DOI:
10.1039/D0RA02702B
(Paper)
RSC Adv., 2020,
10, 19747-19750
Emodin as a novel organic photocatalyst for selective oxidation of sulfides under mild conditions†
Received
24th March 2020
, Accepted 18th May 2020
First published on 27th May 2020
Abstract
Herein, we have developed naturally-occurring Emodin, which is commercially available at low-cost, as a novel organic photocatalyst for the first time. Emodin was successfully employed in the selective oxidation of sulfides promoted by visible-light, delivering valuable sulfoxides with high efficiency. Mechanistic investigations suggested both single-electron transfer (SET) and energy transfer (EnT) pathways might be involved in the oxidation reaction.
Visible-light-promoted reactions have attracted great interest from chemists over the past decade for the usage of sunlight as the renewable energy source.1 As a logical consequence, the development of novel photocatalysts plays an essential role in the field of photocatalysis.2 Among them, organo-photocatalysts, such as Rose bengal,3 Eosin Y,4 9,10-dicyanoanthracene (DCA),5 etc, were more appealing due to the avoidance of toxic, expensive and environmentally unfriendly metals (Fig. 1).6 However, the investigations of organic photocatalysts were restricted in a limited number of well-developed skeletons. Thus, it is worth further developing more varieties of organo-photocatalysts with versatile frameworks.
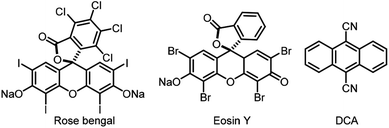 |
| Fig. 1 Selected examples for organic photocatalysts. | |
Meanwhile, nature generously provided plentiful natural products as high-efficient catalysts.7 Recently, we have successfully exploited cercosporin8 from plant pathogenic fungi Cercospora species as a novel photocatalyst in oxidation,9 cycloaddition10 and cross-coupling11 reactions. Take it into consideration that Emodin12 has the similar quinone skeleton with hydroxy groups and photostablity (see ESI†) as cercosporin, we rationalize naturally-occurring Emodin might have potential photophysical properties13 as a novel photoredox catalyst, which has yet not been reported to the best of our knowledge (Scheme 1a).
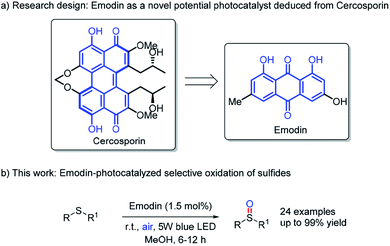 |
| Scheme 1 Emodin-catalyzed selective oxidation of sulfides. | |
On the other hand, selective oxidation is a kind of fundamental reactions along with significant challenges because of the over-oxidation and the hazardous oxidizing agents employed in the reactions.14 With the growing environmental concerns, photocatalytic selective oxidation has been considered as an alternative method utilizing oxygen as green terminal oxidant and visible-light as renewable energy source.15 Based on our continuous interest in developing novel photocatalysts from natural products and their applications in photoredox-catalyzed reactions, herein, we will report the first example of Emodin-catalyzed selective oxidation of sulfides with the promotion of visible-light (Scheme 1b).
After systematic reaction condition evaluations, the selective oxidation of thioanisole was successfully achieved in 99% yield with Emodin (1.5 mol%) as the photocatalyst in methanol at room temperature under air atmosphere (Table 1, entry 1). Solvents screening revealed that protonic solvents (MeOH and EtOH) showed better results than others like DMF, DMSO, dioxane, THF and toluene (Table 1, entries 2–7). Increasing the amount of Emodin had little influence on the reaction, while decreasing the amount to 1 and 0.5 mol% would decelerate the reaction (Table 1, entries 8–10). Additionally, in this selective oxidation process, 5 W blue LED was proved better light source than corresponding CFL and green LED (Table 1, entries 11–12), which shows that when the maximum absorption wavelength of the photocatalyst is consistent with the emission spectrum of the light source (see ESI†), the catalytic efficiency is the highest. Unexpectedly, a reduced result was obtained using 30 W blue LED, which probably resulted from the side-reactions induced by high energy of the light source (Table 1, entry 13). Other organic photocatatlysts were also tested, Eosin Y has the similar photocatalytic efficiency compared to Emodin (Table 1, entry 14), while the photocatalytic activities of other organic catalysts (methylene blue, methyl orange and Rhodamine B) were obviously lower than that of Emodin (Table 1, entries 15–17).
Table 1 Effect of reaction parametersa
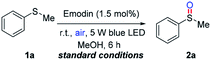
|
Entry |
Variation from the standard conditions |
Yieldb (%) |
Conducted with 1a (0.25 mmol), Emodin (1.5 mol%) in methanol (2 mL) at room temperature under air atmosphere for 6 h. Isolated yield. |
1 |
None |
99 |
2 |
EtOH instead of MeOH |
82 |
3 |
DMSO instead of MeOH |
25 |
4 |
DMF instead of MeOH |
41 |
5 |
Dioxane instead of MeOH |
53 |
6 |
THF instead of MeOH |
19 |
7 |
Toluene instead of MeOH |
24 |
8 |
Emodin (2%) instead of Emodin (1.5%) |
99 |
9 |
Emodin (1%) instead of Emodin (1.5%) |
90 |
10 |
Emodin (0.5%) instead of Emodin (1.5%) |
76 |
11 |
5 W CFL instead of 5 W blue LED |
71 |
12 |
5 W green LED instead of 5 W blue LED |
29 |
13 |
30 W blue LED instead of 5 W blue LED |
58 |
14 |
Eosin Y instead of Emodin |
93 |
15 |
Methylene blue instead of Emodin |
51 |
16 |
Methyl orange instead of Emodin |
None |
17 |
Rhodamine B instead of Emodin |
None |
With the optimal reaction conditions, we next investigated the substrate scope of aryl alkyl sulfides, and the results were summarized in Table 2. Electron-donating group (–OMe) and halogen groups (–Br, –Cl) at the para-position of the benzene ring led to the oxidation products 2b–2d in 93–99% yields; electron-withdrawing group (–CN) led to 2e in moderate yield. Products 2f–2h with meta-substitutions were produced in 65–99% yields. Unfortunately, substrate with unprotected amino group (1i) was an unsuccessful example in the reaction. When methoxy group was substituted at the ortho-position of the benzene ring, 2j could be delivered in 98% yield; while 2k and 2l with bromine and chlorine atoms at the ortho-position showed poor efficiency probably due to the steric hindrance. Moreover, this oxidative reaction was tolerant of ethyl (2m, 2n), allyl (2o) and benzyl (2p) sulfides.
Table 2 Substrate scope of aryl alkyl sulfidesa,b
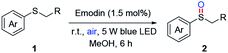
|
Conducted with 1 (0.25 mmol), Emodin (1.5 mol%) in methanol (2 mL) at room temperature under air atmosphere for 6–12 h. Isolated yield. |
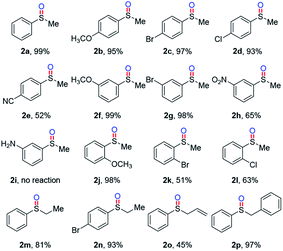 |
Subsequently, other sulfides with two alkyl groups or two aryl groups were tested (Table 3). Benzyl sulfoxide 2q and n-butyl sulfoxide 2r were achieved in good to excellent yields (77–97%). What is more, symmetric (1s, 1t) and unsymmetrical (1u–1y) di-phenyl sulfides were also compatible in the oxidation reactions, producing corresponding diphenyl sulfoxides 2s–2y in 20–79% yields.
Table 3 Substrate scope of other sulfidesa,b
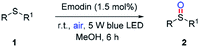
|
Conducted with 1 (0.25 mmol), Emodin (1.5 mol%) in methanol (2 mL) at room temperature under air atmosphere for 6–12 h. Isolated yield. |
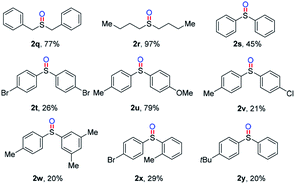 |
Next, several control experiments were carried out to gain more insight into the mechanism of this novel Emodin-catalyzed oxidation reaction of sulfides. The reaction was completely inhibited without the catalyst or the light source; and the reaction was not affected when air was changed to oxygen, but failed if air was replaced by nitrogen, which revealing the roles of these factors (Scheme 2a). Furthermore, the radical and singlet oxygen inhibition experiments suggested that both O2˙− and 1O2 are responsible for the Emodin-catalyzed oxidation reaction of sulfides (Scheme 2b). According to the above-mentioned results and previous literature, we have proposed the reaction mechanism in Scheme 2c. First, the photocatalyst Emodin was excited with light irradiation to generate the excited species Emodin*. In the SET cycle, reductive quenching occurred to produce sulfide radical cation 3 along with the radical anion Emodin˙−. Subsequently, Emodin˙− was oxidized by oxygen to regenerate Emodin into the next catalytic cycle and achieve the reactive radical anion O2˙−, which further reacted with radical cation 3 to deliver methyl phenyl sulfoxide 2a. While in the EnT cycle, singlet oxygen 1O2 was produced to oxidize 1a to the product 2a via the intermediate 4, and photocatalyst Emodin was regenerated simultaneously.
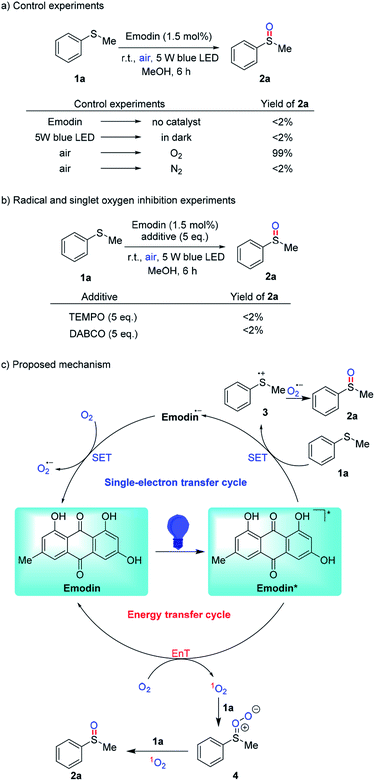 |
| Scheme 2 Mechanistic insights. | |
Conclusions
The natural product Emodin has been developed as a novel organic photocatalyst for the first time. Emodin-photocatalyzed selective oxidation reaction of sulfides with the promotion of visible-light proceeded in high efficiency and exhibited good functional group tolerance. Two possible mechanism including SET and Ent cycles were proposed according to the mechanistic investigations. Further investigations on Emodin-catalyzed photoredox reactions are currently underway in our laboratory.
Conflicts of interest
There are no conflicts to declare.
Notes and references
- For selected reviews, see:
(a) D. Ravelli, S. Protti and M. Fagnoni, Chem. Rev., 2016, 116, 9850–9913 CrossRef CAS;
(b) M. N. Hopkinson, B. Sahoo, J.-L. Li and F. Glorius, Chem.–Eur. J., 2014, 20, 3874–3886 CrossRef CAS;
(c) D. P. Hari and B. König, Angew. Chem., Int. Ed., 2013, 52, 4734–4743 CrossRef CAS;
(d) C. K. Prier, D. A. Rankic and D. W. C. MacMillan, Chem. Rev., 2013, 113, 5322–5363 CrossRef CAS;
(e) J. Xuan and W.-J. Xiao, Angew. Chem., Int. Ed., 2012, 51, 6828–6838 CrossRef CAS PubMed;
(f) J. M. R. Narayanam and C. R. J. Stephenson, Chem. Soc. Rev., 2011, 40, 102–113 RSC;
(g) T. P. Yoon, M. A. Ischay and J. Du, Nat. Chem., 2010, 2, 527–532 CrossRef CAS PubMed.
- For selected reviews, see:
(a) F. Glaser and O. S. Wenger, Coord. Chem. Rev., 2020, 405, 213129 CrossRef CAS;
(b) B. M. Hockin, C. Li, N. Robertson and E. Zysman-Colman, Catal. Sci. Technol., 2019, 9, 889–915 RSC;
(c) D. M. Arias-Rotondo and J. K. McCusker, Chem. Soc. Rev., 2016, 45, 5803–5820 RSC;
(d) D. Sudha and P. Sivakumar, Chem. Eng. Process., 2015, 97, 112–133 CrossRef CAS;
(e) P. Liao and E. A. Carter, Chem. Soc. Rev., 2013, 42, 2401–2422 RSC.
- For selected review, see:
(a) S. Sharma and A. Sharma, Org. Biomol. Chem., 2019, 17, 4384–4405 RSC. For selected examples, see:
(b) M. Singsardar, S. Mondal, S. Laru and A. Hajra, Org. Lett., 2019, 21, 5606–5610 CrossRef CAS PubMed;
(c) P. Li and G.-W. Wang, Org. Biomol. Chem., 2019, 17, 5578–5585 RSC;
(d) J.-R. Xin, Y.-H. He and Z. Guan, Org. Chem. Front., 2018, 5, 1684–1688 RSC;
(e) Y. Pan, C. W. Kee, L. Chen and C.-H. Tan, Green Chem., 2011, 13, 2682–2685 RSC.
- For selected examples, see:
(a) K. Wu, L. Wang, S. Colón-Rodríguez, G.-U. Flechsig and T. Wang, Angew. Chem., Int. Ed., 2019, 58, 1774–1778 CrossRef CAS;
(b) G. Zhao and T. Wang, Angew. Chem., Int. Ed., 2018, 57, 6120–6124 CrossRef CAS PubMed;
(c) H. Wang, Y. Li, Z. Tang, S. Wang, H. Zhang, H. Cong and A. Lei, Acs Catal, 2018, 8, 10599–10605 CrossRef CAS;
(d) A. K. Yadav and L. D. S. Yadav, Green Chem., 2016, 18, 4240–4244 RSC;
(e) M. Majek and A. J. von Wangelin, Angew. Chem., Int. Ed., 2015, 54, 2270–2274 CrossRef CAS.
- For selected examples, see:
(a) H.-T. Qin, X. Xu and F. Liu, Chemcatchem, 2017, 9, 1409–1412 CrossRef CAS;
(b) C. Yang, J.-D. Yang, Y.-H. Li, X. Li and J.-P. Cheng, J. Org. Chem., 2016, 81, 12357–12363 CrossRef CAS PubMed;
(c) G. Pandey and R. Laha, Angew. Chem., Int. Ed., 2015, 54, 14875–14879 CrossRef CAS PubMed.
- For selected reviews on organo-photocatalysts, see:
(a) M. Z. Rahman, M. G. Kibria and C. B. Mullins, Chem. Soc. Rev., 2020 10.1039/c9cs00313d;
(b) Y. Zhang, W. Schilling and S. Das, Chemsuschem, 2019, 12, 2898–2910 CrossRef CAS PubMed;
(c) C. Li, Y. Xu, W. Tu, G. Chen and R. Xu, Green Chem., 2017, 19, 882–899 RSC;
(d) N. A. Romero and D. A. Nicewicz, Chem. Rev., 2016, 116, 10075–10166 CrossRef CAS PubMed;
(e) S. Fukuzumi and K. Ohkubo, Org. Biomol. Chem., 2014, 12, 6059–6071 RSC.
- For selected examples, see:
(a) S. B. Kasar and S. R. Thopate, Curr. Org. Synth., 2018, 15, 110–115 CrossRef CAS;
(b) W. Li, S. Fedosov, T. Tan, X. Xu and Z. Guo, Appl. Biochem. Biotechnol., 2014, 173, 278–290 CrossRef CAS PubMed;
(c) H.-F. Jiang, J.-W. Ye, C.-R. Qi and L.-B. Huang, Tetrahedron Lett., 2010, 51, 928–932 CrossRef CAS.
- For selected reviews on Emodin, see:
(a) Y. Tu, Z. Wu, B. Tan, A. Yang and Z. Fang, Oncol. Rep., 2019, 42, 1259–1271 CAS;
(b) L. Li, X. Song, Z. Yin, R. Jia, Z. Li, X. Zhou, Y. Zou, L. Li, L. Yin, G. Yue, G. Ye, C. Lv, W. Shi and Y. Fu, Microbiol. Res., 2016, 186, 139–145 CrossRef PubMed;
(c) X. Dong, J. Fu, X. Yin, S. Cao, X. Li, L. Lin, Huyiligeqi and J. Ni, Phytother. Res., 2016, 30, 1207–1218 CrossRef CAS;
(d) W.-T. Wei, S.-Z. Lin, D.-L. Liu and Z.-H. Wang, Oncol. Rep., 2013, 30, 2555–2562 CrossRef CAS;
(e) I. Izhaki, New Phytol., 2002, 155, 205–217 CrossRef CAS.
-
(a) S. Kuyama and T. Tamura, J. Am. Chem. Soc., 1957, 79, 5725–5726 CrossRef CAS;
(b) S. Kuyama and T. Tamura, J. Am. Chem. Soc., 1957, 79, 5726–5729 CrossRef CAS;
(c) M. E. Daub and M. Ehrenshaft, Annu. Rev. Phytopathol., 2000, 38, 461–490 CrossRef CAS PubMed;
(d) M. E. Daub, Phytopathology, 1987, 77, 1515–1520 CrossRef CAS.
- J. Li, W. Bao, Z. Tang, B. Guo, S. Zhang, H. Liu, S. Huang, Y. Zhang and Y. Rao, Green Chem., 2019, 21, 6073–6081 RSC.
-
(a) J. Li, W. Bao, Y. Zhang and Y. Rao, Org. Biomol. Chem., 2019, 17, 8958–8962 RSC;
(b) Y. Zhang, Y. Cao, L. Lu, S. Zhang, W. Bao, S. Huang and Y. Rao, J. Org. Chem., 2019, 84, 7711–7721 CrossRef CAS.
-
(a) Z. Tang, J. Li, F. Lin, W. Bao, S. Zhang, B. Guo, S. Huang, Y. Zhang and Y. Rao, J. Catal., 2019, 380, 1–8 CrossRef CAS;
(b) S. Zhang, Z. Tang, W. Bao, J. Li, B. Guo, S. Huang, Y. Zhang and Y. Rao, Org. Biomol. Chem., 2019, 17, 4364–4369 RSC.
-
(a) L. Wang, Z. Zhang and B. Ye, Electrochim. Acta, 2006, 51, 5961–5965 CrossRef CAS;
(b) D. Li and B. Jin, J. Electrochem., 2017, 23, 347–355 CAS.
- For selected reviews on selective oxidation reactions, see:
(a) T. Pu, H. Tian, M. E. Ford, S. Rangarajan and I. E. Wachs, Acs Catal, 2019, 9, 10727–10750 CrossRef CAS;
(b) C. Dai, J. Zhang, C. Huang and Z. Lei, Chem. Rev., 2017, 117, 6929–6983 CrossRef CAS PubMed;
(c) A. E. Wendlandt and S. S. Stahl, Angew. Chem., Int. Ed., 2015, 54, 14638–14658 CrossRef CAS PubMed;
(d) K. Liu, H. Ou, X. Shi, X. Dong, W. Ma and J. Wei, Chinese J. Org. Chem., 2014, 34, 681–692 CrossRef CAS.
- For selected reviews on photocatalytic selective oxidation, see:
(a) L. Chen, J. Tang, L.-N. Song, P. Chen, J. He, C.-T. Au and S.-F. Yin, Appl. Catal. B-Environ., 2019, 242, 379–388 CrossRef CAS;
(b) X. Lang, J. Zhao and X. Chen, Angew. Chem., Int. Ed., 2016, 55, 4697–4700 CrossRef CAS PubMed;
(c) N. Zhang, Y. Zhang, X. Pan, X. Fu and Y. Xu, Sci. Sin. Chim., 2011, 41, 1097–1111 CrossRef.
Footnotes |
† Electronic supplementary information (ESI) available. See DOI: 10.1039/d0ra02702b |
‡ These authors contributed equally to this work. |
|
This journal is © The Royal Society of Chemistry 2020 |
Click here to see how this site uses Cookies. View our privacy policy here.