DOI:
10.1039/D0RA03977B
(Paper)
RSC Adv., 2020,
10, 36439-36451
Ionomic profiling of pericardial fluid in ischemic heart disease†
Received
2nd May 2020
, Accepted 27th September 2020
First published on 2nd October 2020
Abstract
Metals are essential cofactors that play a crucial role in heart function at the cell and tissue level. Information regarding the role of metals in the pericardial fluid and its ionome in ischemic heart disease (IHD) is limited. We aimed to determine the association of elements in pericardial fluid and serum samples of IHD patients and their correlation with systolic and diastolic function. IHD patients have been studied with systolic and diastolic dysfunction categorized on the basis of echocardiographic parameters. We measured concentrations of sixteen elements in the pericardial fluid and serum of 46 patients obtained during open heart surgery with IHD by ICP-MS. The levels of chromium and nickel in pericardial fluid were significantly higher as compared with serum samples of IHD patients (p < 0.05). The chromium, nickel and manganese levels in pericardial fluid were lower in patients with ejection fraction (EF) < 45% as compared to EF > 45% (p < 0.05). There was no significant difference in pericardial concentrations of elements in diastolic dysfunction grade 0–1 with 2 in IHD patients. We also found that decreased concentration of these elements in pericardial fluid is associated with decreased systolic function. These results suggest that pericardial fluid concentrations of these metals may reflect the extent of ischemic heart disease. These findings are hypothesis generating with regards to a role in the pathogenesis of the disorder.
Introduction
Micronutrients are essential cofactors needed only in small amounts for energy transfer in cells and thus play a crucial role in heart function at the cell and tissue level.1 These metals play role as regulators of oxidative stress, as antioxidants and as regulators of inflammatory response and immune cell activity.2 There is emerging evidence suggesting an important role of trace elements like chromium, zinc, cobalt, selenium, manganese and nickel in the heart and that their homeostasis imbalance may lead to an increase in the risk of cardiac remodelling in heart failure.3 Studies have shown that these micronutrients are intricately linked to Ischemic Heart Disease (IHD).4–7 It is well established that IHD is a global health issue and the major cause of mortality and morbidity worldwide.8,9
IHD is an important risk factor for the development of systolic and diastolic dysfunction of the heart and is intricately linked to their pathophysiology.10–12 Systolic dysfunction refers to the impaired pump function of the heart, whereas diastolic dysfunction refers to the increased resistance to filling of the heart with increased filling pressures.13 Echocardiography is a non-invasive method to assess the systolic as well as diastolic function or dysfunction.13 Systolic dysfunction is assessed by estimation of the ejection fraction (EF) and regional wall motion, whereas diastolic dysfunction can be diagnosed indirectly by means of a normal or nearly normal EF and changes of the mitral filling pattern.13 Several studies have shown that systolic or diastolic dysfunction, even if asymptomatic, may be a precursor to symptomatic heart failure which is linked to increased mortality.14,15 Recent guidelines also place special emphasis on the detection of subclinical left ventricular systolic and diastolic dysfunction and timely identification of risk factors.16
Pericardial fluid (PCF) is a dynamic and composite biological serous fluid, present within the pericardial cavity surrounding the heart fluids.17,18 This fluid is derived by plasma ultrafiltration through the epicardial capillaries and interstitial fluid from the underlying myocardium during the cardiac cycle.19 PCF is therefore in a strategic position to provide important information regarding the pathologies that distress the pericardial space as well as the heart itself. Studies in greyhounds have shown that the concentrations of sodium, chloride, calcium and magnesium were lower in the pericardial fluid than in the plasma. On the contrary, the concentration of potassium was higher than the plasma, which was attributed to the potassium leakage from the myocardial interstitium toward the pericardial cavity, during systole.20,21 The current research work on the redox nanomaterial was done on the ischemia heart disease, such as cerium oxide nanoparticles (nCe) have been used extensively, possess oxygen-modulating properties which have shown therapeutic utility in various disease models.22,23
Ben-Horin et al.24 had studied the composition of pericardial fluid in patients with elective open heart surgery but there is no study which reported the in-depth analysis of the PCF ionome particularly in IHD patients. This study is aimed to identify ionomic profiles of PCF and their paired serum samples in IHD patients with systolic and diastolic dysfunction. Identification and quantification of common and differential elements in these two biological fluids may be helpful in understanding the mechanism of disease development and thus ultimately prove helpful in devising interventions to delay the progression of IHD to heart failure by its early characterization.
Material and methods
Patient's selection and classification
Patients with confirmed IHD were admitted for coronary artery bypass grafting (CABG); and were selected for this study after informed consent. The study was approved by the Institutional Review Board (IRB) of ICCBS and the Ethics Review Committee (ERC) of the Aga Khan University. Pericardial fluid and blood samples were collected at the time of CABG procedure. Echocardiographic assessment of systolic and diastolic function and left ventricular (LV) morphology was done before start of the procedure and reported according to the American Society of Echocardiography (ASE) 2009 guidelines.
Patients were classified into different categories according to ejection fraction (EF < 45% and EF > 45%) for systolic function assessment; and in grades (0–1 and 2) for diastolic dysfunction as depicted in Table 1. In normal heart, EF is between 50 and 70%. In our study, EF < 45% is referred to as reduced ejection fraction and EF > 45% indicates preserved ejection fraction.
Table 1 Clinical characteristics of study patients with IHDa
Patient characteristics |
Serum samples |
PCF samples |
μ; mean, σ; standard deviation, BMI; body mass index, SBP; systolic blood pressure, DBP; diastolic blood pressure, BUN; blood urea nitrogen. |
Number of samples |
62 |
46 |
Gender (male/female) |
54/8 |
44/2 |
Age male (years; μ ± σ) |
59 ± 8 |
58 ± 7 |
Age female (years; μ ± σ) |
58 ± 10 |
65 ± 11 |
Height (cm) |
164 ± 9 |
166 ± 6 |
Body weight (kg) |
72 ± 12 |
75 ± 12 |
BMI (kg m−2) |
27 ± 5 |
27 ± 4 |
SBP (mm Hg) |
129 ± 19 |
130 ± 21 |
DBP (mm Hg) |
76 ± 9.69 |
77 ± 11 |
![[thin space (1/6-em)]](https://www.rsc.org/images/entities/char_2009.gif) |
Medical history |
Frequency |
Diabetes/non-diabetes |
37/25 |
25/21 |
Hypertension/non-hypertension |
41/21 |
29/17 |
Chest pain/non-chest pain |
48/14 |
32/14 |
Current smoker/non-smokers |
11/51 |
6/40 |
Hypercholesterolemia/non-hypercholesterolemia |
6/56 |
5/41 |
![[thin space (1/6-em)]](https://www.rsc.org/images/entities/char_2009.gif) |
Echocardiographic parameters |
Ejection fraction (N; <45%/>45%) |
12/50 |
12/34 |
Diastolic dysfunction grade (N; 0–1/2) |
45/9 |
36/5 |
![[thin space (1/6-em)]](https://www.rsc.org/images/entities/char_2009.gif) |
Biochemical parameters |
Creatinine (mg dL−1) |
0.99 ± 0.26 |
1.06 ± 0.26 |
BUN (mg dL−1) |
18 ± 8 |
17 ± 7 |
Random blood glucose (mg dL−1) |
184 ± 65 |
199 ± 61 |
Fasting blood glucose (mg dL−1) |
165 ± 58 |
169 ± 56 |
Diastolic dysfunction grades were of three types which include grade I (impaired relaxation of LV with or without increased in filling pressure), grade II (moderate increase in LV filling pressure with pseudo-normalization of LV) and grade III (marked increase in filling pressure with restrictive LV filling).25,26 Patients with malignancy, constrictive pericarditis and infiltrative, established pulmonary disease, renal insufficiency, moderate to severe valvular disease, hypertrophic cardiomyopathies and metabolic bone diseases were excluded from the study.
Sample collection and processing
The approved protocol from review board was followed for collection and storage of blood and PCF samples. 4 mL of blood sample was collected by the help of BD® Vacutainer tubes based on gel (BD Franklin Lakes NJ, USA, ref: 367381) from the patient. The serum samples were separated immediately by centrifugation at 2000 rpm for 10 min at 4 °C. After centrifugation, serum was aliquoted into locking Eppendorf tube stored at −80 °C until processing. Pericardial fluid was collected in clean and sterile falcon tubes. These tubes were centrifuged for about 15 min at 3500 rpm. The clear supernatant was collected and stored at −80 °C until further processing.
Reagents and standards
Extremely pure de-ionized water (resistivity 18.2 MΩ cm) was obtained from a Milli-Q water purification system (Thermo Fisher Scientific, MA USA). All reagents used during the study were of analytical grade. Trace metal grade ≥ 30% (v/v) concentrated H2O2 was obtained from Merck KGaA Company (Darmstadt, Germany). 70% (v/v) concentrated HNO3 (RCI Labscan Ltd, Bangkok, Thailand) was used during analysis after purification with NanoPure acid purification system (Nanonex, USA). Multi-element calibration standard (part number: 8500-6940) with concentration (10 μg mL−1) of each element, the tuning solution (1 μg L−1) concentration of Mg, Li, Tl, Y, Co and Ce in 2% (v/v) HNO3 and internal standard (Sc, Bi, Ir, Lu, Ge, Rh and Tb) of concentration (100 μg mL−1) were acquired from Agilent Analytical Technologies Company (Santa Clara, CA, USA). The optimization of ICP-MS was carried out by using tuning solution. All glassware, polypropylene tubes and bottles were acquired from Agilent Technologies (Santa Clara, CA, USA). All tubes and bottles were immersed overnight in 10% (v/v) HNO3 and cleaned by rinsing three times with high purity deionized water and kept in laminar-flow hood (Airstream® ESCO, Singapore) to dry. All operations were in a clean hood and working table to avoid of air contaminant postulate.
Preparation of standard solutions
To prepare all solutions, 5% (v/v) nitric acid was used as matrix. A total of sixteen points calibration curves (0.0076–1000 μg L−1) were prepared. A blank was also prepared with same matrix. The sensitivity was measure by least-square regression equation. The standard solution was also used for the validation of current study by calculating LOD, LOQ and correlation coefficient.
Preparation of the standard reference material
Certified reference material Seronorm™ serum L-1 (Sero, Billingstad, Norway) was use for validation of method. The validation was accomplished by precision and accuracy. The certified reference material (CRM) was prepared as per the manufacturer protocol. Briefly, the contents vial were completely dissolved by the addition of 3 mL of high purity de-ionized water. The vial content was smoothly rolled for 30 min so that all the contents were mixed entirely. The total quantity of material was transferred to polypropylene tubes and further diluted with sterile deionized water. The diluted solution was finally analyzed for each trace and ultra trace element through ICP-MS. The CRM is most used for serum but there is no standard reference material available for pericardial fluid to check the concentration of metals.
Sample preparation for ICP-MS
The digestion of serum and pericardial fluid were carried out in a closed vessel sealed microwave system equipped with 64MG5-T64 rotor (Anton Paar GmbH, Austria). In the microwave system, the program with multiwave ECO software (version 1.51) was setup. The standard apparatus such as polytetrafluoroethylene (PTFE) lip seal tube and screw cap (Wheaton® 15 × 45 mm, cap 13-425) were used during analysis.
For digestion of samples, a total of 50 μL aliquot of every sample was taken (serum and pericardial fluid), added in the MG5 vials (Anton Paar, Hungary). 50 μL of ≥30% (v/v) H2O2 and 150 μL of 70% (v/v) HNO3 were also added, mixed, and kept in laminar fuming hood for 10 minutes so that fumes were evolve. Vials were sealed with the PTFE lip and screw cap. Sealed vials were placed in microwave digestion system. The samples were digested in two steps via regulating same parameters in Anton Paar microwave digestion system with same fan (1), ramp (10.0 min), hold (30.0 min), power (850 W), stir rate (medium) but the temperature set 90 °C for step 1 while 150 °C for step 2. After the completion of digestion all sample vials were kept in laminar hood and allowed to cool at room temperature. Then gently pierced the septa by steal pin to release pressure of gas in vials, the resultant samples were took into 15 mL of polypropylene tubes and diluted with 3 mL of high purity deionized water. All samples were analyzed in triplicate on ICP-MS instrument.
Inductive coupled plasma-mass spectrometry (ICP-MS) analysis
The quantification of trace and ultra-trace elements were carried by Agilent 7700x ICP-MS system (version B.01.01) (Santa Clara, CA USA). The 7700x ICP-MS was improved with 1600 W power and it was operated at 27 MHz radio frequency (RF). To remove contamination from ICP-MS, washing solution containing ultra-pure water, 2% (v/v) HNO3 and 0.1% (v/v) HCl was analyzed after each sample. The Mass Hunter software was used for the operation of ICP-MS data. The acquisition parameter of Agilent 7700x ICP-MS are given in the ESI Table S1.†
ICP-MS data pre-processing and statistical analysis
The fully licenced Mass Profiler Professional (MMP) software were purchased form Agilent (Santa Clara, CA, USA) and used for data pre-treatment and statistical analysis. Filtering of the data involved using all available data and minimum absolute abundance of 10
000 counts with 3 number of ions/fragments minimum. Match factor 0.3, retention time tolerance 0.05 and delta MZ (low resolution) 0.2 were set as alignment parameters. External scalar was used for normalization of data. In this method of normalization, for lth samples an external scalar value “Sl” is subtracted from the abundance value “Ml” using the formula: normalized abundance = log2
Ml − log2
Sl. Z transform was selected as base lining option treating all the compounds equally irrespective of their intensity. After alignment of the data, entities were filtered by frequency (i.e. compounds appearing in more than 50% of samples in at least one group of samples).
Statistical analyses were carried out on PCF and serum samples of ischemic heart disease patients which were categorized on the basis of ejection fraction (<45% and >45%) as diastolic dysfunction and different grades (0–1 and 2) as systolic dysfunction. The statistical analysis was done by Mann–Whitney unpaired test for comparing two different groups. The p-value computation was done by asymptotically method and multiple test correction by Benjamini–Hochberg FDR. The analyzed variables with corrected p-value cut-off < 0.05 and fold change (FC) > 1.5 were selected as significant variables throughout the analysis.
SIMCA MKS Umetrics AB (version 14.1) software was used for multivariate analyses such as principal component analysis (PCA), partial least squares discriminant analysis (PLS-DA) and orthogonal partial least squares discriminant analysis (OPLS-DA) and their loading plots, which show overview, relation between the two groups of variables, class discrimination and variable correlation, respectively.
Method validation and data quality assessment
The concentration range 0.0076–1000 μg L−1 was selected for all elements to obtain linear calibration curve plot. The plot between concentration and count per seconds for each element was analysed by regression methods. The method of quantification was validated by limit of quantification (LOQ), correlation coefficients (R2) and limit of detection (LOD). The obtained correlation coefficients (R2) for all the element were in range of 0.993 and 1.000, shows good linear relationship. The formula of LOQ = 10σ/S and LOD = 3.3σ/S were used, where (S) is the slope and (σ) is standard deviation of the regression line. The range 0.002–9.551 μg L−1 and 0.006–28.941 μg L−1 were obtained for the optimized method for LOD and LOQ, respectively. The R2, LOD, LOQ values and regression equations are shown in ESI Table S2.†
The developed method was also validated externally to check the accuracy and precision for elements analysis in biological fluid by using Seronorm™ trace elements serum L-1 as certified reference material (CRM). The obtained data was found with nonsignificant variation and calculated value agrees with the theoretical certified values. The range of 81.879–113.779% were obtained for the percent recovery as shown in ESI Table S3.†
The spiking experiment were performed in real samples of serum and pericardial fluid for the validation of current method in order to check the precisions (RSD, %) and reliability. The precision of the developed method was calculated by RSD, % = [SD/CM] × 100, whereas SD and CM stands for standard deviation and measured mean concentration of elements. The percent RSD was found to be below 10%. The obtained coefficient of variation (% RSD) for the present method was in range of 0.052–7.580 and 0.076–5.733 for serum and pericardial fluid, respectively as given in the ESI Table S4.† This result shows the accuracy with good precision and sensitivity of our method.
Results
Ionomic profilling of pericardial fluid and serum for IHD patients
In this study element analysis was carried out through the ICP-MS for pericardial fluid and serum samples of the IHD patients. Significance testing was conducted on a total of 16 elements. From these, 14 elements were found to show significant differences at a probability level of <0.05. Out of these 14 elements, the concentration of twelve elements (copper, zinc, aluminum, manganese, lithium, cobalt, calcium, lead, silver, cadmium, selenium and arsenic) were higher while chromium and nickel were lower in serum samples in comparison to PCF samples of IHD patients as listed in Table 2. The heatmap of all significant elements with averaged normalized intensity of two groups is mentioned in ESI Fig. S1.†
Table 2 List of elements in pericardial fluid of IHD patients that are significantly different from serum of IHD patients. Mann–Whitney unpaired t-test was used with Benjamini–Hochberg FDR p-value correction method. Elements with p-value < 0.05 and fold change (FC) > 1.5 were referred as significant variables
Elements |
p (corr) |
log FC (PCF IHD/SR IHD) |
60Ni |
1.23 × 10−3 |
−0.6289 |
52Cr |
2.24 × 10−2 |
−0.4514 |
63Cu |
7.71 × 10−4 |
0.6560 |
66Zn |
2.45 × 10−5 |
0.7132 |
27Al |
3.79 × 10−5 |
0.7945 |
55Mn |
8.78 × 10−7 |
0.9292 |
7Li |
6.02 × 10−7 |
0.9446 |
59Co |
8.08 × 10−8 |
1.0070 |
44Ca |
5.10 × 10−18 |
1.4472 |
208Pb |
1.00 × 10−22 |
1.5683 |
107Ag |
1.00 × 10−27 |
1.6657 |
111Cd |
1.59 × 10−28 |
1.6806 |
78Se |
3.39 × 10−34 |
1.7604 |
75As |
0.00 × 100 |
1.9128 |
For further data mining, multivariate analysis was performed. The PCA was first performed in order to obtain a trend of separation of samples (Fig. 1A). This plot showed two components with value of R2X 0.376 and 0.167 on x-axis and y-axis, respectively. The score plot showed the outliers with confidence limit of 95% by Hotelling's T2-test, hence outliers were removed before further analyses. There are clear differences related to serum and PCF samples of IHD patients observed in PCA analysis. For classification analysis on groups, PLS-DA and OPLS-DA models were also generated by using normalized concentrations of significant elements. Both 3D score plot showed strong separation between two different biofluids (Fig. 1B and C).
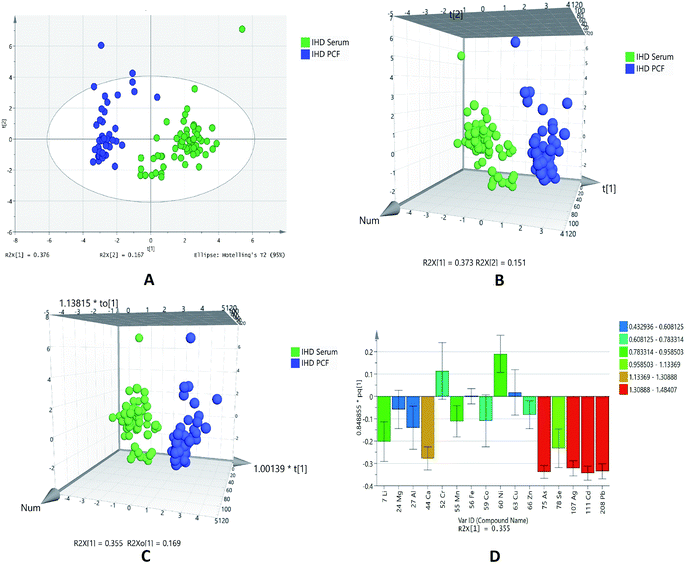 |
| Fig. 1 Scores scatter plots (A) PCA, (B) 3D PLS-DA, (C) 3D OPLS-DA and (D) OPLS-DA loadings plot colored as a function of VIP of serum (SR) (green) and pericardial fluid (PCF) from ischemic heart disease (IHD) (blue). | |
The sensitivity and specificity for the generated models were calculated as the number of diseases samples predicted as true positive divided by total number of disease samples and number of referents predicted as true negative divided by total number of referents, respectively. The models of PLSDA and OPLSDA shows 100% specificity, sensitivity and classification rate.
The model of OPLSDA was validated by ROC plot (ESI Fig. S2†) and permutation (ESI Fig. S3†); which showed least difference between predictive ability and goodness of fit of model with good area under the curve. In the OPLSDA loading plot (Fig. 1D), it is observed that elements like arsenic, silver, cadmium and lead is most responsible for group discrimination.
Ionomic profilling of pericardial fluid and serum for IHD patients with systolic dysfunction
The element concentrations studied in the pericardial fluid of patients with systolic dysfunction is based on the two groups, EF < 45% and EF > 45%. Table 3 shows significant metals in pericardial fluid based on groups EF < 45% and EF > 45%. It was found that only three metals, that are chromium, manganese and nickel, are statistically significant. These three metals are higher level in group EF > 45% as compared to EF < 45%. It seems that level of these metals is decreased as the ejection fraction reduces.
Table 3 List of elements in PCF of patients with EF > 45% and EF < 45% that are significantly different. Mann–Whitney unpaired t-test was used with Benjamini–Hochberg FDR p-value correction method. Elements with p-value < 0.05 and fold change (FC) > 1.5 were referred as significant variables
Elements |
p (corr) |
log FC (PCF EF < 45%/PCF EF > 45%) |
52Cr |
0.026399003 |
0.1544 |
55Mn |
0.026399003 |
0.1691 |
60Ni |
0.016587258 |
0.2560 |
Based on the normalized concentration of elements, PCA score plot in Fig. 2A did not show clear separation on the basis of ejection fraction. However, at 95% confidence limit from Hotelling's T2-test resulted in few outliers. The first component at x-axis gave more variance with the value of R2X 0.245 while the second component is 0.198. For class discrimination, all variables were used to perform PLSDA as shown in Fig. 2B. It was found that 3D-PLSDA score plot showed that samples were widely scattered and clear trend of separation in IHD patient with EF > 45% and < 45%. Addition of another orthogonal projection to the above model (Fig. 2C) did not affect the sensitivity and specificity of OPLS-DA model considerably. In loading plot, variables were arranged according to their performance for discrimination among the groups in the OPLS-DA model. Fig. 2D showed that nickel and manganese are mainly responsible for group separation.
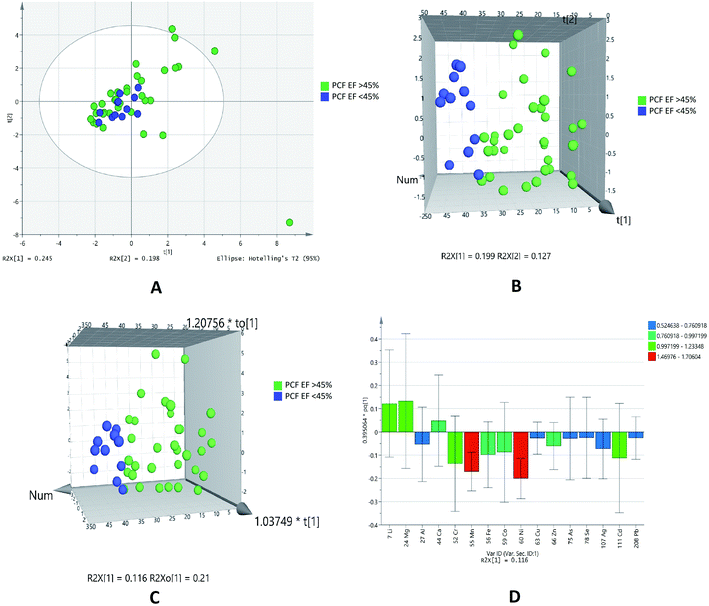 |
| Fig. 2 Scores scatter plots (A) PCA, (B) 3D PLS-DA, (C) 3D OPLS-DA and (D) OPLS-DA loadings plot of pericardial fluid in patients with EF > 45% (green) and EF < 45% (blue). | |
The statistical analyses of two different biofluids (PCF and serum) of IHD patients were carried out. We have also compared the data of paired samples of EF > 45% of IHD patients. It was found that out of sixteen elements, list of 14 was generated at probability of <0.05 in EF > 45% of pericardial fluid and serum samples of IHD. Out of fourteen elements zinc, copper, manganese, aluminum, cobalt, lithium, calcium, lead, cadmium, silver, selenium and arsenic were higher in concentration, while chromium and nickel were lower in PCF as compared to serum of IHD patients with EF > 45% as listed in Table 4.
Table 4 List of elements in pericardial fluid that are significantly different from serum of IHD patients with EF > 45%. Mann–Whitney unpaired t-test was used with Benjamini–Hochberg FDR p-value correction method. Elements with p-value < 0.05 and fold change (FC) > 1.5 were referred as significant variables
Elements |
p (corr) |
log FC (PCF EF > 45%/SR EF > 45%) |
60Ni |
1.06 × 10−4 |
−0.6999 |
52Cr |
0.0093986 |
−0.6493 |
66Zn |
0.002758518 |
0.5061 |
63Cu |
5.19 × 10−5 |
0.5391 |
55Mn |
0.005190234 |
0.6625 |
27Al |
1.16 × 10−4 |
0.8279 |
59Co |
4.02 × 10−5 |
0.9176 |
7Li |
5.68 × 10−14 |
0.9890 |
44Ca |
3.68 × 10−9 |
1.3989 |
208Pb |
3.03 × 10−13 |
1.6140 |
111Cd |
5.68 × 10−14 |
1.6268 |
107Ag |
4.08 × 10−13 |
1.6297 |
78Se |
5.16 × 10−13 |
1.7894 |
75As |
5.68 × 10−14 |
1.9070 |
The plots were drawn on the basis of normalized concentration of elements in serum and pericardial fluid of IHD patients with EF > 45%. The overview of samples can easily be seen with outliers at 95% confidence limit that generated from Hotelling's T2-test in PCA score plot (Fig. 3A). It was observed that component on x-axis R2X [1] 0.368 gave more variance as compared to R2X [2] 0.188. 3D PLSDA (Fig. 3B) and OPLSDA (Fig. 3C) score plots for showed clear trend of separation, distinction and discrimination between the EF > 45% groups. For the PLSDA and OPLSDA the sensitivity, specificity and classification rate were found to be 100%. Four elements, lead, cadmium, silver and arsenic were mainly accountable for group differentiation as shown in the VIP loading plot of OPLSDA (Fig. 3D).
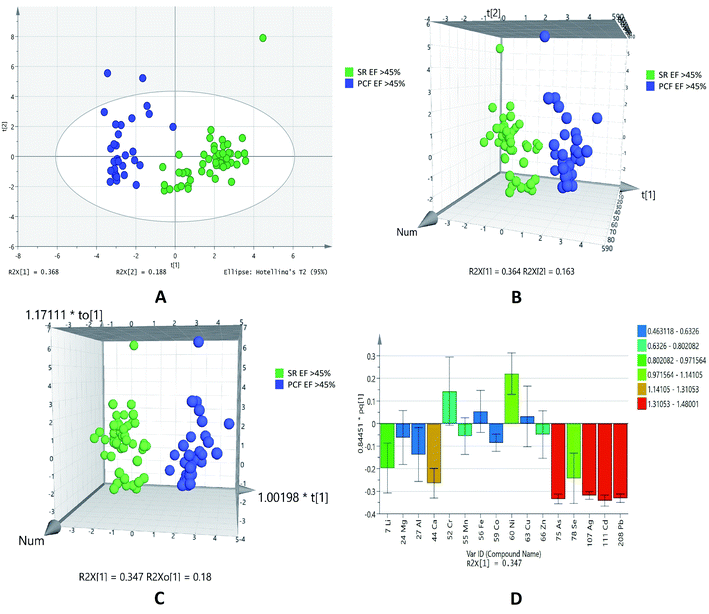 |
| Fig. 3 Scores scatter plots (A) PCA, (B) 3D PLS-DA, (C) 3D OPLS-DA and (D) OPLS-DA loadings plot colored as a function of VIP of serum (SR) EF > 45% (green) and pericardial fluid (PCF) EF > 45% from ischemic heart disease (IHD) (blue). | |
This type of comparison was performed as paired samples of EF < 45% of IHD patients were available. Out of 16 elements analyses, eleven elements (lithium, cobalt, copper, lead, zinc, selenium, calcium, manganese, silver, arsenic and cadmium) showed statistical significance. These elements were higher in serum as compared to PCF in patients with EF < 45% (Table 5).
Table 5 List of elements that are significantly different in serum as compared to pericardial fluid in patients with EF < 45%. Mann–Whitney unpaired t-test was used with Benjamini–Hochberg FDR p-value correction method. Elements with p-value < 0.05 and fold change (FC) > 1.5 were referred as significant variables
Elements |
p (corr) |
log FC (PCF EF < 45%/SR EF < 45%) |
7Li |
1.09 × 10−4 |
0.7856 |
59Co |
2.20 × 10−4 |
1.2880 |
63Cu |
0.0015 |
1.3165 |
208Pb |
2.98 × 10−5 |
1.4221 |
66Zn |
3.23 × 10−5 |
1.6478 |
78Se |
1.07 × 10−4 |
1.6500 |
44Ca |
6.78 × 10−5 |
1.6740 |
55Mn |
3.23 × 10−5 |
1.6763 |
107Ag |
3.23 × 10−5 |
1.7608 |
75As |
3.21 × 10−5 |
1.9190 |
111Cd |
3.20 × 10−5 |
1.9306 |
PCA (Fig. 4A) showed outliers in samples and data was more scattered. PLSDA (Fig. 4B) and OPLSDA (Fig. 4C) 3D score plot were also produce and it shows serum and PCF EF < 45% were clearly separated and differentiated with each other. However, the sensitivity, specificity and classification rate of the model are 100% for all. Lead, cadmium, silver, arsenic, calcium and manganese are most responsible elements in discrimination of serum and PCF EF < 45% (Fig. 4D).
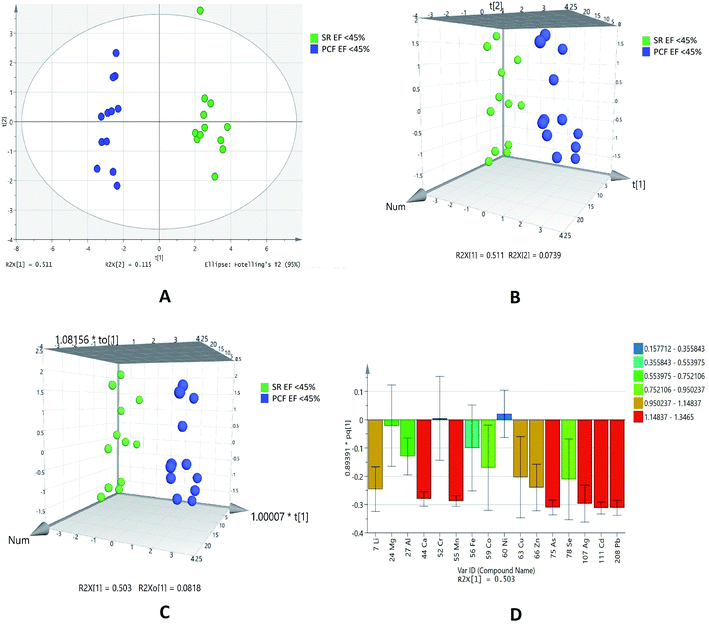 |
| Fig. 4 Scores scatter plots (A) PCA, (B) 3D PLS-DA, (C) 3D OPLS-DA and (D) OPLS-DA loadings plot colored as a function of VIP of serum (SR) EF < 45% (green) and pericardial fluid (PCF) EF < 45% from ischemic heart disease (IHD) (blue). | |
Ionomic profilling of pericardial fluid and serum for IHD patients with diastolic dysfunction (grade 0–1 and grade 2)
Diastolic dysfunction (DD) was determined by echocardiography according to defined criteria in American Society of Echocardiography (ASE) 2009 guidelines. In the PCA chemometric model of PCF grades, no separation trend in PCA was observed. This could possibly due to less number of samples in higher grades (Fig. 5A). The PLSDA and OPLSDA (Fig. 5B and C, respectively) models do not show visual separation of PCF grades of IHD patients. Due to less number of samples at higher grades of disease, it is unable to generate a specific model as required. It was found that PLSDA model showed 100% sensitivity and 40% specificity. In the loading plot of OPLSDA three elements were responsible for group discrimination, which were iron, manganese and cadmium (Fig. 5D). No element was found to be statistically significant while comparing grades of PCF with serum grades. Hence, further analyses related to parallel grades of PCF and serum were not performed.
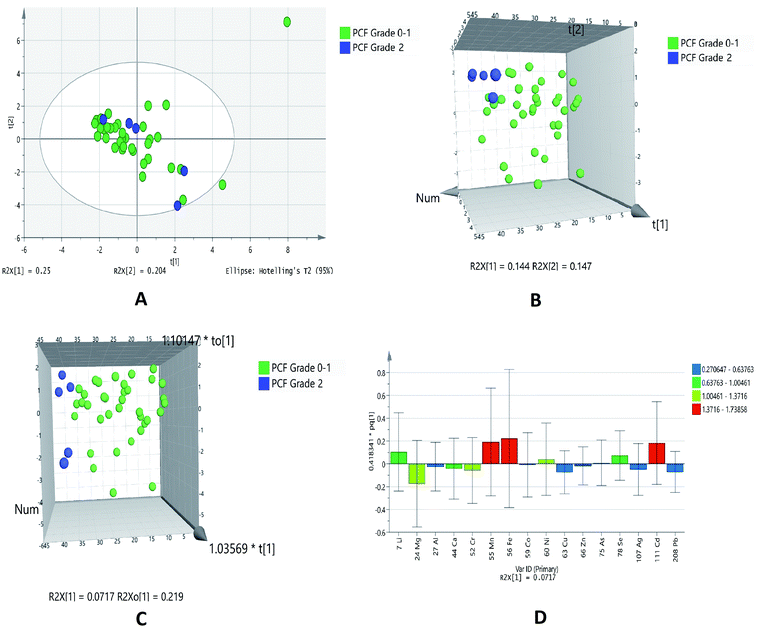 |
| Fig. 5 Scores scatter plots (A) PCA, (B) 3D PLS-DA, (C) 3D OPLS-DA and (D) OPLS-DA loadings plot of pericardial fluid with DD grade 0–1 (green) and DD grade 2 (blue) of diastolic dysfunctions. | |
Discussion
Our study is reporting quantification of metals (trace elements) in pericardial fluid and their comparison with serum levels in samples of patients with ischemic heart disease its correlation with severity of disease categorized on the basis of their systole function (i.e. EF < 45% and EF > 45%) and diastolic function (DD grades 0–1 and 2).
The results show clear image of elements that are differently regulated in IHD patients with systolic and diastolic dysfunctions. Our results describe the comparison of paired samples of pericardial fluid and serum of IHD patients.
We demonstrated that the level of chromium and nickel were higher in pericardial fluid, whereas, copper, zinc, aluminum, manganese, lithium, cobalt, calcium, lead, silver, cadmium, selenium and arsenic were lower in PCF of IHD patients when compared with their serum. Like other serosal fluids, pericardial fluid is believed to be a transudate created as a result of net hydrostatic pressure and osmotic gradient between pericardial fluid and plasma,24 but there is paucity of data regarding the concentration of these micronutrients in the pericardial fluid.
Our study found that the level of chromium, nickel and manganese are higher in the PCF of patients with EF > 45 as compared to EF < 45. When the pericardial fluid was analyzed for diastolic dysfunction grades, the results were not conclusive.
Chromium is important for various functions in the body, a major role is in the metabolism of lipid and carbohydrate.27 Chromium is involved in insulin signaling and promotes enhancement of insulin sensitivity, a role well recognized in reducing the risk of cardiovascular diseases and type 2 diabetes.28 It is also important in the maintenance of glucose tolerance and redox reaction in the cell.29,30 It has been demonstrated that chromium inhibits the glycosylation of proteins and oxidative stress in erythrocytes,31 both risk factors in the development of cardiovascular diseases. Studies have shown that chromium reduced systolic blood pressure and ameliorated insulin resistance in both animals and human subjects.32,33 It has been reported that chromium concentrations in the blood stream are lower in patients with coronary artery disease than in subjects with normal arteries34 which suggested that a risk factor for cardiovascular disease might be chromium deficiency.35
The essential role of nickel consists of the action or formation of cyclic guanosine monophosphate, a signaling agent that regulates various physiological processes including blood pressure control, sperm physiology, sodium metabolism among many others.36 In animals, nickel deficiency inhibits growth, reduces reproductive rates, and alters glucose and lipid metabolism that are associated with anemia, hemoglobin reduction, alternations of metal ion contents, and reduced activity of several enzymes.37,38 Toxic exposure to nickel, however, can cause cardiac dysfunction due to reactive oxygen and free radical cellular damage.39 Ni ions have been reported to induce vasoconstriction and early after depolarization in isolated rat heart and canine coronary artery by enhancing Ca ion influx into vascular smooth muscle cells.40–42
Chromium and nickel were found as significant element in this study, however, from the bio-inorganic chemistry field there is no clear evidence of the biological role of these elements in humans (or mammals), although for nickel the biological role in bacteria, archaea, fungi and plants is well established as mentioned in the above paragraphs. Obviously, these elements are present in the environment and will occur in biological samples as contaminants. Furthermore, it is clear that various ions of chromium or nickel have toxic (and allergic) effects that are well described.43–45 Hence it suggests that these elements in IHD patients might be a source of contamination.
Manganese was also found to be significantly higher in PCF of patients with EF > 45%. Manganese, another trace element, is a vital and an essential part of many enzyme systems46 e.g., superoxide dismutase, succinate dehydrogenase, glutamine synthetase, diamine oxidase, arginase, pyruvate carboxylate and phosphoglucomutase.47 Manganese is particularly important in superoxide dismutase and adenylyl cyclase, the two antioxidant enzymes fighting oxidative stress in the body.48 It plays a crucial role in the vascular contractility and low level of manganese resulted in decreased superoxide dismutase effect.49 Manganese plays important role in the cholesterol metabolism, oxidative phosphorylation, fatty acids, urea cycle and mucopolysaccharide metabolism.50 Many metabolic processes are carried by metalloprotein and metal binding proteins; the metals, manganese, nickel, iron, magnesium and zinc work as cofactors.38,51 Studies have shown that the serum levels of manganese are lower in patients with atherosclerosis and it decreases with the severity of the disease,52 however, some conflicting reports indicate that high level of manganese was observed in coronary artery disease as compared to healthy subjects.53 A study done on the aortic tissue of normal as well as atherosclerotic patients, showed low level of manganese, however there was no information related to cause and effect.54
This is interesting to note that in the group of IHD patients with EF > 45% the PCF had higher levels of chromium, nickel and manganese as compared to the group with EF < 45% (Fig. 2). If PCF of EF > 45% was compared with serum of EF > 45% we found higher levels of manganese and lower levels of chromium and nickel in the PCF compared to serum of patients with EF > 45% (Fig. 3). If PCF of EF < 45% is compared with serum of patients with EF < 45%, we found that only manganese was lower in PCF, the other two metals failed to show any significant association (Fig. 4).
We do not know why pericardial fluid levels and serum levels are showing different trends of up and down regulation of these metals. The only link we seem to find which is substantiated by previous work is that pericardial fluid chromium, nickel and manganese levels fall with decreasing ejection fraction which corresponds to the severity of the disease. We also found that the concentration of chromium and nickel were lower in serum as compared to PCF of IHD patients with EF > 45% and chromium and nickel were not picked up in the analysis of PCF and serum of patients with EF < 45% (Fig. 5).
We can say that chromium and nickel together are present in significantly higher levels in patients with EF > 45% as compared to EF < 45% and this is reflected in the PCF more strongly than serum.
Conclusion
Our study has shown that pericardial concentrations of essential trace element chromium, nickel and manganese were significantly higher in the IHD group than in the serum of IHD patients. We also found that concentration of these elements in pericardial fluid is associated with decreased systolic function. These results suggest that pericardial fluid concentrations of these metals may reflect the extent of ischemic heart disease and may also play a role in the pathogenesis of the disorder. As the pericardial fluid cannot be obtained from normal subjects, hence no information is available for the physiological concentrations of these metals. We only had a few cases of advanced diastolic dysfunction so it may be postulated that our study lacked the power to detect a difference in ionome in diastolic dysfunction. However, the present results indicate that pericardial fluid, once it is obtained, maybe a useful source for examining the severity and extent of coronary artery disease.
Authors' contributions
NK: performed experiment, data analysis, interpretation and manuscript writing. SH: study design, patient's recruitment, data collection, samples collection, results interpretation and manuscript writing. AJS: experimental guidance, data analysis and interpretation. SF: data analysis, results interpretation. SAS: clinical examination of CABG patients, recruitment and results interpretation. NB: clinical examination, echocardiography and results interpretation. SSB: echocardiography and results interpretation. HS: clinical examination of CABG patients, recruitment and results interpretation. SJ: patient recruitment, samples and patients case history collection. SGM: study design, experimental design, results interpretation and manuscript writing. All authors read and approved the final manuscript.
Compliance with ethical standards
All procedures performed in studies involving human participants were in accordance with the ethical standards of the institutional and/or national research committee and with the 1964 Helsinki declaration and its later amendments or comparable ethical standards.
Abbreviations
PCF | Pericardial fluid |
EF | Ejection fraction |
SR | Serum |
FC | Fold change |
PCA | Principal component analysis |
PLS-DA | Partial least squares discriminant analysis |
OPLS-DA | Orthogonal partial least squares discriminant analysis |
IHD | Ischemic heart disease |
DD | Diastolic dysfunctions |
μ | Mean |
σ | Standard deviation |
BMI | Body mass index |
SBP | Systolic blood pressure |
DBP | Diastolic blood pressure |
BUN | Blood urea nitrogen |
Conflicts of interest
The authors have no relationships with industry to report and have no conflict of interest including financial, personal, political interest in this study.
Acknowledgements
We are thankful to the Aga Khan University and South City Hospitals cardiologists and cardiothoracic surgeons for their invaluable work in patient's recruitment, samples and data collection. We also acknowledged the financial support from the Pakistan Academy of Sciences (project I.D. 5-9/PAS/745) for conducting this research. Noman (pin no. 2PS5-089) is grateful to the Higher Education Commission, Government of Pakistan for financial support in the PhD program. Authors are thankful to Mr Arsalan Tahir, Junaid-ul-Haq for his technical assistance in ICP-MS analysis.
References
- V. Soukoulis, J. B. Dihu, M. Sole, S. D. Anker, J. Cleland, G. C. Fonarow, M. Metra, E. Pasini, T. Strzelczyk and H. Taegtmeyer, Micronutrient deficiencies: an unmet need in heart failure, J. Am. Coll. Cardiol., 2009, 54, 1660–1673 CrossRef CAS.
- N. A. McKeag, M. C. McKinley, J. V. Woodside, M. T. Harbinson and P. P. McKeown, The role of micronutrients in heart failure, J. Acad. Nutr. Diet., 2012, 112, 870–886 CrossRef CAS.
- K. T. Weber, W. B. Weglicki and R. U. Simpson, Macro- and micronutrient dyshomeostasis in the adverse structural remodelling of myocardium, Cardiovasc. Res., 2009, 81, 500–508 CrossRef CAS.
- F. Jossa, M. Trevisan, V. Krogh, E. Farinaro, D. Giumetti, G. Fusco, R. Galasso, S. Paneco, S. Frascatore and C. Mellone, Serum selenium and coronary heart disease risk factors in southern Italian men, Atherosclerosis, 1991, 87, 129–134 CrossRef CAS.
- J. Salonen, G. Alfthan, J. Huttunen, J. Pikkarainen and P. Puska, Association between cardiovascular death and myocardial infarction and serum selenium in a matched-pair longitudinal study, Lancet, 1982, 320, 175–179 CrossRef.
- M. Tellez-Plaza, M. R. Jones, A. Dominguez-Lucas, E. Guallar and A. Navas-Acien, Cadmium exposure and clinical cardiovascular disease: a systematic review, Curr. Atheroscler. Rep., 2013, 15, 356 CrossRef.
- H. P. Kodali, B. T. Pavilonis and C. M. Schooling, Effects of copper and zinc on ischemic heart disease and myocardial infarction: a Mendelian randomization study, Am. J. Clin. Nutr., 2018, 108, 237–242 CrossRef.
- A. T. F. Members, J. J. McMurray, S. Adamopoulos, S. D. Anker, A. Auricchio, M. Böhm, K. Dickstein, V. Falk, G. Filippatos and C. Fonseca, ESC guidelines for the diagnosis and treatment of acute and chronic heart failure 2012: the task force for the diagnosis and treatment of acute and chronic heart failure 2012 of the European Society of Cardiology. Developed in collaboration with the Heart Failure Association (HFA) of the ESC, Eur. J. Heart Failure, 2012, 14, 803–869 CrossRef.
- C. W. Yancy, M. Jessup, B. Bozkurt, J. Butler, D. E. Casey, M. H. Drazner, G. C. Fonarow, S. A. Geraci, T. Horwich and J. L. Januzzi, 2013 ACCF/AHA Guideline for the Management of Heart Failure: A Report of the American College of Cardiology Foundation/American Heart Association Task Force on Practice Guidelines, J. Am. Coll. Cardiol., 2013, 62, e147–e239 CrossRef.
- T. A. McDonagh, C. E. Morrison, A. Lawrence, I. Ford, H. Tunstall-Pedoe, J. J. McMurray and H. J. Dargie, Symptomatic and asymptomatic left-ventricular systolic dysfunction in an urban population, Lancet, 1997, 350, 829–833 CrossRef CAS.
- J. M. Aroesty, R. G. McKay, G. V. Heller, H. D. Royal, A. Als and W. Grossman, Simultaneous assessment of left ventricular systolic and diastolic dysfunction during pacing-induced ischemia, Circulation, 1985, 71, 889–900 CrossRef CAS.
- A. Jamiel, A. M. Ahmed, I. Farah and M. H. Al-Mallah, Correlation between diastolic dysfunction and coronary artery disease on coronary computed tomography angiography, Heart Views, 2016, 17, 13 CrossRef.
- M. Federmann and O. Hess, Differentiation between systolic and diastolic dysfunction, Eur. Heart J., 1994, 15, 2–6 CrossRef.
- J. D. Sara, T. Toya, R. Taher, A. Lerman, B. Gersh and N. S. Anavekar, Asymptomatic Left Ventricle Systolic Dysfunction, Euro. Cardio. R., 2020, 15, e13 Search PubMed.
- X. Ren, B. Ristow, B. Na, S. Ali, N. B. Schiller and M. A. Whooley, Prevalence and prognosis of asymptomatic left ventricular diastolic dysfunction in ambulatory patients with coronary heart disease, Am. J. Cardiol., 2007, 99, 1643–1647 CrossRef.
- T. Kuznetsova, L. Herbots, B. Lopez, Y. Jin, T. Richart, L. Thijs, A. Gonzalez, M. Herregods, R. Fagard and J. Diez, Prevalence of left ventricular diastolic dysfunction in a general population, Circ.: Heart Failure, 2009, 2(2), 105–112 Search PubMed.
- F. W. Maurer, M. F. Warren and C. K. Drinker, The composition of mammalian pericardial and peritoneal fluids: studies of their protein and chloride contents, and the passage of foreign substances from the blood stream into these fluids, Am. J. Physiol., 1940, 129, 635–644 CrossRef CAS.
- K. Vogiatzidis, S. G. Zarogiannis, I. Aidonidis, E. I. Solenov, P.-A. Molyvdas, K. I. Gourgoulianis and C. Hatzoglou, Physiology of pericardial fluid production and drainage, Front. Physiol., 2015, 6, 62 Search PubMed.
- R. H. Stewart, D. A. Rohn, S. J. Allen and G. A. Laine, Basic determinants of epicardial transudation, Am. J. Physiol.: Heart Circ. Physiol., 1997, 273, H1408–H1414 CrossRef CAS.
- A. Gibson and M. Segal, A study of the composition of pericardial fluid, with special reference to the probable mechanism of fluid formation, J. Physiol., 1978, 277, 367–377 CrossRef CAS.
- J. P. Holt, The normal pericardium, Am. J. Cardiol., 1970, 26, 455–465 CrossRef CAS.
- A. Jain, M. Behera, C. Mahapatra, N. R. Sundaresan and K. Chatterjee, Nanostructured Polymer Scaffold Decorated with Cerium Oxide Nanoparticles Toward Engineering an Antioxidant and Anti-hypertrophic Cardiac Patch, Mater. Sci. Eng., C, 2020, 111416 Search PubMed.
- I.-S. Park, C. Mahapatra, J. S. Park, K. Dashnyam, J.-W. Kim, J. C. Ahn, P.-S. Chung, D. S. Yoon, N. Mandakhbayar and R. K. Singh, Revascularization and limb salvage following critical limb ischemia by nanoceria-induced Ref-1/APE1-dependent angiogenesis, Biomaterials, 2020, 119919 CrossRef CAS.
- S. Ben-Horin, A. Shinfeld, E. Kachel, A. Chetrit and A. Livneh, The composition of normal pericardial fluid and its implications for diagnosing pericardial effusions, Am. J. Med., 2005, 118, 636–640 CrossRef.
- S. R. Ommen, R. A. Nishimura, C. P. Appleton, F. Miller, J. K. Oh, M. M. Redfield and A. Tajik, Clinical utility of Doppler echocardiography and tissue Doppler imaging in the estimation of left ventricular filling pressures: a comparative simultaneous Doppler-catheterization study, Circulation, 2000, 102, 1788–1794 CrossRef CAS.
- S. J. Khouri, G. T. Maly, D. D. Suh and T. E. Walsh, A practical approach to the echocardiographic evaluation of diastolic function, J. Am. Soc. Echocardiogr., 2004, 17, 290–297 CrossRef.
- S. K. Panchal, S. Wanyonyi and L. Brown, Selenium, vanadium, and chromium as micronutrients to improve metabolic syndrome, Curr. Hypertens. Rep., 2017, 19, 10 CrossRef.
- M. Hummel, E. Standl and O. Schnell, Chromium in metabolic and cardiovascular disease, Horm. Metab. Res., 2007, 39, 743–751 CrossRef CAS.
- A. A. Tinkov, E. V. Popova, V. S. Polyakova, O. V. Kwan, A. V. Skalny and A. A. Nikonorov, Adipose tissue chromium and vanadium disbalance in high-fat fed Wistar rats, J. Trace Elem. Med. Biol., 2015, 29, 176–181 CrossRef CAS.
- S. Lewicki, R. Zdanowski, M. Krzyzowska, A. Lewicka, B. Debski, M. Niemcewicz and M. Goniewicz, The role of chromium III in the organism and its possible use in diabetes and obesity treatment, Ann. Agric. Environ. Med., 2014, 21, 331–335 CrossRef CAS.
- S. K. Jain, P. Patel, K. Rogier and S. K. Jain, Trivalent chromium inhibits protein glycosylation and lipid peroxidation in high glucose-treated
erythrocytes, Antioxid. Redox Signaling, 2006, 8, 238–241 CrossRef CAS.
- H. G. Preuss, B. Echard, D. Bagchi and N. V. Perricone, Comparing effects of carbohydrate (CHO) blockers and trivalent chromium on CHO-induced insulin resistance and elevated blood pressure in rats, J. Am. Coll. Nutr., 2013, 32, 58–65 CrossRef CAS.
- A. E. Yanni, N. S. Stamataki, P. Konstantopoulos, M. Stoupaki, A. Abeliatis, I. Nikolakea, D. Perrea, V. T. Karathanos and N. Tentolouris, Controlling type-2 diabetes by inclusion of Cr-enriched yeast bread in the daily dietary pattern: a randomized clinical trial, Eur. J. Nutr., 2018, 57, 259–267 CrossRef CAS.
- H. A. Newman, R. Leighton, R. Lanese and N. Freedland, Serum chromium and angiographically determined coronary artery disease, Clin. Chem., 1978, 24, 541–544 CrossRef CAS.
- M. Simonoff, Chromium deficiency and cardiovascular risk, Cardiovasc. Res., 1984, 18, 591–596 CrossRef CAS.
- K. Yokoi, E. Uthus and F. Nielsen, The essential use of nickel affects physiological functions regulated by the cyclic-GMP signal transduction system, in Proceedings of the 7th international symposium on metal ions in biology and medicine, St. Petersburg, Russia, 2002, pp. 5–9 Search PubMed.
- L. Samal and C. Mishra, Significance of nickel in livestock health and production, Int. J. Agro Vet. Med. Sci., 2011, 5, 349–361 Search PubMed.
- J. Šimková, M. Milkovičová, M. Valko-Rokytovská, Z. Kostecká, E. Bencúrová, L. Pulzová, Ľ. Čomor and M. Bhide, Analysis of Nickel-Binding Proteins from Various Animal Sera, Folia Vet., 2018, 62, 59–66 Search PubMed.
- E. Novelli, Y. Diniz, T. Machado, V. Proenca, T. Tibiricá, L. Faine, B. Ribas and J. Almeida, Toxic mechanism of nickel exposure on cardiac tissue, Toxic Subst. Mech., 2000, 19, 177–187 CrossRef CAS.
- V. Golovko, I. Bojtsov and L. Kotov, Single and multiple early afterdepolarization caused by nickel in rat atrial muscle, Gen. Physiol. Biophys., 2003, 22, 275–278 CAS.
- A. Koller, G. Rubanyi, L. Ligeti and A. Kovach, Effect of verapamil and phenoxybenzamine on nickel-induced coronary vasoconstriction in the anaesthetized dog, Acta Physiol. Acad. Sci. Hung., 1982, 59, 287–290 CAS.
- G. Rubanyi and A. Kovach, Cardiovascular actions of nickel ions, Acta Physiol. Acad. Sci. Hung., 1980, 55, 345–353 CAS.
- J. B. Vincent, The biochemistry of chromium, J. Nutr., 2000, 130, 715–718 CrossRef CAS.
- F. W. Sunderman Jr, M. I. Decsy and M. D. McNeely, Nickel metabolism in health and disease, Ann. N. Y. Acad. Sci., 1972, 199, 300–312 CrossRef CAS.
- K. R. Di Bona, S. Love, N. R. Rhodes, D. McAdory, S. H. Sinha, N. Kern, J. Kent, J. Strickland, A. Wilson and J. Beaird, Chromium is not an essential trace element for mammals: effects of a “low-chromium” diet, JBIC, J. Biol. Inorg. Chem., 2011, 16, 381–390 CrossRef CAS.
- C. E. Ekpenyong, Essential trace element and mineral deficiencies and cardiovascular diseases: facts and controversies, Int. J. Nutr. Food Sci., 2017, 6, 53 CrossRef CAS.
- L. Prashanth, K. K. Kattapagari, R. T. Chitturi, V. R. R. Baddam and L. K. Prasad, A review on role of essential trace elements in health and disease, J. NTR Univ. Health Sci., 2015, 4, 75 CrossRef.
- H. Fujimoto, J.-i. Taguchi, Y. Imai, S. Ayabe, H. Hashimoto, H. Kobayashi, K. Ogasawara, T. Aizawa, M. Yamakado and R. Nagai, Manganese superoxide dismutase polymorphism affects the oxidized low-density lipoprotein-induced apoptosis of macrophages and coronary artery disease, Eur. Heart J., 2008, 29, 1267–1274 CrossRef CAS.
- M. C. P. Franco, A. P. V. Dantas, E. H. Akamine, E. M. Kawamoto, Z. B. Fortes, C. Scavone, R. C. Tostes, M. H. C. Carvalho and D. Nigro, Enhanced oxidative stress as a potential mechanism underlying the programming of hypertension in utero, J. Cardiovasc. Pharmacol., 2002, 40, 501–509 CrossRef CAS.
- G. L. Rehnberg, J. F. Hem, S. D. Carter, R. S. Linko and J. W. Laskey, Chronic ingestion of Mn3O4 by rats: tissue accumulation and distribution of manganese in two generations, J. Toxicol. Environ. Health, Part A, 1982, 9, 175–188 CAS.
- W. Shi, C. Zhan, A. Ignatov, B. A. Manjasetty, N. Marinkovic, M. Sullivan, R. Huang and M. R. Chance, Metalloproteomics: high-throughput structural and functional annotation of proteins in structural genomics, Structure, 2005, 13, 1473–1486 CrossRef CAS.
- B. Bagheri, M. Shokrzadeh, N. Akbari, V. Mokhberi, S. Azizi, A. Khalilian, M. Nabati, M. Dabirian and R. Piran, The relationship between serum level of manganese and severity of coronary atherosclerosis, Zahedan J. Res. Med. Sci., 2015, 17, 30–33 CAS.
- J. Manthey, M. Stoeppler, W. Morgenstern, E. Nüssel, D. Opherk, A. Weintraut, H. Wesch and W. Kübler, Magnesium and trace metals: risk factors for coronary heart disease? Association between blood levels and angiographic findings, Circulation, 1981, 64, 722–729 CrossRef CAS.
- S. Mendis, Magnesium, zinc, and manganese in atherosclerosis of the aorta, Biol. Trace Elem. Res., 1989, 22, 251–256 CrossRef CAS.
Footnote |
† Electronic supplementary information (ESI) available. See DOI: 10.1039/d0ra03977b |
|
This journal is © The Royal Society of Chemistry 2020 |
Click here to see how this site uses Cookies. View our privacy policy here.