DOI:
10.1039/D0RA05058J
(Paper)
RSC Adv., 2020,
10, 34903-34909
The solvothermal synthesis and characterization of quaternary arsenic chalcogenides CsTMAsQ3 (TM = Hg, Cd; Q = S, Se) using Cs+ as a structure directing agent: from 1D anionic chains to 2D anionic layers†
Received
8th June 2020
, Accepted 5th September 2020
First published on 22nd September 2020
Abstract
Four new quaternary transition metal arsenic chalcogenide Cs–TM–As–Q compounds (TM = Hg, Cd; Q = S, Se) were synthesized using different mixed solvents. A 1,4-diaminobutane(1,4-dab)/water mixed solvent system was used to solvothermally synthesize the selenoarsenates CsTMAsSe3 (TM = Hg (1), Cd (2)). In 1, the eight-membered ring anion chain consists of trigonal-planar [HgSe3] and trigonal-pyramid [AsSe3]. Compound 2 is similar to the eight-membered ring chain anion of compound 1, and [CdAsSe3]− further joins, through μ3-Se and Cd, to form the layer anions [CdAsSe3]−. A 2-diaminopropane (1,2-dap) and water mixed solvent system was used to synthesize two thioarsenates, Cs2TM2As2S6 (TM = Cd (3), Hg (4)). Compounds 3, 4 and 2 are isostructural; the Cd and Hg atoms are four coordinated. Compounds 1–4 utilize Cs+ cations as a structural directing agent. Finally, the structural and optical properties of the novel series of arsenic chalcogenides were characterized.
1 Introduction
Crystalline chalcogenides have attracted extensive research interest owing to their fascinating structures and their potential applications in the fields of photoelectrochemistry,1–3 magnetism,4 nonlinear optics,5 ion exchange,6,7 semiconductors8 and so on. Generally, chalcogenides can be prepared using several traditional methods, including a high temperature solid-state approach, molten flux methods, electrochemical methods and hydrothermal thermal methods.9–11 Since the study by Bedard et al.12 was published in 1989, the solvothermal synthesis of main-group chalcogenidometalates using amines has attracted extensive attention, as it is a significant method for the synthesis of chalcogenides.1,9 During the past few decades, owing to the diversity of the bonding modes of the chalcogen atoms, the stereochemical effect of the lone electron pairs and the various coordination orientations of As/Sb(III) by sulfur/selenium atoms, large structural and compositional diversities have been observed for multinary chalcogenides, and therefore a large number of metal chalcogenides compounds have been prepared. Recently, it has been reported that in the combination of the structural units of AsxQy (Q = S, Se) the AsIII ions tend to adopt the diverse AsQn (Q = S, Se) (n = 3–6) ligands that can share corners and/or edges with transition metal ions (TM = Ag+, Cu2+, Mn2+),11,13–24 and could form interesting structural various multinary chalcogenides,8 such as 1D chains, 2D layers, 3D framework. For example, RbAg2As3Se6,10 NaAg2AsS3·H2O,13 (NH3CH2CH2NH3)AgAsS4,14 CsAgAs2Se4,15 K2AgAs3Se6 and Rb2AgAs3Se6,16 [Ni(dap)3]4[As10Cu2S18],17 [enH][Cu3As2S5],18 Na2(H2dap)0.5[Cu8(μ8-Se)(AsSe4)6/2],19 [pipH2][Mn2As2S6]20 and [Mn-(en)3]2[Mn(en)2AsS4][As3S6].21 There have been comparatively few reports on the incorporation of the transition metals Cd and Hg into the framework of the main-group metal chalcogenides by means of solvothermal synthesis, For example, Cs2HgSb4S8 and Cs2Hg2Sb2Se6,3 Rb8[Cd2(AsS4)2(AsS5)2],25 (Ph4p)2[Hg2As4S9] and (Me4N)[HgAs3S6],26 (Me4N)[HgAsSe3], (Et4N)[HgAsSe3] and (Ph4P)2[Hg2As4Se11].27 From the above instances we can see clearly that it is relatively rare for alkali metals cations used as structural directing agents to contain Hg/Cd arsenic chalcogenides in the M–TM–As–Q (M = alkali metals; TM = Hg, Cd; Q = S, Se) systems.8,28 There is a common feature in the structures of these compounds, there are various degrees of condensation of the basic structural [AsQ3]3− (Q = S, Se) units through the chalcogen atoms as the ligands approach the transition metals.22,28,29 Organic amines have been widely used in the solvothermal synthesis of quaternary transition metal chalcogenoarsenates owing to their structure directing effects. Our previous research has shown that K2CdSnS4,30 Rb2HgSnS4,31 Cs8Mn4Sn4Se16,32 Cs2HgSb4S8 and Cs2Hg2Sb2Se6,3 have different chains or layered structures, in contrast, ones that contain alkali-metal ions are still uncommon. In view of their significant potential to generate novel structures the transition metals Hg and Cd have been incorporated into the chalcogenoarsenates unit, to further the extend and investigate alkali metal ions as structural directing agents in chalcogenide systems. Recently, we have further expanded our work on solvothermal synthesis and synthesized four novel quaternary arsenic chalcogenides in the Cs–TM–As–Q system. In addition, we studied the properties of the four compounds.
2. Experimental section
2.1 Materials and methods
In this study, all of the chemical reagents used were of analytical grade, are commercially available and were used without further purification. The powder X-ray diffractometry (PXRD) was performed using a Rigaku XRD-6000 diffractometer using an operating power of 40 kV/40 mA and employing graphite-monochromatized Mo-Kα radiation. The solid-state optical absorption spectra were recorded on a Shimadzu UV-2550 double monochromatic spectrophotometer over the range of 220–800 nm at room temperature using Ba2SO4 power as the reflectance. The absorption spectrum data were calculated from the Kubelka–Munk function: α/S = (1 − R)2/2R, in which α is the absorption coefficient, S the scattering coefficient, and R is the reflectance.42,43 Differential scanning calorimetry (DSC) analyses were carried out using a STA 449 F5 Jupiter instrument under a nitrogen atmosphere (35 mL min−1) at 20–600 °C at a rate of 5 °C min−1.3 The DSC data of compounds 1, 2 were obtained using a DSC-200F3 Maia instrument, compounds 3, 4 were obtained using a STA 449 F5 Jupiter instrument.
2.2 Synthesis of compounds 1–4
Synthesis of CsHgAsSe3 (1). HgI2 (12 mg, 0.026 mmol), Cs2CO3 (15 mg, 0.046 mmol), As2S3 (13 mg, 0.053 mmol) and Se (7 mg, 0.089 mmol) were placed into a Pyrex glass tube, and 1,4-dab (540 mg, 6.13 mmol) and H2O (20 mg, 1.11 mmol) were added. The glass tube was sealed with a 10% filling, placed into a Teflon-lined stainless steel autoclave, and heated at 160 °C for 6 d. The products were washed with ethanol and water, respectively, and pure red crystals were obtained (17% yield). The crystals were washed with ethanol and dried and stored under vacuum.
Synthesis of CsCdAsSe3 (2). First, Cs2CO3 (12 mg, 0.026 mmol), As2S3 (14 mg, 0.057 mmol) and Se (7 mg, 0.089 mmol) were combined, HgI2 was replaced with CdI2 (11 mg, 0.030 mmol) and the reaction was performed in the same way as for (1), the mixture was heated to 150 °C for 6 d under the same mixed solvent. The other conditions remained unchanged to obtain yellow block crystals of the compound CsCdAsSe3 (2) (19% yield).
Synthesis of Cs2Cd2As2S6 (3). Yellow block crystals of the compound Cs2Cd2As2S6 (3) were synthesized from Cs2CO3 (17 mg, 0.052 mmol), As2S3 (12 mg, 0.05 mmol), CdI2 (19 mg, 0.051 mmol) and S (6 mg, 0.2 mmol) in 1,2-dap (531 mg, 6.91 mmol) and H2O (12 mg, 6.89 mmol), the reaction was heated at 140 °C for 7 d to obtain compound 3 (20% yield).
Synthesis of Cs2Hg2As2S6 (4). Cs2Hg2As2S6 (4) was synthesized using the same method as that used for compounds 1–3, the majority of conditions remained unchanged, in 1,2-dap (512 mg, 6.91 mmol) and H2O (17 mg, 6.89 mmol), Cs2CO3 (17 mg, 0.052 mmol), As2S3 (12 mg, 0.05 mmol) and S (7 mg, 0.022 mmol) were combined, CdI2 was used instead of HgI2 (22 mg, 0.049 mmol) to obtain yellow block crystals of compound Cs2Hg2As2S6 (4) (22% yield).
2.3 Single-crystal X-ray diffraction
The PXRD patterns of 1–4 are shown in Fig. S3.† The 2θ values for all peaks exhibit a good match with the corresponding simulated results from the single-crystal diffraction data, indicating the high purity of all of the above-mentioned samples. Polycrystalline samples of the title compounds of 1–4 with dimensions of 0.23 × 0.20 × 0.14 mm, 0.26 × 0.21 × 0.19 mm, 0.28 × 0.25 × 0.22 mm and 0.28 × 0.25 × 0.20 mm were collected with the crystal diffraction intensity data on a Bruker APEX-II CCD diffractometer equipped with graphite monochromated Mo-Kα radiation (λ = 0.71073 Å) at 296 K. The structures of 1–4 were solved using direct methods and refined using full-matrix least-square methods on F2 by the SHELXL 97 package.43,44 The related crystallographic data and structural refinement parameters for the 1–4 compounds are summarized in Table S1,† and some part relevant bond distances and bond angles at listed in Tables S2–S5 (ESI).†
3. Results and discussion
3.1 Synthetic considerations
The formation of crystals is affected by the different proportions of the initial reactant. The described synthesis methods were found to be the most effective to yield the title compounds and the reproducibility of all the products was good. In compound 1, HgI2 was replaced with CdI2 powder to obtain 2, other salts were formed that contained mercury but did not form crystals. No target crystals were obtained in repeated experiments in which the Se powder was replaced with S powder. For compound 3, when Cd powder was used instead of CdI2 as the reactant, a small number of crystals were found, but there were more black impurities, which may be because Cd2+ had a stronger reactivity than Cd. For 4, other mercury-containing salts and a small number of crystals were formed, with further impurities and a low yield. In addition, in the solvent system for the synthesis of these four compounds, attempts were made to replace HgI2 or CdI2 with salts containing other transition metal ions, and no crystal formation was observed. When other carbonates of alkali metals were used instead of Cs2CO3 as reactants, it was found that no crystals were formed, as the size of the counter ion radius has a significant influence on the synthesis. Meanwhile, the counter ion may play a certain role in the stability of the anionic skeleton. For 1 and 2, the use of other organic solvents, instead of 1,4-dab, did not result in crystals. For compounds 3 and 4, if 1,4-dab or ethanediamine were used instead of 1, 2-dap as the reaction solvent, no crystals were formed. In conclusion, in the synthetic environment of compounds 3 and 4, 1,2-dap is more suitable for the synthesis of compounds 3 and 4 than the other organic solvents. If the reaction solvent used for compounds 1–4 is not added with water, the synthesized crystals are all gathered together, therefore water may increase the solubility of the reactants during the reaction.
3.2 Structural descriptions
The crystal data for compounds 1–4 and their related parameters are shown in Table S1 (ESI†), and the structure of CsHgAsSe3 (1) shown using single-crystal X-ray diffraction analysis23 reveals that 1 crystallizes in the monoclinic space group P21/n. It is isostructural with (Me4N)[HgAsSe3] and (Et4N)[HgAsSe3].27 The compound contains one-dimensional chains consisting of trigonal-planar Hg2+ ions and [AsSe3]3− units. The [HgAsSe3]nn− chains are parallel to the crystallographic a axis and separated by Cs+ cations (Fig. S1, ESI).†
For 1, the alternating connection of the two trigonal planar [HgSe3] and two trigonal pyramids [AsSe3] (Fig. 1a) gives rise to a heterometallic 8-membered [Hg2As2Se6] ring (Fig. 1b), which further connects with the neighbouring 8-membered rings and forms a [HgAsSe3]nn− anion chain by edge-sharing (Hg1–Se2–As1) (Fig. 1c) the two coordination Se as a bridge using As and Hg. The common [HgAsSe3]nn− 1D anion chain of compound 1 is similar to that observed in (R4N)[HgAsSe3] (R = Me, Et).26 Both structures contain the Hg2As2Q4 8-membered rings, but the counterions are different. The relationship lies in the fact that the structural motif in the [HgAsSe3]nn− can be derived from that of [Hg2As4S9]n2n− by substitution of one fourth of the arsenic atoms for Hg.27 As above, a similar chain consists of heterometallic 8-membered rings, such as the infinite anionic [In2Sb2S8]n4n− chain by two [InS4] and two [SbS3] units, which joined to yield an 8-membered [In2Sb2S4] ring in a chair-like conformation, which is further interconnected at the In atom.33 In compounds K2MSbS3(SH) (M = Zn, Cd), each of the [M2Sb2S8(SH)2]8− eight-membered rings is formed by two [MS3(SH)]5− tetrahedra and two [SbS3]3− pyramids by corner sharing, the eight-membered rings with an opposite orientation are further connected to form an eight-membered ring 1-D chain.34
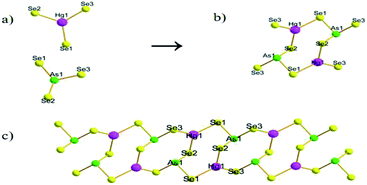 |
| Fig. 1 (a) The [HgSe3] unit and [AsSe3] unit. (b) The 8-membered [Hg2As2Se6] ring. (c) The structure of the [HgAsSe3]nn− anion chain in 1. | |
The [HgAsSe3]nn− anion contains three crystallographically distinct Se atoms, one Hg atom and one As atom. The Hg2+ is in the trigonal-planar environment with bond angles of Se1–Hg1–Se3 at 120.24(6)°, Se1–Hg1–Se2 at 121.95(5)°, and Se3–Hg1–Se2 at 117.41(6)° (Table S2†). The Hg–Se distances are in the range of 2.571(2)–2.592(2) Å, and the Hg–Se bond lengths match well with those observed in the HgSe3 trigonal-planar of (Me4N)[HgAsSe3] and (Et4N)[HgAsSe3] (Hg–Se: 2.572(2)–2.594(1) Å).27 All As atoms are coordinated by three Se atoms at distances in the range of 2.372(2)–2.404(2) Å, with Se–As–Se angles in the range of 97.94(7)–101.20(7), forming typical trigonal pyramidal AsSe3 units. The bond lengths and angles of the AsSe3 units are consistent with the corresponding values reported in selenoarsenate compounds.21,26 All selenium atoms join an As and a Hg atom with As–Se–Hg angles in the range of 90.48(8)–93.31(7)° (Table S2†).
Apparently, the different coordination modes of the Hg atom, to some extent, affect their roles in constructing the structures. In addition, when the Hg atoms in compound 1 are replaced with Cd atoms, they undergo interesting structural changes, an eight-membered ring chain structure similar to that found in compound 1 is further connected by Cd–Se to form a layered structural compound CsCdAsSe3 (2) (Fig S2, ESI†).
The [CdAsSe3]nn− anion contains three crystallographically distinct Se atoms, one Cd atom and one As atom. The As atom is in the same coordinated form as in compound 1, the distances are in the range of 2.351(4)–2.406(4) Å, with Se–As–Se angles in the range of 98.58(14)–102.00(14) (Table S3†), the bond lengths and angles of the AsSe3 units are consistent with the corresponding values reported in selenoarsenate compounds.23 The Cd atom is coordinated by four Se atoms at distances in the range of 2.597(4)–2.719(4) Å, forming a significantly distorted CdSe4 tetrahedron with Se–Cd–Se angles in the range of 94.58(11)–122.96(12) (Table S3†). Except for Se(3), which is coordinated by two Cd and an As atom, with Cd–Se–As(Cd) angles in the range of 97.14(12)–115.22(11)°, the remaining two crystallographically independent selenium atoms join the As and a Cd atoms to form As–Se–Cd angles in the range of 88.34(12)–95.55(12)° (Table S3†). Considering the diversity of the transition metal–selenium bonding, the introduction of different transition metal can lead to different ways of bridging the selenoanionic species, consequently producing a variety of structures. This result shows that the cadmium ions in 2 play a key role in the formation of the layers. The [CdAsSe3]nn− anion chain forms a 2D layered structure, which consists of rings similar to the heterometallic 8-membered rings that form the [HgAsSe3]nn− chains in compound 1. Similarly structural eight-membered rings of the Cd2As2Se6 units were also observed in (Me4N)[HgAs3S6],27 C20H80In6N20Ni3S21Sb6,33 KAgAsS2,35 Cs2Hg3M2S8 (M = Sn, Ge),36 [1,2-pdaH]HgSbS3 and [Ni(en)3]0.5HgSbS3,37 but the connections were different. For example, the 2D [Hg3M2S8]2− layer is formed by the two coordinated Hg atom between the chains of alternating eight-membered ring chains in Cs2Hg3M2S8 (M = Sn, Ge).36 The oppositely orientated adjacent [CdAsSe3]nn− chains A/B connect with each other via Cd1–Se3 bonds to form a 2D network C (Fig. 2). Simultaneously, 6-membered rings of [Cd2AsSe3] can arise between the 8-membered ring chains. Similar heterometallic 6-membered rings to those described above were also observed in NaAg2AsS3·H2O and KAg2AsS3.12 KCu2SbS3,38 in the compound KCu2SbS6, are six-membered hetero rings [Cu2SbS3] that edge-share to form a single layer, these layers are combined together by Cu(3)–S and Cu(4)–S that form a 2D double-layered structure,38 the compound KCu2SbS3 is composed of distorted 6-membered Cu2SbS3 rings attached via edge sharing to form [Cu2SbS3] hexagonal nets.39 As far as we know, only the compounds RbCu4AsS4,35 (1,4-DABH2)Cd2Sb2S6,40 and [enH2]0.5HgSbS3 (ref. 37) have 8-membered rings and 6-membered rings, but the connections and the structure of the connection in compound 2 are different. The structural difference between them indicates that amine, alkali and alkaline earth metals have different structure-directing effects on the crystallization of the arsenic chalcogenides. The transition metals also have structure directing roles in quaternary chalcogenides, although they differ from that of alkali-, alkaline earth and amines in the synthesis of chalcogenides.41
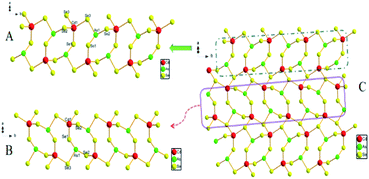 |
| Fig. 2 The opposite orientation [CdAsSe3]nn− chains in A/B formation in the 2D network C for compound 2. | |
Therefore, further understanding of the role of the structural directing agent will be important for the rational design and synthesis of novel chalcogenides. The ternary Cd–As–Q frameworks are rare compared with a variety of Hg–As–Q, Cu–As–Q and Ag–As–Q networks. CsCdAsSe3 (2) is the only example of the cadmium selenoarsenates that contain the charge-balancing ions of cesium cations. To the best of our knowledge, this type of layer was never reported in the M–As–Q compounds. The formation of 1 and 2 indicates that the Hg2+ and Cd2+ ions are respectively incorporated into the 1D and 2D selenoarsenate(III) networks. If the 1,4-diaminobutane is replaced with 1,2-diaminopropane, two new compounds are formed, Cs2M2As2S6 (M = Cd (3), Hg (4)). The Cd and Hg atoms are four coordinated. Single-crystal X-ray diffraction analysis22 reveals that 3 and 4 are isostructural, and like 2 they crystallize in the monoclinic space group P21/n.
To the best of our knowledge, different structural chalcogenides with similar chemical compositions to {Hg/Cd–Sb/As-Q3} have been reported, but synthesis of the common eight-membered ring structures by different structural directing agent, including (R4N)[HgAsSe3](R = Me, Et),27 (Ph4P)2[Hg2As4S9], 26(RH2)0.5[HgSbS3](R = en, teta) has not previously been reported.37 The layers of compounds 2–4 were similar to (RH2)0.5[HgSbS3] (R = en, teta), although they are all composed of six-membered Hg(Cd)2–As(Sb)/Q3 (Q = S, Se) rings and eight-membered Hg(Cd)2 As(Sb)2/Q4 rings, the arrangement of the two types of rings are quite different.
3.3 Thermogravimetric and differential scanning calorimetry analyses
The DSC data for compounds 1, 2 were obtained under an Ar atmosphere, the data for compounds 3, 4 were obtained under an N2 atmosphere from 20–530 °C and 25–600 °C (Fig. S4†). The data shows the DSC curves of compounds 1–4 display endothermic peaks at 429.9, 429.9, 452 and 357 °C respectively. The main reason for this is that the structure of compounds 1–4 collapses at different temperature (Fig. S4, ESI†).29
3.4 Optical properties
The UV-vis reflectance spectroscopy of the powder samples of compounds 1–4 was recorded at room temperature. The solid-state optical absorption data for compounds 1–4 are shown in Fig. 3. The absorption data indicates that the optical band gaps are 1.91 eV for 1, 2.12 eV for 2, 2.71 eV for 3 and 2.58 eV for 4, respectively, as calculated from the reflectance data using the Kubelka–Munk function. The band gaps for compounds 1–4 are similar to the selenidoarsenates and thioantimonates Cs3AgAs4Se8 (2.10 eV), CsAgAs2Se4 (1.79 eV),15 (R4N)[HgAsSe3] (R = Me, Et) (2.4 eV),27 [Mn(en)3]2As2Se5 (2.67 eV),45 [Mn(en)3]2As2S5 (2.71 eV),43 Cs2AgAsS4 (2.7 eV),46 RbCu2AsIIIS3 (1.96 eV), RbCu4AsIIIS4 (2.15 eV),35 CsCu2AsS3 (2.3 eV),41 and CsAg2AsS3 (2.7 eV),47 all of which have semiconductor properties.
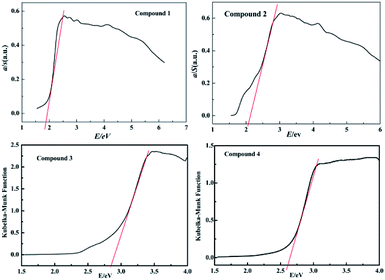 |
| Fig. 3 Solid-state optical absorption spectra for compounds 1–4. | |
4. Conclusions
In this work, we have successfully prepared quaternary chalcogenides and synthesized four novel compounds in the Cs–TM–As–Q system using a solvothermal method. The solid-state optical absorption spectra indicate that the four compounds are semiconductors. Although the four compounds have similar chemical compositions and the same proportions, their structures and transition metal ion coordination modes are different from each other. It can be seen that different organic solvents, used as mineralizers, and solvothermal methods can be used to synthesize arsenic chalcogenides containing mercury or cadmium, Hg/Cd–As–Q (Q = S, Se), connected in various modes and result in Hg/Cd arsenic chalcogenides with architectures that range from 1D chains to 2D layers. The chain lengths of the different bidentate organic amines are different and their roles in selenides and sulfides are different. This not only enriches the structural chemistry, but also provides novel method for the synthesis of arsenic chalcogenides. This could be used to investigate which properties have significance for the synthesis of arsenic chalcogenides containing different transition metal elements in an anionic framework.
Conflicts of interest
There are no conflicts to declare.
Acknowledgements
This work was supported by the National Natural Science Foundation of China (21461019).
Notes and references
- Ke Z. Du, M. L. Feng, X. H. Qi, Zu Ju Ma, L. H. Li, J. R. Li, C. F. Du, G. D. Zou and X. Y. Huang, (enH2)4.5[In(AsVS4)3][As2III(μ-S2)S3]Cl and (enH2)MnAsIII AsVS6: two thioarsenates(III,V) with mixed-valent optical properties, Dalton Trans., 2014, 43, 2733–2736 RSC.
- C. Zhang, M. Ji, S.-H. Ji and Y.-L. An, Mild Solvothermal Syntheses and Characterization of Layered Copper Thioantimonates(III) and Thioarsenate(III), Inorg. Chem., 2014, 53, 4856–4860 CrossRef CAS.
- C. X. Du, F. Y. Qi, J. Chen and M. Baiyin, Two Mercury Antimony Chalcogenides Cs2HgSb4S8 and Cs2Hg2Sb2Se6 with Cesium Cations as Counterions, ACS Omega, 2018, 3, 15168–15173 CrossRef CAS.
- Yu J. Zheng, Y. F. Shi, C. Bin Tian, H. Lin, Li M. Wu, X. T. Wu and Qi L. Zhu, An unprecedented pentanary chalcohalide with Mn atoms in two chemical environments: unique bonding characteristics and magnetic properties, Chem. Commun., 2019, 55, 79–82 RSC.
- H. Lin, W. Bo Wei, H. Chen, X. T. Wu and Qi L. Zhu, Rational design of infrared nonlinear optical chalcogenides by chemical Substitution, Coord. Chem. Rev., 2020, 406, 213150 CrossRef CAS.
- R. qi Wang, H. jie Chen, Yi Xiao, H. Ido, Ke jun Bu, X. Zhang, J. Pan, Y. Gu, Z. nan Guo, Fu qiang Huang and G. K. Mercouri, Kx[Bi4−xMnxS6], Design of a Highly Selective Ion Exchange Material and Direct Gap 2D Semiconductor, J. Am. Chem. Soc., 2019, 141, 16903–16914 CrossRef CAS.
- R. C. Zhang, J. C. Zhang, Z. Cao, J. J. Wang, S. S. Liang, H. J. Cong, He J. Wang, D. J. Zhang and Y. L. An, Unusual Flexibility of Microporous Sulfifides during Ion Exchange, Inorg. Chem., 2018, 57, 13128–13136 CrossRef CAS.
- A. Abudurusuli, K. Wu, A. Tudi, Z. Yang and S. Pan, ABaSbQ3 (A = Li, Na; Q = S, Se): diverse arrangement modes of isolated SbQ3 ligands regulating the magnitudes of birefringences, Chem. Commun., 2019, 55, 5143–5146 RSC.
- K. Y. Wang, Bo Zhang, X. H. Qi, N. N. Shen, C. F. Du, Z. F. Wu, M. L. Feng and X. Y. Huang, New Members of the Family of In–Sb–S Compounds: Different Roles of Organic Amines, Cryst. Growth Des., 2015, 15, 29–38 CrossRef CAS.
- M. Wachhold and G. K. Mercouri, RbAg2As3Se6: A Unique Three-Dimensional Silver-Selenoarsenate Framework ∞3 [Ag2As3Se6]−, Assembled from [As3Se6]3− Rings and “Ag22+” Dumbbell Units, Inorg. Chem., 1999, 38, 4178–4180 CrossRef CAS.
- J. Zhou, X. F. Tan, X. Liu, M. Qing, R. Q. Zhao and Q. Tang, A series of new manganese thioarsenates(V) based on different unsaturated [Mn(amine)x]2+ Complexes, Dalton Trans., 2015, 44, 16430–16438 RSC.
- R. L. Bedard; S. T. Wilson, L. D. Vail, J. M. Bennett and E. M. Flanigen, in Zeolites: Facts, Figures, Future, ed. P. Jacobs and R. van Santen, Elsevier, Amsterdam, 1989, pp. 375–378 Search PubMed.
- ming Y. Dong, P. Hou, L. Chang, W. Chai, X. Zheng, L. Zhang, M. jia Zhi, C. mei Zhou and Yi Liu, Effect of alkali cations on two-dimensional networks of two new quaternary thioarsenates (III) prepared by a facile surfactant-thermal Method, J. Solid State Chem., 2016, 241, 47–53 CrossRef.
- Y. lin An, X. feng Li, X. Liu, M. Ji and C. Jia, A solvothermal synthesis of a novel 1D ladder-like (NH3CH2CH2NH3)AgAsS4 containing N–H…S hydrogen bonding, Inorg. Chem. Commun., 2013, 6, 1137–1139 CrossRef.
- M. Wachhold and M. G. Kanatzidis, Cs3AgAs4Se8 and CsAgAs2Se4: selenoarsenates with Infinite 1∞[AsSe2]− chains in different Ag+, coordination environments, Inorg. Chem., 2000, 39, 2337–2343 CrossRef CAS.
- M. Wachhold and M. G. Kanatzidis, Condensation of pyramidal [AsSe3]3− anions for the construction of polymeric networks: solventothermal synthesis of K3AgAs2Se5·0.25MeOH, K2AgAs3Se6, and Rb2AgAs3Se6, Inorg. Chem., 1999, 38, 3863–3870 CrossRef CAS.
- X. Liu, J. Zhou, L. An, G. Q. Bian, R. qing Zhao and Z. wen Mo, [Ni(dap)3]4[As10Cu2S18]: a new thioarsenate containing the rare [As3CuS6] cluster with mixed-valence As2+/As3+ ions, Dalton Trans., 2014, 43, 3055–3058 RSC.
- W. W. Xiong, E. U. Athresh, Y. T. Ng, J. Ding, T. Wu and Q. C. Zhang, Growing crystalline chalcogenidoarsenates in surfactants: from zero-dimensional cluster to three-dimensional framework, J. Am. Chem. Soc., 2013, 135, 1256–1259 CrossRef CAS.
- R. C. Zhang, C. Zhang, D. J. Zhang, J. J. Wang, Z. F. Zhang, M. Ji and Y. L. An, Copper-Rich Framework Selenoarsenates Based on Icosahedral Cu8Se13 Clusters, Z. Anorg. Allg. Chem., 2012, 638(15), 2503–2507 CrossRef CAS.
- D. D. Yang, Y. Song, Bo Zhang, N. N. Shen, G. L. Xu, W. W. Xiong and X. Y. Huang, Exploring the Surfactant – Thermal Synthesis of Crystalline Functional Thioarsenates, Cryst. Growth Des., 2018, 18, 3255–3262 CrossRef CAS.
- M. Fu, G. C. Guo, L. Z. Cai, Z. J. Zhang and J. S. Huang, Incorporating transition metal complexes into tetrathioarsenates(V): syntheses, structures, and properties of two unprecedented [Mn(dien)2]n[Mn(dien)AsS4]2n−·4nH2O and [Mn(en)3]2[Mn(en)2AsS4][As3S6], Inorg. Chem., 2005, 44, 184–186 CrossRef CAS.
- J. H. Chou, J. A. Hanko and M. G. Kanatzidis, Synthesis and characterization of (Ph4P)2[Ni2As4S8], (Me4N)2[Mo2O2As2S7] and (Et4N)2[Mo2O2As2Se7] new one-dimensional polymeric compounds containing thio- and selenoarsenate ligands, Inorg. Chem., 1997, 36, 4–9 CrossRef CAS.
- T. M. Martin, P. T. Wood, G. L. Schimek, W. T. Pennington and J. W. Kolis, New metal carbonyl Complexes of mixed group 15/16 anions: structural characterization of [Fe(As3Se3)2(CO)]2−, [Mn(As3Se8)(CO)3]2−, and [Fe2(AsTe4)2(CO)4]2−, Inorg. Chem., 1995, 34, 4385–4391 CrossRef CAS.
- D. X. Jia, J. Zhao, Y. L. Pan, W. W. Tang, B. Wu and Y. Zhang, Solvothermal synthesis and characterization of polyselenidoarsenate salts of transition metal complex cations, Inorg. Chem., 2011, 50, 7195–7201 CrossRef CAS.
- R. G. Iyer and M. G. Kanatzidis, [Mn2(AsS4)4]8− and [Cd2(AsS4)2(AsS5)2]8−: Discrete Clusters with High Negative Charge from alkali metal polythioarsenate fluxes, Inorg. Chem., 2004, 43, 3656–3662 CrossRef CAS.
- J. H. Chou and M. G. Kanatzidis, Hydrothermal synthesis of (Ph4P)2[Hg2As4S9] and (Me4N)[HgAs3Se6], extended chains and layers based on the condensation of [AsS3]3− units, Chem. Mater., 1995, 7, 5–8 CrossRef CAS.
- J. H. Chou and M. G. Kanatzidis, Hydrothermal Synthesis and Characterization of (Me4N)[HgAsSe3], (Et4N)[HgAsSe3], and (Ph4P)2[Hg2As4Se11]: Novel 1-D Mercury Selenoarsenates, J. Solid State Chem., 1996, 123, 115–122 CrossRef CAS.
- C. Wang and R. C. Haushalter, A discrete cubane-like [Cu7Te] cluster: synthesis and structural characterization of (NBu4)4Cu7As3Te13, Chem. Commun., 1997, 1457 RSC.
- J. R. Li and X. Y. Huang, [(Me)2NH2]0.75[Ag1.25SnSe3]: A three-dimensionally microporous chalcogenide exhibiting framework flexibility upon ion-exchange, Dalton Trans., 2011, 40, 4387–4390 RSC.
- B. Y. Menghe, G. Gang and N. R. Jiruga, K2CdSnS4 Solvothermal Synthesis and Crystal Structure, J. Inorg. Chem., 2014, 30(2), 405–410 Search PubMed.
- B. Meng-he, L. F. ying, X. Xiao, T. Na, Mi-la NA, B. Wu yun and B. Yong-sheng, Solvothermal Synthesis and Characterization of Sheet Structure Containing Adamantane [Hg2Sn2S10]8− Anions, Chem. Res. Chin. Univ., 2011, 27(6), 915–918 Search PubMed.
- W. Han and M. BaiYin, Solvothermal synthesis and characterization of chain Cs8Mn4Sn4Se16 and[Ni(1,2-dap)3]2Cd2Sn2S8 (1,2-dap), J. Appl. Chem., 2018, 35(6), 701–707 Search PubMed.
- J. Zhou, X. Liu, L. An, F. Hu, Y. Kan, R. Li and Z. ming Shen, Solvothermal synthesis and characterization of thioindate–thioantimonates with transition-metal complexes: the first examples of the incorporation of transition metal ions into In–S–Sb frameworks, Dalton Trans., 2013, 42, 1735–1742 RSC.
- X. Zhang, Na Yi, R. Hoffmann, C. Zheng, J. Lin and F. Huang, Semiconductive K2MSbS3(SH) (M = Zn, Cd) Featuring One Dimensional [M2Sb2S6(SH2)]4− Chains, Inorg. Chem., 2016, 55, 9742–9747 CrossRef CAS.
- H. G. Yao, C. F. Tang, Y. L. An, Zi J. Ou, G. H. Wu, P. Lan and Yi L. Zheng, Solvothermal syntheses and characterization of three new silver(I)/copper(I)-thioarsenates based on As2+/As3+ ions, J. Solid State Chem., 2017, 24, 87–91 CrossRef.
- G. A. Marking, J. A. Hanko and M. G. Kanatzidis, New Quaternary Thiostannates and Thiogermanates A2Hg3M2S8 (A= Cs, Rb; M=Sn, Ge) through Molten A2Sx. Reversible Glass Formation in Cs2Hg3M2S8, Chem. Mater., 1998, 10, 1191–1199 CrossRef CAS.
- De N. Kong, Z. L. Xie, M. L. Feng, D. Ye, Ke Z. Du, J. R. Li and X. Y. Huang, From One-Dimensional Ribbon to Three-Dimensional Microporous Framework: The Syntheses, Crystal Structures, and Properties of a Series of Mercury Antimony Chalcogenides, Cryst. Growth Des., 2010, 10(No. 3), 1364–1372 CrossRef CAS.
- R. qi Wang, X. Zhang, J. qiao He, C. Zheng, J. hua Lin and Fu qiang Huang, Synthesis, crystal structure, electronic structure, and photoelectric response properties of KCu2SbS3, Dalton Trans., 2016, 45, 3473–3479 RSC.
- L. Chang, P. Hou, M. Zhi, C. Zhou, W. Chai, Q. Zhang, Yi Liu and Ya ying Shen, Facile surfactant-thermal syntheses and characterization of quaternary copper thioantimonates(III) ACu2SbS3 (A =K, Rb, Cs), J. Alloys Compd., 2016, 660, 171–177 CrossRef.
- G. Gang, M. BaiYin, X. Wang, NaR. Jiruga, X. Xu, W. Bai and D. SiQin, Solvothermal synthesis and characterization of layered polythioantimony(III)acid cadmium compounds(II)(1,4-DABH2)Cd2Sb2S6, J. Inorg. Chem., 2013, 29(5), 979–984 CAS.
- H. G. Yao, M. Ji, S. H. Ji and Y. Lin An, Synthesis, structure and characterization of two new copper(I)-thioarsenates (III) constructed by the [AsS3]3− and CuSx units, J. Solid State Chem., 2013, 198, 289–294 CrossRef CAS.
-
(a) Z. Wang, H. Zhang and C. Wang, Synthesis and Characterization of One-Dimensional and Molecular M(tren)InAsS4 (M = Mn, Co and Zn) Compounds with a Noncondensed AsS33− Unit, Inorg. Chem., 2009, 48, 8180–8185 CrossRef CAS;
(b) W. W. Wendlandt and H. G. Hecht, Reflectance Spectroscopy, Inter-science Publishers, New York, 1966 Search PubMed.
-
(a) X.-F. Tan, X. Liu, J. Zhou, L. Zhu, R. Zhao and Q. Huang, Two Quaternary Copper Thiostannates with Lanthanum(III) Complexes, J. Cluster Sci., 2016, 27, 257–265 CrossRef CAS;
(b) G. M. Sheldrick, SHELXL-97, Program for the Refinement of Crystal Structures, University of Göttingen, Germany, 1997 Search PubMed.
- W. Xiao jing, B. Meng he, G. Gang, N. JiRu ga, N. Mi la and X. Xiao qing, Solvothermal Synthesis and Characterization of Novel Chain-like Compound Mn3(dap)2(AsS3)2, J. Synth. Cryst., 2012, 41(12), 6 Search PubMed.
- D.-X. Jia, Q.-X. Zhao, J. Dai, Y. Zhang and Q.-Y. Zhu, Chalcogenidoarsenates with Transition Metal Complex Cations: [M(en)3]2As2S5(M=Mn, Ni) and [Mn(en)3]2As2Se5, Z. Anorg. Allg. Chem., 2006, 632, 349353 CrossRef.
- P. T. Wood, G. L. Schimek and J. W. Kolis, Synthesis and Characterization of Novel One-Dimensional Phases from Supercritical Ammonia: Cs3Ag2Sb3S8, α- and β-Cs2AgSbS4, and Cs2AgAsS4, Chem. Mater., 1996, 8, 721–726 CrossRef CAS.
- H. G. Yao, P. Zhou, S. H. Ji, R. C. Zhang, M. Ji, Y. L. An and G. L. Ning, Syntheses and Characterization of a Series of Silver-Thioantimonates(III)and Thioarsenates(III) Containing Two Types of Silver-Sulfur Chains, Inorg. Chem., 2010, 49(3), 1186–1190 CrossRef CAS.
Footnotes |
† Electronic supplementary information (ESI) available: CIFs, structures of the compounds, a summary of the crystal data, single crystal X-ray determination, PXRD patterns, DSC curves, and tables are available. CCDC 2005276, 2005275, 1880651 and 1880652. For ESI and crystallographic data in CIF or other electronic format see DOI: 10.1039/d0ra05058j |
‡ These authors contributed equally to this work. |
§ First author. |
¶ Co-first authors. |
|| Second author. |
|
This journal is © The Royal Society of Chemistry 2020 |
Click here to see how this site uses Cookies. View our privacy policy here.