DOI:
10.1039/D0RA01906B
(Paper)
RSC Adv., 2020,
10, 13866-13871
Highly efficient catalyst-free domino conjugate addition, decarboxylation and esterification/amidation of coumarin carboxylic acid/esters with pyrazolones: a green chemistry approach†
Received
28th January 2020
, Accepted 13th March 2020
First published on 6th April 2020
Abstract
Tandem conjugate addition, decarboxylation and esterification/amidation of coumarin 3-carboxylic acid derivatives with pyrazolones have been developed. The reactions were performed with coumarin 3-carboxylic acid/esters and pyrazolone in alcohol as a solvent to afford the corresponding pyrazolyl 2-hydroxy phenylpropionate derivatives. Amines and green solvents were employed for amidation in the addition reaction. The methodology has advantages such as excellent yields, a broad substrate scope, catalyst-free, easy purification by simple filtration without any workup, mild conditions and does not require any organic solvents, ligands, base or any additives. This is a green and general synthetic protocol, which could be applicable for the synthesis of substituted pyrazolyl phenyl propionate/amide derivatives. This approach demonstrates the importance of the coumarin 3-carboxylic acid/ester core structure for Michael addition.
Introduction
Coumarins are naturally occurring and one of the most important classes of organic molecules.1 These compounds have gained considerable interest due to their wide range of biological activities such as anti-oxidants,2 antiasthmatics,3 anti-coagulants,4 anti-inflammatories,5 antivirals,6 antidepressants,7 antimicrobials,8 antinociceptives,9 and anti-tumour agents;10 some naturally occurring coumarin derivatives include warfarin,11 aesculetin,12 and herniarin.13 Furthermore, coumarins show optical properties and their wide applications in laser dyes,14 fluorescent probes,15 and solar cells16 have been reported. On the other hand, pyrazolones are also an important class of compounds in synthetic and medicinal chemistry. These derivatives show numerous bioactivities such as edaravone is marketed as a drug for treating brain and myocardial ischemia,17 and has antitumor,18 anti-tubercular,19 anti-inflammatory,20 antifungal,21 antibacterial,22 antimycobacterial,23 antifilarial24 and antidepressant25 activities.
The hybridization of potential molecules has been known to show improved biological activities compared to their corresponding individual lead compounds. Due to the interesting properties of coumarin and pyrazole, we were interested to synthesise a new class of compounds, which could show better properties.26 In recent years, coumarin 3-carboxylic acids are used as Michael accepters to generate 3,4-dihydro coumarin derivatives. However, these reactions require harsh conditions, activation by a catalyst because of coumarin loses its aromaticity partially, thus limiting the reaction scope.
Recently, Xiao et al.27 reported the synthesis of 3,4-dihydrocoumarines via the addition/decarboxylation of coumarin/thiocoumarin carboxylic acids with indole (Scheme 1). Franz et al.28 disclosed the La(OTf)3-catalysed three-component reaction of coumarin 3 carboxylates for the synthesis of indolylmalonamides. Lin et al.29 reported the α-addition of γ-butyrolactums to coumarin derivatives, and Han et al.30 reported the Rh-catalysed C sp2–H arylation of coumarins for the synthesis of 3,4-dihydro coumarins. To the best of our knowledge, to date, there is no direct report on the addition of pyrazolone on coumarins for the synthesis of pyrazolyl phenylpropionate derivatives.31 Therefore, herein, we report a simple catalyst-free domino conjugate addition, decarboxylation and esterification/amidation of coumarins for the synthesis of pyrazolyl 2-hydroxy phenyl propionic ester/amide derivatives under green reaction conditions.
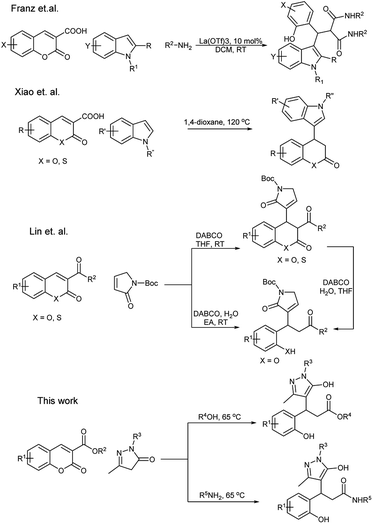 |
| Scheme 1 Decarboxylative additions on coumarins. | |
Results and discussion
In recent times, various investigations have focused on non-traditional synthetic protocols to replace volatile harmful solvents with green solvents, such as aqueous media, ionic liquids, and medium derived from natural feedstocks or solvent-free conditions.32,43 In pursuit of developing a green methodology for the synthesis of bioactive molecules,33 we began our study for the synthesis of pyrazolyl phenyl propionates 3 with coumarin 3-carboxylic acid 1a and 5-methyl-2,4-dihydro-3H-pyrazol-3-one 2a (Scheme 2). The reaction was performed in various solvents for solvent screening at room temperature for 48 h but did not yield any product.
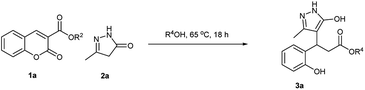 |
| Scheme 2 Optimization of reaction conditions. | |
Then, we carried out the reaction at various temperatures, and reaction at 65 °C in water, surprisingly, afforded the pyrazolyl phenylpropionic acid 3a in 87% yield as a white solid instead of pyrazolyl 3,4-dihydro coumarin after 18 h. The reaction at a higher temperature did not show any effect on the yield of the product. The alcohol solvents were afforded with the corresponding esters as the products. The results are summarised in Table 1. Therefore, from Table 1, it was concluded that the reaction at 65 °C in water/alcohol/Me-THF is the best-optimised reaction condition.
Table 1 Optimization of condition for the synthesis of pyrazolyl coumarin 3-carboxylates
Entrya |
Solvent |
Temp (°C) |
Time (h) |
Yieldb (%) |
All reactions were carried out using coumarin 1a (1 mmol) and pyrazolone 2a (1 mmol) in the specified solvents (0.25 M). Isolated yield. Obtained acid derivative of 3a. Methyl ester of 3a obtained. i-propyl ester of 3a obtained. |
1 |
H2O |
r.t |
48 |
N.R |
2 |
CHCl3 |
r.t |
48 |
N.R |
3 |
MeOH |
r.t |
48 |
N.R |
4 |
THF |
r.t |
48 |
N.R |
5 |
H2O |
65 |
18 |
87c |
6 |
H2O |
100 |
18 |
87c |
7 |
MeOH |
65 |
18 |
92d |
8 |
i-PrOH |
65 |
18 |
96e |
9 |
Me-THF |
65 |
18 |
92c |
Under the optimised condition, the scope of the methodology was studied by varying the substitution on coumarin 3-carboxylic acid's phenyl ring such as alkyl, alkoxy and halogen group at 6th position, and we found that the reaction was clean and afforded the products 3b–e in high yields (Table 2). Further, the reaction was performed with various methyl/ethyl carboxylates of coumarin 1 and 5-methyl-2,4-dihydro-3H-pyrazol-3-one 2a in water afforded the same pyrazolyl phenyl propionic acids 3a–e.
Table 2 Substrate scope for the decarboxylative addition of pyrazolonesab
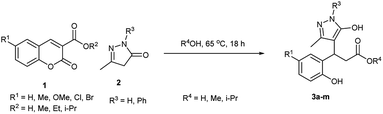
|
All reactions were carried out using coumarin 3-carboxylic acid/ester 1 (1 mmol) and pyrazolone 2 (1 mmol) in the specified solvents (0.25 M). Isolated yield. |
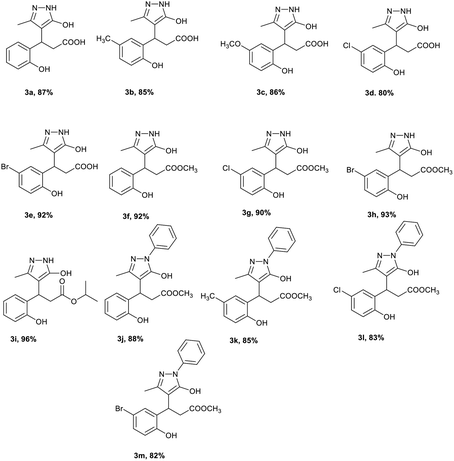 |
The reaction was carried out in different alcohols such as methanol, and isopropyl alcohol as solvents under the optimised conditions, and we obtained the corresponding ester derivatives 3f–i. It is worth mentioning that edaravone is a commercially available drug molecule, which was employed in the reaction under the optimised conditions with various coumarin 3-carboxylic acid/esters which afforded the pyrazolyl phenyl propionic acid 3j–m derivatives in good yields. It is interesting to note that irrespective of the coumarin 3-carboxylic acid/ester as a starting material the formation of the acid/ester as the product depends on the solvent used. In an aqueous reaction medium, coumarin 3-carboxylic acid and esters afforded pyrazolyl phenyl propionic acid 3a–e as the product, and alcohol (MeOH, i-PrOH) as a solvent afforded the corresponding pyrazolyl phenyl propionic ester 3f–m as the product. In view of the above observations, we were interested to explore the possibility of the amidation reaction as most of the drug molecules and natural products commonly possess amide functionality. Several coumarin amide derivatives are shown to be pesticidal,34 anti-bacterial,35 cytotoxic,36 chemosensors,37 and hMAO inhibitors.38 In recent years, several methods were reported for the synthesis of amines from the corresponding carboxylic acids and amines catalysed by diborane,39 tetramethyl orthosilicate,40 aryl boronic acid,41 and XtalFluor-E.42
However, these methods involve expensive catalysts, volatile solvents and anhydrous inert conditions. Therefore, to synthesis amides from coumarins via a catalyst-free method, a one-pot domino decarboxylative amidation reaction was performed in methyl THF as a green solvent using coumarin 3-carboxylic acid 1, 5-methyl-2,4-dihydro-3H-pyrazol-3-one 2 and benzylamine 4 as the substrates. To our delight, the corresponding pyrazolyl amide product 5a was afforded in 92% yield (Table 3). Under the optimised condition, the reaction was explored for the substrate scope. The reaction proceeded smoothly with benzylamine and methyl, bromo coumarins to afford the amide products 5b and 5c. We further explored the scope of the reaction with p-methoxy benzylamine on various coumarins. Simple, Me, OMe and halogenated coumarins performed exceptionally well to give the desired amides 5d–h in high yields. Halo benzylamine also afforded the desired product 5i–o in good yields. To explore the steric effect, the reaction was planned with α-substituted amines. (R)-α-Methyl benzylamine employed on various coumarins afforded an approximately equal amount of inseparable diastereomeric mixture of amide derivatives 5p–t in high yields. Coumarin 3-carboxylic acids and esters specifically afforded exclusively same amides 5a–c under a mild reaction condition.
Table 3 Substrate scope for the decarboxylative addition of pyrazolones and an amidation reactionab
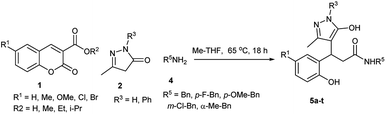
|
All reactions were carried out using coumarin 3-carboxylic acid/ester 1 (1 mmol), pyrazolone 2 (1 mmol) and amine 4 (1.1 mmol) in Me-THF (0.25 M). Isolated yield. |
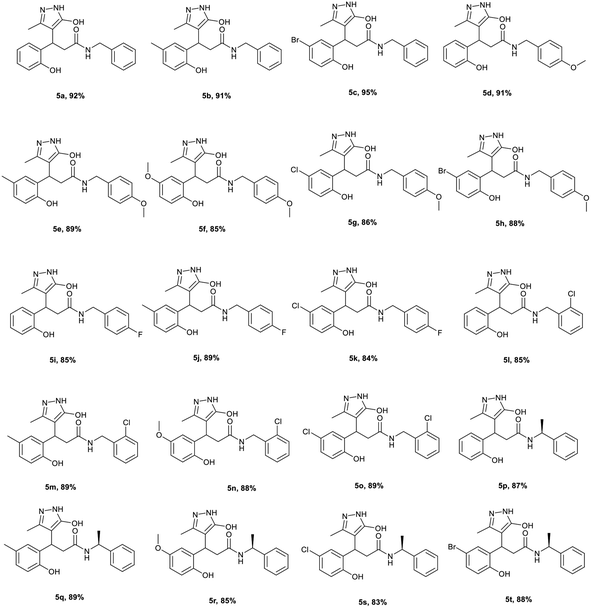 |
We made efforts to synthesis these derivatives via the direct Michael addition of pyrazolone 2a to coumarin 3-carboxylic 1a acid to get the corresponding Michael adduct or product 3a/5a. Therefore, the reaction performed using hydroxycinnamic acid 6 and pyrazolone 2a in methanol refluxed for 20 h did not yield any product (Scheme 3). Similarly, the reaction with benzyl amines in Me-THF failed to give the desired products. Reaction with 3-acyl coumarin 7 and pyrazolone 2a did not yield any product and led to the decomposition of the starting material. However, the chalcone 8 afforded Michael adduct 9. These observations enhanced the importance of the present protocol and importance of the coumarin structure with 3-carboxylic acid/ester, which could make a better Michael acceptor in this reaction.
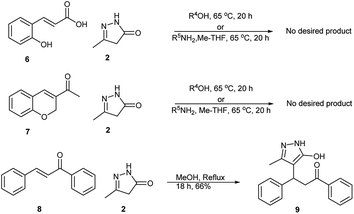 |
| Scheme 3 Direct Michael addition with other substrates. | |
Based on our observations and previous reports, we propose the following mechanism for the reaction. The substrate 5-methyl-2,4-dihydro-3H-pyrazol-3-one 2 will be in equilibrium with its enol form 10 and undergoes Michael addition in the first step to afford the Michael adduct 11 (Fig. 1). The adduct 11 undergoes decarboxylation to afford intermediate 12, which on isomerization forms intermediate 13. The addition of solvent ROH will form intermediate 14, which subsequently undergoes the ring-opening to afford the product 3.29 The mass spectroscopic data was recorded after 5, 10, 15, 20 min intervals of the reaction. The intermediate 11 and 13 were observed in mass spectrum m/z at 289 and 245, respectively, within 5 min of the reaction and the product 3 appeared after 15 min. These observations support the proposed mechanism.
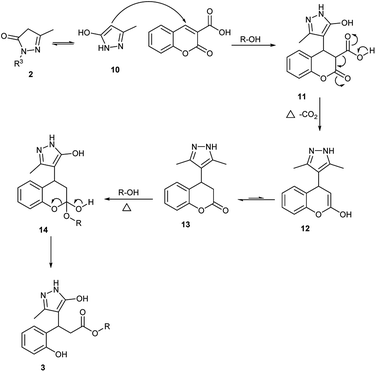 |
| Fig. 1 Plausible reaction mechanism. | |
Conclusions
In conclusion, we have developed a simple green methodology for the synthesis of pyrazolyl phenyl propionic acid via tandem Michael addition, decarboxylation and esterification/amidation in one pot operation under mild conditions without the use of any toxic solvents or metals. The methodology has a broad substrate scope with good yields. In particular, acids were obtained in aqueous reactions and corresponding esters in alcohol as solvents. A series of amides were synthesised by employing this catalyst-free method. The green methodology could be useful for the development of bioactive molecules or lead compounds in a one-pot operation.
Experimental
In a 5 mL round bottom flask containing a solution of coumarin 3-carboxylic acid 1 (1 mmol) in 4 mL of solvent (water/MeOH/i-PrOH), 5-methyl-2,4-dihydro-3H-pyrazol-3-one 2a (1 mmol) was added. The reflux condenser was fixed, and the reaction mixture was stirred for 18 h at 65 °C (the reaction mixture becomes homogeneous while the reaction is in progress). The progress of the reaction was monitored by TLC. After the completion of the reaction, the solid product 3a was filtered using a sintered glass funnel and washed with water (2 × 10 mL) and MeOH (2 × 10 mL). The solid was collected and dried in a vacuum. The obtained product was pure and did not require any further purification.
Conflicts of interest
There are no conflicts to declare.
Acknowledgements
SRL thanks UGC-India for Non-NET fellowship, VS thanks UGC-India for RGNF, Authors thanks to CUG-India for financial and infrastructural support.
Notes and references
- F. G. Medina, J. G. Marrero, M. Macías-Alonso, M. C. González, I. Córdova-Guerrero, A. G. T. Garcíaa and S. Osegueda-Roblesa, Nat. Prod. Rep., 2015, 32, 1472 RSC.
- I. Kostova, S. Bhatia, P. Grigorov, S. Balkansky, V. S. Parmar, A. K. Prasad and L. Saso, Curr. Med. Chem., 2011, 18, 3929 CrossRef CAS PubMed.
- A. Sánchez-Recillas, G. Navarrete-Vázquez, S. Hidalgo- Figueroa, M. Y. Rios, M. Ibarra-Barajas and S. Estrada- Soto, Eur. J. Med. Chem., 2014, 77, 400 CrossRef PubMed.
- X. M. Peng, G. L. Damu and C. Zhou, Curr. Pharm. Des., 2013, 19, 3884 CrossRef PubMed.
- Y. Bansal, P. Sethi and G. Bansal, Med. Chem. Res., 2013, 22, 3049 CrossRef.
-
(a) J. R. Hwu, S. Y. Lin, S. C. Tsay, E. De Clercq, P. Leyssen and J. Neyts, J. Med. Chem., 2011, 54, 2114 CrossRef PubMed;
(b) E. B. B. Ong, N. Watanabe, A. Saito, Y. Futamura, K. H. A. El Galil, A. Koito, N. Najimudin and H. Osada, J. Biol. Chem., 2011, 286, 14049 CrossRef PubMed.
- K. V. Sashidhara, A. Kumar, M. Chatterjee, K. B. Rao, S. Singh, A. K. Verma and G. Palit, Bioorg. Med. Chem. Lett., 2011, 21, 1937 CrossRef PubMed.
-
(a) D. A. Ostrov, J. A. Hernandez-Prada, P. E. Corsino, K. A. Finton, N. Le and T. C. Rowe, Antimicrob. Agents Chemother., 2007, 51, 3688 CrossRef;
(b) F. Chimenti, B. Bizzarri, A. Bolasco, D. Secci, P. Chimenti, A. Granese, S. Carradori, D. Rivanera, A. Zicari, M. M. Scaltrito and F. Sisto, Bioorg. Med. Chem. Lett., 2010, 20, 4922 CrossRef PubMed.
- T. A. De Almeida Barros, L. A. R. De Freitas, J. M. B. Filho, X. P. Nunes, A. M. Giulietti, G. E. De Souza, R. R. Dos Santos, M. B. P. Soares and C. F. Villarreal, J. Pharm. Pharmacol., 2010, 62, 205 CrossRef PubMed.
-
(a) A. Lacy and R. O'Kennedy, Curr. Pharm. Des., 2004, 10, 3797 CrossRef PubMed;
(b) M. A. Musa, J. S. Cooperwood and M. O. F. Khan, Curr. Med. Chem., 2008, 15, 2664 CrossRef;
(c) L. R. Zacharski, W. G. Henderson, F. R. Rickles, W. B. Forman, C. J. Cornell Jr, R. J. Forcier, R. L. Edwards, E. Headley, S. H. Kim, J. F. O'Donnell, R. O'Dell, K. Tornyos and H. C. Kwaan, Cancer, 1984, 53, 2046 CrossRef.
- F. Borges, F. Roleira, N. Milhazes, L. Santana and E. Uriarte, Curr. Med. Chem., 2005, 12, 887 CrossRef PubMed.
- A. Lacy and R. O'Kennedy, Curr. Pharm. Des., 2004, 10, 3797 CrossRef PubMed.
-
(a) A. Ahmad and L. N. Misra, Int. J. Pharmacogn., 1997, 35, 121 CrossRef CAS;
(b) O. R. Gottlieb, K. Herrmann, R. D. H. Murray, G. Ohloff and G. Pattenden, Progress in the Chemistry of Organic Natural Products, New York, Springer-Verlag, 1978 Search PubMed.
- L. L. Skowronski, O. Krupka, V. Smokal, A. Grabowski, M. Naparty and B. Derkowska-Zielinska, Opt. Mater., 2015, 47, 18 CrossRef CAS.
-
(a) M. Tasior, D. Kim, S. Singha, M. Krzeszewski, K. Han Ahn and D. T. Gryko, J. Mater. Chem. C, 2015, 3, 1421 RSC;
(b) G. Jones II and M. A. Rahman, J. Phys. Chem., 1994, 98, 13028 CrossRef.
- K. Hara, T. Sato, R. Katoh, A. Furube, Y. Ohga, A. Shinpo, S. Suga, K. Sayama, H. Sugihara and H. Arakawa, J. Phys. Chem. B, 2003, 107(2), 597 CrossRef.
-
(a) T. Watanabe, S. Yuki, M. Egawa and H. Nishi, J. Pharmacol. Exp. Ther., 1994, 268, 1597 Search PubMed;
(b) H. Kawai, H. Nakai, M. Suga, S. Yuki, T. Watanabe and K. I. Saito, J. Pharmacol. Exp. Ther., 1997, 281, 921 Search PubMed;
(c) T. W. Wu, L. H. Zeng, J. Wu and K. P. Fung, Life Sci., 2002, 71, 2249 CrossRef.
- F. A. Pasha, M. Muddassar, M. M. Neaz and S. J. Cho, J. Mol. Graphics Modell., 2009, 28, 54–61 CrossRef.
- P. Gunasekaran, S. Perumal, P. Yogeeswari and D. Sriram, Eur. J. Med. Chem., 2011, 46, 4530 CrossRef.
- E. A. M. Badawey and I. M. El-Ashmawey, Eur. J. Med. Chem., 1998, 33, 349 CrossRef.
- M. A. Al-Haiza, S. A. El-Assiery and G. H. Sayed, Acta Pharm., 2001, 51, 251 Search PubMed.
- F. Moreau, N. Desroy, J. M. Genevard, V. Vongsouthi, V. Gerusz, G. Le Fralliec, C. Oliveira, S. Floquet, A. Denis, S. Escaich, K. Wolf, M. Busemann and A. Aschenbsenner, Bioorg. Med. Chem. Lett., 2008, 18, 4022 CrossRef.
- D. Castagnolo, F. Manetti, M. Radi, B. Bechi, M. Pagano, A. De Logu, R. Meleddu, M. Saddi and M. Botta, Bioorg. Med. Chem., 2009, 17, 5716 CrossRef.
- P. M. S. Chauhan, S. Singh and R. K. Chatterjee, Indian J. Chem., Sect. B: Org. Chem. Incl. Med. Chem., 1993, 32, 858 Search PubMed.
- D. M. Bailey, P. E. Hansen, A. G. Hlavac, E. R. Baizman, J. Pearl, A. F. Defelice and M. E. Feigenson, J. Med. Chem., 1985, 28, 256 CrossRef.
- P. P. Ghosh, G. Pal, S. Paul and A. R. Das, Green Chem., 2012, 14, 2691 RSC.
- Z. Shao, L. Xu, L. Wang, H. Wei and J. Xiao, Org. Biomol. Chem., 2014, 12, 2185 RSC.
- J. J. Jennings, C. P. Bhatt and A. K. Franz, J. Org. Chem., 2016, 81, 6211 CrossRef PubMed.
- S. Yang, G. M. Reddy, M. Liu, T. Wang, J. Yu and W. Lin, J. Org. Chem., 2017, 82, 781 CrossRef.
- F. Han, S. Xun, L. Jia, Y. Zhang, L. Zou and X. Hu, Org. Lett., 2019, 21, 5907 CrossRef.
- M. Bakthadoss, D. Kannana and R. Selvakumar, Chem. Commun., 2013, 49, 10947 RSC.
-
(a) K. D. Dwivedi, B. Bora and L. R. Chowhan, Front. Chem., 2020, 7, 944 CrossRef;
(b) K. D. Dwivedi, M. S. Reddy, N. S. Kumar and L. R. Chowhan, ChemistrySelect, 2019, 4, 8602 CrossRef;
(c) L. R. Chowhan, M. S. Reddy and N. S. Kumar, J. Chem. Sci., 2017, 129, 1205 CrossRef;
(d) C. J. Clarke, W. Tu, O. Levers, A. BröhlJason and P. Hallett, Chem. Rev., 2018, 118, 747 CrossRef.
-
(a) P. Ramesh, M. S. Reddy, N. S. Kumar, B. Rathod, R. S. Prakasham and L. R. Chowhan, ChemistrySelect, 2018, 3, 9096 CrossRef;
(b) M. S. Reddy, L. R Chowhan, N. S. Kumar, P. Ramesh and S. B. Mukkamala, Tetrahedron Lett., 2018, 59, 1366 CrossRef;
(c) L. R. Chowhan and S. Raghavan, Tetrahedron Lett., 2019, 60, 151132 CrossRef;
(d) V. Singh, S. R. Lakshmi and L. R. Chowhan, ChemistrySelect, 2019, 4, 13601 CrossRef.
- Y. Wei, K. Miao and S. Hao, Molecules, 2018, 23, 122 CrossRef.
- M. Chen, D. Lu and X. Zhang, Chem. Pap., 2017, 71, 1579 CrossRef CAS.
- T. Nasr, S. Bondock, H. M. Rashed, W. Fayad, M. Youns and T. M. Sakr, Eur. J. Med. Chem., 2018, 151, 723 CrossRef CAS.
-
(a) Q. Wu, Z. Liu, D. Cao, R. Guan, K. Wang, Y. Shan, Y. Xu and L. Ma, Mater. Chem. Phys., 2015, 161, 43 CrossRef CAS;
(b) Z. Wang, Q. Wu, J. Li, S. Qiu, D. Cao, Y. Xu, Z. Liu, X. Yu and Y. Sun, Spectrochim. Acta, Part A, 2017, 183, 1 CrossRef CAS;
(c) S. Sumiya, Y. Shiraishi and T. Hirai, J. Phys. Chem. A, 2013, 117, 1474 CrossRef CAS.
-
(a) F. Chimenti, D. Secci, A. Bolasco, P. Chimenti, B. Bizzarri, A. Granese, S. Carradori, M. Yáñez, F. Orallo and F. Ortuso, J. Med. Chem., 2009, 52, 1935 CrossRef CAS;
(b) C. Binda, J. Wang, L. Pisani, C. Caccia, A. Carotti, P. Salvati, D. E. Edmondson and A. Mattevi, J. Med. Chem., 2007, 50, 5848 CrossRef CAS;
(c) A. Stefanachi, F. Leonetti, L. Pisani, M. Catto and A. Carotti, Molecules, 2018, 23, 250 CrossRef.
- D. N. Sawant, D. B. Bagal, S. Ogawa, K. Selvam and S. Saito, Org. Lett., 2018, 20(15), 4397 CrossRef CAS.
- D. C. Braddock, P. D. Lickiss, B. C. Rowley, D. Pugh, T. Purnomo, G. Santhakumar and S. J. Fussell, Org. Lett., 2018, 20, 950 CrossRef CAS.
-
(a) T. M. E. Dine, W. Erb, Y. Berhault, J. Rouden and J. Blanchet, J. Org. Chem., 2015, 80, 4532 CrossRef;
(b) N. Gernigon, R. M. Al-Zoubi and D. G. Hall, J. Org. Chem., 2012, 77, 8386 CrossRef CAS.
- A. Orliac, D. G. Pardo, A. Bombrun and J. Cossy, Org. Lett., 2013, 15, 902 CrossRef CAS PubMed.
- M. S. Reddy, N. S. Kumar and L. R. Chowhan, RSC Adv., 2018, 8, 35587–35593 RSC.
Footnote |
† Electronic supplementary information (ESI) available. See DOI: 10.1039/d0ra01906b |
|
This journal is © The Royal Society of Chemistry 2020 |