DOI:
10.1039/D0RA07549C
(Paper)
RSC Adv., 2020,
10, 44815-44823
Surface cross-linked thermoplastic starch with different UV wavelengths: mechanical, wettability, hygroscopic and degradation properties
Received
3rd September 2020
, Accepted 7th December 2020
First published on 18th December 2020
Abstract
Here, we report a method to improve the properties of thermoplastic starch (TPS) by surface ultraviolet (UV) cross-linking. TPS sheets were prepared by injection molding and coated with an ethanol solution of photo-initiator TPO (2,4,6-trimethyl benzoyl diphenyl phosphine oxide), then, irradiated by UV with different wavelengths for 15 min. Untreated and irradiated TPS sheets were characterized using tensile and bending tests, impact tests, dynamic mechanical thermal analysis (DMTA) and infrared spectroscopy (FTIR). FTIR spectra showed that UV irradiation can effectively trigger surface cross-linking of TPS sheets. The mechanical and dynamic mechanical properties of the TPS were improved and the optimized properties were obtained by 308 nm UV irradiation. A tensile strength of 4.1 MPa, a bending strength of 2.7 MPa, an impact strength of 96.8 kJ m−2, and the corresponding activation energy of 251.22 kJ mol−1 were obtained. The water contact angle and moisture absorption of the samples were also investigated and the 308 nm UV irradiated sheets have a contact angle of 74°. Moisture absorption rate as a function of the square root of time showed a sigmoid curve including a linear stage which conforms to Fick's second law. The samples irradiated by 308 nm UV had the lowest equilibrium moisture absorption rate M∞ and the longest time T0 to enter into the Fick's diffusion stage and the lowest slope K and diffusion coefficient D. All samples displayed biodegradable properties when buried in soil. This method has potential applications for agricultural mulch films, packing and medical film products.
1. Introduction
Great attention has been paid to environmentally friendly biodegradable materials over the last two decades.1 As a natural biopolymer, starch is abundant, renewable, biodegradable, and low cost, and is considered as one of the promising raw materials for developing biodegradable plastics especially in sustainable packaging.2 Nowadays, thermoplastic starch (TPS) cannot be used widely because of retrogradation and unsatisfactory mechanical properties, particularly in wet and dry environments.3,4 In order to improve the mechanical and water resistant properties of TPS, some strategies have been adopted: (1) adding reinforcing fillers in the starch matrix such as inorganic minerals,5 and organic fibers;6,7 (2) acetylation,8 oxidation9 of hydroxyl groups of the starch chains; (3) cross-linking modification of starch.10
Cross-linking modification is an efficient method in limiting excessive water swelling and macromolecular motion of the starch matrix and thus avoid ageing. Treating granular starch with chemical cross-linking agent in heterogeneous media was made early in 1973 in which epichlorohydrin was used as cross-linker.11 Other cross-linking agent such as sodium trimetaphosphate and phosphoryl chloride were also reported to improve the property of starch.12–14 Although properties improvement has been made, use of chemical agents in starch modifications is still not recommended because of its increased processing costs and environmental and health issues.15 As an alternative method, radiative treatment such as gamma irradiation, electron beam irradiation, and photo-irradiation depending on the ionising sources either in the solid state or liquid state has been widely considered,16,17 and has been claimed to covalent cross-link of the polymer chain and improve the properties of the polymer.18
Unlike gamma irradiation or electron beam, UV light provides lower energy and cannot directly cleave the C–C or C–H bonds of starch molecules during the short periods of exposure time and is an energy-saving, environmental friendly and easy to operate modification method.19 In literature, both bulk and surface cross-linking are taken. e.g., Joly et al. present to use UV exposure to cross-link the thermoplastic starch after extruding the TPS film containing a low percentage of sensitizer, which is a bulk photo-crosslinking.20 Unlike bulk photo-crosslinking, modification on the surface of thermoplastic starch is an interesting approach which can change some surface properties of materials without using large amount of organic solvent and give rise to environmental and health concerns. Moreover, comparing with the bulk photo-crosslinking, the amounts of sensitizers used in surface photo-crosslinking was significantly lower, and the cross-linking reaction in the surface layer would be easier. Zhou et al. (2008) soaked the starch sheets in a photosensitizer sodium benzoate aqueous solution and cross-linked by UV irradiation.21 The results of water contact angle measurements and moisture absorption measurements showed that surface photo-crosslinking modifications significantly reduced the hydrophilic character of the starch sheet surface and enhanced water resistance of the starch sheets. Niazi et al. (2015) use UV cross-linking as a tool to steer the mechanical properties of TPS based films. Sodium benzoate was used as photosensitizer in the process and the films' retro-gradation was suppressed at 50% and 100% relative humidity.22
TPO (2,4,6-trimethyl benzoyl diphenyl phosphine oxide) was found to have the highest efficiency to manipulate the photo cross-linking process in microcapsules.23 So, TPO was used as photoinitiator in this study. TPS samples were prepared by injection molding and then coated with TPO/ethanol solution. Aiming to guarantee the efficiency of radical production of TPO and the following cross-linking of starch, three different UV wavelengths were chosen according to the UV absorption spectrum of TPO. We mainly focused on the effect of different UV wavelengths on mechanical (tensile, bending and impact), dynamic mechanical properties, surface wettability, hygroscopicity, and degradation properties of the TPS in this study.
2. Materials and methods
2.1 Materials
The corn starch (moisture content is 13.6 wt%, amylose content is 27%) was provided by Shandong Hengren Industry and Trade Company (Zaozhuang, China). Glycerin (CP) and ethanol were purchased from Sinopharm Holding Chemical Reagent Co., Ltd. (Shanghai, China). TPO was purchased from Nanjing Wali Chemical Technology Co., Ltd. (Nanjing, China). NaCl, NaBr, KCl, K2SO4 were provided by Nanjing Jiaozi Teng Scientific Equipment Co., Ltd. (Nanjing, China).
2.2 Preparation of UV surface crosslinked TPS
The starch and glycerin were pre-mixed manually at a ratio of 3
:
1, and the mixture was fed to a twin-screw plastic extruder running at 150 rpm (SHJ20, Nanjing, China). TPS dumbbell-shaped samples were prepared by injection molding machines (BV90, Shanghai, China). The 2 wt% TPO was prepared by dissolving 2 g TPO in 100 mL absolute ethanol, and then uniformly coated on the surface of the sample with a brush. The sample surface was irradiated with a UV mini-crosslink machine (SCIENTZ03-II, Ningbo, China) at 254, 308 and 365 nm UV wavelength for 15 min, respectively. The process was illustrated in Fig. 1.
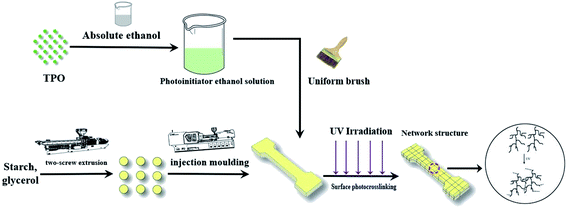 |
| Fig. 1 Schematic of the preparation of UV cross-linked TPS samples. | |
2.3 Mechanical measurements
After injection molding, the samples were immediately placed in some polyethylene bags to avoid moisture absorption and regeneration. Before testing, all samples were stored at a temperature of 23 ± 2 °C and humidity of 50% according to the ASTM D638 test standard. The tensile and bending test was then performed on a testing machine (E44.304, MTS, Shenzhen, China) at room temperature in accordance with ASTM D638. Impact measurement was performed on a testing machine (501J, Wance, Shenzhen, China) under the ASTM D256-10. Five to eight replicates were carried out per formulation.
Dynamic mechanical thermal analysis (DMTA) was performed using a Netzsch 242E instrument (NETZSCH 242E, Germany) in a three-point bending mode at a frequency of 1, 3.3, 5, 10 Hz. The temperature is in the range of −120 to 120 °C, and the heating rate is 3 °C min−1.
2.4 Spectral analysis
2.4.1 Ultraviolet spectrum analysis. UV absorption of TPO was measured at wavelength ranges of 200 nm to 400 nm, using a UV-2700 Ultraviolet-visible (UV-vis) spectrophotometer (Shimadzu, Japan).
2.4.2 Fourier transforms infrared spectroscopy. A Fourier transform infrared spectrometer (VERTEX 70, Bruker, Germany) was used to record the absorbance spectra of the TPS samples in attenuated total reflectance (ATR) mode. Powder samples were pressed by potassium bromide. IR spectra was measured at wavelengths from 500 cm−1 to 4000 cm−1.
2.5 Contact angle
Measurement of the contact angle was carried out at room temperature. The wetting behavior of the samples was measured and analyzed using a contact angle analyzer (DSA100, KRUSS, and Germany) and a watered syringe. A drop of water was dropped on the sample, and its angle of incidence was measured soon after deposition using software. Each photo was taken for 0.016 s to 1 s.
2.6 Moisture absorption process and model
The sample was cut into small pieces of about 15 × 15 mm and equilibrated for 24 h at room temperature. Configure saturated NaBr solution (57% relative humidity at room temperature), saturated NaCl solution (75% relative humidity at room temperature), saturated KCl solution (84% relative humidity at room temperature), and saturated K2SO4 solution (98% relative humidity at room temperature) in a desiccator and, then put the samples in it for moisture absorption test. At set intervals, the samples were taken out and weighed. The moisture absorption was calculated according to the following equation:
, where Wt is the weight of the sample at time t (g), W0 is the initial weight of the sample. When the weight of the sample changed little and almost no moisture was absorbed, that is, the moisture absorption reached equilibrium, the Mt–t1/2 curve was drawn. The slope K of the linear stage of moisture absorption curve can be obtained by the formula:
, in which Mt1 and Mt2 are the moisture absorption (%) of the sample at time t1 and t2, respectively. In this stage, the diffusion behavior of water complies with the Fick's second law:
. In the formula: D is the diffusion coefficient; M is the amount of moisture absorption; t is the time of moisture absorption; z is the thickness direction of the sample. The diffusion coefficient D can be obtained by the separation variable method and expressed as follows:
, where h is the thickness of the sample.
2.7 Soil burial test
A soil burial test was carried out according to Riyajan et al.24 The soil medium was poured into a plastic tray up to a thickness of approximately 18 cm. The samples were buried under 10 cm of soil, at an ambient temperature (∼25 °C) with a humidity conditions of 70∼80%. After that, water was sprayed twice a day to sustain the moisture in the medium. Every three days, the samples were collected and reweighed. The weight loss was calculated using equation as follows: weight loss (%) = [(W0 − Wt)/W0] × 100, where W0 and Wt is the weight of the sample before and after the soil burial test, respectively.25
2.8 Statistical analysis
For mechanical property measurement, five to eight specimens of each formulation were tested as mentioned above. Other measurements were repeated three times. Mean ± SD of every measurements was reported in the manuscript. SD was calculated and given by Origin Pro 8 software.
3. Results and discussion
In order to improve the properties of TPS, surface photo cross-linking was taken in this study. TPS samples were prepared by injection molding and then coated with TPO solution. Different wavelengths were selected to irradiate TPS samples with the aim to efficiently trigger cross-linking between starch macro-molecules. It is expected that UV irradiation will help to improve the mechanical and hydrophobic properties of TPS without affecting its degradation property.
3.1 Spectral analysis
UV absorption efficiency of photo initiator can affect the primary radical production and the following inter-molecules cross-linking of starch. So, the UV absorption spectrum of TPO in ethanol solution was determined and presented in Fig. 2a. It can be seen that the absorption peak of TPO is at 250, 296 and 380 nm, which is in accordance with the previous study.23 In this study, three different wavelengths including 254, 308, and 365 nm which are close to the maximum absorption of TPO were selected to investigate the effect of UV wavelength on the properties of TPS. Under these UV irradiation, TPO was deduced to decompose to benzoyl and phosphoryl which is α-cleavage in a Norrish-type I photo-reaction, then, the produced free-radical triggered cross-linking between starch macro molecules.26
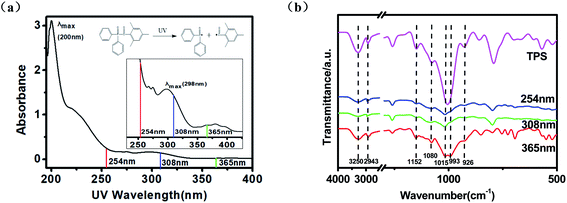 |
| Fig. 2 (a) UV absorption spectrum of TPO; (b) ATR-FTIR spectra of untreated or irradiated TPS samples. | |
To see the possible chemical and physical interaction during photo cross-linking, ATR-FTIR spectroscopic analysis of the untreated and irradiated TPS samples was also carried out and the results were shown in Fig. 2b. The broad bands at 3000–3500 cm−1 are ascribed to the stretching vibration of –OH of starch. This band became less intense when irradiated by UV, indicating the decrease of free –OH due to the cross-linking interaction between the starch chains.27 Accordingly, the intense of the peaks at 926, 1080 and 1152 cm−1 which are correspond to C–O stretching vibrations in C–O–H groups decreased. The intensity decrease of the bands at 1006 cm−1 which can be assigned to the C–O–C bond stretching of the starch ring, especially after 308 nm UV irradiation, also indicated the occurrence of cross-linking between starch chains.28,29 The bands at 993 cm−1, which is related to intramolecular hydrogen bonding of the hydroxyl group at C-6 almost disappear when irradiated by 308 nm UV wavelength. The mechanism of TPO decomposition and the following cross-linking of starch were shown in Fig. 3.19,30
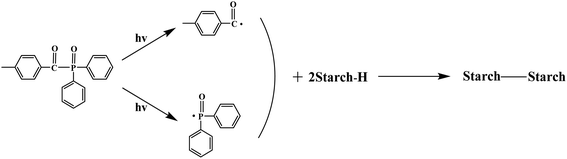 |
| Fig. 3 Mechanism of TPO decomposition and cross-linking between starch macromolecules. | |
3.2 Mechanical properties
The effect of both UV irradiation and their wavelength on the large strain behavior of TPS was analyzed up to their failure. The maximum tensile strength and elongation at break of TPS samples were shown in Fig. 4a. The tensile strength of TPS was obviously increased after irradiating by UV and have the maximum value of 4.1 MPa for the TPS irradiated by 308 nm UV, the TPS without irradiation is 2.9 MPa and an increase of 41.4% was obtained. The elongation at break of the photo-irradiated TPS samples has no obvious decrease (from 227.7 to 216%) compared with the untreated TPS. This mechanical improvement can be attributed to the cross-linking network structure formed on the surface of TPS by UV irradiation. Similar mechanical improvement was found in previous reports in which photo-crosslinked starch was prepared. e.g., Niazi et al. (2015) prepared TPS films using surface photo-crosslinking and found that UV irradiation improved the mechanical properties and decreased the solubility and degree of swelling.22 Kumar et al. (2008) investigated the effect of photo-crosslinking on the property of starch/cellulose composite films and found that both physical and mechanical properties were improved and the property increase was correlated with irradiation time.31 Goudarzi et al. (2018) developed a photo-modified starch/kefiran/TiO2 bio-nanocomposite and found that increasing UV-A exposure time brought about an increase of 14.9% in the tensile strength of the bio-nanocomposites.32
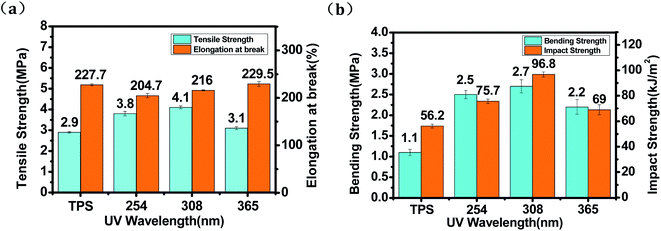 |
| Fig. 4 Tensile strength, elongation at break (a) and bending, impact strength (b) of untreated TPS and irradiated TPS with different UV wavelengths. | |
In this study, TPS sheets were prepared instead of films, so, the bending and impact strength of TPS sheets was also determined (shown in Fig. 4b). All the irradiated samples have an increased bending and impact strength. The bending and impact strength of TPS irradiated by 308 nm UV increased to 2.7 MPa and 96.8 kJ m−2, which is obvious higher than TPS without UV irradiation (1.1 MPa and 56.2 kJ m−2). Photo cross-linking efficiently improved the bending resistance and toughness of TPS materials.
3.3 Dynamic mechanical thermal properties
Fig. 5 is a three-dimensional mode of the storage modulus and loss factor curve in dynamic mechanical thermal analysis for untreated and UV irradiated TPS by performing a temperature scan at 1, 3.3, 5 and 10 Hz. Tg of the samples at 5.0 Hz from the loss factor curve was listed in Table 1. Tα and Tβ represents the Tg of the starch-enriched region and the glycerol-rich region in TPS, respectively.33,34 Obviously, Tα and Tβ of TPS irradiated by UV are higher than that of the untreated TPS. The maximum value was observed in the TPS samples irradiated by 308 nm UV. This is also corresponded to the aforementioned mechanical analysis results. Formation of the cross-linked network structure on the surface of TPS samples restricted the movement of starch chains and thus led to higher transition temperature in the matrix.
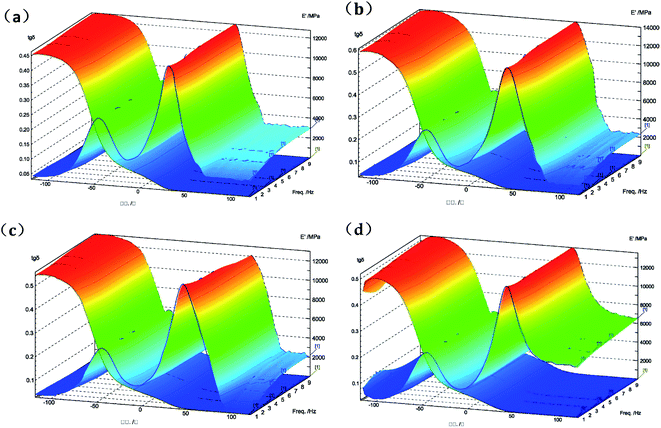 |
| Fig. 5 3D DMA curves of untreated TPS (a) and TPS irradiated with UV wavelength of 254 nm (b), 308 nm (c), and 365 nm (d). | |
Table 1 Glass transition temperature and activation energy of untreated TPS and irradiated TPS with different ultraviolet wavelength
Sample |
Tβ/°C (5.000 Hz) |
Tα/°C (5.000 Hz) |
ΔEα/(kJ mol−1) |
TPS |
−40.57 |
35.34 |
205.99 |
254 nm |
−40.51 |
47.27 |
246.60 |
308 nm |
−37.48 |
50.32 |
251.22 |
365 nm |
−39.46 |
45.17 |
243.96 |
The apparent activation energy, ΔE, was calculated according to the relationship between the peak temperature, Tα (K), and the frequency (Hz) of the tangent (tan
δ), using the following equation,35
where
T (K) is the peak temperature of the loss tangent,
f (Hz) is the frequency, and
R is the molar gas constant, which is 8.3145 J mol
−1 K
−1. Δ
E can be directly deduced from the slope of the graph of log
f with respect to 1/
T, and the results were also listed in
Table 1. Apparently, the TPS irradiated by 308 nm UV has the highest activation energy, which provides another proof for the movement restriction of starch chains in the UV irradiated TPS samples.
3.4 Effect of ultraviolet wavelength on TPS surface contact angle
Surface water wettability of the samples was estimated by water contact angle analysis, shown in Table 2. It can be seen that surface cross-linking by UV irradiation significantly increased the surface contact angle. The maximum contact angle still exists in the samples irradiated by 308 nm UV and was 75°, as expected. The surface contact angle of the untreated TPS was 34°. These results indicated that surface cross-linking could decrease the wettability or enhance the hydrophobicity of the TPS sheets surface. The increment of water contact angle of the UV-irradiated sheets could be explained by the network structure formed on the surface of TPS samples which reduces the number of hydrophilic groups on the starch backbone, and this is also indicated by the results of ATR-FTIR analysis mentioned above.
Table 2 Contact angle of untreated TPS and irradiated TPS with different UV wavelengths
Sample |
TPS |
254 nm |
308 nm |
365 nm |
Angle (°) |
34.28 ± 1.9 |
70.64 ± 1.4 |
75 ± 3.2 |
56.8 ± 0.8 |
Images |
 |
 |
 |
 |
3.5 Effect of ultraviolet irradiation on moisture absorption of TPS
Moisture absorption curves of untreated and irradiated TPS at different relative humidity of 57%, 75%, 84% and 98% were shown in Fig. 6. It can be seen that all samples showed similar variation trends of the relative weight gained as a function of time. There are two stages included in the process. At the first stage, the value of relative weight gained increased fast and reached a linear absorption as a function of the square root of time, then, the moisture absorption rate slows down and gradually approaches equilibrium state. As a whole, the moisture absorption rate of the irradiated samples is lower than the untreated one, and the TPS irradiated by 308 nm UV has the lowest moisture absorption rate. These results are in accordance with the aforementioned surface contact angle, which indicated that the UV irradiation increased surface water resistant ability and weakened the diffusion of water molecular which begins at the surface of the TPS materials.
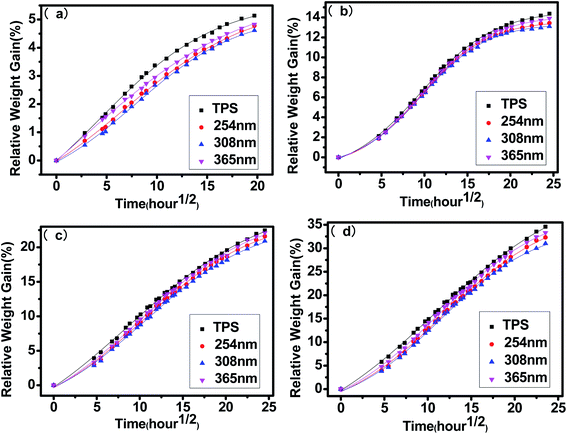 |
| Fig. 6 Measured and fitted moisture absorption of untreated TPS and UV irradiated TPS by different UV wavelengths at (a) 57% humidity (b) 75% humidity (c) 84% humidity (d) 98% humidity. | |
To better understand the moisture absorption performance, sigmoidal fitting of the moisture absorption data in Fig. 6 is carried out, the fitted equation is expressed as follows:
where
C1 and
C2 are constants related to factors such as material and moisture absorption temperature;
t is moisture absorption time;
M∞ is equilibrium moisture absorption rate;
T0 is the start time of linear water absorption stage at which the diffusion of water conforms to Fick's second law. The key parameters during the water absorption of each sample are presented in
Table 3 in which the symbol
K represents the slope of the curve at the Fick's diffusion stage and
D is the corresponding diffusion coefficient as mentioned in Section 2.6. As presented in the table, the equilibrium moisture absorption rate
M∞ of TPS decrease after UV irradiation whatever the relative humidity is. The TPS irradiated by 308 nm has the lowest value of
M∞. Accordingly, the time
T0 for the samples irradiated by 308 nm to enter into the Fick's diffusion stage is the longest, and the slope
K and the diffusion coefficient
D is the lowest. These results indicated that the photo cross-linking reaction at the surface of the samples provides a barrier function for moisture diffusion and this barrier property is most effective when irradiated by 308 nm UV. With the increasing of relative humidity, the diffusion coefficient
D of each sample decreased and the slope
K of the Fick's diffusion stage increased, accordingly, the equilibrium moisture absorption rate
M∞ increased. The plastication of water molecules improves the movement capacity of starch chain and thus facilitate the transfer of water in polymer materials.
36,37
Table 3 Hygroscopic kinetic parameters of untreated and irradiated TPS by different UV wavelengths
Relative humidity |
Sample |
C1 |
M∞ |
t0 |
C2 |
D |
K |
57% |
TPS |
−4.2727 |
6.0051 |
2.7744 |
8.1584 |
0.01010 |
0.3406 |
254 nm |
−1.9091 |
5.5858 |
6.8416 |
6.2610 |
0.00767 |
0.2760 |
308 nm |
−1.2418 |
5.2170 |
7.8252 |
5.2754 |
0.00748 |
0.2547 |
365 nm |
−2.7601 |
5.6972 |
4.3063 |
5.88426 |
0.00895 |
0.3042 |
75% |
TPS |
−1.7215 |
14.7007 |
9.5903 |
4.2910 |
0.00687 |
0.6874 |
254 nm |
−1.1759 |
13.6529 |
9.6644 |
3.8337 |
0.00699 |
0.6440 |
308 nm |
−1.0712 |
13.4050 |
9.6768 |
3.8034 |
0.00708 |
0.6367 |
365 nm |
−1.3716 |
14.1950 |
9.7675 |
4.0496 |
0.00669 |
0.6550 |
84% |
TPS |
−9.3430 |
26.8056 |
8.8081 |
8.0803 |
0.00459 |
1.0244 |
254 nm |
−5.4452 |
24.4594 |
10.4316 |
6.5938 |
0.00452 |
0.9278 |
308 nm |
−4.8750 |
23.7291 |
10.6908 |
6.4481 |
0.00439 |
0.8871 |
365 nm |
−6.0677 |
25.1149 |
10.0840 |
6.7737 |
0.00461 |
0.9627 |
98% |
TPS |
−11.9571 |
43.9561 |
10.7428 |
8.0795 |
0.00384 |
1.5366 |
254 nm |
−6.5866 |
38.3669 |
11.8466 |
6.3510 |
0.00417 |
1.3986 |
308 nm |
−5.0267 |
34.9065 |
11.5166 |
5.6118 |
0.00483 |
1.3691 |
365 nm |
−7.1449 |
39.0313 |
11.2606 |
6.3808 |
0.00454 |
1.4840 |
3.6 Effect of ultraviolet wavelength on TPS degradation property
The degradation rate of the samples was determined by the weight loss during burial time and the results were shown in Fig. 7. There are two main factors affecting the weight loss in this period: (1) the water absorption; (2) polymer degradation. The minus value of the degradation rate during the first 10 days was due to the higher water absorption than degradation, then, degradation rate increased gradually and reached maximum degradation rate after 40 days. Obviously, photo cross-linking lowered the degradation rate of TPS, and the TPS cross-linked by ultraviolet of 308 nm showed the lowest degradation rate. The starch degradation is due to the action of enzymes which is produced by fungi and/or bacteria in the soil, resulting in the formation of carbon dioxide, water and sugar.38 Surface photo cross-linking formed a barrier against the microorganism's attack and thus decreased the degradation rate of TPS. Pictures of the samples during burial period (partial) were shown in Fig. 8. The samples mainly showed swelling property during the first 6 days. After that, samples were broken into pieces and became brown color after 40 days. Notably, a film was observed in the photo cross-linked TPS samples especially after a period of degradation (Fig. 8b) which further manifest that cross-linking occurred at the surface of TPS.
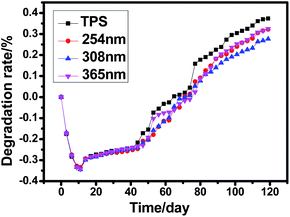 |
| Fig. 7 Degradation curve of untreated and irradiated TPS during 120 days. | |
 |
| Fig. 8 Photos of the untreated and irradiated TPS sheets during the burial period (a) and the films formed in the irradiated TPS sheets (b). | |
4. Conclusions
The thermoplastic starch can be effectively modified by UV cross-linking on its surface. The efficiency of cross-linking is related to the wavelength of UV which determines the efficiency of radical production in photo initiator. For TPO, 308 nm UV has the highest trigger efficiency. The tensile, bending and impact strength of the TPS sheets coated with 2 wt% TPO/ethanol solution can be improved significantly by 308 nm UV irradiation for 15 min. Therefore, UV irradiation is a simple and efficient approach to improve the mechanical property of TPS. Meanwhile, surface photo cross-linking is also an efficient method to improve the water resistance and surface hydrophobic properties. The samples irradiated by 308 nm UV showed higher water contact angle, lower moisture absorption rate. All samples displayed bio-degradation to some extent when berried in soil. The irradiated TPS materials are expected to be used in agricultural mulch film, packing and medical film in the future.
Conflicts of interest
There are no conflicts to declare.
Acknowledgements
The authors thank the Jiangsu Government Scholarship for overseas studies, Higher School in Jiangsu Province College Students' Practice Innovation Training Programs (201910298025Z), and the Natural Science Foundation of Jiangsu Province (BK20140967).
References
- M. A. Hillmyer, The promise of plastics from plants Plant-derived feedstocks are increasingly competitive in plastics production, Science, 2017, 358, 868–870 Search PubMed.
- A. K. Mohanty, S. Vivekanandhan and J. M. Pin, Composites from renewable and sustainable resources: challenges and innovations, Science, 2018, 362, 536–542 Search PubMed.
- M. B. K. Niazi, M. Zijlstra and A. A. Broekhuis, Influence of plasticizer with different functional groups on thermoplastic starch, J. Appl. Polym. Sci., 2015, 132, 42012 Search PubMed.
- A. L. M. Smits, P. H. Kruiskamp, J. J. G. van Soest and J. F. G. Vliegenthart, Interaction between dry starch and plasticisers glycerol or ethylene glycol, measured by differential scanning calorimetry and solid state NMR spectroscopy, Carbohydr. Polym., 2003, 53, 409–416 Search PubMed.
- R. Zhang, X. Wang and M. Cheng, Preparation and characterization of potato starch film with various size of nano-SiO2, Polymers, 2018, 10, 1172 Search PubMed.
- B. Guo, L. J. Wang, P. Yin, B. G. Li and P. X. Li, Ultra-high molecular weight polyethylene fiber-reinforced thermoplastic corn starch composite, J. Thermoplast. Compos. Mater., 2017, 30, 564–577 Search PubMed.
- W. Zhou, D. D. Zha, X. Zhang, J. Xu, B. Guo and Y. N. Huang, Ordered long polyvinyl alcohol fiber-reinforced thermoplastic starch composite having comparable mechanical properties with polyethylene and polypropylene, Carbohydr. Polym., 2020, 250, 116913 Search PubMed.
- B. F. Bergel, S. D. Osorio, L. M. da Luz and R. M. C. Santana, Effects of hydrophobized starches on thermoplastic starch foams made from potato starch, Carbohydr. Polym., 2018, 200, 106–114 Search PubMed.
- Y. R. Zhang, X. L. Wang and G. M. Zhao, Influence of oxidized starch on the properties of thermoplastic starch, Carbohydr. Polym., 2013, 96, 358–364 Search PubMed.
- L. P. Qiu, F. Hu and Y. L. Peng, Structural and mechanical characteristics of film using modified corn starch by the same two chemical processes used in different sequences, Carbohydr. Polym., 2013, 91, 590–596 Search PubMed.
- L. Kuniak and R. H. Marchessault, Study of the crosslinking reaction between epichlorohydrin and starch, Starch/Staerke, 1973, 24, 110–116 Search PubMed.
- J. Jane, A. Xu, M. Radosavljevic and P. A. Seib, Location of amylose in normal starch granules. I. Susceptibility of amylose and amylopectin to cross-linking reagents, Cereal Chem., 1992, 69, 405–409 Search PubMed.
- C. Yook, U. A. Pek and K. H. Park, Gelatinization and retrogradation characteristics of hydroxypropylated and cross-linked rices, J. Food Sci., 1993, 58, 405–407 Search PubMed.
- K. Woo and P. A. Seib, Cross-linking of wheat starch and hydroxypropylated wheat starch in alkaline slurry with sodium trimetaphosphate, Carbohydr. Polym., 1997, 33, 263–271 Search PubMed.
- J. Zhou, J. Zhang, Y. Ma and J. Tong, Surface photo-crosslinking of corn starch sheets, Carbohydr. Polym., 2008, 74, 405–410 Search PubMed.
- N. Detduangchan and T. Wittaya, Effect of UV-treatment on properties of biodegradable film from rice starch, World Acad. Sci. Eng. Technol., 2011, 5, 829–834 Search PubMed.
- J. Delville, C. Joly, P. Dole and C. Bliard, Solid state photocrosslinked starch based films: a new family of homogeneous modified starches, Carbohydr. Polym., 2002, 49, 71–81 Search PubMed.
- M. B. K. Niazi and A. A. Broekhuis, Surface photo-crosslinking of plasticized thermoplastic starch films, Eur. Polym. J., 2015, 64, 229–243 Search PubMed.
- G. J. He, Q. Liu and M. R. Thompson, Characterization of structure and properties of thermoplastic potato starch film surface cross-linked by UV irradiation, Starch/Staerke, 2013, 65, 304–311 Search PubMed.
- J. Delville, C. Joly and P. Dole, Solid state photocrosslinked starch based films: a new family of homogeneous modified starches, Carbohydr. Polym., 2002, 49, 71–81 Search PubMed.
- J. Zhou, J. Zhang and Y. H. Ma, Surface photo-crosslinking of corn starch sheets, Carbohydr. Polym., 2008, 74, 405–410 Search PubMed.
- M. B. K. Niazi and A. A. Broekhuis, Surface photo-crosslinking of plasticized thermoplastic starch films, Eur. Polym. J., 2015, 64, 229–243 Search PubMed.
- X. W. Li, W. D. Lai, S. S. Meng and H. Y. Yu, Photoinitiator Influence on the Photo-crosslink Property in Polyurea Microcapsules, J. Photopolym. Sci. Technol., 2009, 22, 603–608 Search PubMed.
- S. A. Riyajan, Y. Sasithornsonti and P. Phinyocheep, Green natural rubber-g-modified starch for controlling urea release, Carbohydr. Polym., 2012, 89, 251–258 Search PubMed.
- M. Bootklad and K. Kaewtatip, Biodegradation of thermoplastic starch/eggshell powder composites, Carbohydr. Polym., 2013, 97, 315–320 Search PubMed.
- K. Ruhland, F. Habibollahi and R. Horny, Quantification and elucidation of the UV-light triggered initiation kinetics of TPO and BAPO in liquid acrylate monomer, J. Appl. Polym. Sci., 2020, 48357, 1–16 Search PubMed.
- H. J. Wu, Y. L. Lei, J. Y. Lu, R. Zhu, D. Xiao, C. Jiao, R. Xia, Z. Q. Zhang, G. H. Shen, Y. T. Liu, S. S. Li and M. L. Li, Effect of citric acid induced crosslinking on the structure and properties of potato starch/chitosan composite films, Carbohydr. Polym., 2019, 97, 105208 Search PubMed.
- N. Gurler, S. Pasa, M. H. Alma and H. Temel, The fabrication of bilayer polylactic acid films from cross-linked starch as eco-friendly biodegradable materials: synthesis, characterization, mechanical and physical properties, Eur. Polym. J., 2020, 127, 109588 Search PubMed.
- J. Brugnerotto, J. Lizardi, F. M. Goycoolea, W. Arguelles-Monal, J. Desbrieres and M. Rinaudo, An infrared investigation in relation with chitin and chitosan characterization, Polymer, 2001, 42, 3569–3580 Search PubMed.
- A. H. M. Zain, M. K. A. Wahab and H. Ismail, Solid-state photo-cross-linking of cassava starch: improvement properties of thermoplastic starch, Polym. Bull., 2018, 75, 3341–3356 Search PubMed.
- A. P. Kumar and R. P. Singh, Biocomposites of cellulose reinforced starch: improvement of properties by photo-induced crosslinking, Bioresour. Technol., 2008, 99, 8803–8809 Search PubMed.
- V. Goudarzi and I. Shahabi-Ghahfarrokhi, Development of photo-modified starchikefiran/TiO2 bio-nanocomposite as an environmentally-friendly food packaging material, Int. J. Biol. Macromol., 2018, 116, 1082–1088 Search PubMed.
- A. L. Da Roz, A. J. F. Carvalho, A. Gandini and A. A. S. Curvelo, The effect of plasticizers on thermoplastic starch compositions obtained by melt processing, Carbohydr. Polym., 2006, 63, 417–424 Search PubMed.
- K. M. Dang and R. Yoksan, Development of thermoplastic starch blown film by incorporating plasticized chitosan, Carbohydr. Polym., 2015, 115, 575–581 Search PubMed.
- N. Anousheh, F. O. Godey and A. Soldera, Unveiling the Impact of Regioisomerism Defects in the Glass Transition Temperature of PVDF by the Mean of the Activation Energy, J. Polym. Sci., Part A: Polym. Chem., 2017, 55, 419–426 Search PubMed.
- L. R. Bao, A. F. Yee and C. Y. C. Lee, Moisture absorption and hygrothermal aging in a bismaleimide resin, Polymer, 2001, 42, 7327–7333 Search PubMed.
- L. R. Bao and A. F. Yee, Moisture absorption and hygrothermal aging in bismaleimide matrix carbon fiber composites-part I: uni-weave composites, Compos. Sci. Technol., 2002, 62, 2099–2110 Search PubMed.
- S. M. M. Franchetti and J. C. Marconato, Polímeros biodegradáveis-uma solução parcial para diminuir a quantidade dos resíduos plásticos, Quim. Nova, 2006, 29, 811–816 Search PubMed.
|
This journal is © The Royal Society of Chemistry 2020 |