DOI:
10.1039/D0RA06671K
(Paper)
RSC Adv., 2020,
10, 32211-32215
Copper(II)-catalyzed tandem cyclization for the synthesis of benzo[d][1,3]thiazin-2-yl phosphonates involving C–P and C–S bond formation†
Received
2nd August 2020
, Accepted 13th August 2020
First published on 1st September 2020
Abstract
A copper(II)-catalyzed, high-efficiency and atom-economical synthesis of valuable organophosphorus compounds via tandem cyclization of o-alkynylphenyl isothiocyanates with phosphites is described. This protocol, having a good functional-group compatibility, provides a simple and direct pathway to organophosphorus heterocycles in good yields under mild conditions. The method could be efficiently scaled up to gram scale, thus providing a potential application of this cascade cyclization strategy in synthesis.
As the valuable precursors of many biologically active molecules,1 organophosphorus compounds have wide applications in the field of materials science,2 medicinal chemistry,3 organic synthesis,4 natural products,5 and ligand chemistry.6 For example, α-amino and α-hydroxy phosphonic acids have been found to act as antibiotics,7 antitumor agents,8 and enzyme inhibitors.9 Therefore, the synthesis of these organophosphorus compounds is still appealing. The construction of a C(sp2)–P bond on heterocycles is one of the most fundamental methods to synthesize the organophosphorus compounds. Through the efforts of many chemists, several extensively valuable methods have been established and developed.10 For instance, the Duan group and the Ackermann group developed an Ag-mediated C–H/P–H functionalization method to construct a Csp2–P bond by using arylphosphine oxides and internal alkynes as the substrates (Scheme 1a).11 At the same time, Studer and co-workers reported a novel Ag-catalyzed radical cascade reaction for the synthesis of 6-phosphorylated phenanthridines from 2-isocyanobiphenyls and diphenylphosphine oxides (Scheme 1b).12 Recently, Liang and co-workers also developed two cases of cascade functionalization of N-(p-methoxyaryl)-propiolamides and alkynol substrates with diphenylphosphine oxides to construct phosphorylated heterocycles (Scheme 1c and d).13 Although various utilized methods for the construction of Csp2–P bond on heterocycles have been established, the development of a new synthetic strategy from easily prepared starting materials is still a challenging task.
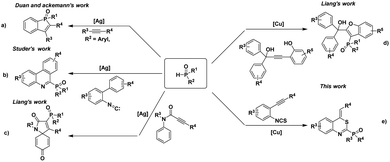 |
| Scheme 1 Synthesis of P-containing heterocycles through Csp2–P bond formation. (a) Previous report through C–H/P–H functionalization. (b) Previous report through radical process. (c) and (d) Previous reports through cascade functionlization. (e) This work: copper-catalyzed cyclization of o-alkynylphenyl isothiocyanates with phosphites. | |
Recently, transition-metal-catalyzed cascade cyclization of o-alkynylphenyl isothiocyanates with various nucleophiles provides a new and powerful synthetic strategy to synthesize different heterocycles. o-Alkynylphenyl isothiocyanates have extensively been used as versatile organic synthons for the construction of different compounds such as indoles,14 quinoline,15 thiazine16 due to its high reactivity and easy preparation. Encouraged by this fascinating research and our continuing interest in the transformation of o-alkynylphenyl isothiocyanates,17 we herein report an efficient copper-catalyzed cyclization of o-alkynylphenyl isothiocyanates with phosphites for the synthesis of phosphorylated heterocycles and related derivatives (Scheme 1e).
According to the literature procedure,14c,18 the starting o-alkynylphenyl isothiocyanates were prepared via the Sonogashira coupling of 2-iodoanilines with terminal alkynes,19 followed by reacting with thiophosgene. At the outset, we used o-phenylethynylphenyl isothiocyanate 1a and diethyl phosphonate 2 as the substrates in a model reaction to optimize the conditions, and the results are summarized in Table 1. Firstly, different copper salts (20 mol%) were screened in the presence of Cs2CO3 (2.0 equiv.) used as the base in MeCN (2 mL) at 80 °C for 18 h (Table 1, entries 1–8). CuCl2 was the best choice, leading to the desired product 3a in 41% yield. It is worth noting that trace amounts of the products were obtained in the absence of metal salts (Table 1, entry 9) and no product was obtained in the absence of base (Table 1, entry 10). These results indicated that the combination of a Lewis acid catalyst and a base is indispensable to afford the target product. Subsequently, we examined the base effect on the reaction (Table 1, entries 11–15). Lower yields were observed when other bases such as K2CO3, K3PO4, t-BuOK, NaOH, and Et3N were employed, whereas DBU gave the best yield (Table 1, entry 15). We next examined the solvent effect (Table 1, entries 16–21). When DCM was employed as the solvent, the highest yield of 60% was obtained (Table 1, entry 20). Finally, we examined the effect of temperature on the reaction. When the reaction temperature was reduced to 45 °C, the reaction was completed with a yield of 75% (Table 1, entry 23). Increasing the reaction temperature to 100 °C or reducing the reaction temperature to 25 °C resulted in a diminished yield (Table 1, entries 22 and 24).
Table 1 Initial studies for the tandem reaction of o-alkynylphenyl isothiocyanate 1a with phosphite 2aa
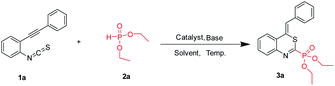
|
Entry |
Catalyst |
Base |
Solvent |
Yieldb (%) |
Reaction was performed with 1a (0.2 mmol), 2a (0.6 mmol), catalyst (0.04 mmol), base (0.6 mmol), in solvent (2 mL) at 80 °C for 18 h. Isolated yield based on o-phenylethynylphenyl isothiocyanate 1a. The temperature is 100 °C. The temperature is 45 °C. The temperature is 25 °C. |
1 |
CuI |
Cs2CO3 |
MeCN |
25 |
2 |
CuBr |
Cs2CO3 |
MeCN |
35 |
3 |
CuCl |
Cs2CO3 |
MeCN |
39 |
4 |
Cu(OTf)2 |
Cs2CO3 |
MeCN |
20 |
5 |
Cu(OAc)2 |
Cs2CO3 |
MeCN |
15 |
6 |
CuO |
Cs2CO3 |
MeCN |
Trace |
7 |
CuCl2 |
Cs2CO3 |
MeCN |
41 |
8 |
CuBr2 |
Cs2CO3 |
MeCN |
39 |
9 |
— |
Cs2CO3 |
MeCN |
Trace |
10 |
CuCl2 |
— |
MeCN |
NR |
11 |
CuCl2 |
K3PO4 |
MeCN |
45 |
12 |
CuCl2 |
t-BuOK |
MeCN |
28 |
13 |
CuCl2 |
NaOH |
MeCN |
32 |
14 |
CuCl2 |
Et3N |
MeCN |
Trace |
15 |
CuCl2 |
DBU |
MeCN |
50 |
16 |
CuCl2 |
DBU |
DMF |
31 |
17 |
CuCl2 |
DBU |
1,4-Dioxane |
45 |
18 |
CuCl2 |
DBU |
THF |
49 |
19 |
CuCl2 |
DBU |
Toluene |
42 |
20 |
CuCl2 |
DBU |
DCM |
60 |
21 |
CuCl2 |
DBU |
DCE |
45 |
22c |
CuCl2 |
DBU |
DCM |
25 |
23d |
CuCl2 |
DBU |
DCM |
75 |
24e |
CuCl2 |
DBU |
DCM |
Trace |
In order to further demonstrate the substrate scope, different o-alkynylphenyl isothiocyanates and phosphites were then explored; the results are summarized in Table 2. All reactions proceeded smoothly, leading to the desired 4H-benzo[d][1,3]thiazin-2-yl phosphonate in moderate to good yields. For example, the substituents on the R2 position of substrates 1 showed obvious electronic effects on the reaction. Compared with the substrates 1 with an electron-rich aryl group such as p-MeOC6H4 and p-MeC6H4 at the R2 position, the reaction of the R2 group in the substrates 1 bearing an electron-deficient aryl, such as p-FC6H4, p-ClC6H4, and p-BrC6H4, could lead to the desired products (3b–3d) in lower yields. Surprisingly, no desired products were obtained when the R2 group was an alkyl group, such as methyl, ethyl, n-butyl, t-butyl, and n-hexyl. However, when the R2 group in the substrate 1 was the cyclopropyl group, the desired 4H-benzo[d][1,3]thiazin-2-yl phosphonate was obtained in 52% yield. Electronic properties and substitution position on the benzene ring of substrate 1 did not hamper the reaction process. With both of electron withdrawing groups, such as F-, Cl-, Br-, CF3-, and electron-donating group Me- on the benzene ring, the reactions could afford desired products 3h–3o in moderate to good yields. The other two substitution products 3p and 3q were also obtained successfully under standard conditions. Diphenylphosphine oxide was also a suitable substrate for this cyclization, by reacting with 1 under the standard conditions, the corresponding products (Z)-(4-benzylidene-4H-benzo[d][1,3]thiazin-2-yl)diphenylphosphine oxide 3s and (Z)-(4-benzylidene-6-bromo-4H-benzo[d][1,3]thiazin-2-yl)diphenylphosphine oxide 3t were obtained in 60% yield and 52% yield, respectively. The structure of 3t was further confirmed using X-ray diffraction analysis (see Fig. S2 in the ESI†). Due to a kinetic effect according to Baldwin's rules and a smaller steric effect compared to the E-isomer, all products were uniformly formed as the Z-isomer.20 It is worth mentioning that all these reactions could be efficiently scaled up to gram scale under the optimal conditions, providing a potential application in the synthesis industry.
Table 2 Substrate scope of different o-alkynylphenyl isothiocyanates and phosphitesa,b
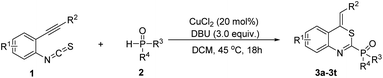
|
Reaction was performed with o-alkynylphenyl isothiocyanate 1 (0.2 mmol), phosphite or diphenylphosphine 2 (0.6 mmol), CuCl2 (0.04 mmol), DBU (0.6 mmol) in DCM (2 mL) under 45 °C for 18 h. Isolated yield based on o-alkynylphenyl isothiocyanate 1. |
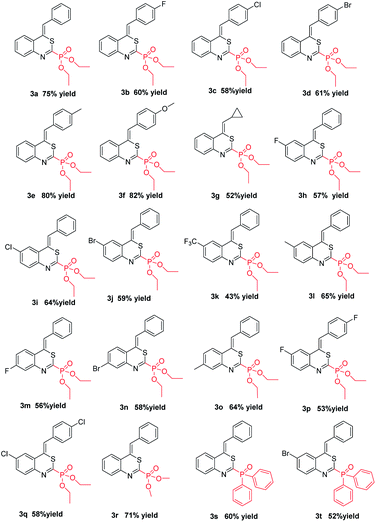 |
The structure of (Z)-(4-benzylidene-6-bromo-4H-benzo[d][1,3]thiazin-2-yl)diphenylphosphine oxide was corroborated by X-ray diffraction analysis of the crystal structure of 3t, the ORTEP diagram of which is displayed in Fig. 1.
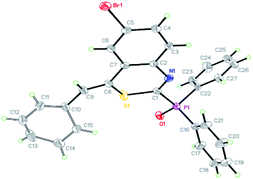 |
| Fig. 1 Single-crystal X-ray diffraction structure of 3t, the thermal ellipsoids are at the 30% probability level, the CCDC number is 2014442.† | |
Next, we examined the reaction of 2-isothiocyanato-3-(phenylethynyl)pyridine with 2a under the standard conditions (Scheme 2), the corresponding product diethyl (Z)-(4-benzylidene-4H-pyrido[2,3-d][1,3]thiazin-2-yl)phosphonate (3u) was obtained in 42% yield.
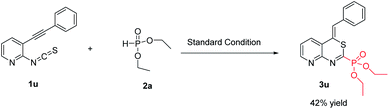 |
| Scheme 2 The reaction of 2-isothiocyanato-3-(phenylethynyl)pyridine with 2a. | |
In order to insight into the reaction mechanism more clearly, two radical control experiments were carried out. The reaction proceeded smoothly by using the radical scavenger 2,2,6,6-tetramethyl-1-piperidinyl-oxy (TEMPO) or butylated hydroxytoluene (BHT) probably suggesting that the reaction may not undergo a radical pathway (Scheme 3).
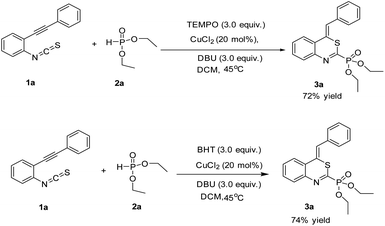 |
| Scheme 3 Control experiments. | |
Taking the experimental results into account, a possible mechanism was proposed, which is shown in Scheme 4. Firstly, in the presence of a base, isothiocyanate moiety in compound 1 was attacked by phosphite to produce the intermediate A. Intermediate A could then undergo isomerization to afford intermediate B. Next, the alkyne moiety of intermediate B was activated by the copper species which was then attacked by the sulfur anion through 6-exo-dig cyclization, leading to the intermediate C. Finally, intermediate C underwent protonolysis to give the target product 3.
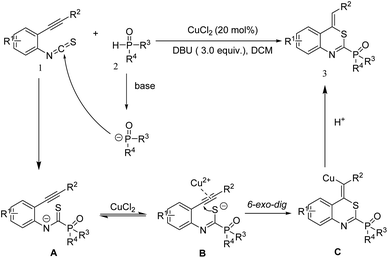 |
| Scheme 4 Proposed mechanism. | |
Conclusions
In summary, we have developed an efficient method for the synthesis of 4H-benzo[d][1,3]thiazin-2-yl phosphonates via the copper(II)-catalyzed tandem cyclization of o-alkynylphenyl isothiocyanates and phosphites. In this reaction, a series of organophosphorus heterocycles could be synthesized in good yields involving C–P and C–S bond formation in one pot. This present cascade cyclization strategy represented an effective way to construct phosphorus-containing small molecular N,S-heterocycles.
Conflicts of interest
There are no conflicts to declare.
Acknowledgements
The financial support from the Natural Science Foundation of China (NSFC) (grant number 21762023), the Natural Science Foundation of Jiangxi Province of China (2018BAB203006) and the Scientific Research Fund of Jiangxi Provincial Education Department (grant number GJJ160285) is gratefully acknowledged.
Notes and references
- The Role of Phosphonates in Living Systems, ed. R. L. Hildebrand, CRC Press, Boca Raton, FL, 1983 Search PubMed.
-
(a) V. Spampinato, N. Tuccitto, S. Quici, V. Calabrese, G. Marletta, A. Torrisi and A. Licciardello, Langmuir, 2010, 26, 8400 CrossRef CAS PubMed;
(b) S. Kirumakki, J. Huang, A. Subbiah, J. Y. Yao, A. Rowland, B. Smith, A. Mukherjee, S. Samarajeewa and A. Clearfield, J. Mater. Chem., 2009, 19, 2593 RSC;
(c) A. George and A. Veis, Chem. Rev., 2008, 108, 4670 CrossRef CAS PubMed;
(d) E.-Q. Li and Y. Huang, Chem. Commun., 2020, 56, 680 RSC.
-
(a) X. Q. Chen, D. J. Kopecky, J. Mihalic, S. Jeffries, X. S. Min, J. Heath, J. Deignan, S. J. Lai, Z. Fu, C. Guimaraes, S. L. Shen, S. Li, S. Johnstone, S. Thibault, H. D. Xu, M. Cardozo, W. Shen, N. Walker, F. Kayser and Z. L. Wang, J. Med. Chem., 2012, 55, 3837 CrossRef CAS PubMed;
(b) Q. Dang, Y. Liu, D. K. Cashion, S. R. Kasibhatla, T. Jiang, F. Taplin, J. D. Jacintho, H. Q. Li, Z. L. Sun, Y. Fan, J. DaRe, F. Tian, W. Y. Li, T. Gibson, R. Lemus, P. D. van Poelje, S. C. Potter and M. D. Erion, J. Med. Chem., 2011, 54, 153 CrossRef CAS PubMed;
(c) T. S. Kumar, S.-Y. Zhou, B. V. Joshi, R. Balasubramanian, T. H. Yang, B. T. Liang and K. A. Jacobson, J. Med. Chem., 2010, 53, 2562 CrossRef CAS PubMed;
(d) L. Bialy and H. Waldmann, Angew. Chem., Int. Ed., 2005, 44, 3814 CrossRef CAS PubMed.
-
(a) A. R. Choudhury and S. Mukherjee, Adv. Synth. Catal., 2013, 355, 1989 CrossRef CAS;
(b) D. P. Zhao and R. Wang, Chem. Soc. Rev., 2012, 41, 2095 RSC;
(c) T. Baumgartner and R. Reau, Chem. Rev., 2006, 106, 4681 CrossRef CAS PubMed;
(d) W. J. Tang and X. M. Zhang, Chem. Rev., 2003, 103, 3029 CrossRef CAS PubMed;
(e) G. Chelucci, G. Orru and G. A. Pinna, Tetrahedron, 2003, 59, 9471 CrossRef CAS;
(f) A. K. Bhattacharya and G. Thyagarajan, Chem. Rev., 1981, 81, 415 CrossRef CAS.
- S.-J. Tan, J.-L. Lim, Y.-Y. Low, K.-S. Sim, S.-H. Lim and T.-S. Kam, J. Nat. Prod., 2014, 77, 2068 CrossRef CAS PubMed.
-
(a) H. Fernandez-Pérez, P. Etayo, A. Panossian and A. Vidal-Ferran, Chem. Rev., 2011, 111, 2119 CrossRef PubMed;
(b) M. N. Birkholz, Z. Freix and P. W. N. M. van Leeuwen, Chem. Soc. Rev., 2009, 38, 1099 RSC.
- F. R. Atherton, C. H. Hassall and R. W. Lambert, J. Med. Chem., 1986, 29, 29 CrossRef CAS PubMed.
- G. Lavielle, P. Hautefaye, C. Schaeffer, J. A. Boutin, C. A. Cudennec and A. Pierre, J. Med. Chem., 1991, 34, 1998 CrossRef CAS PubMed.
- R. Hirschmann, A. B. Smith III, C. M. Taylor, P. A. Benkovic, S. D. Taylor, K. M. Yager, P. A. Sprengler and S. J. Benkovic, Science, 1994, 265, 234 CrossRef CAS PubMed.
-
(a) J.-L. Montchamp, Acc. Chem. Res., 2014, 47, 77 CrossRef CAS PubMed;
(b) B. Yang, T.-T. Yang, X.-A. Li, J.-J. Wang and S.-D. Yang, Org. Lett., 2013, 15, 5024 CrossRef CAS PubMed;
(c) C. K. Li, T. Yano, N. Ishida and M. Murakami, Angew. Chem., Int. Ed., 2013, 52, 9801 CrossRef CAS PubMed;
(d) C.-G. Feng, M. C. Ye, K.-J. Xiao, S. H. Li and J.-Q. Yu, J. Am. Chem. Soc., 2013, 135, 9322 CrossRef CAS PubMed;
(e) C. D. Hou, Y. L. Ren, R. Lang, X. X. Hu, C. G. Xia and F. W. Li, Chem. Commun., 2012, 48, 5181 RSC;
(f) Y. X. Gao, G. Wang, L. Chen, P. X. Xu, Y. F. Zhao, Y. B. Zhou and L.-B. Han, J. Am. Chem. Soc., 2009, 131, 7956 CrossRef CAS PubMed;
(g) K. S. Petrakis and T. L. Nagabhushan, J. Am. Chem. Soc., 1987, 109, 2831 CrossRef CAS;
(h) K. Moonen, I. Laureyn and C. V. Stevens, Chem. Rev., 2004, 104, 6177 CrossRef CAS PubMed;
(i) S. V. Jeught and C. V. Stevens, Chem. Rev., 2009, 109, 2672 CrossRef PubMed;
(j) M. Haji, Beilstein J. Org. Chem., 2016, 12, 1269 CrossRef CAS PubMed;
(k) L. Chen, X.-Y. Liu and Y.-X. Zhou, Adv. Synth. Catal., 2020, 362, 1724 CrossRef CAS.
-
(a) Y.-R. Chen and W.-L. Duan, J. Am. Chem. Soc., 2013, 135, 16754 CrossRef CAS PubMed;
(b) W. B. Ma and L. Ackermann, Synthesis, 2014, 46, 2297 CrossRef CAS.
- B. Zhang, C. G. Daniliuc and A. Studer, Org. Lett., 2014, 16, 250 CrossRef CAS PubMed.
- L.-J. Wang, A.-Q. Wang, Y. Xia, X.-X. Wu, X.-Y. Liu and Y.-M. Liang, Chem. Commun., 2014, 50, 13998 RSC.
-
(a) L. Benati, G. Calestani, R. Leardini, M. Minozzi, D. Nanni, P. Spagnolo, S. Strazzari and G. Zanardi, J. Org. Chem., 2003, 68, 3454 CrossRef CAS PubMed;
(b) M. Minozzi, D. Nanni, G. Zanardi and G. Calestani, ARKIVOC, 2006, 6, 6 Search PubMed;
(c) T. Saito, H. Nihei, T. Otani, T. Suyama, N. Furukawa and M. Saito, Chem. Commun., 2008, 172 RSC;
(d) J. L. Xie, Z. L. Guo, Y. Q. Huang, Y. Qu, H. J. Song, H. B. Song, Y. X. Liu and Q. M. Wang, Adv. Synth. Catal., 2019, 361, 490 CrossRef CAS;
(e) L.-R. Wen, Q.-Y. Shen, W.-S. Guo and M. Li, Org. Chem. Front., 2016, 3, 870 RSC.
-
(a) T. Otani, S. Kunimatsu, H. Nihei, Y. Abe and T. Saito, Org. Lett., 2007, 9, 5513 CrossRef CAS PubMed;
(b) T. Otani, S. Kunimatsu, T. Takahashi, H. Nihei and T. Saito, Tetrahedron Lett., 2009, 50, 3853 CrossRef CAS.
-
(a) M. Kaname and H. Sashida, Tetrahedron
Lett., 2012, 53, 748 CrossRef CAS;
(b) H. Sashida, M. Kaname and M. Minoura, Tetrahedron, 2013, 69, 6478 CrossRef CAS.
-
(a) W. Y. Hao, J. Tian, W. Li, R. Y. Shi, Z. L. Huang and A. W. Lei, Chem.–Asian J., 2016, 11, 1664 CrossRef CAS PubMed;
(b) W. Y. Hao, Y. Y. Jiang and M. Z. Cai, J. Org. Chem., 2014, 79, 3634 CrossRef CAS PubMed;
(c) W. Y. Hao, J. B. Zeng and M. Z. Cai, Chem. Commun., 2014, 50, 11686 RSC;
(d) W. Y. Hao, J. Huang, S. S. Jie and M. Z. Cai, Eur. J. Org. Chem., 2015, 6655 CrossRef CAS;
(e) W. Y. Hao, T. L. Zhang and M. Z. Cai, Tetrahedron, 2013, 69, 9219 CrossRef CAS;
(f) W. Y. Hao, X. Y. Sang, J. Jiang and M. Z. Cai, Tetrahedron Lett., 2016, 57, 1511 CrossRef CAS;
(g) W. Y. Hao, X. Y. Sang, J. Jiang and M. Z. Cai, Tetrahedron Lett., 2016, 57, 4207 CrossRef CAS;
(h) W. Y. Hao, Y. C. Sha, Y. Deng, Y. Luo, L. Zeng, S. Tang, Y. Weng, C.-W. Chiang and A. W. Lei, Chem.–Eur. J., 2019, 25, 4931 CrossRef CAS PubMed;
(i) F. H. Zou, X. W. Chen and W. Y. Hao, Tetrahedron, 2017, 73, 758 CrossRef CAS;
(j) J. K. Miao, Y. H. Zhang, X. Y. Sang and W. Y. Hao, Org. Biomol. Chem., 2019, 17, 2336 RSC.
- L. Benati, G. Calestani, R. Leadini, M. Minozzi, D. Nanni, P. Spagnolo, S. Stazzari and G. Zanardi, J. Org. Chem., 2003, 68, 3454 CrossRef CAS PubMed.
-
(a) K. Sonogashira, Y. Tohda and N. Hagiwara, Tetrahedron Lett., 1975, 16, 4467 CrossRef;
(b) K. Sonogashira, J. Organomet. Chem., 2002, 653, 46 CrossRef CAS.
-
(a) M. Uchiyama, H. Ozawa, K. Takuma, Y. Matsumoto, M. Yonehara, K. Hiroya and T. Sakamoto, Org. Lett., 2006, 8, 5517 CrossRef CAS PubMed;
(b) J. H. Park, S. V. Bhilare and S. W. Youn, Org. Lett., 2011, 13, 2228 CrossRef CAS PubMed;
(c) K. Gilmore and I. V. Alabugin, Chem. Rev., 2011, 111, 6513 CrossRef CAS PubMed.
Footnote |
† Electronic supplementary information (ESI) available. CCDC 2014442. For ESI and crystallographic data in CIF or other electronic format see DOI: 10.1039/d0ra06671k |
|
This journal is © The Royal Society of Chemistry 2020 |