DOI:
10.1039/D0RA03873C
(Paper)
RSC Adv., 2020,
10, 27280-27287
Innovative induction heating of grapefruit juice via induced electric field and its application in Escherichia coli O157:H7 inactivation
Received
29th April 2020
, Accepted 13th July 2020
First published on 21st July 2020
Abstract
The proposed induction heating method was applied in the pasteurization of grapefruit juice. In this processing, an alternating magnetic field acted as the stimulus instead of conventional electrodes to create an induced electric field (IEF) for heat treatment of the continuous-flow juice sample, which excluded the possibility of electrochemical reaction and electrode corrosion that might occur in conventional electric field treatments. As a typical food pathogen, Escherichia coli O157:H7 was selected as a representative to investigate its inactivation by the heating process under different voltages and frequencies, initial temperatures, and flow rates (or retention time). The grapefruit juice was successfully heated up by IEF and the temperature curve was achieved when the juice exposed to IEF. The heating rate and terminal temperature increased with the increasing induced voltage, decreasing frequency and at higher initial temperature. A highest terminal temperature of 93.7 °C for grapefruit juice with an initial temperature of 20 °C was achieved under induced voltage of 2700 V, frequency of 300 Hz and residence time of 400 s. At the same time, E. coli O157:H7 in the grapefruit juice was thoroughly inactivated. There was a trend that the pathogen survival rate was reduced at higher induced voltage, lower frequency and higher initial temperature during the heating treatment. No significant changes in pH and °Brix was observed after this innovative induction heating, but the color of grapefruit juice was brightened. The proposed induction heating can be regarded as a sister technology of ohmic heating, and it provide a reference for the application of this heating method in liquid food pasteurization.
1. Introduction
The food industry aims to satisfy consumer expectations of products with unmodified taste, nutritional value, and microbial safety. Conventional thermal pasteurization ensures food safety and extends shelf life while causing nutritional loss due to low-efficiency heat transfer and long-term treatment.1,2 In recent decades, research on the use of non-conventional thermal technologies to the preparation of food with minimal damage to its physicochemical properties and without adding chemical preservatives, continues to increase. For example, radio-frequency heating,3,4 microwave heating,5,6 ohmic heating,7,8 which enable rapid heating by electromagnetic waves or electric fields, are widely investigated.
Non-conventional techniques are also commonly targeted for the inactivation of microorganisms in liquids foods, among which ohmic heating and pulsed electric field are two common electric field methods.9,10 In particular, ohmic heating technique is examined more superior because of its uniform heat generation as well as high bactericidal efficiency under the combining non-thermal effect and thermal effect. The bactericidal efficiency often depends on the electrical conductivity, pH value, electric field strength and frequency as well as the electrodes shapes and configuration.11,12 However, undesirable electrochemical reactions between electrode and solution interfaces often take place since the inevitable use of electrodes for the generation of electric field or electric current.13 The more acidic food material, the more potentially electrochemical reactions take place, under the electric field, especially at rigorous heat or high current density conditions. The electrode corrosion, solution electrolysis, and electro-deposition may decrease the processing efficiency and degrade the food quality, which hinders the development of electric field processing in food industry.
Based on the magnetoelectric effect, an induced electric field (IEF) can be directly generated in food matrix via alternating magnetic flux, while not using any electrodes. On this occasion, food and metal plates do not contact anymore and electrochemical reactions in traditional electric field processing can be avoided. Theoretically, the current induced inside the food can result in the temperature rise. However, few direct induction heating of food has been reported in the literates, which is owing to the supporting tube of food has great impedance. The induced current or the induced current density is extremely-low nearby the magnetic field which cannot produce significant thermal effect. Moreover, unlike iron-based materials with high magnetic permeability, the food matrix hardly presents a magnetocaloric effect under an alternating magnetic field. Therefore, it is meaningful to research direct induction heating of food.
Grapefruit juices are popular for its pink color, bitter–sour taste, and rich vitamins, which is pleasing, refreshing and considered to be health-beneficial.14,15 It is also an abundant source of biomolecules, such as anthocyanins, polysaccharides, flavonoids and lipids, and thus has a propensity to become contaminated by microbes and then deteriorates. To our knowledge, the inactivation of pathogens in fruit juices under an IEF has never been presented to data due to the lack of experimental observation of thermal effect.16 For the IEF processing, continuous-flow grapefruit juice acted as the secondary winding coils of a transformer, under magnetic flux, which would induce an electric field in the juice and produce the thermal effect.
E. coli O157:H7 is a major foodborne pathogen causing bloody diarrhea and hemolytic-uremic syndrome, which was usually used as a representative to evaluate the method for food pasteurization. Thus, the objective of this study was to examine the thermal effect on the grapefruit juice and the inactivation of E. coli O157:H7 in juice sample under IEF treatments with different processing parameters (induced voltage, frequency, initial temperature, flow rate or retention time). After that, some physicochemical properties including pH, °Brix and color of the treated juice were also investigated, which aimed to provide scientific foundation for the development of an alternative pasteurization technique.
2. Materials and methods
Bacterium cultivation and cell suspension
According to previous researches,9,17,18 a cocktail of three E. coli O157:H7 strains (ATCC 35150, 35218 and 43890) was used as target pathogens to determine the effectiveness of IEF for inactivating E. coli O157:H7. These strains were purchased from Shanghai Beinuo Biological Technology Co. Ltd. (Shanghai, China) and kept at −80 °C in a 50% glycerol solution. After thawed with tap water, the strains were diluted to spread on the tryptic soy agar (TSA) dishes and kept at 37 °C to incubate E. coli O157:H7 for 24 h. The obtained colony after cultivation was transferred with an inoculation loop to tryptic soy broth (TSB, 150 mL) which was previously sterilized at 121 °C for 15 min. The strain-contained TSB was incubated at 37 °C for 24 h with shaking at 180 rpm and then centrifuged at 4 °C and 4000 rpm for 10 min. Each collected strain was resuspended in 0.2% peptone water to a final concentration of about 109 CFU mL−1.
Sample preparation and strain inoculation
A preservative-free concentrate of grapefruit juice (pH 3.77 at 16 °Brix) was purchased from a local supermarket. Before IEF treatment, an equal amount (8 mL) of each strain suspension was incubated into 1000 mL grapefruit juice to prepare the E. coli-contaminated juice samples with an initial concentration of 107 CFU mL−1. The incubated E. coli O157:H7 was enumerated on TSA at 37 °C for 24 h.
Induction heating system
The system (Fig. 1) was comprised of a power supply (KL-1105, KeLian Electronics Co., Ltd. China), a magnetic core (material: oriented silicon steel; saturation flux density 1.8 T; initial magnetic permeability > 1000; total cross-sectional area: 900 cm2), a peristaltic pump (BT100S; Lead Fluid Co., Ltd. China), an excitation coil (primary coil, NP = 6), a sample coil (secondary coil, NS = 18), and an heating tube (a glass tube; L, length: 300 mm; internal diameter: 2 mm; wall thickness: 3 mm). The power supply produced a sine waveform at frequencies of 300, 600 and 900 Hz with a maximum excitation voltage of 900 V (UP). The induced voltage (US) in the closed-loop of samples can be calculated as following: |
 | (1) |
which in theory were 900, 1800 and 2700 V respectively in this study for the induction heating of grapefruit juice. All treatments were conducted within saturated magnetic flux of the core.
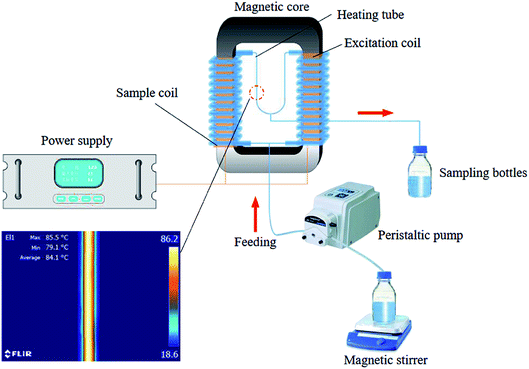 |
| Fig. 1 Schematic diagram of the heating system. | |
Heating tubes and pipelines involved in the microbial experiments were rinsed with sterile Milli-Q water (72 °C, 5 min) for sanitization in advance, followed by cooling down with circumfluence of the same water at room temperature. Then, each juice sample of 2.4 L after inoculation was pumped into the system at different flow rates of 6, 8, 10, 13.3, 15, 17.1, 20, 24, 30, 40, 60, and 120 mL min−1 for this heating. Therefore, retention times for the juice samples to flow through the induction heating system were 20, 40, 60, 80, 100, 120, 140, 160, 180, 240, 300 and 400 s, respectively.
Temperature recording
The temperature recording was conducted according to the method presented in González-Monroy et al. with some modifications.19 Infrared thermography (Model C2, FLIR, Täby, Sweden) was applied to record the temperature history of the heating chamber which was filled with grapefruit juice.
Fluid impedance measurement
The measurement of fluid impedance was performed according to the method presented in Zhang and Yang.20 The 65120B impedance analyzer (Wayne Kerr Electronic. West Sussex, UK) with a 1002J component was used to measure the impedance. The fixture of 1002J component was installed via a 1J1022 acid-proof probe, on which two tubes that functioned as fluids' inlet and outlet ports were connected into the heating system. The probes were adjusted in consistency with the channel's inner diameter. Probe calibration was performed under open circuit, short circuit, and circuit at high frequency (resistance 100 Ω, the capacitance of 10 and 100 pF under the frequency range from 50 Hz to 10 MHz). The impedance of the juice fluid at 400–1000 Hz was measured before the power supply started to work.
Microbiological analysis
In order to perform microbial enumeration, 25 mL of treated samples were transferred immediately into a peptone water-filled (0.2%, 225 mL) sterilized stomacher bag (Labplas, Inc., Sainte-Julie, Quebec, Canada) and then mixed for 2 min with a stomacher (Easy Mix; AES Chem-unex, Rennes, France) to make the pathogens evenly distributed. One mL aliquots were withdrawn from the homogeneous sample and diluted stepwise to ten times with 0.2% peptone water. Each diluent in 0.1 mL amount was plated on tryptic soy agar, incubated at 37 °C for 24–48 h, and finally followed with the colony enumeration.
pH value, °Brix and color examination
The quality analysis was carried out on the grapefruit juice sufficiently pasteurized by the induction heating at various conditions, in which the E. coli O157:H7 was inactivated to below the detectable limit of 1 log CFU mL−1. After treatments, samples were immediately placed in an ice bath for 5 min to cool down. A USPRO colorimeter (UltraScan Pro1166, USA) was employed for color measurement by recording a series of values including L*, a*, b*, and ΔE, which stands for lightness, redness, yellowness, and color difference, respectively, to assess the color difference among samples. Further, the values of °Brix and pH for all the samples were individually detected via a refractometer (Master-M; Atago, Tokyo, Japan) and a PHS-3C digital pH meter, respectively. The untreated grapefruit juice was used as control.
Statistical analysis
Data was expressed as mean value ± standard error. Analysis of variance and mean value were conducted using the SPSS 16.0 (Spss Inc., Chicago, IL) at a significance level of 0.05. Significance difference was determined by Ducan's test.
3. Results
Effect of electrical parameters on temperature rise of juice
The temperature profiles of the grapefruit juice with T0 of 20 °C were recorded. As shown in Fig. 2, the heating rate and terminal temperature were improved with the increase of induced voltage, while declined with the increase of the applied frequency. In current operating conditions of electric voltage and current (the magnetic flux in magnetic core was not saturated), the heating of fruit juice was more obviously affected by electric voltage than current. The induced volage played a more important role than the frequency. The terminal temperatures changed in the range from 73.2 °C to 93.7 °C under different conditions. The fastest heating and highest terminal temperature occurred at induced voltage of 2700 V and frequency of 300 Hz.
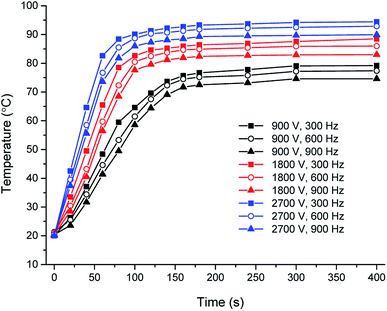 |
| Fig. 2 Effect of induced voltage and frequency on the temperature rise of grapefruit juice (T0 = 20 °C) under IEF treatment. | |
Effect of initial temperature on temperature rise of juice
As shown in Fig. 3, the initial temperature significantly influenced the temperature rise, while slightly impact the terminal temperature of juice that was in the range from 87.1 °C to 94.3 °C at the operation conditions of 2700 V and 300 Hz. It took less time for the grapefruit juice with high initial temperature to reach the heating-up end. This phenomenon could be explained by that the increased temperature weakened the ionic fractional drag force and strengthened the electrical conductivity of the liquid,21 which agreed with the decreased impedance level of the juice at high initial temperature (Table 1). As a result, more rapid magnetoelectric conversion was observed. However, it can be deduced form the slightly-different terminal temperature that the induced current primarily decided the final temperature of the juice.
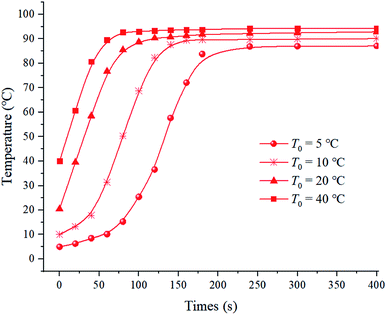 |
| Fig. 3 Effect initial temperature T0 on the temperature rise of grapefruit juice under IEF treatment at 2700 V and 300 Hz. | |
Table 1 Impedance of the grapefruit juice inside probes at different initial temperature and frequencya
Initial temperature (°C) |
Frequency (Hz) |
Impedance (Ω) |
Different lowercase letters indicate the significant differences between various impedance value (p ≤ 0.05). |
5 |
300 |
21.50 ± 1.07a |
600 |
20.46 ± 1.85a |
900 |
19.33 ± 1.35a |
10 |
300 |
15.65 ± 1.03b |
600 |
14.87 ± 1.24b |
900 |
14.03 ± 1.44b |
20 |
300 |
9.62 ± 1.09c |
600 |
8.75 ± 0.81c |
900 |
8.29 ± 1.12c |
40 |
300 |
4.8 ± 0.82d |
600 |
4.21 ± 1.09d |
900 |
3.79 ± 0.74d |
Effect of electrical parameters on inactivation of E. coli O157:H7
The electrical voltage and frequency are two important factors in electrical pasteurization technologies, including high-intensity pulsed electric field treatment and ohmic heating.22,23 With different flow rates, the grapefruit juice flow through the induction heating system and was collected at the outlet for microbiological test. In this case, the results were plotted using time as X-axis by the conversion from flow rate. As shown in Fig. 4, the inactivation of E. coli O157:H7 in grapefruit juice (T0 = 20 °C) was enhanced at high induced voltage regardless of the frequency, which was due to more energy absorption and high-temperature treatment of the juice. The initial concentration of E. coli O157:H7 in juice was determined to be 7.12 log CFU mL−1. More than five-log reduction was achieved following the induction heating treatment at 900 V for 300 s, at 1800 V for 240 s, and at 2700 V for only 180 s, respectively.
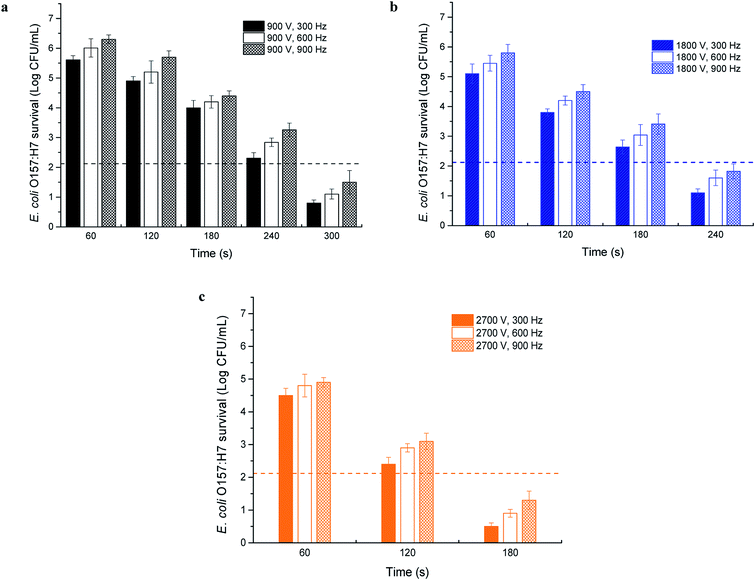 |
| Fig. 4 E. coli O157:H7 inactivation by IEF treatment with different induced voltages ((a) 900 V, (b) 1800 V, (c) 2700 V) and frequencies (T0 = 20 °C). | |
Frequency is related to the change rate of the induced electric field direction. Most bacteria consist of charge-carrying proteins and biological macromolecules, which can give different responses to the frequency of applied electric field. As presented in Fig. 4a–c, less E. coli O157:H7 survived at higher frequency when the induced voltage and the treatment time were fixed. It is indicated that more electricity was absorbed by the juice and transformed into heat, which was in accordance with the temperature profile at various frequencies. This result was similar to previous studies regard the electro electro-inactivation (ohmic heating) of tomato juice and milk products; the lethality for the bacterial pathogens were remarkably reduced by the increase of electrical frequency from 500 Hz to 1000 Hz.9
Effect of initial temperature on inactivation of E. coli O157:H7
The survival of E. coli O157:H7 in the sample with different initial temperatures subjected to the innovative induction heating is shown in Fig. 5. Large population of E. coli O157:H7 was inactivated when the heating treatments started with high initial temperature. For instance, when the initial temperature of grapefruit juice was 40 °C, it was no longer than 120 s to realize 5-log reduction in E. coli O157:H7 numbers after an induced electric field treatment of 2700 V and 300 Hz. In comparison, about 180 s was required for 5-log reduction at 10 °C initial temperature, otherwise nearly 240 s was needed (T0 = 5 °C). As the above analysis of Fig. 3, the high initial temperature also induced an improved terminal temperature and shortened time to reach the heating-up end, which indicated the grapefruit juice underwent more intensive thermal treatment. The strengthened heating intensity thereby increased the inactivation efficiency of pathogenic microorganism. Based upon the bactericidal effect, grapefruit juice obtained at six chosen parameters were further examined to evaluate the effect of IEF treatment on physicochemical properties of the juice.
 |
| Fig. 5 Effect of samples' initial temperature T0 on the inactivation of E. coli O157:H7 by IEF treatment at 2700 V and 300 Hz. | |
pH, °Brix and color of IEF-treated grapefruit juice
Table 2 summarized the pH, °Brix and color of the IEF-treated grapefruit juice at various conditions, in which E. coli O157:H7 population was below the detective limitation of 1 log CFU mL−1. There was no significant change in pH and °Brix between the treated grapefruit juice and the untreated one (P > 0.05). This behavior of pH differed from that of the previous report where ohmic heating resulted in an increasing pH of the pomegranate juice. The authors explained this change was caused by the juice hydrolysis and electrodes corrosion exiting in ohmic heating process.24 In another study, Assiry et al.25 employed HAC–NaCl buffer solution (pH 3.5) as a model of low acid food system and investigated the electrochemical reactions during ohmic heating. As stainless-steel electrodes were employed in heating medium, some undesirable electrochemical reactions took place and resulted in the increase in pH value, leakage of the metal ions as well as formation of brown color. The dependency on electrodes limited the development of ohmic heating in food industry. In this study, an induced electric field was used to heat up the grapefruit juice, where the liquid worked as the secondary coil in a transformer and an inner thermal effect was produced through the electromagnetic induction. The disadvantageous electrochemical reactions could be avoided and then pH of the juice was hardly changed. °Brix, reflecting the soluble solids content in the juice, was usually not altered by thermal treatments, which was similarly observed in ohmic heating treatment of commercial apple juice.26
Table 2 Physicochemical properties of pH, °Brix and color parameters of the grapefruit juice sufficiently pasteurized by different IEF treatmentsa
Treatment conditions |
pH |
°Brix |
L* |
a* |
b* |
ΔE* |
The conducted frequency of the induced electric field is 300 Hz. Different superscript lowercase letters in the same column indicate the significant difference at a level of 0.05. |
Untreated sample |
3.77 ± 0.00a |
11.2 ± 0.00a |
71.22 ± 0.04d |
4.21 ± 0.04c |
33.25 ± 0.03c |
— |
900 V, 300 s, T0 = 20 °C |
3.77 ± 0.00a |
11.2 ± 0.04a |
71.37 ± 0.04c |
4.58 ± 0.11b |
33.50 ± 0.12b |
0.47 ± 0.07d |
900 V, 400 s, T0 = 10 °C |
3.77 ± 0.01a |
11.3 ± 0.09a |
71.51 ± 0.11bc |
4.79 ± 0.08ab |
33.61 ± 0.06b |
0.74 ± 0.12c |
1800 V, 240 s, T0 = 20 °C |
3.78 ± 0.02a |
11.3 ± 0.06a |
71.69 ± 0.06b |
4.83 ± 0.08ab |
33.72 ± 0.11ab |
0.91 ± 0.08b |
1800 V, 180 s, T0 = 40 °C |
3.76 ± 0.02a |
11.4 ± 0.06a |
71.72 ± 0.15ab |
4.88 ± 0.12a |
33.86 ± 0.14a |
1.03 ± 0.18ab |
2700 V, 180 s, T0 = 20 °C |
3.78 ± 0.01a |
11.5 ± 0.05a |
72.14 ± 0.12a |
4.96 ± 0.10a |
33.94 ± 0.04a |
1.37 ± 0.05a |
After the induction heating treatments, all color parameters, L*, a*, and b* slightly shifted more positive, indicating a color of more light, red and yellow. The brightening of juice might be caused by the agglomeration and precipitation of the suspended particles in the juice under this magneto-induced thermal effect because of IEF. Although the changes in each color coordinates were very slight at different processing levels, the total color difference ΔE* of 1.37 obtained at the condition of 2700 V and 180 s was significantly higher than other conditions. It was suggested that the induced voltage could be a critical restriction for heating the grapefruit juice. All ΔE* value changed in the range from 0.47 to 1.37, beyond the lowest noticeable limit of 1.5.27 The quality factors of the grapefruit juice were not severely degraded by this induction heating method. It suggested that this heating method is very potential to realize the electric field pasteurization of fruit juice without the risk of electrochemical reactions that exist in ohmic heating.
4. Discussion
Although thermal processing is wildly used for food pasteurization and pathogens inactivation, the conventional ways of heating would have a negative influence on product quality. This issue promotes the development of alternative heating technology that independent on heat conduction. In current study, the innovative induction heating is defined as a non-conventional electrotechnology because the electric field is produced by an alternating magnetic field and a magnetic core is used as the key component for heating. It can be regarded as a sister technology of ohmic heating, but differently, ohmic heating has been widely reported in the sterilization of liquid food, such as milk, juice and some meat products.12 The rare application of this induction heating could be attributed to that liquid food usually possess high impedance when filled in pipeline; thus the current intensity and density induced in the liquid is too low to generate enough thermal for heating the liquid up. One solution is to improve the induced current density in liquid food, which is realized based on the structure and theory of transformer. Although the induced current density in the grapefruit juice was not able to be detected in this research, its expansion was demonstrated by the remarkable heating effect.
Like previous research on inactivation of foodborne pathogens with electric field techniques such as ohmic heating and pulsed electric field treatment, the effect of operation parameters including electric field strength, frequency, treatment time, pH on the inactivation capacity were also investigated in this study.28,29 These electric field inactivation techniques involve the thermal effect and electric field effect that is also called non-thermal effect.30,31 Ohmic heating mainly take advantage of the thermal effect of electric fields to heat food matrix quickly and thus cause the proteins denaturation and the microbial inactivation, where the electric field effect usually plays an auxiliary role. For pulsed electric field, the temperature of food stuff dose not increase obviously, and the non-thermal effect dominate in the inactivation of microorganisms.
These non-thermal effects in electrotechnologies are called electroporation and electropermeability.32 Under the non-thermal effect of electric field, trans-membranes pores are promoted to form on microbial membranes, causing the leakage of intracellular proteins, DNA, as well as enzymes.33,34 The damage degree is greatly affected by medium temperature and electric field strength, therefore resulting in different reversible or irreversible injury. When the number of pores acculturate to a certain level, the microbial membrane will be destroyed. The critical voltage across the cell is considered to be about 1 V for membrane destruction and microbial sterilization.29
In comparison with the thermal pasteurization method, the application of electric field can decrease the lethal temperature or reduce the operation time. However, the electrode corrosion impedes the application of electric field processing such as ohmic heating due to the potential leakage of metal ions into the food.35 The proposed induced electric field heating inactivation avoid using metal electrodes. Although effective heating and inactivation was observed, further investigations are still required to explore the possible non-thermal effects. Then the biological sublethality, membrane permeability and more nutrients changes will be compared.
5. Conclusions
The E. coli O157:H7 pathogen was effectively inactivated by the application of innovative induction heating in the low-frequency region. The remarkably thermal effect on grapefruit juice was realized based on the magnetoelectric effect and the large current density in the juice. It is different from traditional electro-thermal methods like ohmic heating where the electric field, the current density as well as the thermal effect are generated via the metal electrode inserted in food matrix. But similar to ohmic heating, the heating rate and bactericidal effect of this induction heating were improved with the increase of induced voltage, while it was reduced at the level of frequency, pH and flow rate. The quality of the grapefruit juice could be well preserved after this heating treatment. The alternating magnetic field was used as the stimulus, which is helpful for the avoidance of undesirable electrochemical reaction and electrode corrosion that usually caused by the usage of metal electrodes, when compared to ohmic heating. Low frequency is recommended for the magneto-induced thermal pasteurization of continuous-flow liquid food. Further research concerning other factors such as excitation signal waveform, the diameter of the heating chamber is still needed to be conducted for the enforcement of heating efficiency and bactericidal effect.
Conflicts of interest
There are no conflicts to declare.
Acknowledgements
This study was financially supported by the National Natural Science Foundation of China (No. 31701522), and the Nature Science Foundation of Jiangsu Province (No. BK20170182, BK20180608), the Agricultural Science and Technology Independent Innovation Funds of Jiangsu Province (CX(19)3067, CX(19)3069), and the Opening Foundation of Jiangsu Key Laboratory of Advanced Food Manufacturing Equipment and Technology (No. FM-2019-05, FMZ202004).
References
- A. P. Singh, P. P. L. Yen, H. S. Ramaswamy and A. Singh, Curr. Opin. Food Sci., 2018, 23, 90–96 CrossRef.
- F. J. Barba, L. R. B. Mariutti, N. Bragagnolo, A. Z. Mercadante, V. G. Barbosa-Cánovas and V. Orlien, Trends Food Sci. Technol., 2017, 67, 195–206 CrossRef CAS.
- D. B. Schlisselberg, E. Kler, E. Kalily, G. Kisluk, O. Karniel and S. Yaron, Int. J. Food Microbiol., 2013, 160, 219–226 CrossRef PubMed.
- F. Marra, L. Zhang and J. G. Lyng, J. Food Eng., 2009, 91, 497–508 CrossRef.
- W. Song and D. Kang, Food Microbiol., 2016, 60, 104–111 CrossRef CAS PubMed.
- J. C. Atuonwu and S. A. Tassou, J. Food Eng., 2018, 234, 1–15 CrossRef.
- X. Tian, Q. Yu, W. Wu, X. Li and R. Dai, Int. J. Food Microbiol., 2018, 285, 42–49 CrossRef CAS PubMed.
- S. Ghnimi and L. Fillaudeau, in Ohmic Heating in Food Processing, ed. H. S. Ramaswamy, M. Marcotte, S. Sastry and K. Abdelrahim, CRC Press, Boca Raton, 2014, pp. 183–196 Search PubMed.
- S. Kim, W. Choi and D. Kang, Food Microbiol., 2017, 63, 22–27 CrossRef CAS PubMed.
- M. C. Knirsch, C. A. d. Santo, A. A. M. d. O. S. Vicent and T. C. V. Penna, Trends Food Sci. Technol., 2010, 21, 436–441 CrossRef CAS.
- S. Y. Lee, H. G. Sagong, S. Ryu and D. H. Kang, J. Appl. Microbiol., 2012, 112, 723–731 CrossRef CAS PubMed.
- H. Jaeger, A. Roth, S. Toepfl, T. Holzhauser, K. H. Engel, D. Knorr, R. F. Vogel, N. Bandick, S. Kulling, V. Heinz and P. Steinberg, Trends Food Sci. Technol., 2016, 55, 84–97 CrossRef CAS.
- J. Morren, B. Roodenburg and H. Swhd, Innovative Food Sci. Emerging Technol., 2003, 4, 285–295 CrossRef CAS.
- G. Razo-Aguilera, R. Baez-Reyes, I. álvarez-González, R. Paniagua-Pérez and E. Madrigal-Bujaidar, Food Chem. Toxicol., 2011, 49, 2947–2953 CrossRef CAS PubMed.
- R. M. Aadil, X. Zeng, D. Sun, M. Wang and Z. Zhang, LWT--Food Sci. Technol., 2015, 62, 890–893 CrossRef CAS.
- S. K. Sastry and J. T. Barach, J. Food Saf., 2015, 65, 42–46 CrossRef.
- A. Rezaeimotlagh, K. S. C. Tang, M. Resch, P. J. Cullen and F. J. Trujillo, Food Res. Int., 2018, 106, 780–790 CrossRef CAS PubMed.
- J. B. Gurtler, R. B. Bailey, D. J. Geveke and H. Zhang, Food Control, 2011, 22, 1689–1694 CrossRef CAS.
- A. D. González-Monroy, G. Rodríguez-Hernández, C. Ozuna and M. F. Sosa-Morales, Innovative Food Sci. Emerging Technol., 2018, 49, 51–57 CrossRef.
- M. Zhang, N. Yang, L. Guo, D. Li, S. Wu, F. Wu, Z. Jin and X. Xu, J. Food Eng., 2018, 234, 108–116 CrossRef CAS.
- K. Gomathy, K. Thangavel, M. Balakrishnan and R. Kasthuri, J. Food Process Eng., 2015, 38, 405–413 CrossRef CAS.
- S. Monfort, E. Gayán, J. Raso, S. Condón and I. Álvarez, Food Microbiol., 2010, 27, 845–852 CrossRef CAS PubMed.
- S. S. Kim and D. H. Kang, J. Food Prot., 2015, 78, 1208–1214 CrossRef CAS PubMed.
- H. Darvishi, M. H. Khostaghaza and G. Najafi, J. Saudi Soc. Agric. Sci., 2013, 12, 101–108 Search PubMed.
- A. Assiry, S. K. Sastry and C. Samaranayake, J. Appl. Electrochem., 2003, 33, 187–196 CrossRef CAS.
- N. H. Kim, J. H. Ryang, B. S. Lee, C. T. Kim and M. S. Rhee, Int. J. Food Microbiol., 2017, 246, 80–84 CrossRef CAS PubMed.
- Z. Cserhalmi, M. Sass-Kiss, M. Tóth-Markus and N. Lechner, Innovative Food Sci. Emerging Technol., 2006, 7, 49–54 CrossRef CAS.
- M. Sato, N. M. Ishida, A. T. Sugiarto, T. Ohshima and H. Taniguchi, IEEE Trans. Ind. Appl., 2001, 37, 1646–1650 CrossRef.
- A. H. Baysal and F. Icier, J. Food Prot., 2010, 73, 299–304 CrossRef PubMed.
- T. Ohshima and M. Sato, Adv. Biochem. Eng./Biotechnol., 2004, 90, 113–133 CrossRef CAS.
- I. K. Park and D. H. Kang, Appl. Environ. Microbiol., 2013, 79, 7122–7129 CrossRef CAS PubMed.
- T. Xie, L. Sun and T. Tsong, Biophys. J., 1990, 58, 13–19 CrossRef CAS PubMed.
- R. Pereira, J. Martins, C. Mateus, J. A. Teixeira and A. A. Vicente, Chem. Pap., 2007, 61, 121–126 CAS.
- S. Shiina, T. Ohshima and M. Sato, Biotechnol. Prog., 2004, 20, 1528–1533 CrossRef CAS PubMed.
- G. Patara, G. M. J. Barca, R. N. Pereira, A. A. Vicente, J. A. Teixeira and G. Ferrari, Innovative Food Sci. Emerging Technol., 2014, 21, 66–73 CrossRef.
|
This journal is © The Royal Society of Chemistry 2020 |
Click here to see how this site uses Cookies. View our privacy policy here.