DOI:
10.1039/D0RA05742H
(Paper)
RSC Adv., 2020,
10, 28114-28120
Influence of flaxseed flour as a partial replacement for wheat flour on the characteristics of Chinese steamed bread†
Received
1st July 2020
, Accepted 22nd July 2020
First published on 28th July 2020
Abstract
This study investigated the effects of partially replacing wheat flour with flaxseed flour (FF) on the quality parameters of Chinese Steamed Bread (CSB). FF was utilized as a functional ingredient of CSB at varying levels. The pasting properties of flour blends, the rheological and microstructural characteristics of dough, the textural and quality characteristics and functional group structure of CSB were analyzed. Results showed that FF addition influenced the pasting characteristics of wheat flour by decreasing the final viscosity, breakdown and setback values, but had little effect on the rheological properties of the dough. The microstructure of the dough indicated that the disruption degree of the gluten matrix increased with the increase of FF. Besides, FF addition increased the hardness and chewiness of CSB, while decreasing the cohesiveness and springiness. Additional characteristic peaks were observed at 1745, 2854, and 3006 cm−1 and associated with –C
O, –CH2, and cis-C
CH bond stretching vibrations of flaxseed. Results suggested 12% FF exhibited the best acceptability.
Introduction
Chinese Steamed Bread (CSB), also known as “Mantou”, has been the staple food in China since ancient times and is a traditional fermented and steamed food, it is basically made from wheat flour, water, and yeast. The dough is proofed at 80% relative humidity and a temperature of 34 °C, and then steamed for 15 min in a steamer.1 CSB can be classified into many types based on the composition of basic ingredients and the production process, and it accounts for 40% of the wheat consumption in China.2,3 This product is widely consumed in northern China and has become popular around the world. However, the nutritional composition of pure wheat–CSB is not comprehensive and it is facing the challenge of developing novel CSB with special flavors and health-enhancing characteristics. To further improve the nutrient value and taste of CSB, there are reports on the addition of green tea powder, buckwheat, pitaya peel powder, oatmeal, and potato powder into CSB.1,4–8
Awareness about health and nutrition has driven consumer demand for functional foods. Among different healthy ingredients, flaxseed (Linum usitatissimum) contains functional food factors and bioactive substances, and it can be powdered and added into CSB, which could not only provide sufficient protein riching in essential amino acids, but also supply enough unsaturated lipids with high levels of polyunsaturated fatty acids especially α-linolenic acid (ALA). It is also an important source to meet people's demands for rich content of dietary fiber, lignans and others.9,10 Flaxseed contains functional nutrients that might inhibit the development of metabolic diseases, such as breast cancer and obesity.11 Its hydrolysate shows nutritional functions, such as anti-diabetic and antihypertensive effects.12 Flaxseed is increasingly developed as an important ingredient in functional foods due to its beneficial health-related effect.13
Nowadays, the application of flaxseed in products has been the topical subject of many studies, it can be incorporated into a range of products (e.g. bread, noodles, muffin, biscuits, cookies, dairy products) as ground flaxseed, flaxseed oil, flaxseed mucilage or as flaxseed hulls to improve the nutritional quality of foods.14–22 Flaxseed affects the quality characteristics of these products.14,23,24 Previous studies have reported effect of flaxseed addition on the flavor profile, antioxidant, nutritional and other characteristics of different food products. Flaxseed has increased aroma and flavor of muffin, reduced its sweetness, and optimized its component content and biological activity.20 As a dietary supplement, 10% flaxseed flour (FF) can be added into muffin without causing any adverse effects on its sensory properties.25 Adding FF to wheat bread has shown to improve its physicochemical properties and nutritional status and delay its aging.19,26 Research also indicates that it is possible to add up to about 15% ground linseed in dough and this has minor effects on the overall sensory acceptability of CSB.27 However, studies on the production of CSB added with FF reported were not enough so far. It is necessary to further study the effect of flaxseed addition on CSB's structure and quality.
This study utilized FF at varying levels as a functional ingredient of CSB. The effects of FF on pasting characteristics of flour blends, rheological and microstructural characteristic of dough, and textural and quality characteristics of CSB were studied. The component analysis was conducted via Fourier transform infrared spectroscopy (FTIR), and sensory evaluation was used to explore the effects of FF on the texture, flavour and general quality of CSB.
Materials and methods
Materials and reagents
Flaxseed CSB consisted of wheat flour, FF, distilled water, and yeast. Commercial wheat flour and fresh dry yeast were purchased from a local market in Yangling, Shaanxi Province, China (108°4′27.95E, 34°16′56.24′′N). Flaxseed, small red-brown seeds, was acquired from Huining County, Gansu Province, China (108°4′4.8′′E, 35°43′12′′N). Fatty acid compositions (FAC) of FF were analyzed by gas chromatography (Agilent Technologies, Palo Alto, CA, USA) equipped with an a flame ionization detector, autosampler injector, and a HP-INNOWAX capillary column (0.25 mm × 0.25 μm × 30 m). The injection port temperature and detector were maintained at 250 °C. The temperature of the column was at 180 °C for 2 min and gradually increased from 10 °C to 240 °C for 10 min. High-purity nitrogen was used as a carrier gas with a flow rate of 1.1 mL min−1, while nitrogen, hydrogen, and air were used as combustion gas. Wheat flour was passed through a 100 mesh (125 μm) sieve. Flaxseed was cleaned, ground into flour, and passed through a 40 mesh (425 μm) sieve to obtain FF, which was stored in a refrigerator for further analysis. The control sample (i.e., 100% wheat flour) and various blends of composite flour mixes were prepared by replacing wheat flour with FF. Glutaraldehyde, phosphoric acid, methanol, petroleum ether, potassium hydroxide and other reagents used in this study were purchased from Tianjin Chemical Company, Ltd. (Tianjin, China). All chemicals and reagents were of analytical grade.
Preparation of CSB
Wheat–flaxseed flour (1000 g) with different proportions of flour and FF. Based on the preliminary experimental results, the amount of FF can be added more than 20%. However, considering the color and softness of products, FF was added at 0 (control), 6%, 8%, 10%, 12%, and 14% of the total flour on a weight basis, distilled water (500 mL), and yeast (10 g) were used to produce FF–CSB. First, wheat flour and FF were mixed at low speed (50 rpm) for 5 min by using a Kitchen Aid mixer (Ashton Electric Co., Ltd., Zhejiang, China), and the yeast was activated with 300 mL of distilled water. All ingredients were mixed for 10 min: first at low speed for 3 min and then at medium speed (80 rpm) for the remaining time until smooth dough was formed. After mixing, the dough was fermented at 35 °C and 85% relative humidity for 90 min.
The dough samples were remixed, divided into pieces (50 g per dough), rounded, and molded. After proofing for 10 min, the dough was steamed at 100 °C and atmospheric pressure for 20 min. Each piece of CSB was freeze dried, ground and passed through a 100 mesh sieve for related analysis.
Analysis methods
Proximate composition. The CSB samples were prepared with a mixture of flour and FF in different proportions. The moisture, ash, fat, protein, falling number and crude fiber of both materials and CSB products were estimated using American Association of Cereal Chemists (AACC) methods 44-15A, 08-01, 30-10, 46-10, 56-81B and 32-25, respectively. The nitrogen content was determined using a Kjeltec™ auto protein tester (Kjeltec™ 8400, Foss Inc., Sweden) and protein calculated as (N × 6.25).
Pasting properties of flour blends
Pasting properties were measured with a rapid viscosity analyzer (TechMaster, Botong Instrument Company, Sweden) using the method described by Kaur and Singh.28 The six parameters recorded were peak, trough, breakdown, final, setback viscosities, and pasting temperature. Viscosity profiles were recorded by mixing 3 g of flour sample with 25 mL of distilled water.
Rheological properties of dough
The rheological properties of flour with FF were measured using Mixolab analyzer (Chopin, Tripette et Renaud, Paris, France) in accordance with the method of Codină and Mironeasa with little difference.29 Each mixture was blended with distilled water to produce 75 g of dough. Dual-mixing was conducted at 80 rpm, and the water tank temperature was maintained at 30 °C. Mixolab curve indicators were determined as follows: WA (%) – water absorption; C1 (Nm) – initial torque during mixing; C2 (Nm) – minimum torque while being subjected to mechanical and thermal constraints during mixing and initial heating; C3 (Nm) – peak torque during heating stage; C4 (Nm) –minimum torque during cooling to 50 °C; C5 (Nm) – final torque after cooling at 50 °C; (C3–C4) (Nm) – protein weakening; (C3–C4) (Nm) – starch breakdown; and (C5–C4) (Nm) – starch setback; DT (min) – development time, time required to reach torque of 1.1 Nm; mixing stability (min) – time during which the torque produced is at level of 1.1 Nm; α – protein weakening; β – starch gelatinization; γ – starch breakdown.
Tensile characteristics of dough and textural analysis of CSB
The textural characteristics of CSB and tensile characteristics of dough stretching were determined using texture profile analysis with a Texture Analyzer (TA.XT Plus, Stable Micro systems Ltd., England). Texture profile analysis (TPA) was performed to obtain the textural characteristics and measure the hardness, cohesiveness, springiness and chewiness of CSB on the basis of force–time curve as described by Goswami et al.30 CSB samples were cut with a slicer into square-shaped pieces measuring 5 cm on all sides and with approximate thickness of 2.5 cm from the bread crumb. The samples were compressed twice to 50% of their original height with a diameter stainless steel cylinder (P/36). Ten replicates were made for each sample.
Microstructure of dough
The microstructure of the dough samples with different FF ratios was analyzed using scanning electron microscope (SEM) via a Nova Nano-450 SEM (American Fei Inc., USA, Czech) at 5 kV accelerating potential. The dough was cut into approximately 5 × 5 × 5 mm pieces. The samples were prepared in accordance with the method of Ribotta et al.31 All samples were vacuum dried and then placed on the sample holder using double-sided adhesive tape, and exposed to gold sputtering. Micrographs were taken at 1500 × magnification.
Component analysis of CSB
The component of CSB was analyzed using attenuated total reflection-Fourier transform infrared spectroscopy (ATR-FTIR) spectra (Bruker VERTEX 70, Bruker Optics, Germany) with air as the background. ATR crystal was thoroughly cleaned with ethanol prior to measurement. For each sample, 16 scans were performed in absorption mode with 4 cm−1 spectral resolution. The FTIR spectra of the samples were obtained from 4000 cm−1 to 600 cm−1.
Sensory evaluation of CSB
CSB sensory analysis was conducted following the method of Zhu with some modifications due to the differences in the samples.3 Various quality parameters, namely, specific volume, appearance, color, internal structure, texture, chewing performance, and flavor were assigned with maximum scores of 20, 15, 10, 20, 15, 15, and 5, respectively. The overall quality score, which is 100 in total, was the sum of these quality attributes. Among them, specific volume was determined by rapeseed displacement method and other parameters were measured by sensory tests. A total of twenty panellists in the age group of 20–35 years were recruited. Before the formal evaluation, a systematic training was done on the panel in order to obtain objective results. The panellists were provided with fresh CSB with randomized codes at the same time for sensory analysis.
Statistical analysis
Experimental data reported in the tables were the average of triplicate observations unless otherwise specified, and the results were expressed as mean ± standard deviation (SD). Values marked with different letters were significantly different (p < 0.05). One-way ANOVA and Duncan's multiple-range tests were performed for mean comparison with SPSS 20 (SPSS Inc., Chicago, IL, USA). Statistical analysis was conducted using OriginPro 9 (Originlab, Northampton, MA, USA).
Results and discussion
Proximate composition
Wheat flour contains 8.38% protein and 0.46% ash, its falling number is 440 s. FF contains 35.03% fat, 13.54% protein and 5.41% fiber. FAC was determined by identifying and calculating the relative peak areas. It was known that FF contained a large amount of polyunsaturated fatty acid (69.4%) including linoleic acid (15.8%) and linolenic acid (53.6%). Gas chromatogram of fatty acid of FF is shown in Fig. S1.† Data on the proximate CSB composition prepared from the wheat flour and FF mixture are shown in Table S1.† When the FF content in the blend increased from 6% to 14%, ash, fat and protein contents of CSB were increased mostly because flaxseed already contains these nutrients. The fiber content of control CSB is 0.22%, which is significantly (p < 0.05) lower than that of the flaxseed-blended CSB with maximum fiber amount of 1.39%. The nutrients content of flaxseed-incorporated CSB was enhanced compared with that of non-flax CSB.
Pasting properties of wheat flour and FF blends
The pasting characteristics of wheat flour and FF blends are presented in Table 1, and the pasting curves of flour are shown in Fig. S2.† Pasting temperature indicates the minimum temperature required to cook the flour.32 The pasting temperature was almost the same among the flour samples. Compared with that of the control, the results obtained for the pasting temperature showed a negligible reducing trend with the incorporation of FF which were against expectation. Thus, wheat flour is more resistant to swelling and rupturing than FF. A similar reduction in pasting temperature was observed by Kaur and Kaur.25 The viscosity of all flour samples gradually increased with the temperature increase caused by gelatinization of the starch and water absorption of the protein. When the mixture was cooled, the viscosity increased probably due to the aggregation of amylose molecules.
Table 1 Pasting properties of flour blends of wheat flour and FFa
Samples |
PT (°C) |
PV (cP) |
TV (cP) |
FV (cP) |
BD (cP) |
SB (cP) |
Values are expressed as mean ± SD (n = 3). Values followed by letters in the same column are significantly different (p < 0.05). WF, wheat flour; FF, flaxseed flour; PT, pasting temperature; PV, peak viscosity; TV, trough viscosity; FV, final viscosity; BD, breakdown viscosity; SB, setback viscosity. |
Control |
89.23 ± 0.43a |
931.00 ± 5.00bc |
512.00 ± 11.00b |
1191.00 ± 14.00cd |
419.00 ± 6.00b |
679.00 ± 3.00c |
(WF 94% + 6% FF) |
87.23 ± 0.02b |
1076.00 ± 7.00a |
626.50 ± 7.50a |
1410.50 ± 8.50a |
449.50 ± 0.50a |
784.00 ± 1.00a |
(WF 92% + 8% FF) |
88.05 ± 0.00ab |
1004.50 ± 19.50ab |
591.00 ± 16.00ab |
1315.50 ± 27.50b |
413.50 ± 3.50b |
724.50 ± 11.50b |
(WF 90% + 10% FF) |
88.40 ± 0.40ab |
979.50 ± 31.50bc |
584.00 ± 21.00ab |
1275.00 ± 31.00bc |
395.50 ± 10.50bc |
691.00 ± 10.00c |
(WF 88% + 12% FF) |
88.00 ± 0.75ab |
974.00 ± 40.00bc |
602.50 ± 52.50ab |
1250.00 ± 43.00bcd |
371.50 ± 12.50cd |
647.50 ± 9.50d |
(WF 86% + 14% FF) |
87.68 ± 0.42ab |
911.50 ± 3.50c |
550.50 ± 0.50ab |
1182.50 ± 3.50d |
361.00 ± 3.00d |
632.00 ± 4.00d |
With the addition of FF increasing from 6% to 14%, peak viscosity and trough viscosity were observed a small change, while the final viscosity, breakdown and setback value of the blend with addition of FF were observed to a significant decrease. This finding is probably due to the mucilage water in flaxseed that led to gelation.25 As such, starch granules were insufficiently gelatinized and slowly expanded, thus decreasing the peak viscosity. This is attributed to the high flaxseed oil content. The reduction observed in final viscosity, breakdown and setback value of the flour blends was ascribed to the decrease in starch content as the addition of FF increased. Peak viscosity and trough viscosity of 12% FF blend were low but still higher than those of the control, however, its breakdown and setback values were also lower than those of the control, indicating the good thermal stability of blend at this level. The next step should be on studying the pasting characteristics and the retrogradation performance of CSB with a differential scanning calorimeter to provide guidance for its storage.
Rheological properties of dough
The wheat (control) and the mixture were characterized in terms of protein quality and thermo-mechanical behavior by using Mixolab device. The effects of different FF–wheat composite flour dough from Mixolab analysis are shown in Fig. S3,† and the main parameters are presented in Table S2.† The results indicate that with the increased level of FF addition in wheat flour, the water absorption decreased in general. These results are agreed with those obtained by Codină and Mironeasa,29 but in disagreement with the results obtained by Koca and Anil,33 Marpalle et al.19 It may be due to the difference of FF particle size and the fat content in FF, the larger particle size (therefore lower surface area) and the higher fat content of FF in comparison to that of wheat flour decreased the values of dough water absorption. The parameters of dough stability increased at low levels of FF addition and decreased at high ones. Besides, results showed that by FF addition, especially at high doses, the dough became weaker by a decrease of dough development time.
As the temperature increased, the protein aggregated and denatured, and the dough torque decreased. Wheat flour proteins with the highest C2 value showed slightly weakening due to mechanical and thermal constrains. The blend one had more protein reduction, resulting in low protein quality. With the increase temperature of the dough, the proteins begin to denature and changes of proteins became irrelevant, and starch granules play a predominant role to hold water which leading to the increased torque.29,34 The increase in torque is the result from swelling of the starch granules, which were ascribed to moisture absorption and amylose chain leaching into the aqueous intergranular phase.35 Further reduction in torque is the result from the disintegration of starch granules, attributing to mechanical shear stress and temperature constraints. Subsequently, starch denatures upon cooling, and its torque increases.
Compared with the blend one, wheat flour had higher starch gelatinization, which contributes to its good starch performance. The control had longer stability and greater resistance to mechanical restraint compared with other blends with FF. C3 decreased with the increase of FF levels addition to wheat flour. It is probably due to the fact that FF addition modified the starch gelatinization, which may be attributed to an increase in the protein content from the dough system, causing some discontinuities in the dough matrix and resulting in a decrease of torque.29 The reduction of C4 with FF addition may be due to the decrease of available water for the starch granules, which was caused by the combination of soluble fiber present in FF and water. Overall, the addition of FF had insignificant effect on the rheological properties of dough. The dough of flaxseed–wheat composite flour all exhibited low breakdown torques, final torques, setback torques, high cooking stability and consequently low starch retrogradation. This phenomenon could be helpful in CSB firming to extend its shelf life during storage as described by Collar et al.36
Tensile characteristics of dough and textural analysis of CSB
The tensile characteristics of dough and textural properties of CSB are shown in Table 2. The resistance to extension of dough showed a significantly (p < 0.05) reduction and the extensibility of control dough remained higher than that of flaxseed dough at all substitution levels. This is because gluten was diluted and starch interactions of CSB were disrupted by the incorporation of FF. The FF-induced strength reduction of dough became highly evident with the increasing of FF, and these results are consistent with those of Koca and Anil.33
Table 2 Tensile characteristics of dough and textural analysis of CSB supplemented with FFa
Samples |
Dough |
CSB |
Resistance to extension (R, BU) |
Extensibility (E, mm) |
Hardness (g) |
Cohesiveness |
Springiness |
Chewiness (g) |
Values are expressed as mean ± SD (n = 10). See Table 1 footnote for significance difference and abbreviations. |
Control |
83.31 ± 10.77b |
23.43 ± 2.46d |
1569.48 ± 93.71e |
0.820 ± 0.004a |
0.998 ± 0.012a |
1283.62 ± 87.38d |
(WF 94% + 6% FF) |
98.71 ± 16.44a |
18.32 ± 1.78 ab |
1753.12 ± 63.21d |
0.814 ± 0.002 ab |
0.995 ± 0.004 ab |
1419.03 ± 50.54c |
(WF 92% + 8% FF) |
94.22 ± 10.55a |
18.02 ± 1.82ab |
1845.90 ± 19.52cd |
0.809 ± 0.012b |
0.993 ± 0.006 ab |
1482.38 ± 11.22c |
(WF 90% + 10% FF) |
81.51 ± 8.73b |
19.89 ± 1.32c |
1985.19 ± 9.59c |
0.792 ± 0.001c |
0.952 ± 0.001bc |
1495.86 ± 18.25bc |
(WF 88% + 12% FF) |
63.53 ± 8.30c |
19.30 ± 1.41bc |
2213.06 ± 74.05b |
0.772 ± 0.003d |
0.941 ± 0.026 cd |
1607.68 ± 88.89b |
(WF 86% + 14% FF) |
54.41 ± 7.79d |
17.70 ± 1.94a |
2512.04 ± 109.09a |
0.769 ± 0.012d |
0.938 ± 0.004d |
1811.50 ± 90.81a |
The results showed that FF addition significantly increased the hardness (from 1569.48 g up to 2512.04 g) and chewiness (from 1283.62 g up to 1811.50 g) of CSB, but decreasing the cohesiveness (from 0.820 down to 0.769) and springiness (from 0.998 down to 0.938) (Table 2). Because the granule of FF was larger than that of wheat flour, it contributed to the weakness of wheat-based dough, thereby leading to increasing hardness. Besides, FF contains more fiber, lipids and proteins components, and these components can interact with gluten and starch and thus affect the formation of gluten network. The loss in dough structuring from diluting the gluten and starch matrix may reduce the gas holding capacity of dough, resulting in decreased specific volume. It also led to the increase of hardness and chewiness and the decrease of cohesiveness and springiness. Our results indicated that incorporating FF at varying levels adversely affects the texture of CSB. Similar result was also observed from Zhu and Li,27 where FF addition influenced the textural properties of CSB.
Microstructure of dough
The SEM of dough with varying levels of FF and wheat flour is presented in Fig. 1. The micrograph of the control dough containing only wheat flour showed a continuous gluten matrix with embedded starch granules (Fig. 1A). Fig. 1(B–F) are the micrographs of dough prepared with 6–14% FF, respectively. The gluten began to break, relatively large voids gradually appeared, and the network structure was not evident with FF increasing. When the proportion of FF increased, the wet gluten content of mixed powder decreased because gluten content is diluted and gluten structure formation is prevented.27 The dough network stability decreased probably due to the high content of lipids and cellulose from FF and due to the gluten dilution, taking into account that flaxseed contain low amount of gluten. The SEM micrographs further confirmed that FF has a strong dilution effect on gluten, reduces the strength of dough, and hinders the formation of gluten network structure.
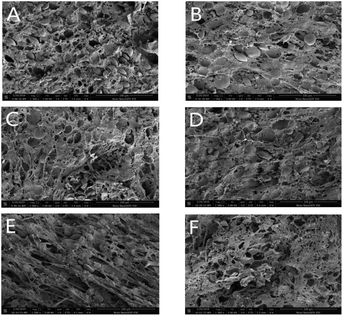 |
| Fig. 1 Microstructure of dough (1500×). (A) Control; (B) 6% FF; (C) 8% FF; (D) 10% FF; (E) 12% FF; (F) 14% FF. | |
Component analysis of CSB
Fig. 2 presents the FTIR patterns for CSB with FF from 4000–1500 cm−1. Several important absorbance bands can be identified as follows. The band in the 3700–3000 cm−1 region is attributed to vibrations of –OH groups owing to hydration of the roux paste.37 The peak at 3006 cm−1 is attributed to the stretching of cis-C
CH bond in unsaturated fatty acids; a high number of bonds indicates high peak intensity.38 The intensity of the –CH2 spectral components at approximately 2852 cm−1 increased as governed by the abundance of long-chain saturated fatty acids.39 The 1745 cm−1 band in the spectra reflects the absorption of lipids and is also related to lipid–starch complex formation. The methylene group in the 2886–2783 cm−1 range and the ester group in the 1793–1693 cm−1 range are the principal chemical groups of fatty acids.40
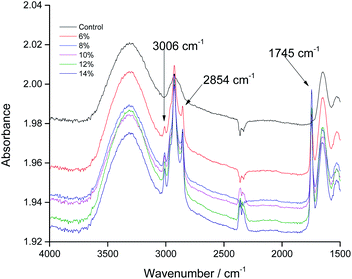 |
| Fig. 2 Spectra of FF–CSB. | |
Additional characteristic bands were observed at 1745, 2854, and 3006 cm−1 and associated with –C
O, –CH2, and cis-C
CH bond stretching vibrations of flaxseed, respectively, as compared with wheat. Considering that FF has high lipid content, the intensity of the ester group at the 1745 cm−1 increased. The increase in peak intensity at 2854 cm−1 is due to the abundance of long-chain fatty acids and that at 3006 cm−1 corresponds to the ALA content. Results were correlated with the proximate composition of CSB. It may be possible to determine the content of FF in food by measuring the intensity of characteristic absorption peaks.
Sensory evaluation of CSB
The sensory qualities of CSB were evaluated through sensory descriptive analysis, as shown in Fig. 3. Specific volume is one of the most important visual characteristics of steamed bread as well, strongly influencing the consumer's choice.41 The control group had a white crumb color, fine and uniform crumb grain size, soft texture, and wheat scent. Addition of FF affected sensory parameters of CSB to different extents. CSB darkened in color with the increasing FF incorporation. This darkening of crumb color was attributed to the influence of the original dark color of FF. FF had unfavorable effects on the internal structure and texture of the products, and its addition decreased the specific volume, while enhancing the flavor and chewing performance of CSB. FF–CSB had better aroma and nutty flavor than the control. FF contains high lipid content and might destroy the gluten network structure, thus, its addition may cause the formation of small and uneven pores which led to a lower appearance score than the control. Sensory analysis partially showed good agreement with textural analysis results. CSB with 12% FF addition was the highly acceptable with the highest overall quality score. Addition of FF had little effect on the results of sensory evaluation indexes. However, sensory evaluation was subjective, instrumental analysis tended to be more sensitive and thus should be combined. For example, the changes in color of CSB should be analyzed using a colorimeter combining consumer's preference through observation. The influence of FF addition on the aroma of CSB and the changes of fragrant substances can be discriminated by flavor analysis. The shelf-life properties of CSB during storage should also be studied since this process may have a great effect on the sensory quality of products. The consumer acceptance tests or questionnaires about consumers' preferences are also necessary to further promote the production of CSB added with FF for potential commercial applications.
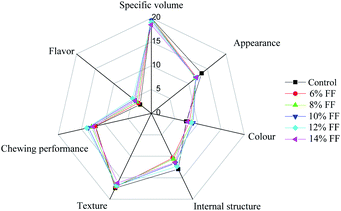 |
| Fig. 3 Radar charts of results for the sensory analysis of FF–CSB. | |
Conclusions
The increase of FF addition contributes to the high lipid content and the decrease in starch content. The mucilage water in FF led to gelation, and starch granules were insufficiently gelatinized and slowly expanded. They are probably the reasons for that increasing of FF affected the pasting behavior of wheat flour with a decrease in peak viscosity, final viscosity, breakdown values and setback values. And FF addition had little effect on the rheological properties of dough. The microstructure of dough indicated that the degree of gluten disruption increased with FF addition. The larger granule of FF contributed to the weakness of wheat-based dough. Fiber, lipids and proteins components of FF may interact with gluten and starch, and then affected the formation of gluten network. The loss in dough structuring from diluting the gluten and starch matrix may reduce the gas holding capacity of dough, resulting in decreased specific volume. In this way, it also led to the increase of hardness and chewiness and the decrease of cohesiveness and springiness of CSB. Addition of FF into CSB can exceed 20%, however, the overall acceptability of CSB determines its potential commercial value, and correlation results suggest that FF could be incorporated in CSB as a partial replacement up to 12% of wheat flour without causing detrimental effects on the physical and sensory properties. In summary, FF can be recommended as a dietary supplement due to its high ω-3 fatty acids especially ALA for its health benefits. This research could provide a basis for the application of FF in wheat-flour foods. CSB fortified with more FF should be taken into consideration, and further research on the influence of FF addition on nutritional quality, in vitro digestibility and antioxidant activity of CSB can be conducted to investigate for improved quality.
Abbreviations
FF | Flaxseed flour |
CSB | Chinese steamed bread |
ALA | α-Linolenic acid |
FTIR | Fourier transform infrared |
FAC | Fatty acid compositions |
AACC | American Association of Cereal Chemists |
SEM | Scanning electron microscope |
ATR | Attenuated total reflection |
SD | Standard deviation |
WF | Wheat flour |
PT | Pasting temperature |
PV | Peak viscosity |
TV | Trough viscosity |
FV | Final viscosity |
BD | Breakdown viscosity |
SB | Setback viscosity |
Conflicts of interest
Tingting Liu, Huping Duan, Xiaohui Mao and Xiuzhu Yu declare that they have no conflict of interest.
Acknowledgements
The authors would like to thank the Key R&D Program Projects of Shaanxi Province, China (2019NY-120) for the financial support.
References
- J. M. Ning, G. G. Hou, J. J. Sun, Z. Z. Zhang and X. C. Wan, Int. J. Food Sci. Technol., 2019, 54, 576–582 CrossRef CAS.
- Z. H. He, A. H. Liu, R. J. Pena and S. Rajaram, Euphytica, 2003, 131, 155–163 CrossRef CAS.
- F. Zhu, Food Chem., 2014, 163, 154–162 CrossRef CAS PubMed.
- X. N. Guo, S. Yang and K. X. Zhu, Food Chem., 2019, 283, 52–58 CrossRef CAS PubMed.
- C. T. Hsu, Y. H. Chang and S. Y. Shiau, Cereal Chem., 2019, 96, 76–85 CrossRef CAS.
- W. Liu, M. Brennan, L. Serventi and C. Brennan, Eur. Food Res. Technol., 2017, 243, 1105–1106 CrossRef CAS.
- Y. Liu, X. L. Wang, T. Ren, Z. Ma, L. Liu, X. P. Li, Q. B. Guo and X. Z. Hu, Cereal Chem., 2019, 96, 349–357 CrossRef CAS.
- Z. Zhao, T. Mu and H. Sun, Food Chem., 2019, 274, 710–717 CrossRef CAS PubMed.
- C. K. O. Dzuvor, J. T. Taylor, C. Acquah, S. Pan and D. Agyei, Molecules, 2018, 23, 2444 CrossRef PubMed.
- P. Kajla, A. Sharma and D. R. Sood, J. Food Sci. Technol., 2015, 52, 1857–1871 CrossRef CAS.
- K. K. Singh, D. Mridula, J. Rehal and P. Barnwal, Crit. Rev. Food Sci. Nutr., 2011, 51, 210–222 CrossRef CAS.
- A. Doyen, C. C. Udenigwe, P. L. Mitchell, A. Marette, R. E. Aluko and L. Bazinet, Food Chem., 2014, 145, 66–76 CrossRef CAS.
- Y. Y. Shim, B. Gui, Y. Wang and M. J. T. Reaney, Trends Food Sci. Technol., 2015, 43, 162–177 CrossRef CAS.
- K. Bialasova, I. Nemeckova, J. Kyselka, J. Stetina, K. Solichova and S. Horackova, Czech J. Food Sci., 2018, 36, 51–56 CrossRef CAS.
- N. Cukelj, D. Novotni, H. Sarajlija, S. Drakula, B. Voucko and D. Curic, LWT--Food Sci. Technol., 2017, 86, 85–92 CrossRef CAS.
- A. C. De Aguiar, M. Boroski, A. R. G. Monteiro, N. E. De Souza and J. V. Visentainer, J. Food Process. Preserv., 2011, 35, 605–609 CrossRef.
- C. M. A. de Moura, M. S. Soares, F. A. Fiorda, M. Caliari, R. Vera and M. V. E. Grossmann, Int. J. Food Sci. Technol., 2016, 51, 1495–1501 CrossRef CAS.
- Y. F. M. Kishk, H. E. Elsheshetawy and E. A. M. Mahmoud, Int. J. Food Sci. Technol., 2011, 46, 661–668 CrossRef.
- P. Marpalle, S. K. Sonawane and S. S. Arya, LWT--Food Sci. Technol., 2014, 58, 614–619 CrossRef CAS.
- A. Santiago, D. Ryland, S. Cui, H. Blewett and M. Aliani, J. Sci. Food Agric., 2019, 99, 831–843 CrossRef CAS PubMed.
- L. Seczyk, M. Swieca, D. Dziki, A. Anders and U. Gawlik-Dziki, Food Chem., 2017, 214, 32–38 CrossRef CAS PubMed.
- F. Yuksel, Qual. Assur. Saf. Crops Foods, 2019, 11, 183–189 CrossRef CAS.
- M. Aliani, D. Ryland and G. N. Pierce, Food Res. Int., 2011, 44, 2489–2496 CrossRef CAS.
- M. Kaur, V. Singh and R. Kaur, Bioact. Carbohydr. Diet. Fibre, 2017, 9, 14–20 CrossRef CAS.
- R. Kaur and M. Kaur, LWT--Food Sci. Technol., 2018, 91, 278–285 CrossRef CAS.
- T. Wandersleben, E. Morales, C. Burgos-Diaz, T. Barahona, E. Labra, M. Rubilar and H. Salvo-Garrido, LWT--Food Sci. Technol., 2018, 91, 48–54 CrossRef CAS.
- F. Zhu and J. C. Li, Int. J. Food Sci. Technol., 2019, 54, 1670–1676 CrossRef CAS.
- M. Kaur and S. Singh, Int. J. Food Prop., 2016, 19, 2432–2442 CrossRef CAS.
- G. G. Codină and S. Mironeasa, J. Food Sci. Technol., 2016, 53, 4149–4158 CrossRef PubMed.
- D. Goswami, R. K. Gupta, D. Mridula, M. Sharma and S. K. Tyagi, LWT--Food Sci. Technol., 2015, 64, 374–380 CrossRef CAS.
- P. D. Ribotta, G. T. Perez, A. E. Leon and M. C. Anon, Food Hydrocolloids, 2004, 18, 305–313 CrossRef CAS.
- M. Kaur and N. Singh, Food Chem., 2005, 91, 403–411 CrossRef CAS.
- A. F. Koca and M. Anil, J. Sci. Food Agric., 2007, 87, 1172–1175 CrossRef CAS.
- W. N. Huang, L. L. Li, F. Wang, J. J. Wan, M. Tilley, C. Z. Ren and S. Q. Wu, Food Chem., 2010, 121, 934–939 CrossRef CAS.
- C. M. Rosell, C. Marco, J. Garcia-Alvarez and J. Salazar, J. Food Process Eng., 2011, 34, 1838–1859 CrossRef.
- C. Collar, C. Bollain and C. M. Rosell, Food Sci. Technol. Int., 2007, 13, 99–107 CrossRef CAS.
- F. J. Warren, M. J. Gidley and B. M. Flanagan, Carbohydr. Polym., 2016, 139, 35–42 CrossRef CAS PubMed.
- A. Rohman and Y. B. C. Man, Int. J. Food Prop., 2013, 16, 1594–1603 CrossRef CAS.
- D. Ami, P. Mereghetti, A. Natalello, S. M. Doglia, M. Zanoni, C. A. Redi and M. Monti, Biochim. Biophys. Acta, 2011, 1813, 1220–1229 CrossRef CAS PubMed.
- L. Chen, Y. Tian, B. Sun, C. Cai, R. Ma and Z. Jin, Food Chem., 2018, 242, 131–138 CrossRef CAS PubMed.
- X. Y. Xu, Y. Xu, N. F. Wang and Y. B. Zhou, J. Cereal Sci., 2018, 81, 76–82 CrossRef CAS.
Footnote |
† Electronic supplementary information (ESI) available. See DOI: 10.1039/d0ra05742h |
|
This journal is © The Royal Society of Chemistry 2020 |