dependent on processing parameters, where volume mean droplet diameter (d4,3) decreased as homogenization pressure and emulsifier concentration increased. The d4,3 of NEs formulated from soybean oil, sunflower oil, and medium-chain triglyceride (MCT) oil was <140 nm, which was much smaller than those prepared using orange oil (d4,3 > 800 nm). The OSW saponin-coated droplets in soybean-based NEs showed stability against thermal treatment (30–90 °C, 30 min), pH (7–9), and ionic strength (NaCl < 50 mM). The NEs exhibited instability with droplet coalescence at relatively low pH (3–6), and high ionic strength (NaCl > 50 mM). The NEs stabilized by 0.5–1% (w/w) OSW were highly stable without an increase in d4,3 during the storage (60 days) at 25 °C. Overall, these results suggest that OSW saponins are an efficient natural emulsifier, with significant potential to replace synthetic emulsifiers in certain practical applications.
1. Introduction
Emulsions, as biphasic liquid systems, are integral components of many commercially available food products, such as creams, milk, salads, sauces, and beverages.1 More recently, oil-in-water (O/W) nanoemulsions (NEs) have increasingly been investigated owing to their promising capability and efficiency for delivering water-insoluble bioactives, including several vitamins, polyunsaturated omega-3 (ω-3) oils, and various carotenoids.2–5 O/W NEs are non-equilibrium systems comprising an oil phase dispersed as small spherical particles (d < 200 nm) inside an aqueous phase.6,7 NEs commonly have higher optical clarity, better physical stability, and oral bioavailability than microemulsions (MEs) (d > 200 nm), which can be attributed to their small droplet size.7,8
Being surface-active substances (surfactants), emulsifiers play a pivotal role in the formulation and stabilization of NEs by minimizing the interfacial tension (IFT) at oil–water interfaces, as well as adsorbing rapidly to the surface of newly created droplets during homogenization.9–11 Emulsifiers also have a significant protective effect in terms of imparting greater stability to emulsions against destabilization during storage by generating strong electrostatic, or steric repulsions between the droplets.11,12 The commercial availability of many emulsifiers makes it feasible for developing a broad range of emulsion-based products in the food industry, differing in aspects such as cost, supply chain reliability, legislative status, and physicochemical properties.13,14 Each emulsifier exhibits unique characteristics that dictate the merits and demerits associated with that particular class of emulsifiers. Consequently, the selection of suitable emulsifiers is vital for developing emulsion-based products in practical applications.
Recently, the utilization of natural emulsifiers in the food industry as a replacement for synthetic emulsifiers has witnessed significant upward growth, primarily as a result of an increase in demand for emulsion-based products without food additives.15,16 Natural emulsifiers generally include polysaccharides, proteins, and phospholipids, and have a wide range of applications in the food processing industries.16 Nevertheless, the utilization of these natural emulsifiers for producing emulsion-based products for commercial applications is still facing various challenges. For instance, polysaccharides (e.g., pectin and gum arabic) are difficult to work with for forming NEs because of their relatively low surface activity.17,18 On the other hand, proteins (e.g., whey protein isolate and sodium caseinate) and phospholipids (e.g., soy lecithin) are highly surface-active and can produce emulsions containing small droplets. However, these emulsified droplets are prone to aggregation and coalescence due to various factors, such as changes in pH, exposure to high ionic strength solutions, and elevated temperatures.19,20
Saponins are secondary plant metabolites that consist of a steroid aglycone or a triterpene moiety covalently attached to one or more sugar molecules.21 The emulsifying activity of saponins can be attributed to the presence of both hydrophilic and hydrophobic groups (sugar residues and aglycone) in their molecular structures. Earlier researchers from McClements and other groups have mostly used saponins isolated from the bark of the soap bark tree (Quillaja saponaria Molina) for the preparation and stabilization of emulsions.14,19,22 Quillaja saponins are capable of producing nano-sized droplets (d < 200 nm) at relatively low emulsifier-to-oil ratios (<0.1), exhibiting broad-ranging stability against pH, ionic strength, and temperatures because of the strong electrostatic repulsive forces combined with some steric repulsion between emulsified droplets.14 More recently, some researchers have found that some other saponins from different plant sources (sugar beets and ginseng roots) also exhibit strong emulsifying properties, and could therefore be used as natural emulsifies to produce O/W emulsions.23–27 Based on these descriptions, it would be meaningful to explore some other natural saponins from different other sources, for instance, from waste materials, as new natural emulsifiers for the food industry applications.
Onion skin waste (OSW) is another saponins-rich resource. The crude saponins isolated from OSW have been shown to possess various pharmacological properties, including anti-hypertensive, anti-inflammatory, anti-oxidative, as well as anti-cancer attributes.28–32 Similar to ginseng saponins, OSW saponins also have both polar and non-polar regions in their molecules, and, therefore, are substances of significant promise from the perspective of their use as biosurfactants in the food and beverage industries. However, the basic knowledge regarding the ability of OSW saponins as natural emulsifiers to formulate stable O/W NEs is still lacking.
In the current study, the interfacial properties of OSW saponins have been characterized at an oil–water interface, followed by systematically investigating against the influence of factors, such as homogenization pressure, oil type, and emulsifier concentration, on the formulation of the NEs by using a high-pressure homogenizer (HPH). Furthermore, the impact of environmental stresses such as thermal treatment, long-term storage, ionic strength, and pH on the stability of the resulting NEs was observed.
2. Materials and methods
2.1 Materials
OSW was collected from local restaurants, later washed with normal tap water, and stored at 4 °C. Soybean oil, orange oil, and sunflower seed oils were procured from a local supermarket. Moreover, oleanolic acid standard (97%), ethanol, sodium chloride, and sodium azide were procured from Sigma-Aldrich (Sigma-Aldrich, St. Louis, MO, USA). Medium-chain triglyceride (MCT) oil was procured from the local market (brand name, Now Sports). Analytical grade chemicals were used for the present work. NEs and all other solutions were prepared by using Milli-Q water.
2.2 Preparation of extract
The OSW was finely ground and added to the mixture of distilled water and ethanol (0–100%, (v/v)), at a solvent
:
OSW ratio of 5
:
1. The suspensions were subjected to continuous agitation for 48 hours at room temperature. After 48 hours, centrifugation was performed, with subsequent filtration for removal of solid waste. The evaporation of the solvent was achieved at 40 °C and 16 hPa (WEV-1001V, Daihan Scientific, Gangwon-do, Korea). The residues were re-dissolved in Milli-Q water to prepare stock solutions of the appropriate concentration. Total saponins content was spectrophotometrically determined according to the method devised by ref. 26 and 33, with minor modifications. 0.1 mL of extract solution, 0.1 mL of a freshly prepared 5% vanillin–acetic acid solution, and 1.2 mL of 60% perchloric acid were mixed and incubated at 70 °C for 20 min. After cooling down to room temperature (25 °C), 5 mL of ethyl acetate was then added to the mixture, and the absorbance was measured at 550 nm against a blank solution as reference. Oleanolic acid (0–0.1 mL of a 2.4 mg mL−1 solution) was used as a standard.
2.3 Preparation of NEs
O/W NEs stabilized by OSW saponins were formulated by applying a two-step homogenization method. The aqueous phase was formulated by mixing 0.01–1% (w/w) of OSW in Milli-Q water. The oil phase used in the study was either sunflower oil, soybean oil, MCT oil, or orange oil. The coarse emulsion was prepared by dissolving 95% (w/w) of the aqueous phase and 5% (w/w) oil phase by using a rotor-stator homogenizer (HG-15D, Daihan Scientific, Gangwon-do, Korea) for 5 min at 5000 rpm. The coarse emulsion was then passed through a high-pressure homogenizer (AH-100B, ATS Engineering Limited, China) for 4 passes at (20–100 MPa) homogenization pressures.34
2.4 Stability evaluation of NEs
The stability of OSW saponin-coated NEs was examined by exposing the primary NEs to different environmental stresses (thermal treatment, pH, ionic strength, and long-term storage), that are typically encountered in commercial applications. In this series, the studied NEs were prepared by using 4 passes at 100 MPa pressure by using soybean oil.
2.4.1 Effect of processing parameters. To observe the influence of processing parameters (heat, pH, and ionic strength), NEs (0.5% (w/w) OSW) were treated for 30 min at varying temperatures (30–90 °C) by using water baths, with varying pH values (pH 3–9), and with different salt concentrations (0–150 mM NaCl) by diluting with the same volume of NaCl solution. Analysis of resulting samples was performed after overnight storage at 5 °C.
2.4.2 Long-term storage stability. To investigate the storage stability, NEs (0.5–1% (w/w) OSW) were stored for a duration of 60 days at room temperature, and their mean droplet size was determined during the storage time.
2.5 Analysis of droplet size and size distribution
A laser light particle size analyzer (Malvern Mastersizer 2000, Worcestershire, UK) was used to measure the mean particle size and size distribution of the formulated NEs.35 The refractive index (RI) of the aqueous phase was set at 1.333. The RI of the soybean oil, MCT oil, sunflower seed oil, and orange oil was set at 1.471, 1.447, 1.465, and 1.473, respectively. The mean droplet size of each sample was reported as volume mean diameter (d4,3): d4,3 = ∑nidi4/∑nidi3, and/or Sauter mean diameter (d3,2): d3,2 = ∑nidi3/∑nidi2. Where ni was the number of droplets i; and di was the size of the droplet. All values were taken in triplicate.
2.6 Determination of ζ-potential
The surface charge (ζ-potential) of the particles was measured using (Zetasizer Nano ZS, Malvern Instruments Ltd., Worcestershire, UK).36 The RI of the aqueous phase was set at 1.333. The RI of the soybean oil, MCT oil, sunflower seed oil, and orange oil was set at 1.471, 1.447, 1.465, and 1.473, respectively. Samples were subjected to the dilution process (100 times) using a 5 mM phosphate buffer solution. All values were taken in triplicate.
2.7 Measurement of interfacial tension
The interfacial tension of OSW at oil–water interfaces was determined by using an interfacial tensiometer (Theta Flex, Biolin Scientific) based on the pendant drop method. In this regard, soybean oil was chosen as a representative oil phase. Firstly, the water phase containing different concentrations of OSW was placed into a 1 mL glass syringe. Then, the needle was dipped into a transparent glass cell filled with soybean oil, and a drop was made until it reached its maximum volume. The interfacial tension was then automatically calculated by the instrument based on the shape of the droplet and the difference in densities between the oil and water phase. A digital density meter (DA130, KEM, Japan; accuracy: ±1.0%) with basic mode was used to measure the densities of the two phases. All the measurements were conducted 15 times at room temperature.
2.8 Statistical analysis
The obtained data were analyzed through one-way ANOVA using Statistix 8.1 software (Tallahassee, USA) and the findings were reported as means with standard deviations. LSD (least significant difference) test was applied to analyze the level of significance (p < 0.05) among various treatments.
3. Results and discussion
3.1 Saponins contents and interfacial characteristics of OSW
The total saponin contents in OSW was evaluated using the polar solvent extraction method using ethanol
:
water solution. In general, all extracts were capable of reducing the interfacial tension at both oil-type interfaces (Fig. 1a). The interfacial characteristics of emulsifiers is a crucial factor in determining their capability to formulate stable emulsions.9 An emulsifier with better emulsifying capability generally produces a greater and more rapid decline in interfacial tension at the interface. For this reason, we determined the interfacial tension at the interface of oil and water as a function of OSW concentration varying from 0.001 to 1% (w/w) (Fig. 1). The findings showed that the interfacial tension at the interface of oil–water sharply declined from 23.5 to 7.5 mN m−1 as the OSW concentration increased from 0.001 to 0.1% (w/w). These results can be elucidated by the fact that an increase in OSW concentration led to more emulsifier molecules available to adsorb onto the oil–water interfaces.37 On the other hand, the interfacial tension reached a relatively constant value of 6.25 mN m−1 with a further increase in the OSW concentration, indicating that the oil–water interfaces were saturated with adsorbed emulsifier molecules.38 Furthermore, critical micelle concentration (CMC) of OSW was calculated by the methods described by ref. 39 and 40, and the CMC value was calculated to be around 0.01% (w/w).
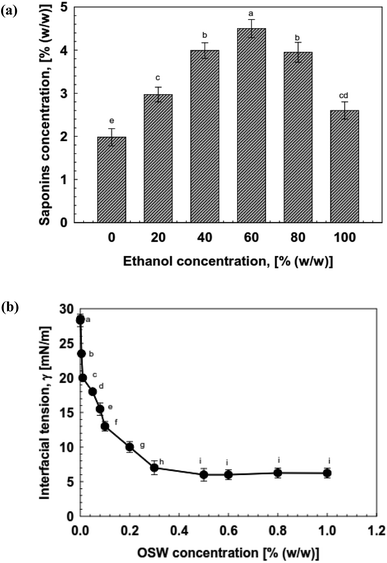 |
| Fig. 1 Evaluation of total saponins in OSW (a) saponins contents with increasing concentration of ethanol : water polarity and (b) effect of OSW concentration on the reduction in interfacial tension at soybean oil–water interfaces. Different symbols present significant different at 95% probability level. | |
3.2 Effect of OSW concentration on NE formulation
In this section, we investigated the effect of OSW concentration (0.01–1% (w/w)) on the formulation of soybean oil-based NEs (Fig. 2). Initially, the increase in OSW concentration from 0.01 to 0.5% (w/w) resulted in a sharp decrease in mean droplet size of NEs, with d4,3 decreasing from 1000 nm to 120 nm, and d3,2 decreasing from 600 nm to 100 nm, respectively (Fig. 2a). This observed behavior could be ascribed to two main mechanisms: (i) higher OSW concentration means greater droplet surface area could be covered by emulsifier molecules;41 (ii) the emulsifier molecules cover the droplet surface more rapidly at higher emulsifier concentrations, which in turn leads to a reduction in droplet re-coalescence during homogenization.11 On the other hand, both d4,3 and d3,2 of emulsified droplets did not significantly (p > 0.05) change further with increasing OSW concentration (Fig. 2a). The observed constant size at high emulsifier concentration could be associated with the fact that minimum droplet size was governed by the disruptive energy produced by the high-pressure homogenizers.11,42 The size distribution measurements also confirmed that higher emulsifier concentration led to small and narrow droplets in the final NEs (Fig. 2b).
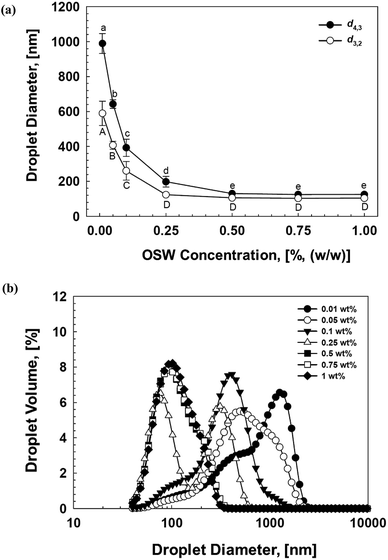 |
| Fig. 2 Effect of OSW concentration on (a) the mean droplet diameter (d4,3 and d3,2), and (b) the droplet size distributions of soybean oil-based NEs prepared by using 4 passes at 100 MPa pressure. Different lowercase letters in the d4,3 of NEs indicate significant differences (p < 0.05). Different uppercase letters in the d3,2 of NEs indicate significant differences (p < 0.05). | |
3.3 Effect of oil type on NE formulation
Different oils can be applied to prepare emulsion-based beverages and foods in commercial applications. For the formulation of stable emulsion-based delivery systems, an important factor is the selection of an appropriate oil phase.43 Therefore, the role of oil type on the formulation of NEs stabilized by 0.5% (w/w) OSW saponins (Fig. 3) was investigated. The NEs were prepared using either of the four oils, orange oil, MCT oil, sunflower seed oil, and soybean oil. A non-significant (p > 0.05) difference in mean droplet size of NEs prepared from soybean oil (d4,3 ≈ 120 nm), sunflower seed oil (d4,3 ≈ 130 nm), and MCT oil (d4,3 ≈ 145 nm) (Fig. 3a) was observed. In contrast, the emulsions produced from orange oil exhibited different behaviors, with their mean droplet size (d4,3 ≈ 800 nm) being much larger compared to the emulsions from three other oils. The findings revealed that the droplet size distribution of orange oil-based emulsions was much larger and wider than those produced from soybean oil, sunflower oil, and MCT oil (Fig. 3b). Similar findings were also reported in the previous investigations.39,44,45 Orange oil, a polar flavor oil, has a relatively high water-solubility which has been linked to high susceptibility to Ostwald ripening in emulsions produced from orange oil and similar flavor-oil-based emulsions, thereby leading to rapid droplet growth and large droplet size.46
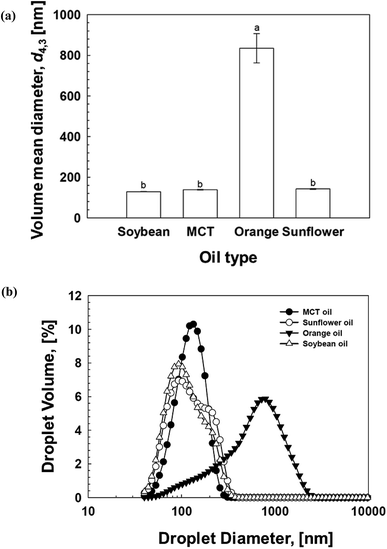 |
| Fig. 3 Effect of oil type on the (a) the mean droplet diameter (d4,3), and (b) the droplet size distributions of soybean oil-based NEs (0.5% (w/w) OSW) prepared by using 4 passes at 100 MPa pressure. | |
3.4 Effect of homogenization pressure on NE formulation
Different researchers have reported that homogenization conditions such as the number of cycles and pressure have a significant effect on the formulation and stabilization of NEs.47–49 Therefore, we determined the impact of homogenization pressure on the droplet characteristics of soybean oil-based NEs (Fig. 4). Overall, it was observed that the mean droplet size of the NEs reduced, with d4,3 decreasing from 450 nm to 120 nm as the homogenization pressure was increased from 20 to 100 MPa (Fig. 4a). The examination of droplet size distribution also suggested that the droplets in NEs became smaller and narrowly distributed with increasing operating pressure (Fig. 4b). These observed behaviors were due to the fact that higher pressure generates stronger disruptive energy, which leads to smaller droplets.47 Overall, these outcomes demonstrate that OSW saponins are effective emulsifiers for the formulation of NEs.
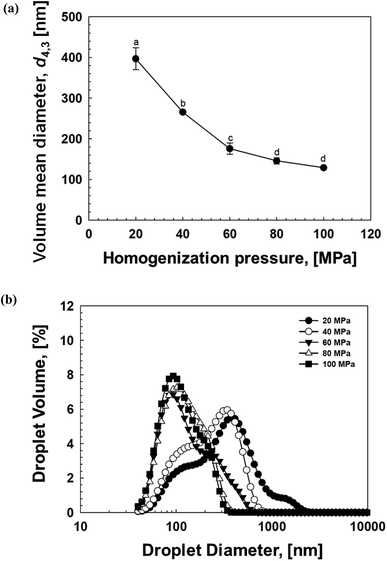 |
| Fig. 4 Effect of homogenization pressure on (a) the mean droplet diameter (d4,3), and (b) the droplet size distributions of soybean oil-based NEs containing 0.5% (w/w) OSW (4 passes at different pressures). | |
3.5 Evaluation of NE stability
The stability of NE-based beverages and foods is very important for effective industrial applications in terms of processing conditions, storage duration, transportation, and utilization. They often encounter different pH conditions, mineral concentrations, heat processing (variable temperatures), which may affect both their stability, as well as composition. Thus, we examined the effect of environmental conditions (temperature, ionic strength, pH, and storage periods) on the stability of soybean oil-based NEs prepared using OSW saponins as natural emulsifiers.
3.5.1 Effect of thermal treatment on the stability. To observe the stability of 0.5% (w/w) OSW saponins-stabilized NEs under thermal treatment, emulsions were treated at temperatures of 30–90 °C for 30 min. The measurements of mean droplet size and ζ-potential were taken after overnight incubation at 5 °C (Fig. 5). All NEs remained stable during the thermal treatment over the selected temperature range, as demonstrated by non-significant (p > 0.05) change in their d4,3, with no evidence of visible instability, such as creaming, and phase separation detected (Fig. 5a). OSW saponins have relatively small sugar groups (hydrophilic heads), that have the tendency to form thin protective layers. Therefore, the steric repulsion produced by OSW saponins might be insufficient (relative to the van der Waals attraction forces) for preventing the droplets from aggregation under harsh conditions, for instance, elevated temperatures. The ζ-potential measurements showed that OSW saponins-coated droplets were highly negative charged across the temperature range of 30–90 °C (Fig. 5b), suggesting the electrostatic repulsion mechanism has a significant role in the stability of resulting NEs. It has been reported that in saponin-based emulsifiers, the presence of carboxylic groups is responsible for the strong repulsive forces between droplets.14,16 Similarly, OSW might also contain some saponins with the presence of carboxylic groups,50 which could impart OSW-coated droplets with good thermal stability observed in our study.
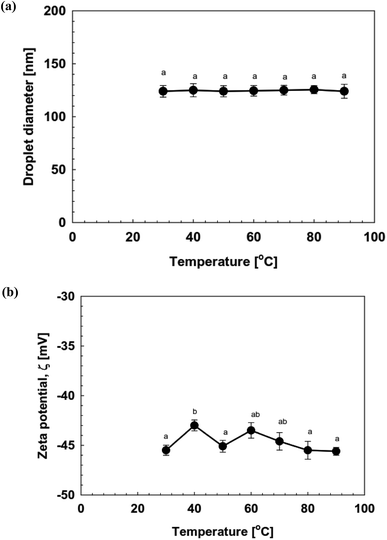 |
| Fig. 5 Effect of thermal processing (30–90 °C, 30 min) on (a) the mean droplet diameter (d4,3), and (b) the ζ-potential of soybean oil-based NEs (0.5 wt% OSW) prepared by using 4 passes at 100 MPa pressure. | |
3.5.2 Effect of pH on the stability. The results indicating the impact of pH on the ζ-potential, mean droplet size, and physical stability of NEs produced by 0.5% (w/w) OSW were presented in Fig. 6. In general, a significant impact has been observed on the stability of NEs due to pH change. Between the pH range of 7–9, there was no apparent change in the d4,3, and no visible creaming and phase separation were observed, indicating they were highly stable (Fig. 6a). Between pH 4–6, although no phase separation was observed, the NEs showed signs of instability, due to a significant (p < 0.05) increase in d4,3 (Fig. 6a). At pH 3, the oil layer was observed on the surface which made the NEs highly unstable, suggesting extensive droplet coalescence had occurred (Fig. 6a).
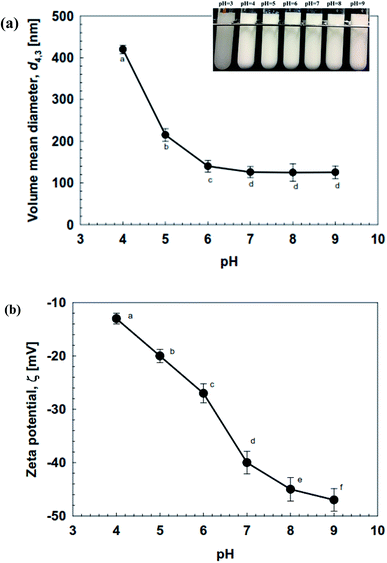 |
| Fig. 6 Effect of pH (3–9) on (a) the mean droplet diameter (d4,3), and (b) the ζ-potential of soybean oil-based NEs (0.5% (w/w) OSW) prepared by using 4 passes at 100 MPa pressure. | |
The ζ-potential on the OSW saponin-coated droplets was determined at different pH values, in order to provide an insight into the pH-instability of NEs. As presented in Fig. 6b, the ζ-potential of OSW-stabilized NEs switched from strongly negative (−43 mV) at pH 9 to weakly negative (−15 mV) at pH 4. A previous study has indicated that the pKa of the carboxylic acid group on the saponin-based emulsifiers was around pH 3.25.16 The carboxylic groups possess a negative charge (–COO−) at high pH values (pH ≫ pKa), which improves the stability of OSW-coated droplets. At pH close to pKa, the carboxylic acid groups would lose the negative charge, which is due to their protonation effects (–COOH). Consequently, the electrostatic repulsive forces between OSW-coated droplets would not be strong enough to maintain the stability of NEs.
3.5.3 Effect of ionic strength on the stability. The influence of NaCl on the physical stability of 0.5% (w/w) OSW saponin-stabilized NEs was determined based on d4,3 and ζ-potential (Fig. 7). The NaCl concentration in NEs exhibited a significant effect on the stability of OSW saponin-coated droplets. At an NaCl concentration of <50 mM, NEs were stable with no evidence of droplet coalescence, as there was non-significant (p > 0.05) increase in d4,3 (Fig. 7a). However, at higher NaCl concentrations (>100 mM), there was an appreciable increase in d4,3, thereby decreasing the stability of emulsions with evidence of excessive oiling off (Fig. 7a). These results suggested that OSW saponin-stabilized NEs were sensitive to droplet coalescence owing to the presence of NaCl even at low levels. As mentioned in Section 3.5.1, electrostatic repulsive forces were the main reason for the stabilization of OSW saponin-coated droplets. The electrostatic repulsion between negatively charged droplets could be reduced by adding counter-ions in the system (due to electrostatic screening effects).51 Thus, the observed salt-induced instability of OSW saponin-coated droplets might be due to the weakened electrostatic repulsion at high NaCl concentrations. This effect was also substantiated by the measured values of the electrical charge of the resultant samples, indicating that with the increase in the NaCl concentration in the aqueous phase, the magnitude of the ζ-potential decreased (Fig. 7b). Also, the presence of elevated salt levels might change the optimum curvature of the emulsifier layer, in turn, inducing the droplets to be more easily broken up.51
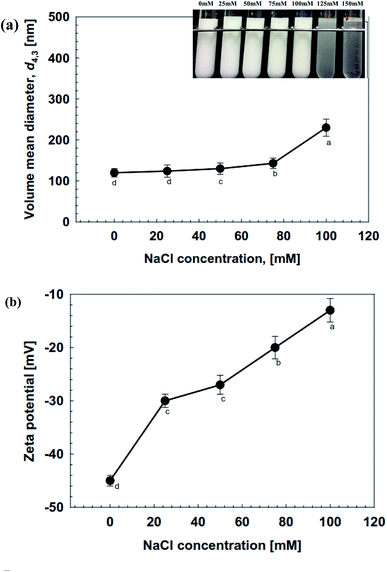 |
| Fig. 7 Effect of ionic strength (0–150 mM NaCl) on (a) the mean droplet diameter (d4,3), and (b) the ζ-potential of soybean oil-based NEs (0.5 wt% OSW) prepared by using 4 passes at 100 MPa pressure. | |
3.5.4 Effect of long-term storage on the stability. The storage stability of the NEs is a particularly important parameter for understanding the shelf life and potential applications of the emulsified products. Therefore, the stability of soybean oil-based NEs were investigated during the storage period of 60 days. The variation in d4,3 of 0.5–1% (w/w) OSW saponin-stabilized NEs during storage was presented in Fig. 8. All of the NEs exhibited a non-significant change in their d4,3 after 60 days of storage. These findings depicted that the NEs stabilized by OSW saponins have excellent storage stability. Several reasons accounted for the good storage stability observed. First and foremost, the NEs had nano-sized droplets, which could avert the creaming instability, as the Brownian motion effect could domain and overcome the gravitational separation force. Secondly, the NEs were produced using soybean oil, which is highly water-insoluble, comprising of long-chain triglycerides. It has been reported that NEs with higher water-insoluble droplets are more stable towards the destabilizing effect of Ostwald ripening.52 The good storage stability can also be attributed to the ability of the strong electrostatic repulsion contributed by OSW saponins for protection against droplet coalescence.
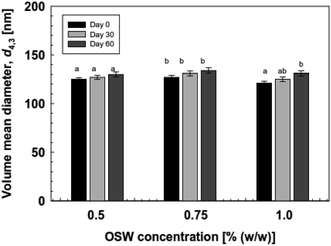 |
| Fig. 8 Effect of storage time on the mean droplet diameter of soybean oil-based NEs (0.5–1 wt% OSW) prepared by using 4 passes at 100 MPa pressure. Different letters indicate significant differences (p < 0.05) between the NEs stabilized by the same emulsifier concentration. | |
4. Conclusion
In this study, a new natural emulsifier based on OSW saponins has been used to formulate O/W NEs by a high-pressure homogenizer. OSW saponins demonstrate high surface activity that could strongly minimize the interfacial tension at soybean oil–water interfaces. OSW saponins can produce nano-droplets with d4,3 < 150 nm, which was strongly reliant on the emulsifier concentration, oil type, and homogenization pressure. Environmental stresses significantly influenced the stability of the resultant OSW saponin-coated droplets. The NEs had poor stability at acidic pH, and against high ionic strength, which might be ascribed to the decrease in electrostatic repulsion between OSW saponin-coated droplets. Therefore, further investigations are required for assessment of the protection ability of oil droplets against processing conditions and oxidation. There is significant potential in terms of the use of saponins for the increase of the chemical stability of NEs. To gain a better knowledge of how special compounds in OSW affect the emulsifying capability and stability of the NEs, the chemical compositions and molecular structures in OSW should be further analyzed.
The result of this work called attention to the requirement for additional investigations concerning the formulation of NEs from natural emulsifiers. Further investigations are as yet needed to set up the premise of novel formulations, in which it is necessary to describe systematically and contrast the ability to form and stabilize emulsions.
Conflicts of interest
The authors declare no conflict of interest in writing this paper.
Acknowledgements
This work is funded by Deanship of Scientific Research – Imam Abdulrahman Bin Faisal University, Dammam Saudi Arabia via project no. CPH-2019-364.
References
- D. J. McClements, Food Emulsions: Principles, Practices, and Techniques, CRC Press, 2015 Search PubMed.
- K. E. Lane, W. Li, C. J. Smith and E. J. Derbyshire, J. Funct. Foods, 2016, 23, 306–314 CrossRef CAS.
- C. Qian, E. A. Decker, H. Xiao and D. J. McClements, Food Chem., 2012, 132, 1221–1229 CrossRef CAS.
- G. Shu, N. Khalid, Y. Zhao, M. A. Neves, I. Kobayashi and M. Nakajima, Food Res. Int., 2016, 90, 320–327 CrossRef CAS.
- N. Khalid, G. Shu, B. J. Holland, I. Kobayashi, M. Nakajima and C. J. Barrow, Food Res. Int., 2017, 102, 364–371 CrossRef CAS.
- W. He, Y. Tan, Z. Tian, L. Chen, F. Hu and W. Wu, Int. J. Nanomed., 2011, 6, 521 CAS.
- M. Guttoff, A. H. Saberi and D. J. McClements, Food Chem., 2015, 171, 117–122 CrossRef CAS.
- D. J. McClements, Soft Matter, 2011, 7, 2297–2316 RSC.
- H. Schubert and R. Engel, Chem. Eng. Res. Des., 2004, 82, 1137–1143 CrossRef CAS.
- I. Kralova and J. Sjöblom, J. Dispersion Sci. Technol., 2009, 30, 1363–1383 CrossRef CAS.
- S. M. Jafari, E. Assadpoor, Y. He and B. Bhandari, Food Hydrocolloids, 2008, 22, 1191–1202 CrossRef CAS.
- P. Walstra, Chem. Eng. Sci., 1993, 48, 333–349 CrossRef CAS.
- G. L. Hasenhuettl and R. W. Hartel, Food emulsifiers and their applications, Springer, 2008 Search PubMed.
- Y. Yang, M. E. Leser, A. A. Sher and D. J. McClements, Food Hydrocolloids, 2013, 30, 589–596 CrossRef CAS.
- D. J. McClements and C. E. Gumus, Adv. Colloid Interface Sci., 2016, 234, 3–26 CrossRef CAS.
- B. Ozturk and D. J. McClements, Curr. Opin. Food Sci., 2016, 7, 1–6 CrossRef.
- B. Ozturk, S. Argin, M. Ozilgen and D. J. McClements, Food Chem., 2015, 188, 256–263 CrossRef CAS.
- R. Charoen, A. Jangchud, K. Jangchud, T. Harnsilawat, O. Naivikul and D. J. McClements, J. Food Sci., 2011, 76, E165–E172 CrossRef CAS.
- B. Ozturk, S. Argin, M. Ozilgen and D. J. McClements, J. Food Eng., 2014, 142, 57–63 CrossRef CAS.
- D. J. McClements, Curr. Opin. Colloid Interface Sci., 2004, 9, 305–313 CrossRef CAS.
- W. Oleszek and A. Hamed, Surfactants Renewable Resour., 2010, 239 CAS.
- H. Salminen, S. Aulbach, B. H. Leuenberger, C. Tedeschi and J. Weiss, Colloids Surf., B, 2014, 122, 46–55 CrossRef CAS.
- T. Ralla, H. Salminen, M. Edelmann, C. Dawid, T. Hofmann and J. Weiss, J. Agric. Food Chem., 2017, 65, 4153–4160 CrossRef CAS.
- M. T. M. Rosa, E. K. Silva, D. T. Santos, A. J. Petenate and M. A. A. Meireles, J. Food Eng., 2016, 168, 68–78 CrossRef CAS.
- G. Shu, N. Khalid, Z. Chen, M. A. Neves, C. J. Barrow and M. Nakajima, Food Chem., 2018, 255, 67–74 CrossRef CAS.
- N. Taarji, C. A. R. da Silva, N. Khalid, C. Gadhi, A. Hafidi, I. Kobayashi, M. A. Neves, H. Isoda and M. Nakajima, Food Chem., 2018, 246, 457–463 CrossRef CAS.
- Z. Chen, G. Shu, N. Taarji, C. J. Barrow, M. Nakajima, N. Khalid and M. A. Neves, Food Chem., 2018, 261, 322–328 CrossRef CAS.
- M. A. Tanner, X. Bu, J. A. Steimle and P. R. Myers, Nitric Oxide, 1999, 3, 359–365 CrossRef CAS.
- J. Yeo, Y.-J. Kang, S.-M. Jeon, U. J. Jung, M.-K. Lee, H. Song and M.-S. Choi, J. Med. Food, 2008, 11, 709–716 CrossRef CAS.
- J.-M. Lin, C.-C. Lin, H.-F. Chiu, J.-J. Yang and S.-G. Lee, Am. J. Chin. Med., 1993, 21, 59–69 CrossRef CAS.
- J.-C. Chen, K.-W. Lu, M.-L. Tsai, S.-C. Hsu, C.-L. Kuo, J.-S. Yang, T.-C. Hsia, C.-S. Yu, S.-T. Chou and M.-C. Kao, Oral Oncol., 2009, 45, 273–283 CrossRef CAS.
- L. Schild, B. Chen, P. Makarov, K. Kattengell, K. Heinitz and G. Keilhoff, Phytomedicine, 2010, 17, 589–597 CrossRef CAS.
- Z. B. Xiang, C. H. Tang, G. Chen and Y. S. Shi, Nat. Prod. Res. Dev., 2001, 13, 23–26 CAS.
- R. Iqbal, Z. Mehmood, A. Baig and N. Khalid, Food Bioprod. Process., 2020, 123, 304–311 CrossRef CAS.
- F. V. Rodrigues, L. S. Diniz, R. M. Sousa, T. D. Honorato, D. O. Simão, C. R. Araújo, T. M. Gonçalves, L. A. Rolim, P. L. Goto and A. C. Tedesco, Quim. Nova, 2018, 41, 756–761 CAS.
- T. B. Schreiner, A. Santamaria-Echart, A. Ribeiro, A. M. Peres, M. M. Dias, S. P. Pinho and M. F. Barreiro, Molecules, 2020, 25, 1538 CrossRef CAS.
- M. J. Rosen and J. T. Kunjappu, Surfactants and interfacial phenomena, John Wiley & Sons, 2012 Search PubMed.
- R. J. Hunter, Foundations of colloid science, Oxford University Press, 2001 Search PubMed.
- L. Bai and D. J. McClements, J. Colloid Interface Sci., 2016, 479, 71–79 CrossRef CAS.
- X.-P. Liu, J. Feng, L. Zhang, Q.-T. Gong, S. Zhao and J.-Y. Yu, Colloids Surf., A, 2010, 362, 39–46 CrossRef CAS.
- S. Tcholakova, N. D. Denkov and T. Danner, Langmuir, 2004, 20, 7444–7458 CrossRef CAS.
- L. Bai and D. J. McClements, J. Colloid Interface Sci., 2016, 466, 206–212 CrossRef CAS.
- D. McClements, E. Decker and J. Weiss, J. Food Sci., 2007, 72, R109–R124 CrossRef CAS.
- B. Ozturk, S. Argin, M. Ozilgen and D. J. McClements, Food Chem., 2015, 187, 499–506 CrossRef CAS.
- Y. Zhao, N. Khalid, G. Shu, M. A. Neves, I. Kobayashi and M. Nakajima, Int. J. Food Prop., 2017, 20, 1329–1341 CAS.
- J. S. Komaiko and D. J. McClements, Compr. Rev. Food Sci. Food Saf., 2016, 15, 331–352 CrossRef CAS.
- S. Schultz, G. Wagner, K. Urban and J. Ulrich, Chem. Eng. Technol., 2004, 27, 361–368 CrossRef CAS.
- Y. Zhao, N. Khalid, G. Shu, M. A. Neves, I. Kobayashi and M. Nakajima, Carbohydr. Polym., 2017, 176, 91–98 CrossRef CAS.
- C. Qian and D. J. McClements, Food Hydrocolloids, 2011, 25, 1000–1008 CrossRef CAS.
- Z. Yang, Q. Chen and L. Hu, Phytochemistry, 2007, 68, 1752–1761 CrossRef CAS.
- J. N. Israelachvili, Intermolecular and surface forces, Academic press, 2011 Search PubMed.
- D. J. McClements and J. Rao, Crit. Rev. Food Sci. Nutr., 2011, 51, 285–330 CrossRef CAS.
|
This journal is © The Royal Society of Chemistry 2020 |