DOI:
10.1039/D0RA01415J
(Paper)
RSC Adv., 2020,
10, 12800-12809
Retracted Article: Preventive effect of lemon seed flavonoids on carbon tetrachloride-induced liver injury in mice
Received
13th February 2020
, Accepted 18th March 2020
First published on 31st March 2020
Abstract
The aim of this study was to determine the preventive effect of lemon seed flavonoids (LSF) on carbon tetrachloride-induced liver injury in mice. Liver injury was induced by injection with 2 mL kg−1 of carbon tetrachloride after administration of LSF by gavage. Liver index, serological parameters, and expression intensities of related mRNA and protein in the liver tissue were observed. The results indicated that LSF reduced liver weight and liver index, downregulated serum levels of AST, ALT, ALP, TG, TC, BUN, NO, and MDA, and upregulated levels of ALB, SOD, CAT, and GSH-Px in the mice with liver injury. It also downregulated serum cytokines, such as IL-6, IL-12, TNF-α, and IFN-γ in these mice. qPCR and western blot confirmed that LSF upregulated mRNA and protein expression of Mn-SOD, Cu/Zn-SOD, CAT, GSH-Px, and IκB-α, and downregulated expression of NF-κB-p65, iNOS, COX-2, TNF-α, IL-1β, and IL-6 in the liver tissue of mice with liver injury. The preventive effect on carbon tetrachloride-induced liver injury was attributed to (−)-epigallocatechin, caffeic acid, (−)-epicatechin, vitexin, quercetin, and hesperidin, which were active substances that were detected in LSF by HPLC. Moreover, the effect of LSF is similar to that of silymarin, but the synergistic effect of the five active substances working in concert acted to produce a more robust liver-protecting effect.
1 Introduction
Lemon (Citrus limon (L.) Burm. F.) belongs to the Citrus plants of the family Rutaceae. During the industrial processing of lemons, lemon seeds are the main by-product, containing abundant oil, flavonoids, and limonoids, which may cause environmental pollution and resource waste, if carelessly discarded. Currently, there are limited studies on lemon seeds, focusing only on the extraction of oil from the seeds, and there are few studies on the biological activity and its components.1,2 Many types of flavonoids can be extracted from natural plants, most of which possess biological activity. Modern molecular biological methods have been used to prove that the flavonoids extracted from plants have certain anti-oxidant, anti-hypertensive, anti-inflammatory, and anti-cancer effects.3 One animal experiment showed that kaempferol, as a flavonoid, has an inhibitory effect on oxidative stress-induced bladder injury in rats.4 At the same time, the research shows that the Trichilia emetica leaf is rich in flavonoids, which has the in vivo effect of treating type 2 diabetes by target therapy.5 It has also been shown that a polyoxyflavone from citrus, nobiletin, shows anti-inflammatory and anti angiogenic activities in zebrafish in vivo.6 Natural polyphenol flavonoid also has anticancer effect, quercetin can inhibit the establishment of tumor in nude mice by subcutaneously inoculating HepG2 cells, and its anticancer effect can be achieved by regulating the expression of cyclin D1 in cancer cells.7 Lemon, as a kind of fruit, is rich in flavonoids. In addition, it also contains flavanones and flavonols. There are about 20 kinds of flavonoids in lemon juice and pericarp, among which the higher contents are hesperidin, naringin, neohesperidin, quercetin, naringin and citrinin.8 However, the analysis of the components of lemon seeds is lack of relevant research.
The liver is an important metabolic physiological organ, and liver injury will cause severe harm to the human body. Chemical injury to the liver is a common occurrence, and it can occur after consumption of large amounts of alcohol. Drugs and toxic chemical substances in the environment may cause liver injury, and severe chemical liver injury may even result in liver cirrhosis and liver cancer.9 Plant flavonoids have a strong protective effect against experimental liver injury.10–12 Moreover, most plant flavonoids have a strong anti-oxidation effect, as well as anti-liver fibrosis effects, which can reduce the proliferation of hepatic stellate cells (HSCs) and collagen synthesis or inhibit the activation of HSCs to prevent liver fibrosis.13 Carbon tetrachloride is a common chemical that induces liver injury in laboratory animals. During the induction process of liver injury, carbon tetrachloride will cause hepatocytes to secrete large amounts of inflammatory factors that aggravate inflammation and cause liver injury. Moreover, carbon tetrachloride can promote the production of Cl− and CCl3− in liver microsomes, which causes lipid peroxidation of liver microsomes and the cell membrane, destruction of the cell membrane, and liver injury.14
In this study, based on the effect of carbon tetrachloride on the liver, a chemical liver injury model in mice was established, and the preventive effect of LSF on carbon tetrachloride-induced liver injury mice were observed. Furthermore, the molecular biological method was used to measure the serum and liver-related indices, and subsequently elucidate the preventive mechanism of LSF on liver injury in mice. The results will be beneficial for the development and utilization of LSF, and for accumulating a theoretical basis for the use of lemon seed in food processing and research and development of health products.
2 Materials and methods
2.1 Extraction of LSF
Dried lemon seeds (Chongqing Huida Lemon Processing Group Co., Ltd, Chongqing, China) were ground in a mortar and sieved to discard the coarse granules to obtain lemon seed powder. The 300 g powder was degreased with n-hexane by ultrasound for 90 min (degreasing temperature, 50 °C; ratio of material to liquid, 1
:
30; power, 200 W), and then dried by suction filtration. Flavonoids were extracted from the degreased lemon seed powder with 3 L of 95% ethanol by ultrasound for 60 min at 50 °C and power 200 W. The sample solution was obtained by suction filtration.
2.2 Induction of liver injury in mice by carbon tetrachloride
Six old male Kunming mice (body weight: 22 ± 3 g, Chengdu Oulin Biotechnology Co., Ltd, Chengdu, Sichuan, China) were randomly assigned into 5 groups (n = 10): normal group, model group, silymarin group (positive model group), low-dose LSF group (50 mg kg−1), and high-dose LSF group (100 mg kg−1). After acclimation for 1 week, 2 mL normal saline was administered every day by gavage to the mice in the normal group and the model group. Those in the silymarin intragastric group were given 0.2 mL silymarin solution at a dose of 100 mg per kg per day. LSF was administered to the mice in the low-dose (LLSF) and high-dose (HLSF) groups at doses of 50 mg per kg and 100 mg kg per day, respectively, by gavage, for 2 weeks. On the 14th day, all mice except the normal group were injected with carbon tetrachloride as an inducer of liver damage (2 mL kg; CCl4
:
olive oil = 1
:
1, v/v). Then, all mice were fasted, but free water was allowed. After fasting for 24 h, the mice were euthanized by cervical dislocation, followed by obtaining plasma from the heart and dissecting the liver. The liver index was calculated by the formula: liver index = liver weight (g)/body weight of mice (g) × 100.15 All animal procedures were performed in accordance with the Guidelines for Care and Use of Laboratory Animals of Third Military Medical University and approved by the Animal Ethics Committee of Animal Experimental Center of Third Military Medical University.
2.3 Measurement of serological parameters in mice
Plasma was separated by centrifugation at 4000 rpm for 10 min. Serum levels of aspartate transaminase (AST), alanine aminotransferase (ALT), alkaline phosphatase (ALP), triglyceride (TG), total cholesterol (TC), blood urea nitrogen (BUN), albumin (ALB), superoxide dismutase (SOD), nitric oxide (NO), catalase (CAT), malondialdehyde (MDA), and glutathione peroxidase (GSH-Px) were measured using kits (Nanjing Jiancheng Bioengineering Institute, Nanjing, Jiangsu, China).
2.4 Measurement of serum cytokines in mice
Serum levels of cytokines, such as interleukin (IL)-6, IL-12, tumor necrosis factor (TNF)-α, and interferon (IFN)-γ were measured using kits (Nanjing Jiancheng Bioengineering Institute).
2.5 Observation of liver sections
Pieces of 0.5 cm2 liver tissue were fixed in 10% formalin solution for 48 h, dehydrated, cleared, waxed, embedded, sectioned, and stained with hematoxylin and eosin (H&E). Morphological changes of the tissue were observed under an optical microscope (BX43 optical microscope, Olympus, Tokyo, Japan).
2.6 Measurement of mRNA expression in the liver by qPCR
The liver tissue was homogenized, from which total RNA was extracted with TRIzol™ reagent (Thermo Fisher Scientific, Inc., Waltham, MA, USA). After the total RNA concentration was diluted to 1 μg μL−1, 1 μL of the solution was used for reverse transcription to obtain a cDNA template. Then, 1 μL cDNA template was reacted with 10 μL SYBR Green PCR Master Mix (Thermo Fisher Scientific), 1 μL each of upstream and downstream primers (Table 1), and 7 μL sterile distilled water at 95 °C for 60 s, followed by 40 cycles of 95 °C, 15 s; 55 °C, 30 s; 72 °C, 35 s; and finally, measured at 95 °C, 30 s; 55 °C, 35 s (Stepone Plus, Thermo Fisher Scientific), using GAPDH as an internal reference. The relative expression was calculated by 2-ΔΔCT.16
Table 1 Sequences of primers used in the qPCR assay
Gene name |
Sequence |
Cu/Zn-SOD |
Forward: 5′-AACCAGTTGTGTTGTCAGGAC-3′ |
Reverse: 5′-CCACCATGTTTCTTAGAGTGAGG-3′ |
Mn-SOD |
Forward: 5′-CAGACCTGCCTTACGACTATGG-3′ |
Reverse: 5′-CTCGGTGGCGTTGAGATTGTT-3′ |
CAT |
Forward: 5′-GGAGGCGGGAACCCAATAG-3′ |
Reverse: 5′-GTGTGCCATCTCGTCAGTGAA-3′ |
GSH-Px |
Forward: 5′-CAGTCGGTGTATGCCTTCTCG-3′ |
Reverse: 5′-GAGGGACGCCACATTCTCG-3′ |
NF-κB p65 |
Forward: 5′-GAGGCACGAGGCTCCTTTTCT-3′ |
Reverse: 5′-GTAGCTGCATGGAGACTCGAACA-3′ |
IκB-α |
Forward: 5′-CGCGGGATGGCCTCAAGAAGGA-3′ |
Reverse: 5′-GCCAAGTGCAGGAACGAGTCT-3′ |
iNOS |
Forward: 5′-GTTCTCAGCCCAACAATACAAGA-3′ |
Reverse: 5′-GTGGACGGGTCGATGTCAC-3′ |
COX-2 |
Forward: 5′-CTGGCGCTCAGCCATACAG-3′ |
Reverse: 5′-CGCACTTATACTGGTCAAATCCC-3′ |
TNF-α |
Forward: 5′-GCCTCCCTCTCATCAGTTCTA-3′ |
Reverse: 5′-GGCAGCCTTGTCCCTTG-3′ |
IL-1β |
Forward: 5′-CTCCATGAGCTTTGTACAAGG-3′ |
Reverse: 5′-TGCTGATGTACCAGTTGGGG-3′ |
IL-6 |
Forward: 5′-TCTATACCACTTCACAAGTCGGA-3′ |
Reverse: 5′-GAATTGCCATTGCACAACTCTTT-3′ |
GAPDH |
Forward: 5′-AGGTCGGTGTGAACGGATTTG-3′ |
Reverse: 5′-GGGGTCGTTGATGGCAACA-3′ |
2.7 Western blot
To prepare the liver for western blotting, 100 mg of liver tissue samples was homogenized with 1 mL of radioimmunoprecipitation assay (RIPA, Thermo Fisher Scientific) buffer and 10 μL of phenylmethylsulfonyl fluoride (PMSF, Thermo Fisher Scientific) at 12
000 rpm, then lysed at 4 °C for 5 min, and centrifuged at 12
000 rpm and 4 °C for 15 min. Protein in the middle layer was measured with a bicinchoninic acid (BCA, Thermo Fisher Scientific) kit. Each sample was diluted to 50 μg mL−1, heated at 100 °C for 5 min with sample buffer at a ratio of 4
:
1, and then cooled on ice for 5 min. Mixing acrylamide, resolving buffer, stacking buffer, distilled water, 10% ammonium persulfate (APS) and N,N,N,N-tetramethylethylenediamine (TEMED, Thermo Fisher Scientific) were added at a given ratio to prepare a sodium dodecyl sulfate-polyacrylamide gel electrophoresis (SDS-PAGE) running gel and stacking gel (Thermo Fisher Scientific). A prestained protein ladder and samples were injected into the gel wells, followed by SDS-PAGE vertical gel electrophoresis for 50 min. A polyvinylidene difluoride (PVDF) membrane was activated with methanol for 1 min, and then sealed for 1 h with 1× Tris-buffered saline (TBS) containing 0.1% Tween-20 (TBST) and 5% skimmed milk (Thermo Fisher Scientific). After sealing, the PVDF membrane was cleaned with 1× TBST, and incubated with primary antibody (Thermo Fisher Scientific) at 25 °C for 2 h. After cleaning 5 times with 1× TBST, the membrane was incubated with secondary antibody (Thermo Fisher Scientific) at 25 °C for 1 h. Finally, the PVDF membrane was sprayed with Supersignal West Pico PLUS and then placed in an iBright FL1000 imaging system for observation.17
2.8 Measurement of LSF components
(−)-Epigallocatechin, caffeic acid, (−)-epicatechin, vitexin, quercetin, and hesperidin were prepared into standard solutions at a concentration of 1 mg mL−1. The LSF solution at the same concentration was detected by high-performance liquid chromatography: column: Accucore C18 column (4.6 mm × 150 mm, 2.6 μm); mobile phase A: 0.5% glacial acetic acid; phase B: acetonitrile; flow rate: 0.5 mL min−1; column temperature: 30 °C; detection wavelength: 285 nm; and injection volume: 5 μL (Ultimate 3000 high performance liquid chromatography, Thermo Fisher Scientific).
2.9 Data analysis
All experiments were repeated three times to obtain the average value of parallel results. SAS 9.1 statistical software was used with the one-way ANOVA method to analyze the significant difference between groups at P < 0.05.
3 Results
3.1 Effect of LSF on the liver index of mice
As shown in Table 2, there was no significant difference in body weight between groups. The liver weight of the model group was significantly higher than that of the other groups (P < 0.05), and that of the normal group was the lowest. Through LSF treatment by gavage, the liver weight of the mice with liver injury decreased due to carbon tetrachloride, and it decreased more in those treated with high-dose LSF. The changes in the liver index were similar to those in liver weight. The liver index of the model group was the highest. With increasing LSF dose, the liver index of the mice in the 100 mg kg−1 LSF (HLSF) group was slightly higher than that of those who received with same dose of silymarin.
Table 2 The liver index of mice with treatment of CCl4 (N = 10)a
Group |
Body weight (g) |
Live weight (g) |
Liver index |
Values presented are the mean ± standard deviation. a–d Mean values with different letters in the same column are significantly different (P < 0.05) according to Duncan's new MRT. Silymarin group: 100 mg per kg per b.w. silymarin treatment dose; LLSF group: 50 mg kg per b.w. lemon seed flavonoids dose; HLSF group: 100 mg per kg per b.w. lemon seed flavonoids dose. |
Normal |
31.14 ± 1.07a |
1.23 ± 0.06d |
3.97 ± 0.27d |
Model |
31.22 ± 0.72a |
2.77 ± 0.07a |
8.87 ± 0.42a |
Silymarin |
31.53 ± 0.76a |
1.73 ± 0.10c |
5.48 ± 0.43c |
LLSF |
31.20 ± 0.52a |
2.32 ± 0.06b |
7.43 ± 0.08b |
HLSF |
31.10 ± 0.83a |
1.85 ± 0.06c |
5.96 ± 0.17c |
3.2 Effects of LSF on serum AST, ALT, ALP, TG, TC, BUN, and ALB
As shown in Table 3, the levels of AST, ALT, ALP, TG, TC, and BUN were the lowest, and ALB was the highest in the normal group. However, the model group of CCl4-induced liver injury shows an opposite trend: the highest levels of AST, ALT, ALP, TG, TC, and BUN, and the lowest level of ALB. Silymarin and LSF by gavage significantly downregulated the levels of AST, ALT, ALP, TG, TC, and BUN, and upregulated the level of ALB in the mice with liver injury. Silymarin treatment resulted in these indices being closest to those of the normal group, while high-dose LSF (HLSF) resulted in indices close to those of mice treated with silymarin at same dose.
Table 3 AST, ALT, ALP, TG, TC, BUN and ALB serum levels of mice with treatment of CCl4 (N = 10)a
Group |
ALT (U L−1) |
AST (U L−1) |
ALP (K-A) |
TG (mmol L−1) |
TC (mmol L−1) |
BUN (mg dL−1) |
ALB (g dL−1) |
Values presented are the mean ± standard deviation. a–e Mean values with different letters in the same column are significantly different (P < 0.05) according to Duncan's new MRT. Silymarin group: 100 mg per kg per b.w. silymarin treatment dose; LLSF group: 50 mg per kg per b.w. lemon seed flavonoids dose; HLSF group: 100 mg per kg per b.w. lemon seed flavonoids dose. |
Normal |
19.48 ± 0.59e |
10.27 ± 0.86e |
5.35 ± 0.10e |
0.34 ± 0.04e |
1.36 ± 0.10e |
17.93 ± 0.41e |
4.35 ± 0.16a |
Model |
78.25 ± 2.57a |
71.26 ± 2.00a |
22.03 ± 1.15a |
2.80 ± 0.07a |
6.30 ± 0.24a |
61.17 ± 1.82a |
1.35 ± 0.09e |
Silymarin |
26.83 ± 1.22d |
17.63 ± 0.84d |
10.91 ± 1.11d |
0.45 ± 0.04d |
1.79 ± 0.15d |
30.72 ± 1.49d |
3.66 ± 0.10b |
LLSF |
66.81 ± 1.42b |
52.64 ± 1.60b |
18.44 ± 0.40b |
1.95 ± 0.08b |
5.08 ± 0.12b |
46.18 ± 1.85b |
1.96 ± 0.08d |
HLSF |
30.99 ± 0.38c |
28.77 ± 2.01c |
14.46 ± 0.26c |
0.67 ± 0.04c |
2.52 ± 0.14c |
35.01 ± 0.60c |
3.25 ± 0.09c |
3.3 Effects of LSF on serum SOD, NO, CAT, MDA, and GSH-Px
In Table 4, carbon tetrachloride reduced the serum levels of SOD, CAT, GSH-Px, and increased the serum levels of NO and MDA. Both silymarin and LSF inhibited these changes, and increased inhibition was observed for silymarin at 100 mg kg−1 (HLSF). The effect of LSF at the same dose was similar to that of silymarin (positive control).
Table 4 SOD, NO, CAT, MDA and GSH-Px serum levels of mice with treatment of CCl4 (N = 10)a
Group |
SOD (U mL−1) |
NO (μmol L−1) |
CAT (U mL−1) |
MDA (μmol L−1) |
GSH-Px (U mL−1) |
Values presented are the mean ± standard deviation. a–e Mean values with different letters in the same column are significantly different (P < 0.05) according to Duncan's new MRT. Silymarin group: 100 mg per kg per b.w. silymarin treatment dose; LLSF group: 50 mg per kg b.w. lemon seed flavonoids dose; HLSF group: 100 mg per kg per b.w. lemon seed flavonoids dose. |
Normal |
136.18 ± 3.76a |
72.80 ± 2.74e |
38.03 ± 2.12a |
5.74 ± 0.17e |
272.80 ± 7.89a |
Model |
71.08 ± 6.72e |
120.78 ± 7.07a |
11.10 ± 0.75e |
17.57 ± 1.05a |
71.95 ± 1.49e |
Silymarin |
119.59 ± 2.27b |
87.42 ± 1.84d |
28.02 ± 1.81b |
8.58 ± 0.30d |
208.23 ± 4.22b |
LLSF |
91.28 ± 2.33d |
102.66 ± 3.63b |
17.54 ± 1.28d |
12.87 ± 0.94b |
114.86 ± 8.31d |
HLSF |
105.61 ± 3.24c |
94.96 ± 3.73c |
24.98 ± 0.79c |
10.01 ± 0.31c |
174.69 ± 4.64c |
3.4 Effects of LSF on serum IL-6, IL-12, TNF-α, and IFN-γ
As shown in Table 5, the serum levels of IL-6, IL-12, TNF-α, and IFN-γ in the model group were significantly higher than those in other groups (P < 0.05). Silymarin and LSF significantly reduced the serum levels of IL-6, IL-12, TNF-α, and IFN-γ, compared with those of the model mice. The most significant effect was observed for silymarin, with the serum levels of cytokines being closer to those in normal mice. LSF also reduced the levels of cytokines, and high-dose LSF at 100 mg kg−1 (HLSF) had a stronger effect.
Table 5 IL-6, IL-12, TNF-α, and IFN-γ serum cytokine levels of mice with treatment of CCl4 (N = 10)a
Group |
IL-6 (pg mL−1) |
IL-12 (pg mL−1) |
TNF-α (pg mL−1) |
IFN-γ (pg mL−1) |
Values presented are the mean ± standard deviation. a–e Mean values with different letters in the same column are significantly different (P < 0.05) according to Duncan's new MRT. Silymarin group: 100 mg per kg per b.w. silymarin treatment dose; LLSF group: 50 mg per kg per b.w. lemon seed flavonoids dose; HLSF group: 100 mg per kg per b.w. lemon seed flavonoids dose. |
Normal |
44.29 ± 3.58e |
208.20 ± 9.15e |
26.22 ± 0.76e |
19.81 ± 0.87e |
Model |
232.37 ± 4.78a |
782.41 ± 12.16a |
92.40 ± 1.60a |
79.65 ± 1.01a |
Silymarin |
71.73 ± 1.94d |
394.65 ± 6.15d |
42.44 ± 1.09d |
30.13 ± 1.29d |
LLSF |
173.30 ± 5.92b |
640.15 ± 21.22b |
75.43 ± 1.22b |
61.61 ± 2.82b |
HLSF |
77.74 ± 3.21c |
471.55 ± 18.02c |
55.79 ± 0.91c |
37.84 ± 3.03c |
3.5 Effect of LSF on liver histopathology
As illustrated in Fig. 1, the central hepatocytes in the liver of the normal group were radially distributed with the central vein as a center, and the cell structure was normal. The central vein of the liver of the model group was irregular, the cells were disorderedly arranged with a damaged cell structure, and a large number of necrotic cells appeared. Silymarin and LSF reduced the necrosis of hepatocytes and the incomplete morphology of the liver caused by CCl4, with a more pronounced effect with increasing doses of LSF.
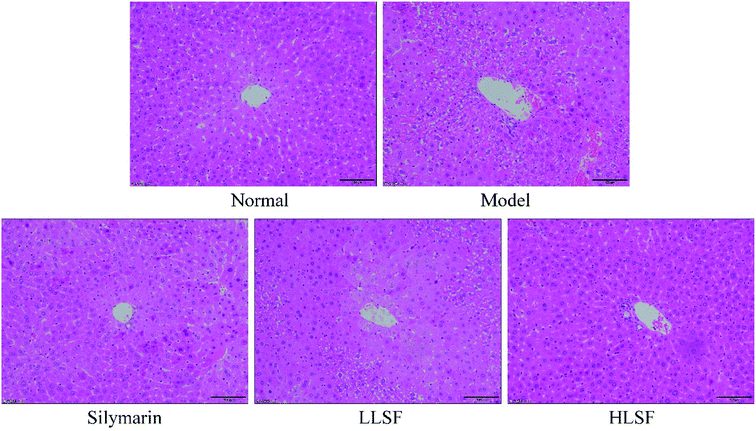 |
| Fig. 1 H&E histopathology observation of liver in mice with treatment of CCl4. Silymarin group: 100 mg per kg per b.w. silymarin treatment dose; LLSF group: 50 mg per kg per b.w. lemon seed flavonoids dose; HLSF group: 100 mg per kg per b.w. lemon seed flavonoids dose. | |
3.6 Effects of LSF on mRNA and protein expression of Mn-SOD, Gu/Zn-SOD, CAT, and GSH-Px in the liver
Fig. 2 illustrates that the mRNA and protein expression of Mn-SOD, Gu/Zn-SOD, CAT, and GSH-Px in the liver of the normal mice was the highest, but that in the model group was the lowest. Silymarin by gavage significantly upregulated the expression of Mn-SOD, Gu/Zn-SOD, CAT, and GSH-Px in the livers of mice with liver injury. The same effects were observed for LSF, but the effects of high-dose LSF (HLSF) were more significant. Semi-quantitative calculations demonstrated that the effects of silymarin and LSF at a dose of 100 mg kg−1 (HLSF) were more significant without significant difference (P > 0.05).
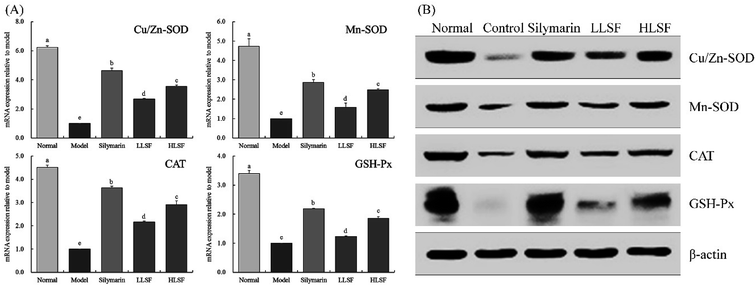 |
| Fig. 2 mRNA (A) and protein (B) expression of Mn-SOD, Gu/Zn-SOD, CAT, and GSH-Px in liver tissue of mice with treatment of CCl4. Values presented are the mean ± standard deviation. a–e Mean values with different letters in the same column are significantly different (P < 0.05) according to Duncan's new MRT. Silymarin group: 100 mg per kg per b.w. silymarin treatment dose; LLSF group: 50 mg per kg per b.w. lemon seed flavonoids dose; HLSF group: 100 mg per kg per b.w. lemon seed flavonoids dose. | |
3.7 Effects of LSF on mRNA and protein expression of NF-κB-p65, IκB-α, iNOS, and COX-2 in the liver
As illustrated in Fig. 3, after CCl4-induced liver injury, the expression of NF-κB-p65, inducible nitric oxide synthase (iNOS), and COX-2 in the model group was significantly higher than that in other groups, but that of IκB-α was significantly lower than that in the other groups (P < 0.05). The expression intensities of NF-κB-p65, IκB-α, iNOS, and COX-2 in the normal group were opposite to those in the model group. Silymarin and LSF downregulated the expression of NF-κB-p65, iNOS, and COX-2 and upregulated the expression of IκB-α. Moreover, the expression of NF-κB-p65, iNOS, COX-2, and IκB-α that was upregulated by high-dose LSF (HLSF) was similar to that of the positive model group (silymarin group) and normal group.
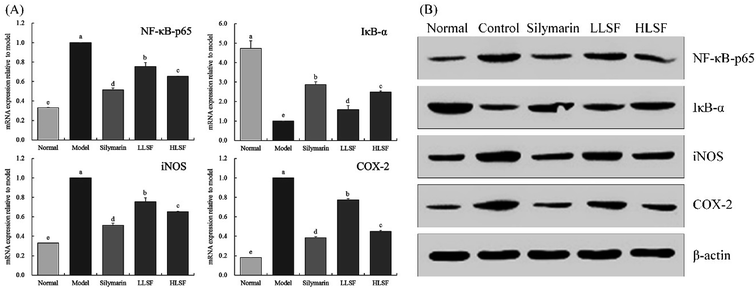 |
| Fig. 3 mRNA (A) and protein (B) expression of NF-κB-p65, IκB-α, iNOS, and COX-2 in liver tissue of mice with treatment of CCl4. Values presented are the mean ± standard deviation. a–e Mean values with different letters in the same column are significantly different (P < 0.05) according to Duncan's new MRT. Silymarin group: 100 mg per kg per b.w. silymarin treatment dose; LLSF group: 50 mg per kg per b.w. lemon seed flavonoids dose; HLSF group: 100 mg per kg per b.w. lemon seed flavonoids dose. | |
3.8 Effect of LSF on the mRNA expression of TNF-α, IL-1β, and IL-6 in the liver
As shown in Fig. 4, the mRNA expression of TNF-α, IL-1β, and IL-6 in the liver of normal mice was the lowest. Carbon tetrachloride enhanced the expression of TNF-α, IL-1β, and IL-6 in the liver, but LSF inhibited the enhanced expression. Additionally, the effect of LSF was slightly weaker than that of silymarin at the same dose.
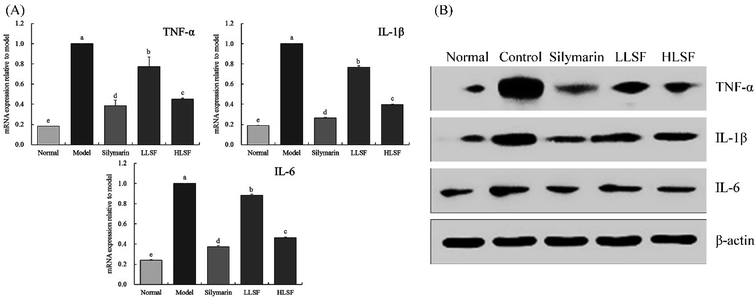 |
| Fig. 4 mRNA (A) and protein (B) expression of TNF-α, IL-1β, and IL-6 in liver tissue of mice with treatment of CCl4. Values presented are the mean ± standard deviation. a–e Mean values with different letters in the same column are significantly different (P < 0.05) according to Duncan's new MRT. Silymarin group: 100 mg per kg per b.w. silymarin treatment dose; LLSF group: 50 mg per kg per b.w. lemon seed flavonoids dose; HLSF group: 100 mg per kg per b.w. lemon seed flavonoids dose. | |
3.9 Components of LSF
As shown in Fig. 5, LSF mainly contains (−)-epigallocatechin, caffeic acid, (−)-epicatechin, vitexin, quercetin, and hesperidin, with retention times of 13.167 min, 20.517 min, 21.413 min, 28.043 min, 31.100 min, and 34.610 min, respectively.
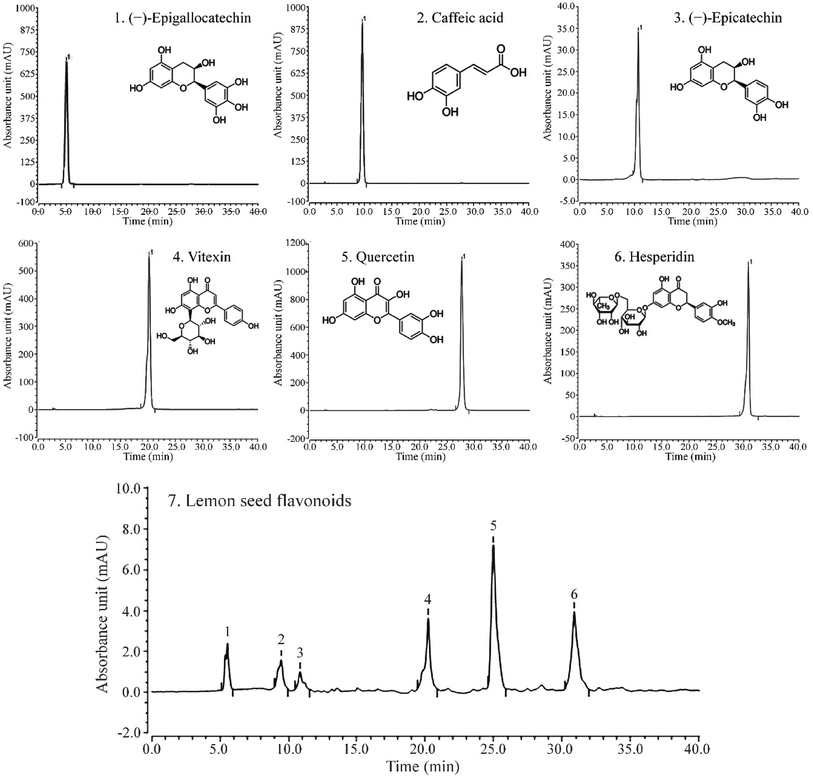 |
| Fig. 5 Liquid chromatogram of (A) standard products and (B) lemon seed flavonoids. (1) (−)-Epigallocatechin, (2) caffeic acid, (3) (−)-epicatechin, (4) vitexin, (5) quercetin, (6) hesperidin. | |
4 Discussion
The liver is the largest substantial organ in the abdominal cavity. Liver injury not only threatens the health of the body, but also endangers lives in serious cases. Liver weight and liver index have been used to evaluate the degree of carbon tetrachloride-induced liver injury. High liver weight and liver index are one of the manifestations of liver injury, which is consistent with our study.18 In the current study, LSF reduced the liver weight and liver index of liver-injured mice with an effect similar to that of silymarin, a drug used for treatment of liver injury.
AST mainly exists in the plasma and mitochondria of hepatocytes, and ALT mainly in the plasma of hepatocytes. Necrosis of hepatocytes will lead to an increase in ALT and AST in the body.19 Carbon tetrachloride causes lipid peroxidation in the liver, which changes the membrane permeability of hepatocytes, and the ALP level in hepatocytes sharply increases.20 Liver injury can result in fatty acid transfer to the liver, leading to elevated TG content in the liver. Additionally, TG and TC also indicate the amount of lipid peroxidation in the liver.21 Liver injury will further damage renal function, increase the blood content of BUN, as well as affect synthesis, transportation, and release of ALB in the body, thus decreasing the blood content of ALB.22 The current study also proved that LSF regulates AST, ALT, ALP, TG, TC, BUN, and ALB so that the indices of the mice with liver injury were normalized, thus preventing liver injury.
Carbon tetrachloride will cause oxidation in the body. The body uses non-enzymatic and enzymatic methods to defend itself against oxidative damage. Regulation of the elevation of SOD, CAT, and GSH-Px is the main mechanism of enzymatic antioxidant activity in the body.23 SOD is capable of catalyzing superoxide free radicals and scavenging free radicals. The synergistic effect of CAT and SOD strengthens the function of scavenging free radicals.24 CAT is an important antioxidant enzyme in the body, and scavenges H2O2 in the body, thus inhibiting oxidative stress, reducing the oxidation in the body caused by carbon tetrachloride, and inhibiting liver injury.25 GSH-Px is an important enzyme for catalyzing the decomposition of H2O2. Catalyzing the reduction of glutathione to H2O2 can protect the cell membrane and prevent cell damage.26 Malondialdehyde (MDA) is a metabolite of lipid peroxidation, and greatly accumulates in the body after liver injury.27 The increase in NO and its oxidation products leads to injury of phospholipid and protein on the cell surface, promoting inflammatory exudation and injury of the tissue.28 NO also reacts with superoxide anion to form peroxynitrite anion ONOO–, aggravating the oxidative stress reaction, and leading to cytotoxicity and aggravation of liver injury.29 LSF can protect the liver and prevent liver injury by reducing the oxidative damage indicated by the serological parameters of mice with liver injury.
Carbon tetrachloride can cause oxidation in the body and inflammation in the liver, which is reflected by the large increase in serum IL-6, IL-12, TNF-α, and IFN-γ in response to exposure to the chemical.30 IL-6 is a factor secreted by Th2 cells that participates in the humoral immune response. When the level of Th2 increases, functional impairment in viscera may occur.31 IL-6 promotes the differentiation and proliferation of T lymphocytes and production of antibodies, changes the viability of G cells, upregulates the function of neutrophils, and enhances the inflammatory response of the body.32 IL-12 is an activator of natural killer (NK) cells with the strongest effect. When liver injury occurs, excessive apoptosis and immune response of hepatocytes will aggravate the injury, which is related to the enhancement of the killing function of CD8+ T cells by IL-12.33 The binding of TNF-α with TNF-αR1 on the hepatocyte membrane causes transformation of double-stranded genomic DNA into oligodeoxynucleotide fragments, promoting apoptosis of stem cells. TNF-α also aggravates the inflammatory expression in the body by activating NF-κB, further aggravating liver injury.34 IFN-γ, a proinflammatory cytokine, enhances the sensitivity of hepatocytes to TNF-α and increases the vulnerability of hepatocytes to injury.35 The oxidative stress status after liver injury causes an imbalance in inflammatory factors, and increases the amounts of TNF-α, IL-1β, and IL-6 in the liver.36 The current study has proved that LSF also prevents liver injury by reducing the inflammatory response induced by liver injury.
Mn-SOD and Gu/Zn-SOD are isomers of SOD in the body. Mn-SOD is a radical scavenger with Mn4+ as an active center in mitochondria. Gu/Zn-SOD is another scavenger with Cu2+ and Zn2+ as the active center in the cytoplasm.37 The liver and the heart are the organs with the most abundant mitochondria. After CCl4 induces liver injury, the activity of Mn-SOD significantly declines, which is consistent with the results from our study.38 Gu/Zn-SOD scavenges the toxic effect caused by O2−˙ in the body, and protects the visceral tissues.39 Carbon tetrachloride has been proved to cause oxidative stress in the body and produce large amounts of free radicals. Mn-SOD and Gu/Zn-SOD inhibit the free radicals in the body to prevent liver injury40 and we observed this in the current study. The regulation that LSF performed on the oxidation-related expression in the liver indicated its degree of relief of oxidative stress-induced liver injury, so as to protect the liver.
NF-κB is a key factor regulating the inflammatory response, with impacts on inflammation-related cytokines, including IL-6 and TNF-α, and is strongly expressed in the inflammatory response. Generally, NF-κB and IκB-α exist in binding states with no activity, and separation is induced by external inflammation. IκB-α binds activated NF-κB to inhibit inflammation.41 NO is a highly active oxidant, generated by activated NOS in hepatocytes. It can promote the high gene expression of iNOS after liver injury develops to the decompensated stage.42 When liver injury occurs, large amounts of inflammatory factors are released due to oxidative stress in hepatocytes. iNOS is an important inflammatory factor, and NO induced by iNOS also promotes liver injury.43 COX-2 is another important inflammatory factor, but it is not expressed in normal tissues. Kupffer cells are activated, and large amounts of COX-2 expression and synthesis occur after hepatocyte injury, which aggravates the inflammatory injury of the liver.44,45 Our experiments confirmed that LSF prevented liver injury by regulating inflammatory expression in the liver.
(−)-Epigallocatechin, caffeic acid, (−)-epicatechin, vitexin, quercetin, and hesperidin are all compounds with a better anti-oxidation effect, endowing LSF with certain anti-inflammatory, anti-cancer, and other biological activities.46–51 The combination of these six substances produced a better synergistic antioxidant effect and played an inhibitory role in the CCl4-induced liver injury.
5 Conclusions
LSF is a flavonoid mixture, and its active substances exert a preventive effect on CCl4-induced liver injury in mice. LSF can inhibit the CCl4-induced abnormalities in serological parameters, including liver functional indices, and return oxidation and inflammation indices to normal. LSF has been also proved to regulate mRNA and protein expression in mice with liver injury, and ameliorate the expression of inflammation and oxidation in the liver so that parameters are similar to those of normal mice. Moreover, because the effect of LSF is similar to that of silymarin, this active substance is worthy of further development and utilization.
Abbreviations
LSF | Lemon seed flavonoids |
AST | Aminotransferase |
ALT | Alanine aminotransferase |
ALP | Alkaline phosphatase |
TG | Triglyceride |
TC | Total cholesterol |
BUN | Blood urea nitrogen |
NO | Nitric oxide |
MDA | Malondialdehyde |
ALB | Albumin |
SOD | Superoxide dismutas |
CAT | Catalase |
GSH-Px | Glutathione peroxidase |
IL-6 | Interleukin 6 |
IL-1β | Interleukin-1 beta |
TNF-α | Tumor necrosis factor alpha |
IFN-γ | Interferon gamma |
Mn-SOD | Manganese superoxide dismutase |
Cu/Zn-SOD | Cuprozinc-superoxide dismutase |
IκB-α | Nuclear factor of kappa light polypeptide gene enhancer in B-cell inhibitor alpha |
NF-κB-p65 | Nuclear factor κ-light-chain-enhancer of activated B-cells |
iNOS | Inducible nitric oxide synthase |
COX-2 | Cyclooxygenase-2 |
qPCR | Quantitative polymerase chain reaction |
HPLC | High performance liquid chromatography |
Conflicts of interest
No conflicts of interest in this article.
References
- M. Klimek-Szczykutowicz, A. Szopa and H. Ekiert, Plants, 2020, 9, E119 CrossRef PubMed.
- L. Liu, Q. L. Dong and M. X. Cui, China Oils Fats, 2007, 32, 57–59 CAS.
- K. Murota, Y. Nakamura and M. Uehara, Biosci., Biotechnol., Biochem., 2018, 82, 600–610 CrossRef CAS PubMed.
- Y. B. Huang, M. W. Lin, Y. Chao, C. T. Huang, Y. H. Tsai and P. C. Wu, Int. J. Urol., 2014, 21, 94–98 CrossRef CAS PubMed.
- K. Konaté, K. Yomalan, O. Sytar, P. Zerbo, M. Brestic, V. D. Patrick, P. Gagniuc and N. Barro, J. Evidence-Based Complementary Altern. Med., 2014, 2014, 867075 Search PubMed.
- K. H. Lam, D. Alex, I. K. Lam, S. K. Tsui, Z. F. Yang and S. M. Lee, J. Cell. Biochem., 2011, 112, 3313–3321 CrossRef CAS PubMed.
- J. Zhou, L. Fang, J. Liao, L. Li, W. Yao, Z. Xiong and X. Zhou, PLoS One, 2017, 12, e0172838 CrossRef PubMed.
- Y. H. Yu and B. N. Jiao, Sci. Technol. Food Ind., 2011, 2011, 518–523 Search PubMed.
- R. Teschke, Biomedicines, 2019, 7, 68 CrossRef CAS PubMed.
- C. Niu, M. Ma, X. Han, Z. Wang and H. Li, Acta Cir. Bras., 2017, 32, 33–640 CrossRef PubMed.
- K. Zhu, G. Huang, J. Xie, X. Zhou, J. Mu and X. Zhao, Food Sci. Nutr., 2019, 7, 3808–3818 CrossRef CAS PubMed.
- Y. Li, L. Tong, J. Zhang, Y. Zhang and F. Zhang, Cell. Physiol. Biochem., 2018, 51, 1354–1363 CrossRef CAS PubMed.
- W. Chen, Z. Zhang, Z. Yao, L. Wang, F. Zhang, J. Shao, A. Chen and S. Zheng, Int. Immunopharmacol., 2018, 56, 148–155 CrossRef CAS PubMed.
- Y. S. Jin, S. I. Heo, M. J. Lee, H. I. Rhee and M. H. Wang, Free Radical Res., 2005, 39, 1351–1358 CrossRef CAS PubMed.
- X. Chen, J. Zhang, R. Yi, J. Mu, X. Zhao and Z. Yang, Int. J. Mol. Sci., 2018, 19, E2212 CrossRef PubMed.
- F. Li, G. Huang, F. Tan, R. Yi, X. Zhou, J. Mu and X. Zhao, Food Sci. Nutr., 2019, 8, 379–389 CrossRef PubMed.
- K. Zhu, X. Zeng, F. Tan, W. Li, C. Li, Y. Song and X. Zhao, Food Sci. Nutr., 2019, 7, 4105–4115 CrossRef CAS PubMed.
- M. Wang, J. Niu, L. Ou, B. Deng, Y. Wang and S. Li, Molecules, 2019, 24, 1964 CrossRef CAS PubMed.
- E. E. J. Iweala, W. O. Evbakhavbokun and E. N. Maduagwu, Sci. Pharm., 2019, 87, 24 CrossRef CAS.
- G. Albasher, R. Almeer, F. O. Al-Otibi, N. Al-Kubaisi and A. M. Mahmoud, Biomolecules, 2019, 9, 261 CrossRef CAS PubMed.
- Y. Yan, W. Wu, L. Lu, J. Ren, J. Mi, X. Liu and Y. Cao, Food Sci. Nutr., 2019, 7, 3435–3442 CrossRef CAS PubMed.
- B. B. Tang and D. H. Hu, J. Hainan Med. Univ., 2016, 22, 124–127 Search PubMed.
- R. Farbiszewski, A. Radecka, M. Chwiecko and A. Holownia, Alcohol, 1992, 9, 403–407 CrossRef CAS PubMed.
- Q. H. Huang, L. Q. Xu, Y. H. Liu, J. Z. Wu, X. Wu, X. P. Lai, Y. C. Li, Z. R. Su, J. N. Chen and Y. L. Xie, J. Evidence-Based Complementary Altern. Med., 2017, 2017, 7953850 Search PubMed.
- Y. G. Li, D. F. Ji, S. Chen and G. Y. Hu, Alcohol Alcohol., 2008, 43, 246–253 CrossRef CAS PubMed.
- X. Wang, M. Liu, C. Zhang, S. Li, Q. Yang, J. Zhang, Z. Gong, J. Han and L. Jia, Molecules, 2018, 23, 481 CrossRef PubMed.
- X. Sun, P. Wang, L. P. Yao, W. Wang, Y. M. Gao, J. Zhang and Y. J. Fu, Environ. Toxicol. Pharmacol., 2018, 60, 110–117 CrossRef CAS PubMed.
- G. E. Arteel, Gastroenterology, 2003, 124, 778–790 CrossRef CAS PubMed.
- L. An, X. Wang and A. I. Cederbaum, Arch. Toxicol., 2012, 86, 1337–1338 CrossRef CAS PubMed.
- C. Eipel, J. Hardenberg, S. Negendank, K. Abshagen and B. Vollmar, Eur. J. Gastroenterol. Hepatol., 2009, 21, 923–931 CrossRef CAS PubMed.
- L. Cheng, J. Wang, X. Li, Q. Xing, P. Du, L. Su and S. Wang, PLoS One, 2011, 6, e17631 CrossRef CAS PubMed.
- L. Cheng, X. Du, Z. Wang, J. Ju, M. Jia, Q. Huang, Q. Xing, M. Xu, Y. Tan, M. Liu, P. Du, L. Su and S. Wang, J. Hepatol., 2014, 61, 1297–1303 CrossRef CAS PubMed.
- H. C. Zhou, H. Wang, K. Shi, J. M. Li, Y. Zong and R. Du, Molecules, 2018, 24, 131 CrossRef PubMed.
- B. Liu, Y. Fang, R. Yi and X. Zhao, Foods, 2019, 8, 48 CrossRef CAS PubMed.
- J. Y. Qiao, H. W. Li, F. G. Liu, Y. C. Li, S. Tian, L. H. Cao, K. Hu, X. X. Wu and M. S. Miao, Molecules, 2019, 24, 2887 CrossRef CAS PubMed.
- C. C. Lai, P. H. Huang, A. H. Yang, S. C. Chiang, C. Y. Tang, K. W. Tseng and C. H. Huang, Am. J. Chin. Med., 2016, 44, 531–550 CrossRef CAS PubMed.
- X. Lu, C. Wang and B. Liu, Fish Shellfish Immunol., 2015, 42, 58–65 CrossRef CAS PubMed.
- S. Kanai and H. Okano, Am. J. Chin. Med., 1998, 26, 333–341 CrossRef CAS PubMed.
- B. Liu, J. Li, R. Yi, J. Mu, X. Zhou and X. Zhao, Foods, 2019, 8, 36 CrossRef CAS PubMed.
- M. D. Wheeler, M. Nakagami, B. U. Bradford, T. Uesugi, R. P. Mason, H. D. Connor, A. Dikalova, M. Kadiiska and R. G. Thurman, J. Biol. Chem., 2001, 276, 36664–36672 CrossRef CAS PubMed.
- M. R. Rusciano, E. Sommariva, V. Douin-Echinard, M. Ciccarelli, P. Poggio and A. S. Maione, Int. J. Mol. Sci., 2019, 20, 4374 CrossRef CAS PubMed.
- C. Brenner, L. Galluzzi, O. Kepp and G. Kroemer, J. Hepatol., 2013, 59, 583–594 CrossRef CAS PubMed.
- O. Lisakovska, I. Shymanskyy, A. Mazanova, A. Khomenko and M. Veliky, Biochem. Cell Biol., 2017, 95, 213–222 CrossRef CAS PubMed.
- M. Wang, J. Niu, L. Ou, B. Deng, Y. Wang and S. Li, Molecules, 2019, 24, E1964 CrossRef PubMed.
- J. Dong, W. Li, L. M. Cheng and G. G. Wang, Int. J. Clin. Exp. Pathol., 2019, 12, 817–825 CAS.
- N. Yamanaka, O. Oda and S. Nagao, FEBS Lett., 1997, 401, 230–234 CrossRef CAS PubMed.
- H. G. Choi, P. T. Tran, J. H. Lee, B. S. Min and J. A. Kim, Arch. Pharmacal Res., 2018, 41, 64–70 CrossRef CAS PubMed.
- I. Ramirez-Sanchez, C. Mansour, V. Navarrete-Yañez, M. Ayala-Hernandez, G. Guevara, C. Castillo, M. Loredo, M. Bustamante, G. Ceballos and F. J. Villarreal, Food Funct., 2018, 9, 4802–4813 RSC.
- S. Inamdar, A. Joshi, S. Malik, R. Boppana and S. Ghaskadbi, Biochem. Biophys. Res. Commun., 2019, 519, 106–112 CrossRef CAS PubMed.
- D. A. Diamantis, S. Ramesova, C. M. Chatzigiannis, I. Degano, P. S. Gerogianni, K. E. Karadima, S. Perikleous, D. Rekkas, I. P. Gerothanassis, D. Galaris, T. Mavromoustakos, G. Valsami, R. Sokolova and A. G. Tzakos, Biochim. Biophys. Acta, Gen. Subj., 2018, 1862, 1913–1924 CrossRef CAS PubMed.
- S. Tejada, S. Pinya, M. Martorell, X. Capó, J. A. Tur, A. Pons and A. Sureda, Curr. Med. Chem., 2018, 25(37), 4929–4945 CrossRef CAS PubMed.
|
This journal is © The Royal Society of Chemistry 2020 |
Click here to see how this site uses Cookies. View our privacy policy here.