DOI:
10.1039/D0RA03478A
(Review Article)
RSC Adv., 2020,
10, 21602-21614
Advances in PEG-based ABC terpolymers and their applications
Received
18th April 2020
, Accepted 18th May 2020
First published on 5th June 2020
Abstract
ABC terpolymers are a class of very important polymers because of their expansive molecular topologies and extensive architectures. As block A, poly(ethylene glycol) (PEG) is one of the most principal categories owing to good biocompatibility and wide commercial availability. More importantly, the synthetic approaches of ABC terpolymers using PEG as a macroinitiator are facile and varied. PEG-based ABC terpolymers from design and synthesis to applications are highlighted in this review. Linear, 3-miktoarm, and cyclic polymers as the architecture are separated. The synthetic approaches of PEG-based ABC terpolymers mainly include the sequential polymerization or coupling of polymers. PEG-based ABC terpolymers have wide applications in the fields of drug carriers, gene vectors, templates for the fabrication of inorganic hollow nanospheres, and stabilizers of metal nanoparticles.
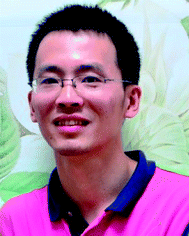 Xiaojin Zhang | Dr Xiaojin Zhang is currently a professor at China University of Geosciences (Wuhan). He received his BSc degree (2007) and PhD degree (2012) from Wuhan University (Renxi Zhuo's group). He then worked as a postdoctoral fellow in Prof. Peter X. Ma's group at the University of Michigan, Ann Arbor. He joined China University of Geosciences (Wuhan) in 2016. His scientific interest is focused on biomedical polymers. |
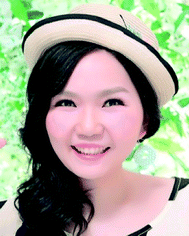 Yu Dai | Dr Yu Dai is currently an Associate Professor at China University of Geosciences (Wuhan). She received her BSc degree (2007) and PhD degree (2012) from Wuhan University (Shengli Chen's group). She then joined China University of Geosciences (Wuhan) in 2012. She worked as a postdoctoral fellow in Prof. Kai Sun's group at the University of Michigan, Ann Arbor from June 2013 to August 2014. Her scientific interest is focused on chemical and biosensor field. |
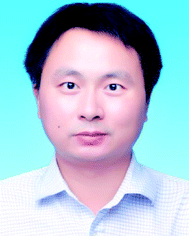 Guofei Dai | Dr Guofei Dai is currently a senior engineer at Jiangxi Institute of Water Sciences. He received his BSc degree (2007) from Wuhan University (Renxi Zhuo's group) and PhD degree (2012) from Institute of Hydrobiology, Chinese Academy of Sciences. He joined Jiangxi Institute of Water Sciences in 2013. His scientific interest is focused on quaternary ammonium polymer for environmental use. |
1. Introduction
Poly(ethylene glycol) (PEG) is a commercial product with good water solubility. It is widely used in many fields such as medicine, health, food, and the chemical industry.1 It can be quickly eliminated by the body without significant toxicity and side effects. Therefore, its application in medicine has received extensive attention and has been recognized by the Food and Drug Administration of the United States (FDA).2 According to the structure, PEG can be divided into monofunctional (e.g. mPEG-OH), homobifunctional (e.g. HO-PEG-OH), heterobifunctional (e.g. HO-PEG-NH2), and multi-arm (e.g. 4-armPEG. 8-armPEG). In practical applications, the functional end groups of PEG are not limited to hydroxyl, but others with stronger reactivity, such as p-toluenesulfonate, amino, carboxyl, aldehyde, thiol, NHS ester, azido, acrylate, acrylamide and epoxide, can also be incorporated into PEG to enlarge the application prospects in macromolecule synthesis, in particular, PEG-based copolymers.3
PEG is usually a hydrophilic segment in PEG-based copolymers. Taking AB diblock copolymers as an example, hydrophilic polymers or hydrophobic polymers are incorporated into PEG to yield double-hydrophilic block copolymers or amphiphilic block copolymers, respectively. By temperature and pH changes or in the presence of a substrate, amphiphilicity is just induced, thus double-hydrophilic block copolymers become amphiphilic block copolymers.4 Amphiphilic block copolymers self-assemble to form various nanoparticulate morphologies (e.g. micelles, polymersomes, rods) in aqueous solution.5 These nanoparticles have potential applications for drug carriers, mesoporous materials, and photoelectric materials, thus have received considerable attention.6 Compared to AB diblock copolymers, ABC terpolymers have a dramatically increased number of unique sequences capable of producing extensive nanoparticles.7 According to the selectivity of solvents toward different blocks, ABC terpolymers can form a series of nanoparticles with different morphologies, such as Janus and multicompartment architectures.8 The morphologies are influenced by many factors such as block length,9 block sequence,10 polymer concentration,11 temperature,12 pH,13 and external light.14 By controlling these factors, various morphologies (e.g. raspberry-like,15 virus-like,16 cloud-like17) can be prepared to meet different needs.
ABC terpolymers are typically synthesized by the sequential polymerization or coupling of polymers.18 In the sequential approach, the monomers one after another are polymerized through various polymerization techniques such as reversible addition-fragmentation chain transfer (RAFT) polymerization,19 atom transfer radical polymerization (ATRP),20 anionic polymerization (AP),21 anionic ring-opening polymerization (AROP),22 cationic ring-opening polymerization (CROP),23 or a combination of several polymerization techniques.24 For example, Pispas et al. reported the synthesis of poly(2-(dimethylamino)ethyl methacrylate)-b-poly(lauryl methacrylate)-b-poly(oligo(ethylene glycol) monomethyl ether methacrylate) (PDMAEMA-b-PLMA-b-POEGMA) by the sequential RAFT polymerization (Scheme 1a).25 In the second approach, AB diblock copolymers (or A homopolymer and B homopolymer) and C homopolymer are covalently bound together to achieve the desired ABC terpolymers.26 For instance, Wei et al. prepared polyfluorene-b-poly(ε-caprolactone)-b-poly(oligo(ethylene glycol) monomethyl ether methacrylate) (PF-b-PCL-b-POEGMA) by Suzuki reaction, ring-opening polymerization (ROP), ATRP, and click coupling (Scheme 1b).27 The synthesis of ABC terpolymers is facile if using commercially available polymers (e.g. PEG, polystyrene, polyethylene) as a macroinitiator.28 Among them, PEG is the most frequent choice due to its advantageous properties and wide commercial availability. In particular, PEG with a functional end group is usually used as a macroinitiator to prepare ABC terpolymers, for example, hydroxyl or amine for ROP, a chain transfer agent (CTA) for RAFT polymerization, 2-bromoisobutyrate for ATRP. It should be noted that poly(ethylene oxide) (PEO) prepared by AROP of ethylene oxide is also widely used to synthesize ABC terpolymers.29 In this review, the developments in the design, synthesis, and applications of PEG-based ABC terpolymers are summarized.
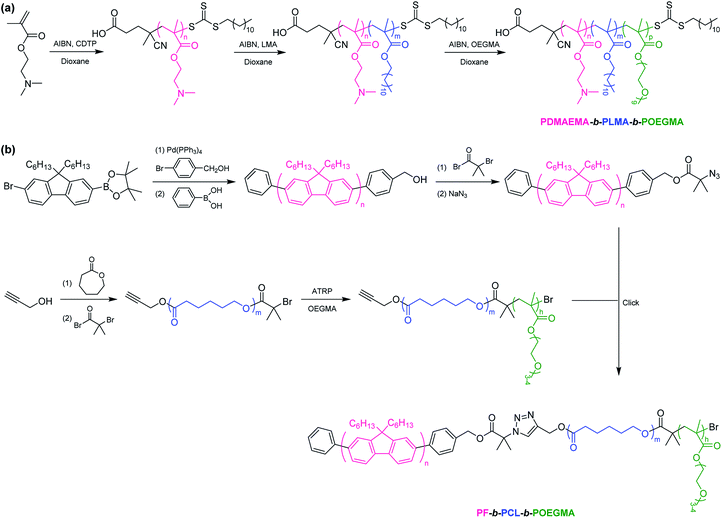 |
| Scheme 1 Synthesis of ABC terpolymers via (a) the sequential RAFT polymerization, (b) Suzuki reaction, ROP, ATRP, and click coupling. | |
2. Design and synthesis
As for the architecture, PEG-based ABC terpolymers may be divided into three major groups: linear, 3-miktoarm, and cyclic (Fig. 1). ABC cyclic terpolymers have rarely been reported. As a case, ABC cyclic terpolymer consisting of poly(isoprene), polystyrene, and poly(2-vinylpyridine), was synthesized via a combination of anionic polymerization and Glaser coupling.30 An α-t-butyldimethylsilyloxy-ω-hydroxy terpolymer, t-butyldimethylsilyloxy-PI1,4-b-PS-b-P2VP-OH was first synthesized by the sequential anionic copolymerization of isoprene (Is), styrene (St), and 2-vinylpyridine (2-VP) with 3-(t-butyldimethylsilyloxy)-1-propyl-lithium as an initiator, followed by end-capping with ethylene oxide. t-Butyldimethylsilyl was deprotected and then hydroxyl was esterified to incorporate alkyne onto both chain ends. Finally, intramolecular ring closure was carried out by Glaser coupling to obtain ABC cyclic terpolymers. As a result, the emphasis of this review will be placed on PEG-based ABC linear and 3-miktoarm terpolymers.
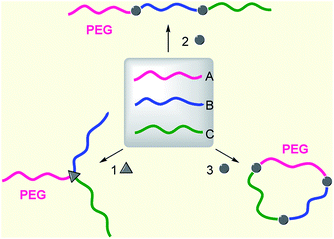 |
| Fig. 1 Topology of PEG-based ABC (linear, 3-miktoarm, cyclic) terpolymers. | |
2.1. Synthesis of PEG-based ABC linear terpolymers
A great deal of PEG-based ABC linear terpolymers are commercially available (e.g. purchased from Polymer Source Inc.). Particularly, polystyrene as block C in ABC linear terpolymers is the major choice in the products. As a result, commercially available PEG-based ABC linear terpolymers may be divided into two major catalogues: PEG as block B and PEG as block A. The corresponding polymers have poly(2,2,3,3,4,4,4-heptafluorobutyl methacrylate)-b-poly(ethylene glycol)-b-polystyrene and poly(ethylene glycol)-b-poly(2-vinyl pyridine)-b-polystyrene (PEG-b-P2VP-b-PS). PEG-b-P2VP-b-PS is the most frequently used PEG-based ABC linear terpolymers for applications in drug carriers,31 the stabilizer of metal nanoparticles,32 and the synthesis of inorganic hollow nanospheres.33 Besides, a large number of PEG-based ABC linear terpolymers have been designed and synthesized through the synthetic approaches (Fig. 2).
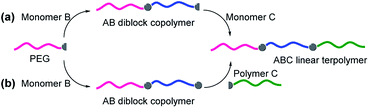 |
| Fig. 2 Synthetic route for the preparation of PEG-based ABC linear terpolymers via (a) the sequential polymerization, (b) polymerization followed by coupling. | |
2.1.1. The sequential polymerization. The sequential approach presenting various polymerization techniques such RAFT polymerization,34–42 ATRP,20,43–45 AROP,22,46 and CROP47–55 has been developed to synthesize PEG-based ABC linear terpolymers. For example, Meier et al. synthesized poly(ethylene glycol)-b-poly(diisopropylaminoethyl methacrylate)-b-poly(styrene sulfonate) (PEG-b-PDPA-b-PSS) by the sequential RAFT polymerization (Scheme 2a).40 Steglich-esterification transformation of PEG-OH was performed to allow a PEG-RAFT macro-CTA that initiated the sequential RAFT polymerization of DPA monomer and SS monomer to afford PEG-b-PDPA-b-PSS. Zhao et al. reported the synthesis of poly(ethylene glycol)-b-poly(2-hydroxyethyl methacrylate)-b-poly(tert-butyl acrylate) (PEG-b-PHEMA-b-PtBA) through the sequential ATRP (Scheme 2b).20 Hydroxyl of PEG-OH was converted into 2-bromoisobutyrate that initiated the sequential ATRP of HEMA monomer and tBA monomer to obtain PEG-b-PHEMA-b-PtBA. Schubert et al. synthesized poly(ethylene glycol)-b-poly(allyl glycidyl ether)-b-poly(tert-butyl glycidyl ether) (PEG-b-PAGE-b-PtBGE) using the sequential AROP (Scheme 2c).22 Hydroxyl of PEG-OH was activated using sodium hydride and the sequential addition of AGE monomer and tBGE monomer generated PEG-b-PAGE-b-PtBGE. Meier et al. reported the synthesis of poly(ethylene oxide)-b-poly(ε-caprolactone)-b-poly(2-methyl-2-oxazoline) (PEO-b-PCL-b-PMOXA) by the sequential CROP (Scheme 2d).52 PEG-OH was chosen as a macroinitiator for the sequential CROP of CL monomer and MOXA monomer to yield PEO-b-PCL-b-PMOXA. A combination of different polymerization techniques grants an accessed pool of monomers and functionalities.56–60 Liu et al. synthesized poly(ethylene glycol)-b-poly(ε-caprolactone)-b-poly(2-(dimethylamino)ethyl methacrylate) (PEG-b-PCL-b-PDMAEMA) via a combination of CROP and ATRP (Scheme 2e).60 After CROP of CL monomer with PEG-OH as a macroinitiator, hydroxyl of PEG-b-PCL was converted into 2-bromoisobutyrate that initiated ATRP of DMAEMA monomer to obtain PEG-b-PCL-b-PDMAEMA. As expected, PEG-b-PCL-b-PDMAEMA could self-assemble into micelles with a pH-responsive characteristic due to pH-responsive PDMAEMA block.
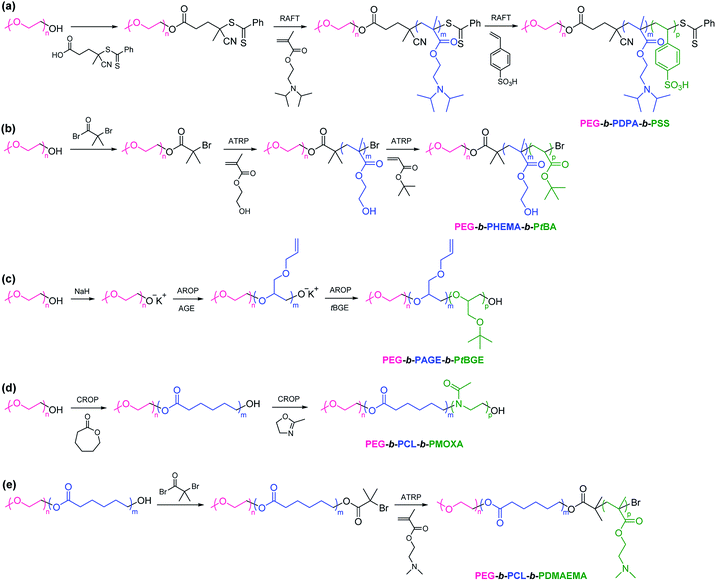 |
| Scheme 2 Synthesis of PEG-based ABC linear terpolymers via the sequential (a) RAFT polymerization, (b) ATRP, (c) AROP, (d) CROP, and (e) a combination of CROP and ATRP. | |
2.1.2. Polymerization followed by coupling. PEG-based ABC linear terpolymers can be engineered through polymerization of monomer B with PEG as an initiator, followed by coupling with polymer C. Such approach requires a functional end group from post/pre polymerization modification.61–64 For example, Liu et al. synthesized poly(ethylene glycol)-b-poly(N-isopropylacrylamide)-b-poly(ε-caprolactone) (PEG-b-PNIPAM-b-PCL) by a combination of ATRP and click coupling (Scheme 3a).26 ATRP of NIPAM monomer was initiated by PEG-Br and then the substitution reaction using sodium azide generated azido-terminated PEG-b-PNIPAM diblock copolymer. Alkynyl-terminated PCL was clicked to azido-terminated PEG-b-PNIPAM by click chemistry to obtain PEG-b-PNIPAM-b-PCL. By introducing the thermoresponsive PNIPAM block, the size of the structure of the resultant micelles changed with temperature increasing. Gu et al. reported poly(ethylene glycol)-b-poly(L-histidine)-b-poly(L-lactide) (PEG-b-PH-b-PLLA) via a combination of CROP and condensation reaction (Scheme 3b).65 CROP of Nim-DNP-L-histidine carboxyanhydride was performed with PEG-NH2 as a macroinitiator to synthesize amino-terminated PEG-b-PH diblock copolymer. Hydroxyl-terminated PLLA was coupled with amino-terminated PEG-b-PH in the presence of succine anhydride to prepare PEG-b-PH-b-PLLA.
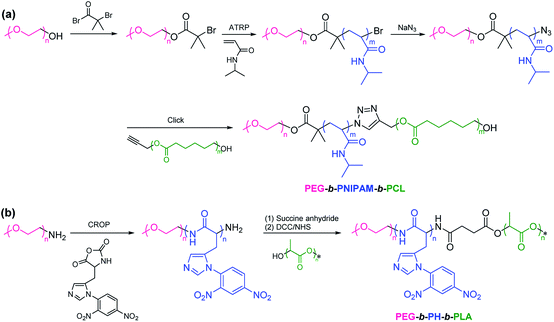 |
| Scheme 3 Synthesis of PEG-based ABC linear terpolymers via a combination of (a) ATRP and click coupling, (b) CROP and condensation reaction. | |
2.2. Synthesis of PEG-based ABC 3-miktoarm terpolymers
PEG-based ABC 3-miktoarm terpolymers can be synthesized by the sequential polymerization or coupling of polymers. The synthetic approaches may be divided detailedly into four major routes (Fig. 3).
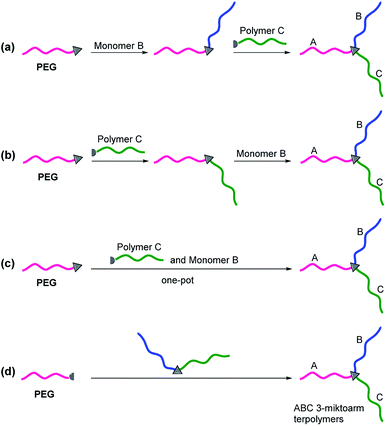 |
| Fig. 3 Synthetic route for the preparation of PEG-based ABC 3-miktoarm terpolymers via (a) polymerization followed by coupling, (b) coupling followed by polymerization, (c) one-pot polymerization and coupling, and (d) coupling. | |
2.2.1. Polymerization followed by coupling. A popular approach for the synthesis of PEG-based ABC 3-miktoarm terpolymers is polymerization of monomer B with difunctional end group-modified PEG as an initiator, followed by coupling of polymer C. For example, Ma et al. developed poly(ethylene glycol)-arm-poly(2-nitrobenzyl methacrylate)-arm-poly(N-isopropylacrylamide) (PEG-arm-PNBM-arm-PNIPAM) via a combination of ATRP and click coupling (Scheme 4a).66 PEG-OH was modified using epoxide that was subsequently ring-opened to provide difunctional end group-modified PEG with both hydroxyl and azido groups. Hydroxyl was converted into 2-bromoisobutyrate that initiated ATRP of NBM monomer. Finally, azido reacted with alkynyl-terminated PNIPAM via click chemistry to produce PEG-arm-PNBM-arm-PNIPAM. The connector is 1-azido-3-hydroxypropan-2-yl 2-bromoisobutyrate with trifunctional groups (hydroxyl, 2-bromoisobutyrate, and azido). Similarly, other PEG-based ABC 3-miktoarm terpolymers such as poly(ethylene glycol)-arm-poly(ε-caprolactone)-arm-poly(benzyl-L-aspartate) (PEG-arm-PCL-arm-PBLA) (the connector is 3-azido-1,2-propanediol),67 poly(ethylene glycol)-arm-poly(2-vinylpyridine)-arm-poly(ε-caprolactone) (PEG-arm-P2VP-arm-PCL) (the connector is glycerol),68,69 poly(ethylene glycol)-arm-poly(2-(dimethylamino)ethyl methacrylate)-arm-polytetrahydrofuran (PEG-arm-PDMAEMA-arm-PTHF) (the connector is 1-azido-3-hydroxypropan-2-yl 2-chloropropionate),70 poly(ethylene glycol)-arm-poly(1H,1H,5H-octafluoropentyl methacrylate)-arm-poly(2-ethylhexyl methacrylate) (PEG-arm-POFPMA-arm-PEHMA) (the connector is 3-azido-1,2-propanediol),71 poly(ethylene glycol)-arm-poly(1H,1H,5H-octafluoropentyl methacrylate)-arm-polystyrene (PEG-arm-POFPMA-arm-PS) (the connector is 3-azido-1,2-propanediol),72 have been synthesized through this approach.
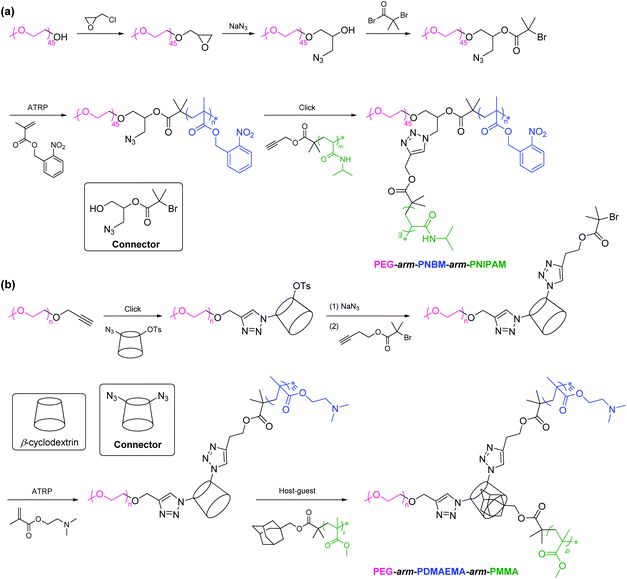 |
| Scheme 4 Synthesis of PEG-based ABC 3-miktoarm terpolymers via a combination of (a) ATRP and click coupling, (b) ATRP and molecular recognition. | |
In the conventional methodology, a multistep reaction and the purification of intermediate polymers are often required. To overcome these problems, it is quite necessary to decrease the functional number of the connector and replace one of the covalent connections by non-covalent recognition. The resulting polymers are also called as supramolecular ABC 3-miktoarm terpolymers. For instance, Zhu et al. designed and synthesized poly(ethylene glycol)-arm-poly(2-(dimethylamino)ethyl methacrylate)-arm-poly(methyl methacrylate) (PEG-arm-PDMAEMA-arm-PMMA) via a combination of ATRP and molecular recognition (Scheme 4b).73 Alkynyl-terminated PEG reacted with mono-6-deoxy-6-(p-tolylsulfonyl)-β-cylcodextrin-azide via click chemistry. Then, p-tolylsulfonyl was converted into azido that was linked with 2-bromoisobutyrate via click chemistry. The as-prepared macromolecular initiator was employed for ATRP of DMAEMA monomer. Molecular recognition between β-cylcodextrin and adamantane (adamantane-terminated PMMA) resulted in the formation of PEG-arm-PDMAEMA-arm-PMMA. The connector is diazido cyclodextrin with difunctional groups (azido) and a hydrophobic cavity capable of incorporating guest molecules selectively to form the inclusion complexes. Apart from host–guest interaction, Hamilton wedge/α-cyanuric acid complementary recognition (H-bonding) is an accessed method to construct supramolecular ABC 3-miktoarm terpolymers.74
2.2.2. Coupling followed by polymerization. An alternative approach for the synthesis of PEG-based ABC 3-miktoarm terpolymers is coupling of polymer B with difunctional end group-modified PEG, followed by polymerization of monomer C. For example, Lin et al. synthesized poly(ethylene glycol)-arm-polystyrene-arm-poly[6-(4-methoxy-azobenzene-4′-oxy) hexyl methacrylate] (PEG-arm-PS-arm-PMMAZO) by a combination of click coupling and ATRP (Scheme 5).75 Epoxide-capped PEG was ring-opened for the preparation of difunctional end group-modified PEG bearing azido and hydroxyl. PS was coupled to PEG by click chemistry. Hydroxyl of the resulting polymer was converted into 2-bromoisobutyrate that further initiated ATRP of MMAZO monomer to afford PEG-arm-PS-arm-PMMAZO. The azobenzene chromophores of PEG-arm-PS-arm-PMMAZO located in different aggregation states had a significant effect on the photo-induced isomerization behaviors. The connector is 1-azido-3-hydroxypropan-2-yl 2-bromoisobutyrate. Similarly, other PEG-based ABC 3-miktoarm terpolymers such as poly(ethylene glycol)-arm-poly(ε-caprolactone)-arm-poly(benzyl-L-aspartate) (PEG-arm-PCL-arm-PBLA) (the connector is 3-azido-1,2-propanediol),67 poly(ethylene glycol)-arm-poly(ε-caprolactone)-arm-polyphosphoester (PEG-arm-PCL-arm-PPE) (the connector is 3-azido-1,2-propanediol),76 poly(ethylene glycol)-arm-polystyrene-arm-poly[(3-triisopropyloxysilyl)propyl methacrylate] (PEG-arm-PS-arm-PIPSMA) (the connector is 1-azido-3-hydroxypropan-2-yl 2-bromoisobutyrate),77 poly(ethylene glycol)-arm-polystyrene-arm-poly(2-(N,N-diethylamino)ethyl methacrylate) (PEG-arm-PS-arm-PDEA) (the connector is 1-azido-3-hydroxypropan-2-yl 2-bromoisobutyrate),78 have been synthesized through this approach.
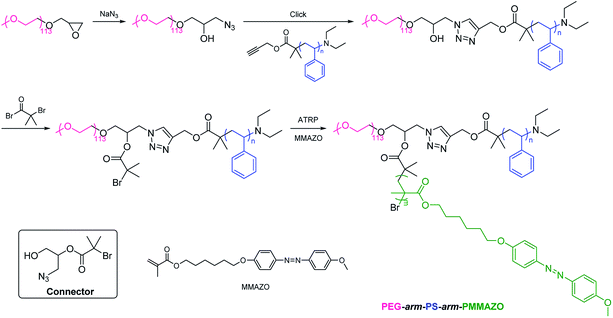 |
| Scheme 5 Synthesis of PEG-based ABC 3-miktoarm terpolymers via coupling followed by polymerization. | |
2.2.3. One-pot polymerization and coupling. A facile approach for the synthesis of PEG-based ABC 3-miktoarm terpolymers is one-pot polymerization of monomer B and coupling of polymer C with difunctional end group-modified PEG. As a case, Ghaemy et al. reported one-pot synthesis of poly(ethylene glycol)-arm-poly(tert-butylacrylate)-arm-poly(ε-caprolactone) (PEG-arm-PtBA-arm-PCL) by a combination of click coupling and single electron transfer living radical polymerization (SET-LRP) (Scheme 6).79 As mentioned above, difunctional end group-modified PEG with azido and 2-bromoisobutyrate groups was synthesized by epoxide-capping, ring-opening, and the nucleophilic reaction. One-pot simultaneous SET-LRP of tBA monomer and click coupling of alkynyl-terminated PCL in the presence of difunctional end group-modified PEG to obtain PEG-arm-PtBA-arm-PCL. The connector is 1-azido-3-hydroxypropan-2-yl 2-bromoisobutyrate. Another example is the preparation of poly(ethylene glycol)-arm-poly(ε-caprolactone)-arm-polystyrene (PEG-arm-PCL-arm-PS).80 The connector is anthracen-9-ylmethyl 2-((2-bromo-2-methylpropanoyloxy)-methyl)-2-methyl-3-oxo-3-(prop-2-ynyloxy)propyl succinate. Furan-protected maleimide-terminated PEG, tetramethylpiperidine-1-oxyl-terminated PCL, and azide-terminated PS were conjugated to the connector through triple click reactions such as Diels–Alder, copper-catalyzed azide–alkyne cycloaddition, and nitroxide radical coupling.
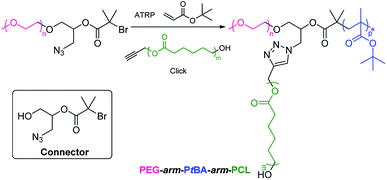 |
| Scheme 6 Synthesis of PEG-based ABC 3-miktoarm terpolymers via one-pot polymerization and coupling. | |
2.2.4. Coupling. An interesting approach for the synthesis of PEG-based ABC 3-miktoarm terpolymers is coupling of monofunctional group-modified PEG with diblock copolymer bearing a functional group at the junction site between blocks. As an example, Yagci et al. developed the synthesis of poly(ethylene glycol)-arm-polystyrene-arm-poly(ε-caprolactone) (PEG-arm-PS-arm-PCL) via click coupling of alkynyl-terminated PEG and PS-b-PCL diblock copolymer with azido (Scheme 7).81 Alkynyl-terminated PEG was synthesized through the esterification of PEG-OH and 5-pentynoic acid. Thiol-terminated PS was synthesized via ATRP of St monomer, xanthate functionalization, and 1,2-ethandithiol reduction. PS was conjugated to 1-(allyloxy)-3-azidopropan-2-ol by thiol–ene click chemistry. Subsequently, ROP of ε-caprolactone monomer was performed through hydroxyl of difunctional end group-modified PS to form PS-b-PCL diblock copolymer with azido. Finally, alkynyl-terminated PEG was clicked onto PS-b-PCL to give PEG-arm-PS-arm-PCL. The connector is 3-azido-1,2-propanediol. Similarly, poly(ethylene glycol)-arm-polystyrene-arm-poly(lactic acid) (PEG-arm-PS-arm-PLA) have been synthesized through this approach.82 They also synthesized PEG-arm-PS-arm-PCL by triple click reactions such as thiol–ene, copper catalyzed azide–alkyne cycloaddition, and Diels–Alder reaction.83 The core is 1-(allyloxy)-3-azidopropan-2-yl (anthracen-9-ylmethyl) succinate with allyl, azide, and anthracene functionalities. Thiol-terminated PS, alkynyl-terminated PCL, and maleimide-terminated PEG were sequentially conjugated to allyl, azide, and anthracene of the core, respectively.
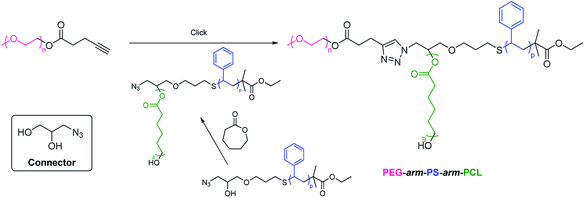 |
| Scheme 7 Synthesis of PEG-based ABC 3-miktoarm terpolymers via coupling. | |
3. Applications
If taking hydrophobic polymer as one or more blocks, ABC terpolymers will self-assemble with subdivided hydrophobic cores, resulting in different morphologies with respect to the spatial restrictions imparted by the chain architecture (Fig. 4).84 Interestingly, the morphologies also depend on the preparation method.85 This subject has been paid extensive attention in part because of their sequestration properties.
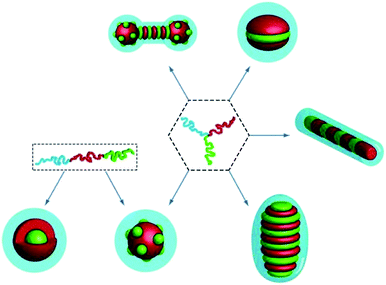 |
| Fig. 4 Illustration depicting different morphologies produced by self-assembly of ABC linear or 3-miktoarm terpolymers. Reprinted with permission.84 Copyright (2012) American Chemical Society. | |
As a case, Le et al. developed a series of ABC terpolymers composed of poly(ethylene glycol) (PEG, block A), poly(α-carboxylate-ε-carprolactone) (PCCL, block B), and poly(ε-caprolactone) (PCL, block C) with either block BC or block CB. Carboxyl on block B was further modified with mercaptohexylamine. The proposed structures of two different types of micelles in terms of block sequence are illustrated in Fig. 5.86 The results showed that ABC terpolymers with PCCL block at the middle display superior micelle stability. Particularly, an alternating triple lamellar morphology from self-assembly of ABC terpolymer composed of poly(ethylene glycol) (PEG, block A), poly(ε-caprolactone) (PCL, block B), and poly(L-lactide) (PLLA, block C) was reported.87
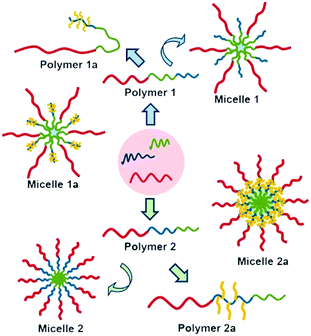 |
| Fig. 5 Proposed structures and post-functionalization of micelles produced by self-assembly of ABC linear terpolymers. Reprinted with permission.86 Copyright (2016) American Chemical Society. | |
3.1. Drug carriers
AB diblock copolymers are often used as drug carriers.88 Introduction of a third block C dramatically expands the efficiency, addressability, or applicability. For instance, we developed pH-responsive polymeric micelles mediated via hydrogen-bonding interaction between phenol of 4,4′-(1,2-diphenylethene-1,2-diyl)diphenol (TPE-2OH) and amino of poly(ethylene glycol)-b-linear polyethylenimine-b-poly(ε-caprolactone) (PEG-b-PEI-b-PCL) (Fig. 6).89 TPE-2OH, an aggregation-induced emission (AIE) characteristic luminogen, was mainly distributed in the interlayer between the core and the shell of polymeric micelles. If pH decreased to 6.5 or lower, amino of PEG-b-PEI-b-PCL was protonated, resulting in the disassociation of hydrogen-bonding. Therefore, tunable aggregation-induced emission and controllable drug release were conducive to cell imaging and cancer therapy. We also described polymeric micelles stabilized polyethylenimine–copper (C2H5N–Cu) coordination between amino of PEG-b-PEI-b-PCL and divalent copper cation.90 The coordination improved drug loading capacity and enabled sustainable drug release. Cargo-carrier interactions significantly contributed to the properties of encapsulated drugs and their localization within the micelles.91
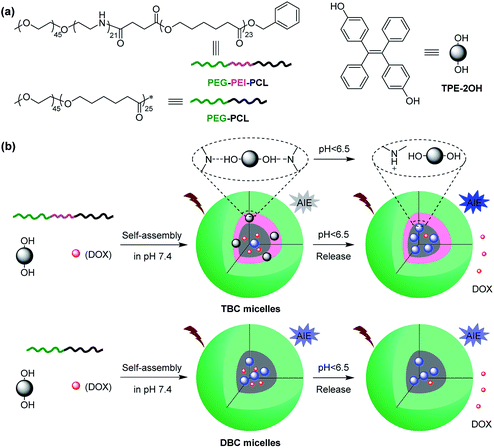 |
| Fig. 6 pH-responsive polymeric micelles with tunable aggregation-induced emission and controllable drug release prepared from ABC linear terpolymers. Reprinted with permission.89 Copyright (2019) Springer. | |
3.2. Gene vectors
Using cationic polymers as a block in ABC terpolymers has the potential for the DNA/RNA complexation, representing a multifunctional gene vector.92 For example, Zhang et al. synthesized poly(ethylene glycol)-b-poly(D,L-lactide)-b-polyarginine (PEG-b-PLA-b-R15) and fabricated cationic polymeric micelles that were capable of binding siRNA to form micelle/siRNA complexes (micelleplexes) (Fig. 7a).93 The micelleplexes could efficiently carry siRNA into cancer cells through endocytosis. The endosomal escape and the biodegradation of ABC terpolymers led to siRNA release. siRNA induced significant gene silencing effects at the cellular level. In addition to the complexation, the conjugation of siRNA to AB diblock copolymers is also a pioneering strategy for siRNA delivery. Bulmus et al. conjugated thiol-modified siRNA to ω-pyridyl disulfide modified poly(ethylene glycol)-b-poly(cholesterol methacrylate) (PEG-b-PCMA) (Fig. 7b).94 The resulting PEG-b-PCMA-b-siRNA modular terpolymer released PCMA-b-siRNA in acidic condition and then released siRNA in reducing condition.
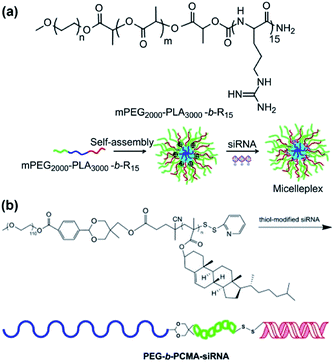 |
| Fig. 7 (a) Micelleplex self-assembled from PEG-b-PLA-b-R15 with negatively charged siRNA by electrostatic interaction for siRNA delivery. Reprinted with permission.93 Copyright (2012) Elsevier. (b) Conjugation of siRNA to form ABC terpolymers for siRNA delivery. Reprinted with permission.94 Copyright (2013) Elsevier. | |
3.3. Template for the fabrication of inorganic hollow nanospheres
The pioneering application of PEG-based ABC terpolymers is the formation of core–shell–corona polymeric micelles as the template for the fabrication of inorganic hollow nanospheres (Fig. 8).95 Polymeric micelles are mainly divided into three classes with neutral, cationic, and anionic shell structures that can absorb the corresponding inorganic precursors to allow the fabrication of varied inorganic hollow nanospheres, such as silicas, hybrid silicas, metal oxide, metal carbonate, metal sulfate, metal phosphate, and metal borates. As a case of neutral micelle, Nakashima et al. reported the synthesis of hollow silica nanospheres using poly(ethylene glycol)-b-poly(2-vinyl pyridine)-b-polystyrene (PEG-b-P2VP-b-PS).96 They also developed cationic micelle of poly(ethylene glycol)-b-poly((3-(methacryloylamino)propyl)trimethylammonium chloride)-b-polystyrene (PEG-b-PMAPTAC-b-PS) for the synthesis of periodic organosilica hollow nanospheres.97 Anionic micelle of poly(ethylene glycol)-b-poly(acrylic acid)-b-polystyrene (PEG-b-PAA-b-PS) has been used for the fabrication of hollow nanospheres of CaCO3.98
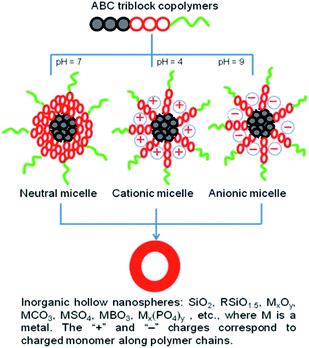 |
| Fig. 8 ABC terpolymers used for the fabrication of inorganic hollow nanospheres. Reprinted with permission.95 Copyright (2014) American Chemical Society. | |
3.4. Stabilizer of metal nanoparticles
ABC terpolymers have attached increasing attention as the stabilizer of metal nanoparticles,99 for example, poly(ethylene glycol)-b-poly(2,3-dihydroxypropyl methacrylate)-b-poly(2-(diisopropylamino)ethyl methacrylate) (PEG-b-PDHPMA-b-PDPAEMA)100 and poly(ethylene glycol)-b-poly(N-isopropylacrylamide)-b-poly((3-acrylamidopropyl)trimethyl ammonium chloride) (PEG-b-PNIPAM-b-PN).101 We used poly(ethylene glycol)-b-linear polyethylenimine-b-poly(ε-caprolactone) (PEG-b-PEI-b-PCL) as the stabilizer to prepare highly dispersed gold nanoparticles (Fig. 9).102 PEG-b-PEI-b-PCL self-assembled in aqueous solution and Au ions were absorbed in the interlayer of polymeric micelles. After the addition of sodium borohydride, ABC terpolymer-stabilized gold nanoparticles were prepared. The fabrication route for ABC terpolymer-stabilized gold nanoparticles is mild, convenient, and reproducible. The as-prepared ABC terpolymer-stabilized gold nanoparticles had high catalytic activity for the reduction of 4-nitrophenol in aqueous solution. The results demonstrated that ABC terpolymers have potential for the synthesis of metal nanoparticles. Similarly, poly(ethylene glycol)-b-poly(2-vinyl pyridine)-b-polystyrene (PEG-b-P2VP-b-PS), a commercially available ABC terpolymer, was employed as the stabilizer of gold nanoparticles.32
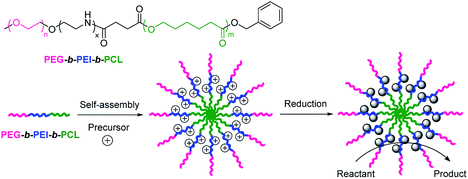 |
| Fig. 9 Formation and catalytic reaction of ABC terpolymer-stabilized gold nanoparticles. Reprinted with permission.102 Copyright (2015) Elsevier. | |
4. Conclusion and outlook
PEG-based ABC terpolymers using commercially available PEG as block A are highlighted. PEG-based ABC terpolymers with PEG as block B103 or prepared by AROP of ethylene oxide104 have been also reported. PEG-based ABC terpolymers are mainly divided into linear, 3-miktoarm, and cyclic as for the architecture. It has been demonstrated that cyclic copolymers synthesized via cyclization of linear AB diblock105 or ABA triblock106–108 copolymers show a longer degradation time and form more stable dense micelles in aqueous solution than linear analogs arising from the cyclic topology. To explore synthetic access of PEG-based ABC miktoarm terpolymers, some techniques such as the Passerini three component reaction109 for the synthesis of difunctional end group-modified PEG and Janus polymerization110 for a combination of cationic and anionic polymerizations into the two ends of PEG have been employed to prepare topologically defined polymers.
PEG-based ABC terpolymers as a kind of advanced polymers have been widely investigated and developed. Because of their varied topologies and molecular variables, PEG-based ABC terpolymers have been widely applied in drug carriers, gene vectors, the template for the fabrication of inorganic hollow nanospheres, and the stabilizer of metal nanoparticles. However, there are few cases in clinical use. PEG-based ABC terpolymers usually require high purity monomers and multi-step synthesis. So far, it is still a challenge to easily and effectively synthesize well-defined and precise controlled ABC terpolymers and to reduce the cost of synthesis. PEG exhibits good biocompatibility and non-toxicity, however, other blocks such as PNIPAM may introduce the toxicity. To fulfil their proposed biomedical potential, methods to prepare non-toxic PEG-based ABC terpolymers are desirable. These challenges will undoubtedly inspire more research in the future.
Conflicts of interest
There are no conflicts to declare.
Acknowledgements
This work was financially supported by the National Natural Science Foundation of China (No. 51703209 and 31660138) and National Key Research and Development Plan (2017YFE0125700, 2008CB418000, 2006CB932302).
Notes and references
- A. A. D'Souza and R. Shegokar, Expert Opin. Drug Delivery, 2016, 13, 1257–1275 CrossRef PubMed.
- A. Kolate, D. Baradia, S. Patil, I. Vhora, G. Kore and A. Misra, J. Controlled Release, 2014, 192, 67–81 CrossRef CAS PubMed.
- A. B. Kutikov and J. Song, ACS Biomater. Sci. Eng., 2015, 1, 463–480 CrossRef CAS PubMed.
- A. El Jundi, S. Buwalda, A. Bethry, S. Hunger, J. Coudane, Y. Bakkour and B. Nottelet, Biomacromolecules, 2020, 21, 397–407 CrossRef CAS PubMed.
- Y. Y. Mai and A. Eisenberg, Chem. Soc. Rev., 2012, 41, 5969–5985 RSC.
- A. Rosler, G. W. M. Vandermeulen and H. A. Klok, Adv. Drug Delivery Rev., 2012, 64, 270–279 CrossRef.
- F. S. Bates, M. A. Hillmyer, T. P. Lodge, C. M. Bates, K. T. Delaney and G. H. Fredrickson, Science, 2012, 336, 434–440 CrossRef CAS PubMed.
- J. Z. Du and R. K. O'Reilly, Chem. Soc. Rev., 2011, 40, 2402–2416 RSC.
- T. I. Lobling, O. Borisov, J. S. Haataja, O. Ikkala, A. H. Groschel and A. H. E. Muller, Nat. Commun., 2016, 7, 12097 CrossRef PubMed.
- Y. Zhou, X. P. Long, X. G. Xue, W. Qian and C. Y. Zhang, RSC Adv., 2015, 5, 7661–7664 RSC.
- S. Li, J. L. He, M. Z. Zhang, H. R. Wang and P. H. Ni, Polym. Chem., 2016, 7, 1773–1781 RSC.
- Y. P. Wu, Y. C. Tao, K. Cai, S. W. Liu, Y. Zhang, Z. G. Chi, J. R. Xu and Y. Wei, Chin. J. Chem., 2015, 33, 1338–1346 CrossRef CAS.
- R. Fernandez-Alvarez, E. Hlavatovicova, K. Rodzen, A. Strachota, S. Kereiche, P. Matejicek, J. Cabrera-Gonzalez, R. Nunez and M. Uchman, Polym. Chem., 2019, 10, 2774–2780 RSC.
- T. Nomoto, S. Fukushima, M. Kumagai, K. Machitani, Arnida, Y. Matsumoto, M. Oba, K. Miyata, K. Osada, N. Nishiyama and K. Kataoka, Nat. Commun., 2014, 5, 3545 CrossRef PubMed.
- R. Bahrami, T. I. Lobling, H. Schmalz, A. H. E. Muller and V. Altstadt, Polymer, 2015, 80, 52–63 CrossRef CAS.
- A. Dag, J. C. Zhao and M. H. Stenzel, ACS Macro Lett., 2015, 4, 579–583 CrossRef CAS.
- E. V. Konishcheva, U. E. Zhumaev, M. Kratt, V. Oehri and W. Meier, Macromolecules, 2017, 50, 7155–7168 CrossRef CAS.
- E. Konishcheva, D. Daubian, J. Gaitzsch and W. Meier, Helv. Chim. Acta, 2018, 101, e1700287 CrossRef.
- S. Davaran, A. Ghamkhari, E. Alizadeh, B. Massoumi and M. Jaymand, J. Colloid Interface Sci., 2017, 488, 282–293 CrossRef CAS PubMed.
- J. Jin, J. Tian, X. M. Lian, P. C. Sun and H. Y. Zhao, Soft Matter, 2011, 7, 11194–11202 RSC.
- T. I. Lobling, P. Hiekkataipale, A. Hanisch, F. Bennet, H. Schmalz, O. Ikkala, A. H. Groeschel and A. H. E. Muller, Polymer, 2015, 72, 479–489 CrossRef.
- M. J. Barthel, U. Mansfeld, S. Hoeppener, J. A. Czaplewska, F. H. Schacher and U. S. Schubert, Soft Matter, 2013, 9, 3509–3520 RSC.
- K. Kempe, A. Baumgaertel, R. Hoogenboom and U. S. Schubert, J. Polym. Sci., Part A: Polym. Chem., 2010, 48, 5100–5108 CrossRef CAS.
- J. Bian, Y. Hao, J. L. He, W. L. Zhang, M. Z. Zhang and P. H. Ni, J. Polym. Sci., Part A: Polym. Chem., 2014, 52, 3005–3016 CrossRef CAS.
- A. Skandalis and S. Pispas, Polym. Chem., 2017, 8, 4538–4547 RSC.
- J. C. Chen, M. Z. Liu, H. H. Gong, Y. J. Huang and C. Chen, J. Phys. Chem. B, 2011, 115, 14947–14955 CrossRef CAS PubMed.
- X. Z. Zhao, K. C. Deng, F. J. Liu, X. L. Zhang, H. R. Yang, J. L. Peng, Z. K. Liu, L. W. Ma, B. Y. Wang and H. Wei, ACS Biomater. Sci. Eng., 2018, 4, 566–575 CrossRef CAS.
- H. Wang, Y. Yao, L. Han and S. A. Che, Chem. Res. Chin. Univ., 2014, 30, 863–867 CrossRef CAS.
- M. J. Barthel, F. H. Schacher and U. S. Schubert, Polym. Chem., 2014, 5, 2647–2662 RSC.
- G. Polymeropoulos, P. Bilalis and N. Hadjichristidis, ACS Macro Lett., 2016, 5, 1242–1246 CrossRef CAS.
- V. Musteata, B. Sutisna, G. Polymeropoulos, A. Avgeropoulos, F. Meneau, K. V. Peinemann, N. Hadjichristidis and S. P. Nunes, Eur. Polym. J., 2018, 100, 121–131 CrossRef CAS.
- Y. Dai, T. Ren, Y. Wang and X. J. Zhang, Gold Bull., 2017, 50, 123–129 CrossRef CAS.
- M. Nuruzzaman, Y. J. Liu, M. M. Rahman, R. Naidu, R. Dharmarajan, H. K. Shon and Y. C. Woo, Langmuir, 2018, 34, 13584–13596 CrossRef CAS PubMed.
- S. Chavda, S. Yusa, M. Inoue, L. Abezgauz, E. Kesselman, D. Danino and P. Bahadur, Eur. Polym. J., 2013, 49, 209–216 CrossRef CAS.
- G. D. Deng, Z. Qiang, W. Lecorchick, K. A. Cavicchi and B. D. Vogt, Langmuir, 2014, 30, 2530–2540 CrossRef CAS PubMed.
- H. B. Liu, W. Wang, H. Y. Yin and Y. J. Feng, Langmuir, 2015, 31, 8756–8763 CrossRef CAS PubMed.
- H. B. Liu, Z. R. Guo, S. He, H. Y. Yin and Y. J. Feng, RSC Adv., 2016, 6, 86728–86735 RSC.
- S. J. Lin, J. J. Shang and P. Theato, Polym. Chem., 2017, 8, 2619–2629 RSC.
- S. Guragain and J. Perez-Mercader, Polym. Chem., 2018, 9, 4000–4006 RSC.
- J. Gaitzsch, S. Hirschi, S. Freimann, D. Fotiadis and W. Meier, Nano Lett., 2019, 19, 2503–2508 CrossRef CAS PubMed.
- T. L. Nghiem, T. I. Lobling and H. Groschel, Polym. Chem., 2018, 9, 1583–1592 RSC.
- C. H. Luo, N. Wei, X. F. Luo and F. L. Luo, Macromol. Chem. Phys., 2018, 219, 1800124 CrossRef.
- W. J. Guo, X. D. Tang, J. Xu, X. Wang, Y. Chen, F. Q. Yu and M. S. Pei, J. Polym. Sci., Part A: Polym. Chem., 2011, 49, 1528–1534 CrossRef CAS.
- Y. H. Xing, S. L. Lin, J. P. Lin and X. H. He, Chin. J. Polym. Sci., 2013, 31, 833–840 CrossRef CAS.
- B. B. Xu, W. Q. Yao, Y. J. Li, S. Zhang and X. Y. Huang, Sci. Rep., 2016, 6, 39504 CrossRef CAS PubMed.
- J. Yao, Y. L. Ruan, T. Zhai, J. Guan, G. P. Tang, H. R. Li and S. Dai, Polymer, 2011, 52, 3396–3404 CrossRef CAS.
- C. Q. Mao, J. Z. Du, T. M. Sun, Y. D. Yao, P. Z. Zhang, E. W. Song and J. Wang, Biomaterials, 2011, 32, 3124–3133 CrossRef CAS PubMed.
- Y. L. Zhao, J. Li, H. Yu, G. J. Wang and W. Liu, Int. J. Pharm., 2012, 430, 282–291 CrossRef CAS PubMed.
- Y. Lv, B. Yang, Y. M. Li, Y. Wu, F. He and R. X. Zhuo, Colloids Surf., B, 2014, 122, 223–230 CrossRef CAS PubMed.
- S. Petrova, C. G. Venturini, A. Jager, E. Jager, P. Cernoch, S. Kereiche, L. Kovacik, I. Raska and P. Stepanek, Polymer, 2015, 59, 215–225 CrossRef CAS.
- C. Y. Li, X. L. Liu, S. S. He, Y. B. Huang and D. M. Cui, Polym. Chem., 2016, 7, 1121–1128 RSC.
- E. V. Konishcheva, U. E. Zhumaev and W. P. Meier, Macromolecules, 2017, 50, 1512–1520 CrossRef CAS.
- Y. Pan, Y. X. Qi, N. N. Shao, A. C. Tadle and Y. B. Huang, Biomacromolecules, 2019, 20, 3575–3583 CrossRef CAS PubMed.
- Y. L. Shi, W. W. Ma, M. Gao and Y. X. Yang, Colloids Surf., B, 2019, 184, 110525 CrossRef CAS PubMed.
- Y. J. Lu, X. D. Gao, M. Cao, B. Wu, L. F. Su, P. Chen, J. B. Miao, S. Wang, R. Xia and J. S. Qian, Colloids Surf., B, 2020, 189, 110830 CrossRef CAS PubMed.
- Y. Matter, R. Enea, O. Casse, C. C. Lee, J. Baryza and W. Meier, Macromol. Chem. Phys., 2011, 212, 937–949 CrossRef CAS.
- W. H. Chen, M. Y. Hua and R. S. Lee, J. Appl. Polym. Sci., 2012, 125, 2902–2913 CrossRef CAS.
- C. B. Xi, D. Yang, J. Li, J. J. Yan and J. H. Hu, Chin. J. Org. Chem., 2012, 32, 2166–2170 CrossRef CAS.
- J. Xuan, D. H. Han, H. S. Xia and Y. Zhao, Langmuir, 2014, 30, 410–417 CrossRef CAS PubMed.
- L. Li, B. B. Lu, Q. K. Fan, L. L. Wei, J. N. Wu, J. Hou, X. H. Guo and Z. Y. Liu, RSC Adv., 2016, 6, 27102–27112 RSC.
- T. K. Endres, M. Beck-Broichsitter, O. Samsonova, T. Renette and T. H. Kissel, Biomaterials, 2011, 32, 7721–7731 CrossRef CAS PubMed.
- T. Thambi, V. G. Deepagan, H. Ko, D. S. Lee and J. H. Park, J. Mater. Chem., 2012, 22, 22028–22036 RSC.
- Y. X. Qi, B. Li, Y. P. Wang and Y. B. Huang, Polym. Chem., 2017, 8, 6964–6971 RSC.
- C. F. Yang, Z. L. Xue, Y. L. Liu, J. Y. Xiao, J. R. Chen, L. J. Zhang, J. W. Guo and W. J. Lin, Mater. Sci. Eng., C, 2018, 84, 254–262 CrossRef CAS PubMed.
- R. Liu, B. He, D. Li, Y. S. Lai, J. Z. Tang and Z. W. Gu, Polymer, 2012, 53, 1473–1482 CrossRef CAS.
- H. H. Huo, X. Y. Ma, Y. Q. Dong and F. J. Qu, Eur. Polym. J., 2017, 87, 331–343 CrossRef CAS.
- Y. F. Chu, H. Yu, Y. T. Zhang, G. Y. Zhang, Y. Y. Ma, R. X. Zhuo and X. L. Jiang, J. Polym. Sci., Part A: Polym. Chem., 2014, 52, 3346–3355 CrossRef CAS.
- K. Van Butsele, M. Morille, C. Passirani, P. Legras, J. P. Benoit, S. K. Varshney, R. Jerome and C. Jerome, Acta Biomater., 2011, 7, 3700–3707 CrossRef CAS PubMed.
- S. Cajot, K. Van Butsele, A. Paillard, C. Passirani, E. Garcion, J. P. Benoit, S. K. Varshney and C. Jerome, Acta Biomater., 2012, 8, 4215–4223 CrossRef CAS PubMed.
- Y. Bai, X. D. Fan, W. Tian, H. Yao, W. W. Fan, T. T. Liu, J. Dang and X. Z. Zhu, Acta Polym. Sin., 2014, 851–859 CAS.
- X. Y. Liu, Y. L. Ding, J. Liu, S. L. Lin and Q. X. Zhuang, Eur. Polym. J., 2019, 116, 342–351 CrossRef CAS.
- J. Liu, Y. L. Ding, X. Y. Liu, S. L. Lin and Q. X. Zhuang, Eur. Polym. J., 2019, 118, 465–473 CrossRef CAS.
- X. Y. Huan, D. L. Wang, R. J. Dong, C. L. Tu, B. S. Zhu, D. Y. Yan and X. Y. Zhu, Macromolecules, 2012, 45, 5941–5947 CrossRef CAS.
- O. Altintas, D. Schulze-Suenninghausen, B. Luy and C. Barner-Kowollik, Eur. Polym. J., 2015, 62, 409–417 CrossRef CAS.
- W. Q. Sun, X. H. He, C. Y. Gao, X. J. Liao, M. R. Xie, S. L. Lin and D. Y. Yan, Polym. Chem., 2013, 4, 1939–1949 RSC.
- Y. Y. Yuan and J. Wang, Colloids Surf., B, 2011, 85, 81–85 CrossRef CAS PubMed.
- M. Liu, X. L. Chen, Z. P. Yang, Z. Xu, L. Z. Hong and T. Ngai, ACS Appl. Mater. Interfaces, 2016, 8, 32250–32258 CrossRef CAS PubMed.
- M. Huo, H. T. Du, M. Zeng, L. Pan, T. Fang, X. M. Xie, Y. Wei and J. Y. Yuan, Polym. Chem., 2017, 8, 2833–2840 RSC.
- R. Alizadeh and M. Ghaemy, Polymer, 2015, 66, 179–191 CrossRef CAS.
- U. S. Gunay, H. Durmaz, E. Gungor, A. Dag, G. Hizal and U. Tunca, J. Polym. Sci., Part A: Polym. Chem., 2012, 50, 729–735 CrossRef CAS.
- B. Iskin, G. Yilmaz and Y. Yagci, J. Polym. Sci., Part A: Polym. Chem., 2011, 49, 2417–2422 CrossRef CAS.
- S. Ozlem, B. Iskin, G. Yilmaz, M. Kukut, J. Hacaloglu and Y. Yagci, Eur. Polym. J., 2012, 48, 1755–1767 CrossRef CAS.
- B. Iskin, G. Yilmaz and Y. Yagci, Polym. Chem., 2011, 2, 2865–2871 RSC.
- A. O. Moughton, M. A. Hillmyer and T. P. Lodge, Macromolecules, 2012, 45, 2–19 CrossRef CAS.
- N. Ghasdian, D. M. A. Buzza, P. D. I. Fletcher and T. K. Georgiou, Macromol. Rapid Commun., 2015, 36, 528–532 CrossRef CAS PubMed.
- Q. Zhang, M. R. Vakili, X. F. Li, A. Lavasanifar and X. C. Le, Mol. Pharm., 2016, 13, 4021–4033 CrossRef CAS PubMed.
- J. K. Palacios, A. Tercjak, G. M. Liu, D. J. Wang, J. P. Zhao, N. Hadjichristidis and A. J. Muller, Macromolecules, 2017, 50, 7268–7281 CrossRef CAS.
- H. Cabral, K. Miyata, K. Osada and K. Kataoka, Chem. Rev., 2018, 118, 6844–6892 CrossRef CAS PubMed.
- Y. Dai, D. J. Wu, S. J. Lin, X. Ma, X. J. Zhang and F. Xia, J. Nanopart. Res., 2019, 21, 5 CrossRef.
- Y. Dai, X. J. Zhang and R. X. Zhuo, RSC Adv., 2016, 6, 22964–22968 RSC.
- A. T. Press, A. Ramoji, M. V. Luhe, A. C. Rinkenauer, J. Hoff, M. Butans, C. Rossel, C. Pietsch, U. Neugebauer, F. H. Schacher and M. Bauer, NPG Asia Mater., 2017, 9, e444 CrossRef CAS.
- T. Endres, M. Y. Zheng, M. Beck-Broichsitter, O. Samsonova, H. Debus and T. Kissel, J. Controlled Release, 2012, 160, 583–591 CrossRef CAS PubMed.
- Z. X. Zhao, S. Y. Gao, J. C. Wang, C. J. Chen, E. Y. Zhao, W. J. Hou, Q. Feng, L. Y. Gao, X. Y. Liu, L. R. Zhang and Q. Zhang, Biomaterials, 2012, 33, 6793–6807 CrossRef CAS PubMed.
- X. Huang, S. I. Sevimli and V. Bulmus, Eur. Polym. J., 2013, 49, 2895–2905 CrossRef CAS.
- M. Sasidharan and K. Nakashima, Acc. Chem. Res., 2014, 47, 157–167 CrossRef CAS PubMed.
- D. A. Liu, M. Sasidharan and K. Nakashima, J. Colloid Interface Sci., 2011, 358, 354–359 CrossRef CAS PubMed.
- M. Sasidharan, K. Nakashima, N. Gunawardhana, T. Yokoi, M. Ito, M. Inoue, S. Yusa, M. Yoshio and T. Tatsumi, Nanoscale, 2011, 3, 4768–4773 RSC.
- B. P. Bastakoti, S. Guragain, Y. Yokoyama, S. Yusa and K. Nakashima, Langmuir, 2011, 27, 379–384 CrossRef CAS PubMed.
- Y. Dai and X. J. Zhang, Macromol. Mater. Eng., 2018, 303, 1800105 CrossRef.
- R. C. B. Scaravelli, R. L. Dazzi, F. C. Giacomelli, G. Machado, C. Giacomelli and V. Schmidt, J. Colloid Interface Sci., 2013, 397, 114–121 CrossRef CAS PubMed.
- R. Pamies, K. Z. Zhu, A. L. Kjoniksen and B. Nystrom, J. Nanopart. Res., 2016, 18, 319 CrossRef.
- Y. Dai, Y. Li and S. P. Wang, J. Catal., 2015, 329, 425–430 CrossRef CAS.
- I. Freudensprung, M. Klapper and K. Mullen, Macromol. Rapid Commun., 2016, 37, 209–214 CrossRef CAS PubMed.
- C. Zhou, M. A. Hillmyer and T. P. Lodge, Macromolecules, 2011, 44, 1635–1641 CrossRef CAS.
- B. Y. Zhang, H. Zhang, Y. J. Li, J. N. Hoskins and S. M. Grayson, ACS Macro Lett., 2013, 2, 845–848 CrossRef CAS.
- E. Baba, S. Honda, T. Yamamoto and Y. Tezuka, Polym. Chem., 2012, 3, 1903–1909 RSC.
- K. Heo, Y. Y. Kim, Y. Kitazawa, M. Kim, K. S. Jin, T. Yamamoto and M. Ree, ACS Macro Lett., 2014, 3, 233–239 CrossRef CAS.
- E. Baba, T. Yatsunami, Y. Tezuka and T. Yamamoto, Langmuir, 2016, 32, 10344–10349 CrossRef CAS PubMed.
- L. Li, X. W. Kan, X. X. Deng, C. C. Song, F. S. Du and Z. C. Li, J. Polym. Sci., Part A: Polym. Chem., 2013, 51, 865–873 CrossRef CAS.
- Y. Li, M. von der Luhe, F. H. Schacher and J. Ling, Macromolecules, 2018, 51, 4938–4944 CrossRef CAS.
|
This journal is © The Royal Society of Chemistry 2020 |
Click here to see how this site uses Cookies. View our privacy policy here.