DOI:
10.1039/D0RA07212E
(Paper)
RSC Adv., 2020,
10, 33155-33160
Electrochemical sulfonylation of alkenes with sulfonyl hydrazides: a metal- and oxidant-free protocol for the synthesis of (E)-vinyl sulfones in water†
Received
21st August 2020
, Accepted 1st September 2020
First published on 8th September 2020
Abstract
An efficient electrochemical transformation of a variety of alkenes and sulfonyl hydrazides into vinyl sulfones with a catalytic amount of tetrabutylammonium iodide in water is reported. The reaction proceeds smoothly to afford vinyl sulfones with good selectivities and yields at room temperature under air in an undivided cell. Cyclic voltammograms and control experiments have been performed to provide preliminary insight into the reaction mechanism. The key features of this reaction include using pure water as solvent, transition metal- and oxidant-free conditions, and being easily scaled up to gram-scale synthesis.
Introduction
To achieve green and sustainable procedures, metal-free strategies have attracted much attention during the past decade.1 In particular, transition-metal-free couplings in water could provide great opportunities for more sustainable synthetic chemistry.2 In addition, the development of transition-metal-free reactions in water enabled by efficient and cleaner techniques could explore novel approaches to construct chemical products in an environmentally benign manner.3
Vinyl sulfones are important and useful building blocks for various organic transformations and exhibit a broad range of biological properties.4 As a consequence, various synthetic routes to vinyl sulfones have been developed.5,6 In terms of these synthetic methodologies, the cross-coupling of a variety of alkenes with sulfonyl hydrazides has garnered considerable attention for the synthesis of vinyl sulfones.6 Despite some great advantages of these reactions, the methods still suffered from certain shortcomings, such as requiring strong oxidants,6a–d employment of transition metal catalysts,6e or high temperature.6f Therefore, it is desirable to synthesize vinyl sulfones directly from styrenes and sulfonyl hydrazides under the metal and oxidant-free conditions.
Organic electrosynthesis has been recognized as an atom-economical and eco-friendly benign synthetic strategy, wherein the electric current can be used to replace a stoichiometric amount of a redox agent.7 Recently, an elegant example has been devoted to the electrosynthesis of vinyl sulfones by Terent'ev and co-workers.8 However, the protocol still involved excessive KI and organic solvent. Thus, a more green and efficient strategy for the electrosynthesis of (E)-vinyl sulfones between styrenes and sulfonyl hydrazides under milder reaction condition is desired. As a benign solvent, water could lead to new reactivity, as well as environmentally.9 As part of our ongoing activities in developing electrochemical aqueous-phase reactions,10 we report here an electrochemical cross-coupling of readily available styrenes and sulfonyl hydrazides using iodide salt as the catalyst with an elegant constant current in water at room temperature under air.
Results and discussion
At the beginning of our investigation, we used styrene 1a and p-toluenesulfonyl hydrazide 2a as the model substrates to screen the optimized conditions. After some experiments, the optimal reaction conditions were defined as electrolyzing 1a and 2a in saturated (NH4)2CO3 aqueous solution using 10 mol% of n-Bu4NI as the catalyst at room temperature in an undivided cell with Pt foils (1.0 × 1.5 cm2). Under these mild conditions, the desired product 3a was obtained in 88% yield after the reaction mixture had been stirred for 3.0 h (Table 1, entry 1). It was noted that the yield of 3a increased sharply to 32% when the reaction was run without n-Bu4NI (entry 2). When n-Bu4NI was replaced with some other catalysts, such as NH4I, PhI(OAc)2, n-Bu4NBr and I2, the yield of the desired product 3a was reduced (entries 3–6). Among a series of electrolyte (entries 7–8), sat. aq. (NH4)2CO3 gave the optimal result. Subsequently, the electrode material was also screened. When the Pt foil electrodes were replaced by graphite rods or reticulated vitreous carbon rods, the yield of the desired product dropped (entries 9–10). In addition, the electrolysis at lower or higher current density led to a decrease of the yield (entries 11–12). Finally, it was found that no desired product could be obtained without an electric current in this reaction (entry 13).
Table 1 Optimization of reaction conditionsa

|
Entry |
Variation from the standard conditions |
Yieldb [%] |
Standard conditions: the mixture of 1a (0.5 mmol), 2a (1.0 mmol), n-Bu4NI (10 mol%) in saturated (NH4)2CO3 aqueous solution (5.0 mL) was electrolyzed at constant current (40 mA) in an undivided cell at room temperature for 3.0 hours, anode and cathode: Pt foil (1.0 × 1.5 cm2). The yield of the product was determined by 1H NMR spectroscopy; N.R., no reaction. Graphite rods (diameter: 0.5 cm, height: 1.78 cm). Reticulated vitreous carbon RVC (100 PPI, 1.5 cm × 1 cm × 0.2 cm). |
1 |
None |
88 |
2 |
In the absence of n-Bu4NI |
32 |
3 |
NH4I instead of n-Bu4NI |
77 |
4 |
PhI(OAc)2 instead of n-Bu4NI |
48 |
5 |
n-Bu4NBr instead of n-Bu4NI |
68 |
6 |
I2 instead of n-Bu4NI |
56 |
7 |
Sat. aq. NH4HCO3 instead of sat. aq. (NH4)2CO3 |
53 |
8 |
Sat. aq. CS2CO3 instead of sat. aq. (NH4)2CO3 |
75 |
9 |
Graphite rods as electrodes |
72 |
10c |
Reticulated vitreous carbon RVC as electrodes |
70 |
11d |
30 mA instead of 40 mA, 4 h |
77 |
12 |
50 mA instead of 40 mA, 2.4 h |
81 |
13 |
No electric current |
N.R. |
With the optimized reaction conditions in hand, a series of styrene derivatives were further evaluated. As presented in Scheme 1, the reaction proceeded smoothly with various of styrenes to afford the corresponding vinyl sulfones with moderate to good yields (3a–o). Notably, para-, meta-, or ortho-substituted aryl alkenes, bearing either electron-donating groups (Me and OMe, 3b–g) or electron-withdrawing (F, Br, and Cl, 3h–j) groups on the aryl ring, gave high yields of the desired products. Strong electron-withdrawing groups (CF3, 3k) influenced the reaction and delivered lower yields. Moreover, naphthalene derivative (3l) was also accommodated in the reaction and afforded the desired product in a yield of 74%. Heteroarene-based styrenes were also good substrates for this process and gave the desired products in moderate yields (3m–o). Importantly, excellent E selectivity (E/Z > 99
:
1) were observed in all product. Notably, alkenes with some functional groups-substituted, such as ester, amides, and silyl ethers are not compatible with this electrochemical transformation.
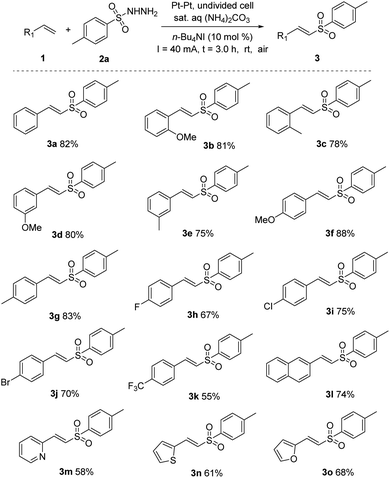 |
| Scheme 1 Scope of the reaction with styrene.a,b aReaction conditions: the mixture of 1 (0.5 mmol), 2a (1.0 mmol), n-Bu4NI (10 mol%) in saturated (NH4)2CO3 aqueous solution (5.0 mL) was electrolyzed at constant current (40 mA) in an undivided cell at room temperature for 3.0 hours, anode and cathode: Pt foil (1.0 × 1.5 cm2). bYields of isolated products. | |
To further examine the scope of this reaction, a range of sulfonyl hydrazides were also used to react with styrene. As shown in Scheme 2, unsubstituted sulfonyl hydrazide afforded the product 4a with 81% yield. A series of sulfonyl hydrazides bearing electron-donating groups (OMe and tBu 4b–d) and electron-withdrawing (F, Br, Cl, and NHAc, 4e–h, 4j) groups produced sulfones in moderate to good yields. The strongly electron-withdrawing group (CF3, 4i) led to a slight decrease in yield. Furthermore, sulfonyl hydrazides with a naphthyl group (4k) or a thienyl group (4l) were examined in this reaction, and the desired products were afforded in yields of 70% and 68%, respectively. It was noteworthy that excellent E selectivity (E/Z > 99
:
1) was observed in all cases.
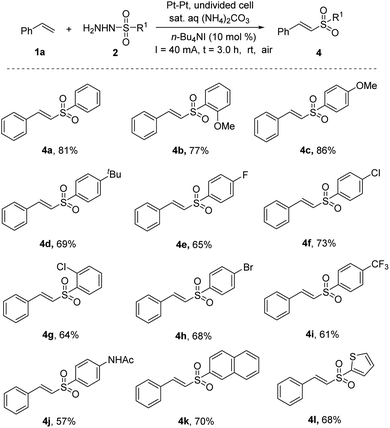 |
| Scheme 2 Scope of the reaction with sulfonyl hydrazides.a,b aReaction conditions: the mixture of 1a (0.5 mmol), 2 (1.0 mmol), n-Bu4NI (10 mol%) in saturated (NH4)2CO3 aqueous solution (5.0 mL) was electrolyzed at constant current (40 mA) in an undivided cell at room temperature for 3.0 hours, anode and cathode: Pt foil (1.0 × 1.5 cm2). bYields of isolated products. | |
To demonstrate the synthetic utility of this transformation, electrochemical synthesis of the (E)-vinyl sulfones in water was performed at a gram scale (Scheme 3). Product 3a could be prepared in 72% of yield by prolonging the time and changing the size of platinum electrodes, further highlighting the utility of the electrochemical protocol.
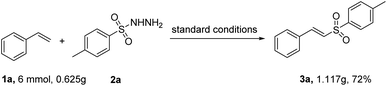 |
| Scheme 3 Gram-scale electrochemical synthesis of 3a. | |
To understand the mechanism of this reaction, a series of preliminary mechanistic experiments were performed (Scheme 4). The reaction of 1a and 2a was inhibited in the presence of radical inhibitors, such as 2,2,6,6-tetramethyl-1-piperidinyloxy (TEMPO) and 2,6-di-tert-butyl-4-methylphenol (BHT) (Scheme 4, eqn (1)) under standard conditions, which supported that the reaction presumably involved a radical pathway. To determine the involvement of molecular iodine, the reaction was carried out in the presence of I2, the desired product 3a was afforded in a 53% yield (Scheme 4, eqn (2)). It was proposed that I2 produced by anode has an effect on the activation of the reaction. In addition, when 4-methylbenzenesulfonyl iodide 4a was subjected to the reaction with 1a under standard conditions, a 78% yield of 3a was obtained (Scheme 4, eqn (3)). Similarly, direct synthesis of desired product 3a from the β-iodosulfone 5a under the standard conditions was also successful (Scheme 4, eqn (4)). But when β-hydroxysulfone 6a was used as the starting material under the standard conditions, no target product 3a was obtained (Scheme 4, eqn (5)). Thus, it was demonstrated that 4-methylbenzenesulfonyl iodide 4a and β-iodosulfone 5a could be active intermediates for this reaction and the involvement of β-hydroxysulfone 6a as an intermediate was ruled out.
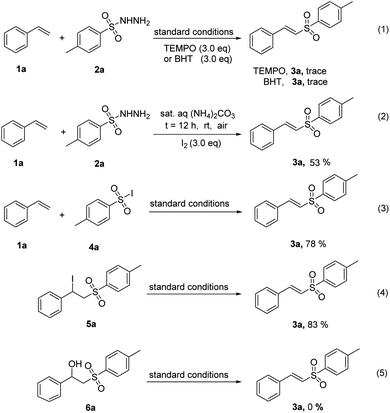 |
| Scheme 4 Preliminary mechanistic studies. | |
To gain more insight into this reaction mechanism, cyclic voltammetry (CV) experiments were carried out. As shown in Scheme 5, the oxidation peak of 1a was observed at Ep = 1.95 V vs. SCE (Scheme 5(I), curve b).8 Then, the CV of 2a displayed two oxidation peaks at 0.36 V vs. SCE and 0.91 V vs. SCE (Scheme 5(I), curve c).6b These results showed that 2a was oxidized preferentially than 1a under the standard conditions. Additionally, we found that the pH of the reaction solution remained at 9.34–9.10 throughout the process (Scheme 6), which implied that the oxidation of 2a could be promoted in basic acidic reaction conditions.11 Next, the role of n-Bu4NI was also studied. The anode potential of n-Bu4NI was observed at 0.90 V vs. SCE (Scheme 5(I), curve d), which indicates that iodine ions (I−) could be oxidized to molecular iodine (I2).6b,8,12 The CV result of 1a and n-Bu4NI mixtures showed no obvious new oxidation peaks (Scheme 5(II), curve e), which indicated that n-Bu4NI did not affect compound 1a. Intriguingly, the addition of n-Bu4NI to 2a led to two new oxidation peaks at 1.11 V vs. SCE and 1.52 V vs. SCE (Scheme 5(III), curve f), which indicated that sulfonyl hydrazide 2a could be oxidized by I2 or its derivatives in the electrolysis process.13 Furthermore, in the mixture solution of 1a, 2a, and n-Bu4NI, it did not significantly change the curve (Scheme 5(IV), curve g). Combined with one of the control experiments (Scheme 3, eqn (2)), it suggested that I2 produced by the electrochemical process is involved in the reaction.
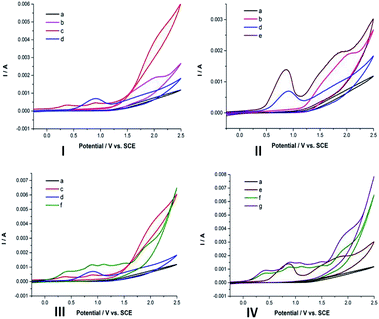 |
| Scheme 5 Cyclic voltammetry experiments.a aCyclic voltammograms of related compounds in sat. aq. (NH4)2CO3, using a glassy carbon electrode as working electrode (d = 3 mm), a Pt wire as counter electrode, and a saturated calomel electrode (SCE) as a reference electrode, at 100 mV s−1 scan rate: (a) none; (b) 1a (0.005 M); (c) 2a (0.005 M); (d) n-Bu4NI (0.001 M); (e) 1a (0.005 M) and n-Bu4NI (0.001 M); (f) 2a (0.005 M) and n-Bu4NI (0.001 M); (g) 1a (0.005 M), 2a (0.005 M) and n-Bu4NI (0.001 M). | |
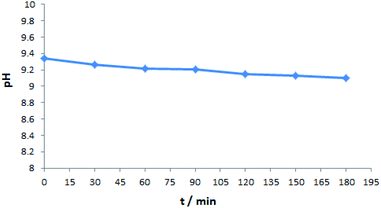 |
| Scheme 6 pH value during the process of the reaction.a aThe pH value was measured every 30 minutes. | |
In light of these experimental results and previous works,6,8,12–14 two possible mechanisms for this reaction are proposed in Scheme 7. In Path A, the iodide ions were oxidized to molecular iodine at the anode. Under the basic aqueous reaction conditions, molecular iodine can further form electrophilic iodine-containing species [I]+. Then, the iodine-containing species (I2, [I]+) reacted with hydrazide 2a to give the sulfonyl iodide 4a, which was decomposed to generate sulfonyl radical 7a and iodine radical. Subsequently, the sulfonyl radical 7a added to styrene 1a to afford the benzylic radical 8a, followed by the reaction with iodine radical to form β-iodosulfone intermediate 5a. Finally, β-iodosulfone intermediate 5a was converted into the desired product 3a through elimination of HI. While in Path B, hydrazide 2a generated the corresponding sulfonyl radical 7a via preferential deprotonation under the basic condition and three electron oxidation together with the release of molecular nitrogen. The sulfonyl radical 7a then reacted with styrene 1a to produce the benzylic radical 8a, which was further oxidized to benzyl cation 9a on the anode. The benzyl cation 9a can generate 3a directly through liberating H+ or react with iodide anion to form β-iodosulfone 5a, which can lead to 3a formation through HI elimination.
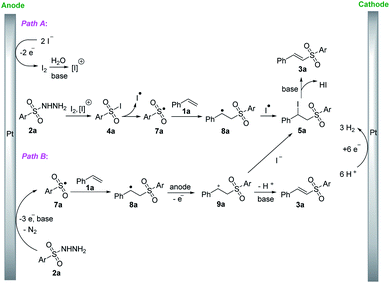 |
| Scheme 7 Proposed mechanism. | |
Conclusions
We developed an efficient electrochemical method for the synthesis of (E)-vinyl sulfones from a variety of alkenes and sulfonyl hydrazides with a catalytic amount of iodide salt in water. The reaction proceeded smoothly to afford (E)-vinyl sulfones with good selectivities and yields in an undivided cell at room temperature under metal- and external oxidant-free conditions. A satisfactory gram-scale reaction showed the synthetic utility of this process. Further exploration of the synthetic applications is undergoing in our lab.
Conflicts of interest
There are no conflicts to declare.
Acknowledgements
The authors thank the Innovation Projects of Department of Education of Guangdong Province (2018KQNCX236, 2019KTSCX162), the Science and Technology Project of Guangdong Province (408-99000213), the Science and Technology Project of Shaoguang city (2018sn047, 2019sn055), Shaoguan University (408-9900416, 408-99000618), National undergraduate innovation and entrepreneurship project (201810576016), National Natural Science Foundation of China (21961002), and the emergency project of Gannan Medical University (YJ202027) for financial support.
Notes and references
- For examples, see:
(a) A. Bhunia, S. R. Yetra and A. T. Biju, Chem. Soc. Rev., 2012, 41, 3140 RSC
;
(b) S. Roscales and A. G. Csaky, Chem. Soc. Rev., 2014, 43, 8215 RSC
;
(c) A. B. Cuenca, R. Shishido, H. Ito and E. Fernandez, Chem. Soc. Rev., 2017, 46, 415 RSC
. -
(a) E. Nakamura and K. Sato, Nat. Mater., 2011, 10, 158 CrossRef CAS
;
(b) C.-L. Sun and Z.-J. Shi, Chem. Rev., 2014, 114, 9219 CrossRef CAS
;
(c) W. Liu, L. Li and C.-J. Li, Nat. Commun., 2015, 6, 6526 CrossRef CAS
. -
(a) A. Albini and M. Fagnoni, Green Chem., 2004, 6, 1 RSC
;
(b) Y. Yuan and A. Lei, Nat. Commun., 2020, 11, 802 CrossRef CAS
. - For examples, see:
(a) G. Wang, U. Mahesh, G. Y. J. Chen and S. Q. Yao, Org. Lett., 2003, 5, 737 CrossRef CAS
;
(b) B. A. Frankel, M. Bentley, R. G. Kruger and D. G. McCafferty, J. Am. Chem. Soc., 2004, 126, 3404 CrossRef CAS
;
(c) F. Hof, A. Schutz, C. Fah, S. Meyer, D. Bur, J. Liu, D. E. Goldberg and E. Diederich, Angew. Chem., Int. Ed., 2006, 45, 2138 CrossRef CAS
;
(d) D. C. Meadows, T. Sanchez, N. Neamati, T. W. North and J. Gervay-Hague, Bioorg. Med. Chem., 2007, 15, 1127 CrossRef CAS
;
(e) M. Uttamchandani, K. Liu, R. C. Panicker and S.-Q. Yao, Chem. Commun., 2007, 1518 RSC
. - For examples, see:
(a) S. Chodroff and W. F. Whitmore, J. Am. Chem. Soc., 1950, 72, 1073 CrossRef CAS
;
(b) I. C. Popoff, J. L. Dever and G. R. Leader, J. Org. Chem., 1969, 34, 1128 CrossRef CAS
;
(c) J. M. Baskin and Z. Wang, Org. Lett., 2002, 4, 4423 CrossRef CAS
;
(d) Y. Xu, X. Tang, W. Hu, W. Wu and H. Jiang, Green Chem., 2014, 16, 3720 RSC
;
(e) A. U. Meyer, S. Jäger, D. Prasad Hari and B. König, Adv. Synth. Catal., 2015, 357, 2050 CrossRef CAS
;
(f) R. Singh, B. K. Allam, N. Singh, K. Kumari, S. K. Singh and K. N. Singh, Org. Lett., 2015, 17, 2656 CrossRef CAS
;
(g) S. Cai, Y. Xu, D. Chen, L. Li, Q. Chen, M. Huang and W. Weng, Org. Lett., 2016, 18, 2990 CrossRef CAS
;
(h) Y. Fang, Z. Luo and X. Xu, RSC Adv., 2016, 6, 59661 RSC
;
(i) L. Kadari, R. K. Palakodety and L. P. Yallapragada, Org. Lett., 2017, 19, 2580 CrossRef CAS
;
(j) H. Yue, C. Zhu and M. Rueping, Angew. Chem., Int. Ed., 2018, 57, 1371 CrossRef CAS
;
(k) Q. Gui, K. Han, Z. Liu, Z. Su, X. He, H. Jiang, B. Tian and Y. Li, Org. Biomol. Chem., 2018, 16, 5748 RSC
;
(l) S. Kumar, R. Singh and K. N. Singh, Asian J. Org. Chem., 2018, 7, 359 CrossRef CAS
. -
(a) X. Li, X. Xu and C. Zhou, Chem. Commun., 2012, 48, 12240 RSC
;
(b) S. Tang, Y. Wu, W.-Q. Liao, R.-P. Bai, C. Liu and A. W. Lei, Chem. Commun., 2014, 50, 4496 RSC
;
(c) B. Aegurla and R. K. Peddinti, Asian J. Org. Chem., 2018, 7, 946 CrossRef CAS
;
(d) W.-H. Bao, W.-W. Ying, X.-D. Xu, G.-D. Zhou, X.-X. Meng, W.-T. Wei, Y.-Y. Liu and Q. Li, Tetrahedron Lett., 2019, 60, 55 CrossRef CAS
;
(e) X.-W. Li, Y.-L. Xu, W.-Q. Wu, C. Jiang, C.-R. Qi and H.-F. Jiang, Chem.–Eur. J., 2014, 20, 7911 CrossRef CAS
;
(f) Z. Zhan, H. Ma, D. Wei, J. Pu, Y. Zhang and G. Huang, Tetrahedron Lett., 2018, 59, 1446 CrossRef CAS
;
(g) M. Pramanik, K. Choudhuri and P. Mal, Asian J. Org. Chem., 2019, 8, 144 CrossRef CAS
. - For recent representative reviews about electrosynthesis, see:
(a) M. Yan, Y. Kawamata and P. S. Baran, Chem. Rev., 2017, 117, 13230 CrossRef CAS
;
(b) Y. Jiang, K. Xu and C.-C. Zeng, Chem. Rev., 2018, 118, 4485 CrossRef CAS
;
(c) J.-I. Yoshida, A. Shimizu and R. Hayashi, Chem. Rev., 2018, 118, 4702 CrossRef CAS
;
(d) K. Liu, C. L. Song and A. Lei, Org. Biomol. Chem., 2018, 16, 2375 RSC
;
(e) A. Wiebe, T. Gieshoff, S. Mçhle, E. Rdrigo, M. Zirbes and S. R. Waldvogel, Angew. Chem., Int. Ed., 2018, 57, 5594 CrossRef CAS
;
(f) S. Mçhle, M. Zirbes, E. Rodrigo, T. Gieshoff, A. Wiebe and S. R. Waldvogel, Angew. Chem., Int. Ed., 2018, 57, 6018 CrossRef
;
(g) G. S. Sauer and S. Lin, ACS Catal., 2018, 8, 5175 CrossRef CAS
;
(h) K. D. Moeller, Chem. Rev., 2018, 118, 4817 CrossRef CAS
;
(i) N. Sauermann, T. H. Meyer, Y. A. Qiu and L. Ackermann, ACS Catal., 2018, 8, 7086 CrossRef CAS
;
(j) C. Ma, P. Fang and T.-S. Mei, ACS Catal., 2018, 8, 7179 CrossRef CAS
;
(k) M. D. Kärkäs, Chem. Soc. Rev., 2018, 47, 5786 RSC
;
(l) S. R. Waldvogel, S. Lips, M. Selt, B. Riehl and C. J. Kampf, Chem. Rev., 2018, 118, 6706 CrossRef CAS
;
(m) R. Francke and D. Little, ChemElectroChem, 2019, 6, 4373 CrossRef CAS
;
(n) C. Song, K. Liu, X. Dong, C.-W. Chiang and A. Lei, Synlett, 2019, 30, 1149 CrossRef CAS
;
(o) T. Noël, Y. Cao and G. Laudadio, Acc. Chem. Res., 2019, 52, 2858 CrossRef
;
(p) P. Xiong and H.-C. Xu, Acc. Chem. Res., 2019, 52, 3339 CrossRef CAS
;
(q) F. Marken and J. D. Wadhawan, Acc. Chem. Res., 2019, 52(12), 3325 CrossRef CAS
;
(r) M. Elsherbini and T. Wirth, Acc. Chem. Res., 2019, 52(12), 3287 CrossRef CAS
;
(s) Y. Yuan and A. Lei, Acc. Chem. Res., 2019, 52(12), 3309 CrossRef CAS
;
(t) J. Chen, S. Lv and S. Tian, ChemSusChem, 2019, 12, 115 CrossRef CAS
;
(u) X. Han, K. Wang, W. Gao and J. Chen, Adv. Synth. Catal., 2019, 361, 2804 CrossRef CAS
;
(v) S. Lv, G. Zhang, J. Chen and W. Gao, Adv. Synth. Catal., 2019, 362, 462 CrossRef
;
(w) M. C. Leech and K. Lam, Acc. Chem. Res., 2020, 53(1), 121 CrossRef CAS
;
(x) K.-J. Jiao, Y.-K. Xing, Q.-L. Yang, H. Qiu and T.-S. Mei, Acc. Chem. Res., 2020, 53(2), 300 CrossRef CAS
;
(y) T. Fuchigami and T. Fuchigami, Acc. Chem. Res., 2020, 53, 322 CrossRef CAS
. - A. O. Terent'ev, O. M. Mulina, D. A. Pirgach, A. I. Ilovaisky, M. A. Syroeshkin, N. I. Kapustina and G. I. Nikishin, Tetrahedron, 2017, 73, 6871 CrossRef
. - For examples, see:
(a) R. Breslow, Acc. Chem. Res., 1991, 24, 159 CrossRef CAS
;
(b) U. M. Lindström, Chem. Rev., 2002, 102, 2751 CrossRef
;
(c) M. C. Pirrung and K. D. Sarma, J. Am. Chem. Soc., 2004, 126, 444 CrossRef CAS
;
(d) R. Breslow, Acc. Chem. Res., 2004, 37, 471 CrossRef CAS
;
(e) S. Narayan, J. Muldoon, M. G. Finn, V. V. Fokin, H. C. Kolb and K. B. Sharpless, Angew. Chem., Int. Ed., 2005, 44, 3275 CrossRef CAS
;
(f) C. J. Li and L. Chen, Chem. Soc. Rev., 2006, 35, 68 RSC
;
(g) N. Mase, Y. Nakai, N. Ohara, H. Yoda, K. Takabe, F. Tanaka and C. F. Barbas III, J. Am. Chem. Soc., 2006, 128, 734 CrossRef CAS
;
(h) Z.-L. Shen, S.-Y. Wang, Y.-K. Chok, Y.-H. Xu and T.-P. Loh, Chem. Rev., 2013, 113, 271 CrossRef CAS
;
(i) M. B. Gawande, V. D. B. Bonifacio, R. Luque, P. S. Branco and R. S. Varma, Chem. Soc. Rev., 2013, 42, 5522 RSC
;
(j) B. Li and P. H. Dixneuf, Chem. Soc. Rev., 2013, 42, 5744 RSC
;
(k) A. Dhakshinamoorthy, A. M. Asiri and H. Garcia, Chem. Commun., 2014, 50, 12800 RSC
. -
(a) Y.-L. Lai and J.-M. Huang, Org. Lett., 2017, 19, 2022 CrossRef CAS
;
(b) Y.-L. Lai, J.-S. Ye and J.-M. Huang, Chem.–Eur. J., 2016, 22, 5425 CrossRef CAS
. - Y. Zhao, Y.-L. Lai, K.-S. Du, D.-Z. Lin and J.-M. Huang, J. Org. Chem., 2017, 82, 9655 CrossRef CAS
. -
(a) X. Gao, G. Yuan, H. Chen, H. Jiang, Y. Li and C. Qi, Electrochem. Commun., 2013, 34, 242 CrossRef CAS
;
(b) H. Huang, G. Yuan, X. Li and H. Jiang, Tetrahedron Lett., 2013, 54, 7156 CrossRef CAS
;
(c) G. Yuan, Z. Zhu, X. Gao and H. Jiang, RSC Adv., 2014, 4, 24300 RSC
. -
(a) O. M. Mulina, N. V. Zhironkina, S. A. Paveliev, D. V. Demchuk and A. O. Terent’ev, Org. Lett., 2020, 22, 1818 CrossRef CAS
;
(b) H.-D. Zuo, W.-J. Hao, C.-F. Zhu, C. Guo, S.-J. Tu and B. Jiang, Org. Lett., 2020, 22, 4471 CrossRef CAS
. -
(a) S. Yamada, D. Morizono and K. Yamamoto, Tetrahedron Lett., 1992, 33, 4329 CrossRef CAS
;
(b) Y.-C. Luo, X.-J. Pan and G.-Q. Yuan, Tetrahedron, 2015, 71, 2119 CrossRef CAS
;
(c) Y. Yuan, Y. Cao, Y. Lin, Y. Li, Z. Huang and A. Lei, ACS Catal., 2018, 8, 10871 CrossRef CAS
;
(d) Z.-Y. Mo, T. R. Swaroop, W. Tong, Y.-Z. Zhang, H.-T. Tang, Y.-M. Pan, H.-B. Sun and Z.-F. Chen, Green Chem., 2018, 20, 4428 RSC
;
(e) Y.-Z. Zhang, Z.-Y. Mo, H.-S. Wang, X.-A. Wen, H.-T. Tang and Y.-M. Pan, Green Chem., 2019, 21, 3807 RSC
;
(f) Y. J. Kim and D. Y. Kim, Tetrahedron Lett., 2019, 60, 1287 CrossRef CAS
;
(g) K. Mahanty, D. Maiti and S. D. Sarkar, J. Org. Chem., 2020, 85, 3699 CrossRef CAS
;
(h) T.-J. He, W.-Q. Zhong and J.-M. Huang, Chem. Commun., 2020, 56, 2735 RSC
;
(i) Z.-Y. Mo, Y.-Z. Zhang, G.-B. Huang, X.-Y. Wang, Y.-M. Pan and H.-T. Tang, Adv. Synth. Catal., 2020, 362, 2160 CrossRef
.
Footnotes |
† Electronic supplementary information (ESI) available. See DOI: 10.1039/d0ra07212e |
‡ These authors contributed equally. |
|
This journal is © The Royal Society of Chemistry 2020 |