DOI:
10.1039/D0RA01237H
(Paper)
RSC Adv., 2020,
10, 13929-13935
Synthesis of 1-(β-coumarinyl)-1-(β-indolyl)trifluoroethanols through regioselective Friedel–Crafts alkylation of indoles with β-(trifluoroacetyl)coumarins catalyzed by Sc(OTf)3†
Received
8th February 2020
, Accepted 20th March 2020
First published on 15th April 2020
Abstract
A highly efficient Friedel–Crafts alkylation of indole derivatives with β-(trifluoroacetyl)coumarins using Sc(OTf)3 as a catalyst has been developed, which gives regioselective 1,2-adducts to afford 1-(β-coumarinyl)-1-(β-indolyl)trifluoroethanols. A series of tertiary trifluoroethanols containing different indole and coumarin groups were synthesized in moderate to excellent yields (up to 95%) in the presence of 5 mol% catalyst in a short time (only 2 minutes at least). A mechanism of the reaction, in which the trace amount of water plays the role of proton transfer in catalyzing circulation was proposed and confirmed.
Introduction
In comparison with their non-fluorinated parent compounds, fluorinated organic compounds often show unique and important physical, chemical, and biological properties due to the strong electron-withdrawing properties, low polarizability and small size of the fluorine atom, and the strength of the C–F bond. Over the last decades, such compounds have been used to great effect in the pharmaceutical and agrochemical industries.1 Currently, about 20–30% of agrochemicals and pharmaceuticals owe their effectiveness to the presence of one or more fluorine atoms in their structures.2 In 2018, the U.S. Food and Drug Administration (FDA) approved 38 small molecule drugs and 18 of them contained fluorine.3 Among the fluorinated groups, the trifluoromethyl motif is of particular interest and this unit is widely used in the quest for new bioactive molecules, as illustrated by the recent marketed molecules.2a,b Consequently, a lot of effort has been made to develop an efficient reaction for the synthesis of CF3-containing compounds over the last decades.4 For example, α,β-unsaturated trifluoromethyl ketones, possessing a directly linking electron-withdrawing trifluoromethyl group with a highly reactive π-system, have been used in numerous transformations, including hydrogenation,5 aldol,6 epoxidation,7 Michael-type8 and Diels–Alder reactions9 to construct trifluoromethylated organic compounds.
On the other hand, the Friedel–Crafts (F–C) alkylation of indoles is of interest because the indole nucleus is widely present in numerous natural products in biological and pharmaceutically compounds.10 Among them, the conjugate addition of indoles as nucleophiles to α,β-unsaturated carbonyl compounds is very efficient and convenient. In most cases, the addition of indole to α,β-unsaturated ketones yields the corresponding β-indolylketones, including protic acid or Lewis acid-mediated reactions.11 Until now, most of the available Friedel–Crafts reactions of indoles with α,β-unsaturated carbonyl compounds were limited to 1,4-addition, which can also be considered as a Michael addition of α,β-unsaturated carbonyl compounds with indoles. These α,β-unsaturated carbonyl compounds contains methyl vinyl ketone, acrylic acid, 2-cyclohexen-1-one, ethyl propiolate,12 α,β-unsaturated aldehydes,12,13 chalcones,12,14 α,β-unsaturated trifluoromethyl ketones,8b β-trifluoromethyl-α,β-enones,15 β,γ-unsaturated α-keto esters,16 α,β-unsaturated 2-acyl imidazoles,17 methyl 2-acetamidoacrylate,18 intramolecular α,β-unsaturated carbonyl compounds19 and so on. To the best of our knowledge, the Friedel–Crafts 1,2-addition of indole with α,β-unsaturated carbonyl compounds to afford an α,β-unsaturated alcohol has not been reported yet, although a Friedel–Crafts alkylation of pyrrole with β,γ-unsaturated α-keto esters was developed to afford the β,γ-unsaturated α-hydroxy esters.20
Coumarin-based derivatives also show a wide range of valuable biological activities in medicinal and pharmaceutical areas,21 such as antimicrobial,22 anti-inflammatory,23 antitubercular,24 anti-osteoporotic,25 antidiabetic,26 enzyme inhibitory,27 anticancer,28 and antioxidant23b,29 properties.
Various fluoro-substituted coumarins have been recently reported.30 We recently developed an efficient method to prepare β-(trifluoromethyl)coumarins (1), being a particular α,β-unsaturated carbonyl system and containing diverse functionality, by direct transformation of ethyl 2-hydroxy-2-trifluoromethyl-2H-chromene-3-carboxylates under microwave assisted solvent-free conditions.31 Various trifluoromethyl coumarin thiosemicarbazones and hydrozones were synthesized from ketones 1 and showed excellent antifungal activities.32 Considering the above reports, and as part of our program aimed at developing new methodologies for the preparation of coumarin derivatives with biological activities, we planned to explore the reaction of compound 1 with indoles to prepare novel coumarin derivatives with trifluoromethyl group and indole scaffold.
Here, we report the first synthesis of 1-(β-coumarinyl)-1-(β-indolyl)trifluoroethanols through an addition of β-(trifluoroacetyl) coumarin 1 using Sc(OTf)3 as a catalyst. Different from our previous work,32 which was a 1,4-addition, this work is a regioselective synthesis through 1,2- addition (Scheme 1).
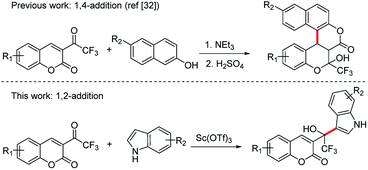 |
| Scheme 1 1,4-Addition and 1,2-addition of β-(trifluoroacetyl)coumarins. | |
Results and discussion
To examine this proposed addition, we started to explore the practicability of this transformation, and chose the reaction of β-(trifluoroacetyl)coumarin 1a with indole 2a as the model (Table 1. For more details, see Table S1 in ESI†).
Table 1 Optimization of reaction conditionsa
Based on similar previous studies, which showed that Lewis acid or protic acid could promote the conjugation addition of indole to α,β-unsaturated ketones, the reaction was initially carried out in the presence of different Lewis acids or protic acids in CH2Cl2 (dichloromethane) at 25 °C for 2 hours. Lewis acids, containing AlCl3, FeCl3, Pb(OAc)2, Cu(OTf)2, Fe(OTf)3, Y(OTf)3, and protic acids, containing p-toluenesulfonic acid (p-TSA), trifluoromethanesulfonyl acid (TfOH) were previously studied as catalysts. However, only a trace or a small amount of adduct 3aa was obtained (entries 2–5, Table 1 or entries 2–9, Table S1 in ESI†). To our delight, Sc(OTf)3 could catalyze the carbonyl addition quite effectively and gave 3aa in 93% yield (entry 6, Table 1). The yield increased to 95% when the reaction time was extended to 5 hours, and only decreased a little when the reaction time was reduced to 30 min. By screening solvents, we found that moderate polar solvents such as CH2Cl2 and CHCl3, weak and nonpolar solvents like toluene, 1,2-dichloroethane and CCl4, worked better than polar solvents (CH3CN, EtOH and HOAc) for the reaction, and CH2Cl2 produced the best satisfactory results (entries 6–13, Table 1). Apart from the above-mentioned factors, the effects of reaction temperature, the amount of catalyst, the ratio of the reactants and the moisture were also investigated (entries 14–20, Table 1). The optimal reaction conditions were determined to be under reflux (45 °C) for 20 min in air atmosphere, with addition of 5% Sc(OTf)3 as catalyst, CH2Cl2 as solvent and 1
:
1 of the ratio 1a
:
2a (entry 14, Table 1).
With the promising results obtained in the model reaction, we subsequently examined the substrate scope of the transformation. The results of 27 examples are summarized in Table 2. The reactions were monitored by HPLC. The reaction time and corresponding isolated yields were shown in the Table 2.
Table 2 Sc(OTf)3-catalyzed addition of indoles 2a–h with 1a–fa
All tested substituted indoles 2a–h reacted with β-(trifluoromethyl) coumarins 1a–f and gave the 1-(β-coumarinyl)-1-(β-indolyl)-trifluoroethanols 3aa–3fa in high to excellent isolated yields (72% to 95%, Table 2). Of note, all the reactions proceeded smoothly and completed in less than 2 hours, and the conditions were tolerant to different substituents. The electronic nature of aromatic ring of coumarins has little influence on the yield of the reactions (3ba, 3ca, 3da, 3ea and 3fa).
The indole ring, which was connected with an electron-donating group (such as methyl or methoxyl), was advantageous to reaction rate (3ab, 3ac, 3ae, 3af and 3eb, 3ec, 3ee, 3ef). The addition was completed in 2 minutes at least. However, in the case of 4- benzyloxyindole (2d), the reaction time was longer than 4-methyloxyindole (2c), such as 3ac and 3ad, 3bc and 3bd, 3cc and 3cd, 3dc and 3dd, 3ec and 3ed, which was perhaps due to the steric effect of benzyloxyl. When an electron-withdrawing group was attached to the indole ring, such as 5-chloroindole (2g) or 6-bromoindole (2h), the reaction became slower obviously (3ag, 3ah and 3eh).
It was similar to coumarins' phenyl ring. Especially, when 8-methoxyl-3-(trifluoroacetyl)coumarin (1e) reacted with 4-benzyloxyindole (2d), the reaction time was reduced from 50 to 16 minutes in contrast to the reaction of 1a with 2d. On the contrary, when coumarins' phenyl ring was connected with an electron-withdrawing group, such as a chlorine or bromine atom (1b and 1c), the reactions all slowed down (3ba, 3bc, 3bd and 3ca, 3cd, 3ce).
When benzo[f]coumarin (1f) was used instead, the reaction rate became higher than that of 1a, because the larger conjugate system increased electron cloud density of coumarin.
The structures of compound 3aa–3fa were confirmed by 1H NMR, 13C NMR and HRMS spectroscopy. All 1H and 13C NMR spectra used d6-DMSO as a solvent to avoid the interference of peaks from residual non-deuterium solvent. A 1H–13C COSY spectrum of 3aa and 1H NMR spectrum of 3aa exchanged by D2O were also given as additional information to confirm the ownership of every signal in 1H NMR and 13C NMR. The 1H–13C COSY spectrum showed that there were only two hydrogens which did not attach to a carbon. In the 1H NMR spectrum of 3aa, these two signals were observed at δ 11.30 ppm and 7.34 ppm which belonged to the indole NH and tertiary alcohol OH respectively, and the integral areas decreased from 1.00 to 0.69 and 0.18 after D2O exchanged for 2 hours (See Fig. S3 in ESI†). Ten aromatic protons appeared in the range of δ 6.87–8.69 ppm. In the 13C NMR spectrum of 3aa, the lactone carbonyl carbon appeared at δ 157.46 ppm, while the carbon atom attached to the oxygen in the coumarin ring was observed at δ 153.55 ppm. The carbon of CF3 and the carbon attached to the hydroxyl and CF3 with their corresponding coupling constants J C,F = 287.9 Hz and 30.0 Hz, appeared as a quartet at δ 125.47 and 74.49 ppm, respectively. Compared with spectrum of 1a and 2a and fitted by ChemDraw software, the ownerships of other signals of 3aa in 1H NMR and 13C NMR are shown in the 1H–13C COSY spectrum (See Fig. S7 in ESI†). In the 19F NMR spectrum of 3aa, only a singlet was observed at δ −74.21 ppm which belongs to the three fluorine atoms of CF3. Compounds 3ab–3fa showed the similar signals in 1H and 13C NMR. Compounds 3aa–3fa all showed the molecular-ion peak [M − H]+ or [M + H]+in the high resolution mass spectrum, matching with the calculated data.
The structures of coumarin derivatives were further confirmed by the X-ray diffraction determination of single crystals of compounds 3aa and 3dd (single crystal X-ray diffraction data of compounds 3aa and 3dd are deposited with CCDC No. 1950379 and 1950380, respectively). The perspective and packing views are shown in Fig. 1 and 2 respectively. The crystal data and refinement details are given in Tables S2–S15 in ESI.† It is seen that compounds 3aa and 3dd are isomorphous, and they crystallize in the monoclinic space P21/c and C2/c respectively with four molecules in the unit cell.
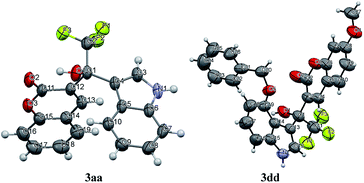 |
| Fig. 1 X-ray structures of products 3aa and 3dd. | |
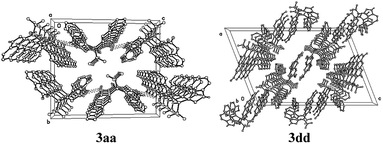 |
| Fig. 2 View of the molecular packings in 3aa and 3dd. | |
In the crystal structure of 3dd, we can see that the C–O bond between C20 and O5 could not rotate freely because of the steric effect of coumarin group. In 1H NMR spectrum of 3dd, the two benzyl hydrogens showed two signals at δ 5.04 and 4.93 ppm, which means they are magnetic nonequivalent. Each hydrogen shows a doublet with a coupling constant J = 12.9 Hz from each other. Similar signals were found in 1H NMR spectrum of other products of 2d, such as 3ad, 3bd, 3cd and 3ed.
The reaction was 1,2-addition instead of 1,4-addition which was confirmed by the crystal structures of the adducts of the Friedel–Crafts alkylation of indoles 2 with β-(trifluoroacetyl)coumarins 1 catalyzed by Sc(OTf)3. We attributed the main reason to the steric effect in the intermediate. Here, we compares the addition of chalcone which has a similar phenyl α,β-unsaturated ketone with 1a (see Scheme 2). In the carbocation formed by 1a in the present of Sc(OTf)3, the carbon next to the trifluoromethyl was more positive than the 4-carbon of the coumarin ring, which was confirmed by the charge computation of density functional theory (DFT). However, only the electronic property was not enough and it still tended to give the 1,4-adduct through Michael-type addition (see examples for 1,4-addition of α,β-unsaturated trifluoromethyl ketones with indoles in ref. 8b). We supposed that in the 1,4-adduct B formed by chalcone and indole 2a, the C–C bond between the phenyl and new tertiary carbon could rotate freely. By contrast, in the 1,4-adduct C from 1a and indole 2a, the C–C bond between the phenyl and new tertiary carbon could not rotate at all, because of the rigid structure of coumarin ring. Thus the indolyl and coumarinyl groups were very crowd in the 1,4-adduct C which was a minor adduct. In fact, only 1,2-adduct 3aa was observed in this reaction.
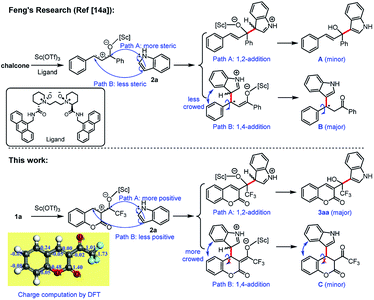 |
| Scheme 2 Comparison between additions of chalcone and 1a with indole 2a. | |
According to the result of above analysis, a proposed mechanism of 1,2-addition of 1a and 2a is shown in Scheme 3. It should be noted that a little water in the solution played the role of transmitting protons, and the reaction could be completed in a very short time (2–120 minutes). The water might come from the solvent which was used without any treatment, and β-(trifluoroacetyl)coumarins 1 which has a very electron-deficient carbonyl and can exist as a hydrate easily (see the crystal structure of hydrate of 1a in ref. 31b or CCDC 967230). Through the DFT computation, Yu et al. discovered that a trace amount of water could play a role of proton transfer in catalyzing circulation.33 By contrast, a reaction of 1a and 2a with Sc(OTf)3, which were dried under vacuum at room temperature in a desiccator for 2 hours to remove moisture, in CH2Cl2 distilled over phosphorus pentoxide was carried on (see entries 33–35, Table S1†). With less water, the reaction slowed down evidently. After 10 minutes, the yield was only 20%. Even after 1 hour, only 61% of product was obtained. Without any treatment, the reaction could be completed in 20 minutes with a 95% yield.
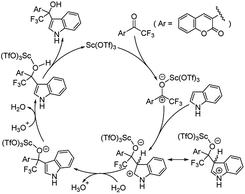 |
| Scheme 3 Proposed mechanism of addition of 1a and 2a. | |
Conclusions
In conclusion, we have shown that Friedel–Crafts alkylation of indole derivatives with kinds of β-(trifluoroacetyl)coumarins can be effectively catalyzed by Sc(OTf)3. The reaction proceeds with good yields (up to 95%) in a short time (only 2–120 minutes) and gives 1,2-adducts to afford 1-(β-coumarinyl)-1-(β-indolyl)trifluoroethanols. The conditions are of application to a large number of substituted indoles and β-(trifluoroacetyl)coumarins.
Experimental
General
All solvents and reagents used are commercially available and were used without further purification unless noted. All 1H and 13C NMR spectra used d6-DMSO as a solvent to avoid the interference of peaks from residual non-deuterium solvent. The NMR data were obtained on a Bruker DPX-400 or 500 Spectrometer, respectively. The MestReNova Software was used to deal with the NMR spectra. Chemical shifts (δ) are reported in ppm and J values are given in hertz. In 1H NMR, the signal of TMS was set as 0.00 ppm unless noted. In 13C NMR, the middle signal of d6-DMSO was set as 39.60 ppm. All the signals represent 1H or 1C except as noted. HPLC analyses for the qualitative and quantitative analysis of the products were carried out using an Agilent 1200 pump equipped with an Agilent 1200 detector. Melting points were determined on an X-5 digital microscopic melting-point apparatus (Beijing Tech Instruments Co., Beijing, China) and are uncorrected. High resolution mass spectrometry were obtained using a Waters Q-Tof MicroTM instrument. X-ray crystallography parameters for data collection and refinement of the compounds are summarized in Table S1 in ESI.† Intensities were collected on a Rigaku Saturn 724 CCD diffractometer (Mo-Kα, λ = 0.71073 Å) at a temperature of 293 K using the SMART and SAINT programs. The structures were solved by direct method and refined on F2 by full-matrix least-squares methods with SHELXTL-97 crystallographic software package. All the non-hydrogen atoms were refined with anisotropic thermal displacement coefficients. The hydrogen atoms were assigned with common isotropic displacement factors and included in the final refinement by using geometrical restrains.
Preparation of β-(trifluoroacetyl)coumarins 1a–f
β-(Trifluoroacetyl)coumarins 1a–f were prepared according the microwave assisted solvent-free route via Knoevenagel condensation of substituted salicylaldehydes with ethyl trifluoroacetoacetate in the presence of silica-immobilized L-proline and subsequently rearrangement (see ref. 31).
Indole 2a and substituted indoles 2b–h were used commercially.
Preparation of 1-(β-coumarinyl)-1-(β-indolyl)trifluoroethan-ols 3aa–3fa
In a typical experiment of Friedel–Crafts alkylation of indoles, a solution of β-(trifluoroacetyl)coumarin 1a (0.2 mmol), indole 2a (0.2 mmol) and Sc(OTf)3 (0.01 mmol, 5% equiv.) in 2 mL CH2Cl2 was stirred under atmosphere at 45 °C for 20 minutes. The reaction was monitored by HPLC. When the reaction completed, the mixture was washed by water (5 mL × 3). Then the water phase was extracted by CH2Cl2 (5 mL × 3). The combined solution was evaporated under reduced pressure. The crude product was recrystallized from ethyl acetate and petroleum ether (1
:
10), to afford the product 3aa as a light yellow powder (95% yield).
Characterization data of new compounds
Representative example 3aa is given below; for other compounds (3ab–3fa), see the ESI.†
1-(Coumarin-3-yl)-1-(1H-indol-3-yl)-2,2,2-trifluoroethanol (3aa). Yield 95%, light yellow powder, mp 244.6–244.9 °C. 1H NMR (400 MHz) δ 11.30 (s, NH), 8.69 (s), 7.99 (d, J = 7.4 Hz), 7.64 (t, J = 7.6 Hz), 7.48 (s), 7.44–7.34 (m, 5H), 7.07 (t, J = 7.5 Hz), 6.89 (t, J = 7.5 Hz). 13C NMR (101 MHz) δ 157.46(C
O), 153.55(C–O), 143.05, 136.30, 132.73, 129.68, 125.47 (q, J = 287.9 Hz, CF3), 125.39, 125.34, 124.80, 124.37, 121.28, 119.65, 119.12, 118.42, 115.87, 111.91, 110.40, 74.49 (q, J = 30.0 Hz, C–CF3). 19F NMR (376 MHz) δ −74.21 (s, 3F, CF3). HRMS: m/z calcd for C19H11F3NO3: 358.0691 [M − H]+; found: 358.0689.
Conflicts of interest
There are no conflicts of interest to declare.
Acknowledgements
This work was supported by the Science and Technology Agency of Henan Province (152102110070, 192102110055) and Guidance Program for Key Scientific Research Items of Higher Education of Henan Province (19B210004, 20B150008).
Notes and references
- For reviews, see:
(a) R. Filler and Y. Kobayashi, Biomedicinal Aspects of Fluorine Chemistry, Elsevier, Amsterdam, 1982 Search PubMed;
(b) J. T. Welch and S. Eswarakrishman, Fluorine in Bioorganic Chemistry, Wiley, New York, 1991 Search PubMed;
(c) A. Becker, Inventory of Industrial Fluoro-Biochemicals, Eyrolles, Paris, 1996 Search PubMed;
(d) J.-P. Begue and D. Bonnet-Delpon, Bioorganic and Medicinal Chemistry of Fluorine, Wiley, Hoboken, 2008 CrossRef;
(e) S. Purser, P. R. Moore, S. Swallowb and V. Gouverneur, Chem. Soc. Rev., 2008, 37, 320–330 RSC;
(f) I. Ojima, Fluorine in Medicinal Chemistry and Chemical Biology, Wiley, Chichester, 2009 CrossRef;
(g) S. Swallow, Fluorine in Medicinal Chemistry (Chapter 2), Progress in Medicinal Chemistry, Elsevier, 2015, vol. 54, pp. 65−133 Search PubMed.
- For reviews, see:
(a) J. Wang, M. Sánchez-Roselló, J. L. Aceña, C. del Pozo, A. E. Sorochinsky, S. Fustero, V. A. Soloshonok and H. Liu, Chem. Rev., 2014, 114, 2432–2506 CrossRef CAS PubMed;
(b) B. C. Wang, L. J. Wang, B. Jiang, S. Y. Wang, N. Wu, X. Q. Li and D. Y. Shi, Mini-Rev. Med. Chem., 2017, 17, 683–692 CrossRef CAS PubMed;
(c) E. P. Gillis, K. J. Eastman, M. D. Hill, D. J. Donnelly and N. A. Meanwell, J. Med. Chem., 2015, 58, 8315–8359 CrossRef CAS PubMed;
(d) N. A. Meanwell, J. Med. Chem., 2018, 61, 5822–5880 CrossRef CAS PubMed;
(e) A. Burriss, A. J. F. Edmunds, D. Emery, R. G. Hall, O. Jacob and J. Schaetzer, Pest Manage. Sci., 2018, 74, 1228–1238 CrossRef CAS PubMed;
(f) E. Carbonnel, T. Poisson, P. Jubault, X. Pannecoucke and T. Besset, Front. Chem., 2019, 7, 111 CrossRef PubMed.
- Y. Pan, ACS Med. Chem. Lett., 2019, 10, 1016–1019 CrossRef CAS PubMed.
- For reviews, see:
(a) J. A. Ma and D. Cahard, Chem. Rev., 2004, 104, 6119–6146 CrossRef CAS PubMed;
(b) J. Nie, H. C. Guo, D. Cahard and J. A. Ma, Chem. Rev., 2011, 111, 455–529 CrossRef CAS PubMed;
(c) X. Y. Yang, T. Wu, R. J. Phipps and F. D. Toste, Chem. Rev., 2015, 115, 826–870 CrossRef CAS PubMed.
- V. G. Nenajdenko, K. I. Smolko and E. S. Balenkova, Tetrahedron: Asymmetry, 2001, 12, 1259–1266 CrossRef CAS.
-
(a) X.-J. Wang, Y. Zhao and J.-T. Liu, Org. Lett., 2007, 9, 1343–1345 CrossRef CAS PubMed;
(b) D. H. Zhang and C. Y. Yuan, Tetrahedron, 2008, 64, 2480–2488 CrossRef CAS;
(c) Y.-H. Deng, J.-Q. Chen, L. He, T.-R. Kang, Q.-Z. Liu, S.-W. Luo and W.-C. Yuan, Chem. - Eur. J., 2013, 19, 7143–7150 CrossRef CAS PubMed;
(d) J. D. Duan, Y. Y. Cheng, J. Cheng, R. Li and P. F. Li, Chem. - Eur. J., 2017, 23, 519–523 CrossRef CAS PubMed.
- C. Zheng, Y. Li, Y. Yang, H. Wang, H. Cui, J. Zhang and G. Zhao, Adv. Synth. Catal., 2009, 351, 1685–1691 CrossRef CAS.
-
(a) X.-J. Wang, Y. Zhao and J.-T. Liu, Synthesis, 2008, 3967–3973 CAS;
(b) S. Sasaki, T. Yamauchi and K. Higashiyama, Tetrahedron Lett., 2010, 51, 2326–2328 CrossRef CAS;
(c) Z.-K. Pei, Y. Zheng, J. Nie and J.-A. Ma, Tetrahedron Lett., 2010, 51, 4658–4661 CrossRef CAS;
(d) J. Hernández-Toribio, R. G. Arrayás, B. Martín-Matute and J. C. Carretero, Org. Lett., 2009, 11, 393–396 CrossRef PubMed;
(e) P. Li, Z. Chai, S.-L. Zhao, Y.-Q. Yang, H.-F. Wang, C.-W. Zheng, Y.-P. Cai, G. Zhao and S.-Z. Zhu, Chem. Commun., 2009, 7369–7371 RSC;
(f) X.-K. Chen, C.-W. Zheng, S.-L. Zhao, Z. Chai, Y. Q. Yang, G. Zhao and W.-G. Cao, Adv. Synth. Catal., 2010, 352, 1648–1652 CrossRef CAS;
(g) A. Sanz-Marco, G. Blay, M. C. Muñoz and J. R. Pedro, Chem. Commun., 2015, 51, 8958–8961 RSC.
-
(a) L. C. Morrill, J. Douglas, T. Lebl, A. M. Z. Slawin, D. J. Fox and A. D. Smith, Chem. Sci., 2013, 4, 4146–4155 RSC;
(b) A. T. Davies, P. M. Pickett, A. M. Z. Slawin and A. D. Smith, ACS Catal., 2014, 4, 2696–2700 CrossRef CAS;
(c) L. C. Morrill, L. A. Ledingham, J.-P. Couturier, J. Bickel, A. D. Harper, C. Fallan and A. D. Smith, Org. Biomol. Chem., 2014, 12, 624–636 RSC;
(d) R. Mose, M. E. Jensen, G. Preegel and K. A. Jørgensen, Angew. Chem., Int. Ed., 2015, 54, 13630–13634 CrossRef CAS PubMed;
(e) Y.-J. Lin, L.-N. Du, T.-R. Kang, Q.-Z. Liu, Z.-Q. Chen and L. He, Chem. - Eur. J., 2015, 21, 11773–11778 CrossRef CAS PubMed;
(f) N. Attaba, J. E. Taylor, A. M. Z. Slawin and A. D. Smith, J. Org. Chem., 2015, 80, 9728–9739 CrossRef CAS PubMed.
- For reviews, see:
(a) R. J. Sundberg, The Chemistry of Indoles. Academic. New York, 1996 Search PubMed;
(b) A.-U. Rahman and A. Basha, Indole Alkaloids. Harwood Academic, Amsterdam, 1998 Search PubMed;
(c) G. R. Humphrey and J. T. Kuethe, Chem. Rev., 2006, 106, 2875–2911 CrossRef CAS PubMed;
(d) K. S. Ryan and C. L. Drennan, Chem. Biol., 2009, 16, 351–364 CrossRef CAS PubMed;
(e) M. Ishikura and K. Yamada, Nat. Prod. Rep., 2009, 26, 803–852 RSC;
(f) M. Ishikura, K. Yamada and T. Abe, Nat. Prod. Rep., 2010, 27, 1630–1680 RSC;
(g) N. K. Kaushik, N. Kaushik, P. Attri, N. Kumar, C. H. Kim, A. K. Verma and E. H. Choi, Molecules, 2013, 18, 6620–6662 CrossRef CAS PubMed;
(h) T. V. Sravanthi and S. L. Manju, Indoles—A promising scaffold for drug development, Eur. J. Pharm. Sci., 2016, 91, 1–10 CrossRef CAS PubMed;
(i) N. Chadha and O. Silakari, Eur. J. Med. Chem., 2017, 134, 159–184 CrossRef CAS PubMed.
- For reviews, see:
(a) T. B. Poulsen and K. A. Jørgensen, Chem. Rev., 2008, 108, 2903–2915 CrossRef CAS PubMed;
(b) S. L. You, Q. Cai and M. Zeng, Chem.
Soc. Rev., 2009, 38, 2190–2201 RSC;
(c) H. Wu, Y. P. He and F. Shi, Synthesis, 2015, 47, 1990–2016 CrossRef CAS;
(d) V. Pirovano, Eur. J. Org. Chem., 2018, 17, 1925–1945 CrossRef;
(e) J. B. Chen and Y. X. Jia, Org. Biomol. Chem., 2017, 15, 3550–3567 RSC.
- Z. G. Li, Z. J. Shi and C. He, J. Organomet. Chem., 2005, 690, 5049–5054 CrossRef CAS.
-
(a) S.-G. Kim, Bull. Korean Chem. Soc., 2009, 30, 2519 CrossRef CAS;
(b) S. B. Jin, C. G. Li, Y. H. Ma, Y. H. Kan, Y. J. Zhang and W. B. Zhang, Org. Biomol. Chem., 2010, 8, 4011–4015 RSC;
(c) M. Ueda, Y. Yagyu and I. Ryu, Tetrahedron: Asymmetry, 2017, 28, 1070–1077 CrossRef CAS.
-
(a) W. T. Wang, X. H. Liu, W. D. Cao, J. Wang, L. L. Lin and X. M. Feng, Chem. - Eur. J., 2010, 16, 1664–1669 CrossRef CAS PubMed;
(b) T. Narumi, S. Tsuzuki and H. Tamamura, Asian J. Org. Chem., 2014, 3, 497–503 CrossRef CAS.
-
(a) G. Blay, I. Fernández, M. C. Muñoz, J. R. Pedro and C. Vila, Chem. - Eur. J., 2010, 16, 9117–9122 CrossRef CAS PubMed;
(b) B. Chaudhary, M. Diwakera and S. Sharma, Org. Chem. Front., 2018, 5, 3133–3137 RSC.
- N. K. Li, L. P. Kong, Z. H. Qi, S. J. Yin, J. Q. Zhang, B. Wu and X. W. Wang, Adv. Synth. Catal., 2016, 358, 3100–3112 CrossRef CAS.
-
(a) H. H. Huo, C. Fu, K. Harms and E. Meggers, J. Am. Chem. Soc., 2014, 136, 2990–2993 CrossRef CAS PubMed;
(b) S.-W. Li, Q. Wan and Q. Kang, Org. Lett., 2018, 20, 1312–1315 CrossRef CAS PubMed.
-
(a) E. Angelini, C. Balsamini, F. Bartoccini, S. Lucarini and G. Piersanti, J. Org. Chem., 2008, 73, 5654 CrossRef CAS PubMed;
(b) V. Pirovano, D. Facoetti, M. Dell'Acqua, E. D. Fontana, G. Abbiati and E. Rossi, Org. Lett., 2013, 15, 3812–3815 CrossRef CAS PubMed.
-
(a) C.-F. Li, H. Liu, J. Liao, Y.-J. Cao, X.-P. Liu and W.-J. Xiao, Org. Lett., 2007, 9, 1847–1850 CrossRef CAS PubMed;
(b) H. H. Butani, D. D. Vachhani, U. C. Bhoya, A. K. Shah and E. V. Van der Eycken, Eur. J. Org. Chem., 2015, 2015, 2124–2128 CrossRef CAS.
- J. N. Sun, Y. B. Hu, Y. N. Li, S. Zhang, Z. G. Zha and Z. Y. Wang, J. Org. Chem., 2017, 82, 5102–5110 CrossRef CAS PubMed.
- For reviews, see:
(a) X.-M. Peng, G. L. V. Damu and C.-H. Zhou, Curr. Pharm. Des., 2013, 19, 3884–3930 CrossRef CAS PubMed;
(b) S. Sandhu, Y. Bansal, O. Silakari and G. Bansal, Bioorg. Med. Chem., 2014, 22, 3806–3814 CrossRef CAS PubMed;
(c) H. Singh, J. V. Singh, K. Bhagat, H. K. Gulati, M. Sanduja, N. Kumar, N. Kinarivala and S. Sharma, Bioorg. Med. Chem., 2019, 27, 3477–3510 CrossRef CAS PubMed.
-
(a) R. Kenchappa, Y. D. Bodke, A. Chandrashekar, S. Telkar, K. S. Manjunatha and M. A. Sindhe, Arabian J. Chem., 2017, 10, S1336–S1344 CrossRef CAS;
(b) H. Osman, S. K. Yusufzai, M. S. Khan, B. M. Abd Razik, O. Sulaiman, S. Mohamad, J. A. Gansau, M. O. Ezzat, T. Parumasivam and M. Z. Hassan, J. Mol. Struct., 2018, 1166, 147–154 CrossRef CAS;
(c) For a review of coumarins as antiviral agents, see: M. Z. Hassan, H. Osman, M. A. Ali and M. J. Ahsan, Eur. J. Med. Chem., 2016, 123, 236–255 CrossRef CAS PubMed.
-
(a) G. Melagraki, A. Afantitis, O. Igglessi-Markopoulou, A. Detsi, M. Koufaki, C. Kontogiorgis and D. J. Hadjipavlou-Litina, Eur. J. Med. Chem., 2009, 44, 3020–3026 CrossRef CAS PubMed;
(b) R. K. Arora, N. Kaur, Y. Bansaln and G. Bansal, Acta Pharm. Sin. B, 2014, 4, 368–375 CrossRef PubMed.
- C. Fang, K. K. Lee, R. Nietupski, R. H. Bates, R. Fernandez-Menendez, E. M. Lopez-Roman, L. Guijarro-Lopez, Y. X. Yin, Z. Z. Peng, J. E. Gomez, S. Fisher, D. Barros-Aguirre, B. K. Hubbard, M. H. Serrano-Wu and D. T. Hung, Bioorg. Med. Chem. Lett., 2018, 28, 3529–3533 CrossRef CAS PubMed.
- K. V. Sashidhara, R. K. Modukuri, D. Choudhary, K. B. Rao, M. Kumar, V. Khedgikar and R. Trivedi, Eur. J. Med. Chem., 2013, 70, 802–810 CrossRef CAS PubMed.
- For a review, see: H. B. Li, Y. F. Yao and L. H. Li, J. Pharm. Pharmacol., 2017, 69, 1253–1264 CrossRef CAS PubMed.
-
(a) B.-F. Ruan, H.-J. Cheng, J. Ren, H.-L. Li, L.-L. Guo, X.-X. Zhang and C. Liao, Eur. J. Med. Chem., 2015, 103, 185–190 CrossRef CAS PubMed;
(b) S.-S. Xie, J.-S. Lan, X. Wang, Z.-M. Wang, N. Jiang, F. Li and L.-Y. Kong, Bioorg. Med. Chem., 2016, 24, 1528–1539 CrossRef CAS PubMed;
(c) J. Joubert, G. B. Foka, B. P. Repsold, D. W. Oliver, E. Kapp and S. F. Malan, Eur. J. Med. Chem., 2017, 125, 853–864 CrossRef CAS PubMed;
(d) A. Stefanachi, F. Leonetti, L. Pisani, M. Catto and A. Carotti, Molecules, 2018, 23, 250–273 CrossRef PubMed;
(e) A. Ibrar, S. A. Shehzadi, F. Saeed and I. Khan, Bioorg. Med. Chem., 2018, 26, 3731–3762 CrossRef CAS PubMed.
- For reviews, see:
(a) A. Thakur, R. Singla and V. Jaitak, Eur. J. Med. Chem., 2015, 101, 476–495 CrossRef CAS PubMed;
(b) J. Dandriyal, R. Singla, M. Kumar and V. Jaitak, Eur. J. Med. Chem., 2016, 119, 141–168 CrossRef CAS PubMed;
(c) L. F. Zhang and Z. Xu, Eur. J. Med. Chem., 2019, 181, 111587 CrossRef CAS PubMed.
- K. Pérez-Cruz, M. Moncada-Basualto, J. Morales-Valenzuela, G. Barriga-González, P. Navarrete-Encina, L. Núñez-Vergara, J. A. Squella and C. Olea-Azar, Arabian J. Chem., 2018, 11, 525–537 CrossRef.
-
(a) K.
M. Mahadevan, H. N. Harishkumar, J. N. Masagalli and H. T. Srinivasa, Mol. Cryst. Liq. Cryst., 2013, 570, 20–35 CrossRef CAS;
(b) V. Y. Korotaev, A. Y. Barkov and V. Y. Sosnovskikh, Tetrahedron, 2013, 69, 9642–9647 CrossRef CAS;
(c) Y. H. Bai, J. Y. Du and X. X. Weng, Spectrochim. Acta, Part A, 2014, 126, 14–20 CrossRef CAS PubMed;
(d) D. D. Fang, X. Q. Zheng, H. Yi, H. Dai, Z. S. Hong, Y. R. Peng and Y. Y. Lin, Sens. Actuators, B, 2019, 283, 407–414 CrossRef CAS.
-
(a) C. L. Xu, G. Y. Yang, C. X. Wang, S. F. Fan, L. X. Xie and Y. Gao, Molecules, 2013, 18, 11964–11977 CrossRef CAS PubMed;
(b) G. Y. Yang, J. T. Yang, C. X. Wang, S. F. Fan, P. H. Xie and C. L. Xu, J. Fluorine Chem., 2014, 168, 1–8 CrossRef CAS;
(c) G. Y. Yang, C. X. Wang, S. F. Fan, P. H. Xie, Q. Jin and C. L. Xu, Chin. J. Org. Chem., 2015, 35, 1173–1178 CrossRef CAS.
- J.-P. Bao, C.-L. Xu, G.-Y. Yang, C.-X. Wang, X. Zheng and X.-X. Yuan, Molecules, 2019, 24, 1745–1758 CrossRef PubMed.
-
(a) Y. Z. Xia, Y. Liang, Y. Y. Chen, M. Wang, L. Jiao, F. Huang, S. Liu, Y. H. Li and Z.-X. Yu, J. Am. Chem. Soc., 2007, 129, 3470–3471 CrossRef CAS PubMed;
(b) F.-Q. Shi, X. Li, Y. Z. Xia, L. M. Zhang and Z.-X. Yu, J. Am. Chem. Soc., 2007, 129, 15503–15512 CrossRef CAS PubMed;
(c) Y. Liang, S. Liu and Z.-X. Yu, Synlett, 2009, 905–909 CAS.
Footnotes |
† Electronic supplementary information (ESI) available. CCDC 1950379 and 1950380. For ESI and crystallographic data in CIF or other electronic format see DOI: 10.1039/d0ra01237h |
‡ These two authors contributed equally to this work. |
|
This journal is © The Royal Society of Chemistry 2020 |