DOI:
10.1039/D0RA04267F
(Paper)
RSC Adv., 2020,
10, 19655-19658
An efficient enantioselective approach to multifunctionalized γ-butyrolactone: concise synthesis of (+)-nephrosteranic acid†
Received
1st May 2020
, Accepted 14th May 2020
First published on 22nd May 2020
Abstract
A short, efficient and novel approach for multifunctionalized γ-butyrolactone paraconic acids and its application to the total synthesis of (+)-nephrosteranic acid from readily available PMB (R)-glycidyl ether as a starting material are described. Key transformations include asymmetric Michael addition catalyzed by chiral diphenylprolinol silyl ether and stereoselective α-methylation.
Bioactive natural products containing a multifunctionalized γ-butyrolactone moiety are found abundantly in nature.1 The paraconic acids (1–10) containing multifunctionalized γ-butyrolactone were isolated from various species of lichens, fungi, moss and cultures of Penicillium sp.2 These acids possess interesting biological activities such as antitumor, antibacterial, antibiotic, antifungal/antiviral and growth regulatory properties.3 The whiskey lactone 11 and cognac lactone 12 have great commercial interest because they are potential key components in the flavor of aged alcoholic beverages.4 Architecturally, the paraconic acid family comprises a variable length alkyl chain at the C5 position, a C4 carboxyl group and methyl or methylene substituents at the C3 position, which play an important role in the biological activities of the paraconic acids (Fig. 1).
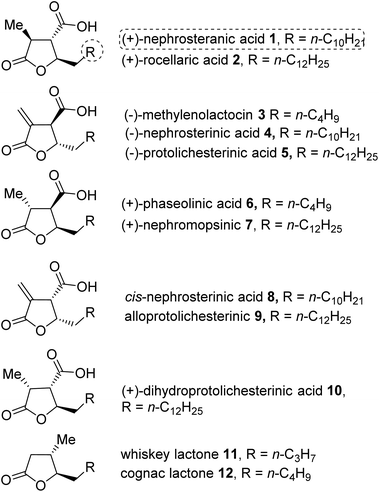 |
| Fig. 1 Representative structures of γ-butyrolactone based paraconic acids and lactones. | |
Intrigued by the unique structural features and biological activities of paraconic acids, hitherto, several total5 and formal6 synthesis of paraconic acids such as (+)-nephrosteranic acid are documented in literature. More recently, Appayee and co-workers disclosed an elegant approach for the stereodivergent synthesis of chiral paraconic acids via dynamic kinetic resolution of 3-acylsuccinimides.7 As part of our research program aimed at developing the asymmetric synthesis of bioactive natural molecules,8 we became attentive in developing a flexible and general approach for the synthesis of multifunctionalised γ-butyrolactone paraconic acids. Herein, we are reporting a short, efficient and novel general approach for the synthesis of paraconic acids and its application to the enantioselective synthesis of (+)-nephrosteranic acid 1 using organocatalyzed Michael addition reaction as key step.
Our general retrosynthetic route for asymmetric synthesis of γ-butyrolactone based paraconic acids and its application to enantioselective synthesis of (+)-nephrosteranic acid 1 was envisaged via the retrosynthetic approach as displayed Scheme 1. We envisioned that the γ-butyrolactone 13 could be used as a key intermediate from which paraconic acids 1–10 including (+)-nephrosteranic acid 1 would be synthesized via methylenation or stereoselective methylation at the C3 centre. The γ-butyrolactone 13 could be achieved from protected nitro-acid derivative 14 via deprotection and in situ lactonization followed by Nef reaction. The nitro-acid derivative 14 in turn could be synthesized from (R)- or (S)-diphenylprolinol silyl ether catalyzed Michael addition of CH3NO2 to α,β-unsaturated aldehyde intermediate obtained from the controlled DIBAL-H reduction of olefinic ester derivative 15 followed by oxidation. The α,β-unsaturated ester 15 could be obtained from protected (R)- or (S)-glycidol 16 by treatment with suitable Grignard reagents, secondary alcohol protection, primary alcohol deprotection and oxidation followed by 2C-Wittig olefination reaction. The desired stereochemistry of paraconic acids 1–10 could be achieved by simply altering the (R)- and (S)-configuration of glycidyl ether and/or by using catalyst (R)- or (S)-diphenylprolinol silyl ether during Michael addition reaction. Thus, in principle, C3, C4 and C5 chiral centres in paraconic acids could be easily manipulated and accessed by this approach.
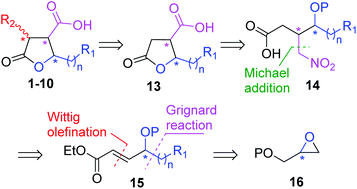 |
| Scheme 1 Retrosynthetic general approach of γ-butyrolactone based some paraconic acids and lactones. | |
As depicted in Scheme 2, the synthesis of (+)-nephrosteranic acid 1 as a representative target compound of paraconic acids was commenced from readily available PMB (R)-glycidyl ether 16a9 which was subjected to copper-catalyzed (CuI) regioselective ring opening with the Grignard reagent, derived from decyl bromide to furnish the alcohol derivative 17 in 85% yield. The alcohol derivative 17 on silyl protection with tert-butyldiphenylsilyl chloride (TBDPSCl) and imidazole with DMAP in catalytic amount afforded the silyl ether derivative 18 in 95% yield which on PMB ether cleavage using CAN (ceric ammonium nitrate) at 0 °C to rt furnished the terminal alcohol derivative 19 in 91% yield. The alcohol derivative 19 on oxidation under Swern conditions10 followed by treatment with (ethoxycarbonylmethylene)triphenylphosphorane in THF afforded the trans-olefinic ester derivative 20 in 92% yield. Our next goal was to carry out the synthesis of multifunctionalized γ-butyrolactone. Towards this end, trans-olefinic ester 20 on controlled reduction with DIBAL-H at −78 °C to α,β-unsaturated aldehyde intermediate and successive conjugate Michael addition11 of nitromethane in the presence of (S)-diphenylprolinol silyl ether (10 mol%) afforded the nitroaldehyde adduct which on subsequent oxidation with oxone12 furnished the nitro-acid derivative 21 in excellent yield.
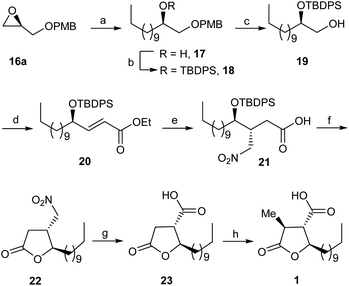 |
| Scheme 2 Reagents and conditions: (a) C10H21MgBr, CuI, dry THF, −30 °C, 6 h, 85%; (b) TBDPSCl, imidazole, cat. DMAP, CH2Cl2, 0 °C-rt, 8 h, 95%; (c) CAN, CH3CN : H2O (4 : 1, v/v), 0 °C-rt, 2 h, 91%; (d) (i) (COCl)2, DMSO, Et3N, CH2Cl2, −78 °C to −60 °C, 3 h; (ii) PPh3CHCOOEt, THF, rt, 20 h, 92% (over two steps); (e) (i) DIBAL-H, CH2Cl2, −78 °C, 1 h; (ii) (S)-diphenylprolinol silyl ether (10 mol%), CH3NO2, benzoic acid, MeOH, rt, 16 h; (iii) oxone, DMF, rt, 12 h, 84% (over 3 steps); (f) TBAF, dry THF, rt, 2 h, 95%; (g) NaNO2, acetic acid, DMSO, rt, 24 h, 94%; (h) NaHMDS, CH3I, dry THF, −78 °C, 3 h, 93%. | |
Further to demonstrate the stereochemistry during the conjugate Michael addition of nitromethane to α,β-unsaturated aldehyde intermediate we carried out the reaction with racemic catalyst (±)-diphenylprolinol silyl ether to get the nitro-aldehyde adduct which on subsequent oxidation with oxone afforded the anti-/syn-nitro acid diastereomers (dr, 1
:
1) in 83% combined yield. However, on the other hand, in presence of (S)-diphenylprolinol silyl ether catalyst the conjugate addition of nitromethane on α,β-unsaturated aldehyde intermediate obtained from 20 followed by oxidation with oxone furnished the anti-nitro acid derivative 21 as a single diastereomer13 in 84% yield.
The anti-nitro acid derivative 21 on TPS deprotection and concomitant cyclisation with TBAF (tetra-n-butylammonium fluoride) furnished the γ-butyrolactone derivative 22 in 95% yield. The nitro-γ-butyrolactone derivative 22 was subjected to treatment with sodium nitrite and acetic acid under Nef reaction conditions14 to afford the γ-butyrolactone acid derivative 23 in 94% yield. Finally, stereoselective methylation at α-position of acid derivative 23 was carried out with methyl iodide and NaHMDS in dry THF to furnish the target compound (+)-nephrosteranic acid 1 in 93% yield ([α]25D + 27.18 (c 1.50, CHCl3), {lit.5s [α]27D + 27.2 (c 1.45, CHCl3)}. The spectral and physical properties of the (+)-nephrosteranic acid 1 were in full agreement with reported values.5a
Conclusions
In summary, we have developed an efficient and enantioselective route to multifunctionalized γ-butyrolactone paraconic acids and its application to the synthesis of the (+)-nephrosteranic acid 1 from readily accessible PMB (R)-glycidyl ether as starting material. Pivotal reaction sequence comprises asymmetric Michael addition catalyzed by (S)-diphenylprolinol silyl ether and stereoselective α-methylation. The overall yield for the (+)-nephrosteranic acid 1 was 47%. The synthetic route presented has further potential for the stereochemical variations in all the positions of the ring and extension to other analogues.
Conflicts of interest
There are no conflicts to declare.
Acknowledgements
S. K. P. is thankful to the Science and Engineering Research Board, New Delhi, for generous funding of the project (grant no. EMR/2016/003649). A. G. thank UGC, New Delhi for research fellowships.
Notes and references
- For reviews on synthesis and isolation of plant lignans, see:
(a) R. R. A. Kitson, A. Millemaggi and R. J. K. Taylor, Angew. Chem., Int. Ed., 2009, 48, 9426 CrossRef CAS PubMed;
(b) R. Bandichhor, B. Nosse and O. Reiser, Top. Curr. Chem., 2005, 243, 43 CrossRef CAS;
(c) M. Saleem, H. J. Kim, M. S. Ali and Y. S. Lee, Nat. Prod. Rep., 2005, 22, 696 RSC;
(d) S. S. C. Koch and A. R. Chamberlin, in Studies in Natural Product Chemistry, ed. Atta-ur-Rahman, Elsevier, New York, 1995, vol. 16, p. 687 Search PubMed.
-
(a) S. Rangaswami and V. Subba Rao, Indian J. Pharm., 1955, 17, 50 CAS;
(b) K. Aghoramurty, S. Neelakantan and T. R. J. Seshadri, Chem. Abstr., 1955, 49, 8900 (J. Sci. Ind. Res., Sect. B, 1954, 13, 326) Search PubMed;
(c) L. G. J. Shah, Ind. Chem. Soc., 1954, 31, 253 CAS;
(d) H. Gertig, Chem. Abstr., 1964, 60, 2041 (Diss. Pharm., 1963, 15, 235) Search PubMed;
(e) Y. Asahina and M. Yasue, Ber. Dtsch. Chem. Ges. B, 1937, 70, 1053 CrossRef;
(f) Y. Asahina and M. Yanagita, Ber. Dtsch. Chem. Ges. B, 1936, 69, 120 CrossRef;
(g) M. Asano and T. Kanematsu, Ber. Dtsch. Chem. Ges. B, 1932, 65, 1175 CrossRef;
(h) Y. Asahina and M. J. Asano, Pharm. Soc. Jpn., 1927, 539, 1 (Chem. Abstr., 1928, 22, 4470) Search PubMed;
(i) W. Zopf, Liebigs Ann. Chem., 1902, 324, 39 CrossRef.
-
(a) S. Drioli, F. Felluga, C. Forzato, P. Nitti and G. Pitacco, Chem. Commun., 1996, 1289 RSC;
(b) M. B. M. de Azevedo, M. M. Murta and A. E. Greene, J. Org. Chem., 1992, 57, 4567 CrossRef CAS;
(c) J. Bérdy, in Handbook of Antibiotic Compounds, CRS Press, Boca Raton, FL, 1982, vol. IX Search PubMed;
(d) S. Shibata, Y. Miura, H. Sugimura and Y. Toyoizumi, Chem. Abstr., 1951, 45, 6691 (J. Pharm. Soc. Jpn., 1948, 68, 300) Search PubMed;
(e) C. J. Cavallito, D. M. Freuhauf and J. H. Bailey, J. Am. Chem. Soc., 1948, 70, 3724 CrossRef CAS PubMed;
(f) S. Huneck and K. Schreiber, Phytochemistry, 1972, 11, 2429 CrossRef CAS.
-
(a) A. P. Pollnitz, G. P. Jones and M. A. Sefton, J. Chromatogr. A, 1999, 857, 239 CrossRef CAS PubMed;
(b) K. Otsuka, Y. Zenibayashi, M. Itoh and A. Totsuka, Agric. Biol. Chem., 1974, 38, 485 CrossRef CAS;
(c) R. E. Kepner, A. D. Webb and C. J. Muller, Am. J. Enol. Vitic., 1972, 23, 103 CAS.
-
(a) J. L. Nallasivam and R. A. Fernandes, Org. Biomol. Chem., 2017, 15, 708 RSC;
(b) H. Wang, P. Tang, Q. Zhou, D. Zhang, Z. Chen, H. Huang and Y. Qin, J. Org. Chem., 2015, 80, 2494 CrossRef CAS PubMed;
(c) J. Fournier, O. Lozano, C. Menozzi, S. Arseniyadis and J. Cossy, Angew. Chem., Int. Ed., 2013, 52, 1257 CrossRef CAS PubMed;
(d) B. Mao, K. Geurts, M. Fañanas-Mastral, A. W. van Zijl, S. P. Fletcher, A. J. Minnaard and B. L. Feringa, Org. Lett., 2011, 13, 948 CrossRef CAS PubMed;
(e) A. K. Perepogu, D. Raman, U. S. N. Murty and V. J. Rao, Synth. Commun., 2010, 40, 686 CrossRef CAS;
(f) S. Saha and S. C. Roy, Tetrahedron, 2010, 66, 4278 CrossRef CAS;
(g) R. Jongkol, R. Choommongkol, B. Tarnchompoo, P. Nimmanpipug and P. Meepowpan, Tetrahedron, 2009, 65, 6382 CrossRef CAS;
(h) M. Ghosh, S. Bose, S. Maity and S. Ghosh, Tetrahedron Lett., 2009, 50, 7102 CrossRef CAS;
(i) S. Hajra, A. Karmakar, A. K. Giri and S. Hazra, Tetrahedron Lett., 2008, 49, 3625 CrossRef CAS;
(j) D. Blanc, J. Madec, F. Popowyck, T. Ayad, P. Phansavath, V. Ratovelomanana-Vidal and J.-P. Genet, Adv. Synth. Catal., 2007, 349, 943 CrossRef CAS;
(k) S. Bazin, L. Feray, N. Vanthuyne, D. Siri and M. P. Bertrand, Tetrahedron, 2007, 63, 77 CrossRef CAS;
(l) N. Selvakumar, P. K. Kumar, K. C. S. Reddy and B. C. Chary, Tetrahedron Lett., 2007, 48, 2021 CrossRef CAS;
(m) G. P. Howell, S. P. Fletcher, K. Geurts, B. terHorst and B. L. Feringa, J. Am. Chem. Soc., 2006, 128, 14977 CrossRef CAS PubMed;
(n) S. Braukmuller and R. Brückner, Eur. J. Org. Chem., 2006, 2110 CrossRef;
(o) F. Schleth and A. Studer, Angew. Chem., Int. Ed., 2004, 43, 313 CrossRef CAS PubMed;
(p) F. Schleth, T. Vogler, K. Harms and A. Studer, Chem.–Eur. J., 2004, 10, 4171 CrossRef CAS PubMed;
(q) R. B. Chhor, B. Nosse, S. Sorgel, C. Bohm, M. Seitz and O. Reiser, Chem.–Eur. J., 2003, 9, 260 CrossRef CAS PubMed;
(r) M. Amador, X. Ariza, J. Garcia and J. Ortiz, J. Org. Chem., 2004, 69, 8172 CrossRef CAS PubMed;
(s) M. T. Barros, C. D. Maycock and M. R. Venture, Org. Lett., 2003, 5, 4097 CrossRef CAS PubMed;
(t) M. P. Sibi, P. Liu, J. Ji, S. Hajra and J.-X. Chen, J. Org. Chem., 2002, 67, 1738 CrossRef CAS PubMed;
(u) P. Kongsaeree, P. Meepowpan and Y. Thebtaranonth, Tetrahedron: Asymmetry, 2001, 12, 1913 CrossRef CAS;
(v) T.-P. Loh and P.-L. Lye, Tetrahedron Lett., 2001, 42, 3511 CrossRef CAS;
(w) Y. Masaki, H. Arasaki and A. Itoh, Tetrahedron Lett., 1999, 40, 4829 CrossRef CAS;
(x) S. Drioli, F. Felluga, C. Forzato, P. Nitti, G. Pitacco and E. Valentin, J. Org. Chem., 1998, 63, 2385 CrossRef CAS;
(y) M. P. Sibi and J. Ji, Angew. Chem., Int. Ed. Engl., 1997, 36, 274 CrossRef CAS;
(z) R. N. Saicic and S. Z. Zard, Chem. Commun., 1996, 1631 RSC;
(a
a) Z. Zhang and X. Lu, Tetrahedron: Asymmetry, 1996, 7, 1923 CrossRef CAS;
(a
b) T. Martin, C. M. Rodriguez and V. S. Martin, J. Org. Chem., 1996, 61, 6450 CrossRef CAS PubMed;
(a
c) S. D. Mawson and R. T. Weavers, Tetrahedron, 1995, 51, 11257 CrossRef CAS;
(a
d) H. Takahata, Y. Uchida and T. Momose, J. Org. Chem., 1995, 60, 5628 CrossRef CAS;
(a
e) M. M. Murta, M. B. M. de Azevedo and A. E. Greene, J. Org. Chem., 1993, 58, 7537 CrossRef CAS;
(a
f) S. Shimada, Y. Hashimoto and K. Saigo, J. Org. Chem., 1993, 58, 5226 CrossRef CAS.
- For formal synthesis of paraconic acids, see:
(a) C. B. Barreto Jr and V. L. P. Pereira, Tetrahedron Lett., 2009, 50, 6389 CrossRef;
(b) Y.-S. Hon, C.-H. Hsieh and H.-F. Chen, Synth. Commun., 2007, 37, 1635 CrossRef CAS;
(c) A. Brecht-Forster, J. Fitremann and P. Renaud, Helv. Chim. Acta, 2002, 85, 3965 CrossRef CAS;
(d) X. Ariza, J. Garcia, M. López and L. Montserrat, Synlett, 2001, 120 CrossRef CAS;
(e) P. K. Mandal and S. C. Roy, Tetrahedron, 1999, 55, 11395 CrossRef CAS;
(f) M. P. Sibi, K. P. Deshpande and A. J. L. Loggia, Synlett, 1996, 343 CrossRef CAS.
- A. M. Sarkale, V. Maurya, S. Giri and C. Appayee, Org. Lett., 2019, 21, 4266 CrossRef CAS PubMed.
-
(a) A. Kaur, R. Prakash and S. K. Pandey, ChemistrySelect, 2018, 3, 105 CrossRef CAS;
(b) Y. Garg, R. Kaur and S. K. Pandey, Eur. J. Org. Chem., 2017, 45, 6700 CrossRef;
(c) Y. Garg, P. Sharma and S. K. Pandey, Tetrahedron Lett., 2017, 58, 3493 CrossRef CAS;
(d) S. Gahalawat and S. K. Pandey, Tetrahedron Lett., 2017, 58, 2898 CrossRef CAS;
(e) S. Gahalawat and S. K. Pandey, Org. Biomol. Chem., 2016, 14, 9287 RSC;
(f) R. Kaur and S. K. Pandey, Tetrahedron: Asymmetry, 2016, 27, 338–340 CrossRef CAS;
(g) S. Gahalawat, Y. Garg and S. K. Pandey, Asian J. Org. Chem., 2015, 4, 1025 CrossRef CAS;
(h) Y. Garg, S. Gahalawat and S. K. Pandey, RSC Adv., 2015, 5, 38846 RSC;
(i) Y. Garg and S. K. Pandey, J. Org. Chem., 2015, 80, 4201 CrossRef CAS PubMed.
- M. Narasimhulu, S. S. Prasad, R. M. Appa, J. Lakshmidevi and K. Venkateswarlu, Arkivoc, 2018, iii, 326 Search PubMed.
-
(a) T. T. Tidwell, Synthesis, 1990, 10, 857 CrossRef;
(b) T. T. Tidwell, Org. React., 1990, 39, 297 CAS.
- H. Gotoh, H. Ishikawa and Y. Hayashi, Org. Lett., 2007, 9, 5307 CrossRef CAS PubMed.
- R. T. Benjamin, S. Meenakshi, G. O. Hollist and B. Borhan, Org. Lett., 2003, 5, 1031 CrossRef PubMed.
- The diastereoselectivity was determined from 1H-NMR and 13C-NMR spectral data.
-
(a) D. Polet and A. Alexakis, Tetrahedron Lett., 2005, 46, 1529 CrossRef CAS;
(b) C. Matt, A. Wagner and C. Mioskowski, J. Org. Chem., 1997, 62, 234 CrossRef CAS PubMed.
Footnote |
† Electronic supplementary information (ESI) available. See DOI: 10.1039/d0ra04267f |
|
This journal is © The Royal Society of Chemistry 2020 |