DOI:
10.1039/D0RA00753F
(Paper)
RSC Adv., 2020,
10, 17171-17179
Hydroxyethyl sulfone based reactive coalescing agents for low-VOC waterborne coatings†
Received
24th January 2020
, Accepted 26th April 2020
First published on 1st May 2020
Abstract
The unique characteristics of water-based hydroxyethyl sulfone (HES)-vinyl sulfone (VS) dynamic equilibrium are exploited in the design of new reactive coalescing agents (RCAs) for the first time to address VOC (Volatile Organic Compound) emission issues from waterborne coatings. New RCAs were synthesized as HES analogues of widely used commercial coalescing agents (CAs) and characterized. These HES based RCAs are found to be effective towards film formation as evidenced by minimum film formation temperature (MFFT) studies. Equilibration of HES to VS of these RCAs was established and the VS intermediate was isolated and characterized. Detailed studies reveal that HES analogues of RCAs react with amine/hydroxyl containing monomers and latex only during the film formation through VS formation, while HES remains unreactive during storage in water or aqueous basic solution. The current study demonstrates the potential use of HES compounds as RCAs towards environmentally benign waterborne coatings. The reactivity of HES analogues towards latex polymer is found to be promoted during the film formation without use of any other external triggers like heat or light.
Introduction
One of the successful industrial approaches for making surface coatings with low environmental impact is adopting waterborne coatings instead of conventional solvent-borne formulations. Waterborne coatings are widely used in architectural and industrial coatings, adhesives, marine coatings, paper coatings etc. Tremendous efforts are currently ongoing by coating formulators to make the coating formulations environmentally benign.1,2 In general, emissions from waterborne coatings are significantly less compared to solvent based coatings due to the presence of water as the dispersing medium. Waterborne coatings use lattices made up of very high molecular weight film forming polymers, possessing a high glass transition temperature (Tg) to achieve desired properties after drying. A successful film formation process is essential to avoid porosity and to achieve properties like gloss, scrub resistance etc. that rely on latex particle deformation and polymer chain diffusion during a key stage of coating drying process3 called as coalescence. Seminal works by Winnik et al.4 and Keddie et al.5 have provided detailed understanding on morphology transformation, polymer chain diffusion and changes in microstructures during the process of coalescence. The usual approach to achieve better polymer chain diffusion is by lowering Tg or minimum film formation temperature (MFFT) of binder latex polymer with the addition of coalescing agents (CAs). Although these CAs exhibit lower volatility, they are eventually released (Fig. 1a) to the environment over time. Traditional commercial waterborne coatings often contain 2–10 wt% of such CA. This is a significant source of environmental pollutants considering global waterborne coatings market that accounts about 49.6 billion pounds (by dry weight) in 2016 (ref. 6) and increasing 4–6% annually.
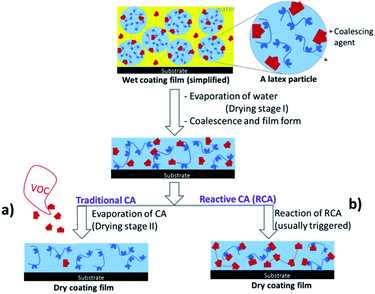 |
| Fig. 1 Film formation process in the presence of coalescing agents (CAs, (a)) and reactive coalescing agents (RCAs, (b)) in waterborne coating. | |
Both industries and academia are constantly addressing VOC emission issues due to environmental concerns, long term health hazards and stringent regulations on the usage of CAs. One of the ways to overcome this issue is to use reactive coalescing agents (RCAs).7 These RCAs act like traditional CAs before, and during coating film formation, however, permanently become integral part of the coating film due to their reactivity either with the latex binder polymer (Fig. 1b) or by the formation of high molecular weight species by reacting themselves. Improvement of Tg and MFFT after reaction of CAs with the latex polymer was recently confirmed8 by both molecular dynamics simulation and calorimetry. A series of low odour CAs containing dicyclopentenyloxyacrylate7,9 functional groups was reported to react in the presence of radical initiators during film formation. 2,2,4-Trimethyl-3-oxopentanoate substituted CAs such as ethylene glycol and butyl carbitol, were known10 to incorporate with latex polymer upon UV curing. Lahtinen et al.11 reported the synthesis of glycidyl esters/ethers and their potential application as RCAs. The efficiency of new RCAs in lowering the MFFT of the latex and film properties was shown to be competitive with commercial non-reactive CA, Nexocat™ 795. Fatty acid based RCAs were found to crosslink with acrylic resin by auto-oxidation process in waterborne formulations and decreased the drying time and MFFT substantially.12 In all the above examples (Fig. 2), except epoxy based RCAs, one or other external triggers are to be employed to trigger reactivity of these RCAs. Usage of external trigger will certainly limits the application area (exterior versus interior coatings), add additional cost (e.g. application of heat or light and use of initiator and metal catalysts) and complexity in the film formation. RCAs that behave like traditional CA during the storage in containers and application, but have the ability to react after the coating is fully dried and specifically without needing of any external trigger would be ideal for coating industries (Fig. 3).
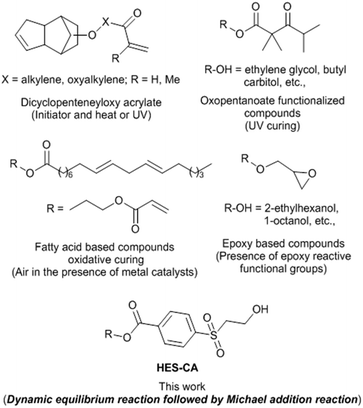 |
| Fig. 2 Comparison of representative known RCAs and new HES based RCAs and trigger methods (within parentheses) employed for their reactivity during film formation. | |
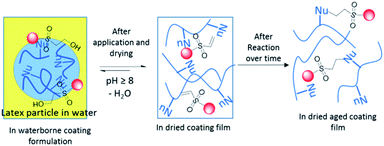 |
| Fig. 3 Hypothesis for the development new HES based RCAs and their reactivity during and after film formation. ‘Nu’ refers to free nucleophiles present in the latex. | |
HES are known to be in equilibrium with vinyl sulfone (VS) and its subsequent reaction with nucleophiles (Nu) via Michael addition reaction13 has been exploited in immobilization,14 solid phase synthesis, dye chemistry and bioconjugation.15 Berrisford et al. have exploited16 this HES-VS dynamic equilibrium reaction to produce crosslinked coating film by mixing complimentary latices having HES and hydroxyl groups followed by drying. Inspired by this work, we intend to develop HES based RCAs from conventional CAs for coating applications by employing HES-VS dynamic equilibrium. We envisage that once the VS is formed from HES, it will undergo Michael addition reaction with the nucleophilic functional groups present in the latex polymer and form a stable coating film. Herein, we report a series of HES compounds as novel RCAs for waterborne coatings. These RCAs were found to react with the polymer during drying in the absence of any external trigger.
Results and discussion
Synthesis and characteristics of hydroxyethyl sulfones (HES)
To test our hypothesis, we have synthesized four HES compounds by connecting β-hydroxylethylsulfone moiety with CAs such as butyl carbitol (BC-OH), ethylene glycol-2-ethylhexyl ether (EEH-OH), 2,2,4-trimethylpentane-1,3-diol (TMPD-OH) and 1-phenoxy-propan-2-ol (PPH-OH) that are widely used in coating and paint formulations. Compound 3 was obtained from commercially available 4-mercaptobenzoic acid in two steps by simple substitution and protection strategies under the reaction conditions described in Scheme 1. The tert-butyldimethyl silyl (TBDMS) protected compound 3 was coupled with hydroxyl containing coalescing agents (BC-OH, EEH-OH, TMPD-OH and PPH-OH) in the presence of 1-ethyl-3-(3-dimethylaminopropyl)carbodiimide (EDC) under basic conditions gave compounds 4a–4d in moderate to good yield. The thioethers 4a–4d were oxidized to sulfone using 1
:
1 mixture of hydrogen peroxide and acetic acid. Under the acidic conditions, we found that the TBDMS also cleaved during sulfoxidation and provided the desired HES compounds in 68–83% yield. The formation of products was confirmed by NMR, mass and elemental analysis. The relevant data are provided in the Experimental section and ESI.† HES-BC and HES-EEH are exist in liquid state, while HES-TMPD and HES-PPH are semi solids. To understand the existence of HES-VS equilibrium and cross-linking characteristics, HES-BC and HES-EEH were chosen for further studies due to their physical features. The volatile nature of HES-BC and HES-EEH were determined by measuring the weight loss at 100 °C for 1 h under EPA (Environmental Protection Agency) method 24 adopted in coating (ESI, Fig S1†). HES based RCAs possess lower percentage of weight loss (HES-BC, 12%; HES-EEH, 6%) in comparison to their CA analogues (BC, 24%; EEH, 53%). These values indicated that the current HES based RCAs could show significantly less VOC in the resultant coating.
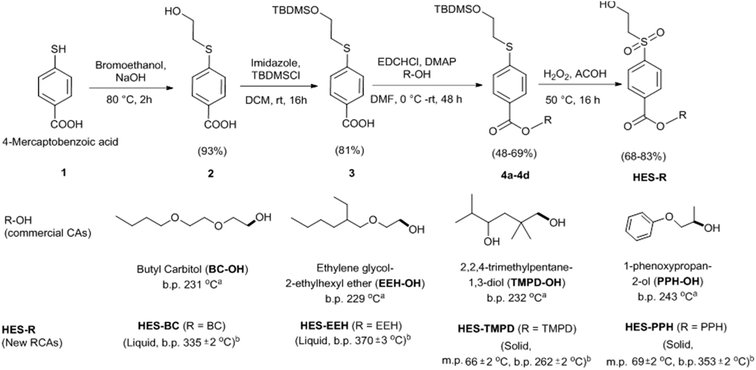 |
| Scheme 1 Synthesis of hydroxyethyl sulfone (HES) based reactive coalescing agents (RCAs). Boiling points of new RCAs and their commercial CA analogues are given for comparison. aReported in the literature. bDetermined. | |
To get preliminary understanding on the film formation features of waterborne latices influenced by the HES based compounds, MFFT measurements were carried out with two commercial latices. In the absence of CA, minimum film formation temperature (MFFT) of latex 1 and latex 2 was measured to be 52.6 °C and 31.2 °C respectively (Fig. 4). When 2 wt% HES-BC and HES-EEH were independently dispersed with latex 1, the MFFT of the resultant emulsion was diminished to 34.1 °C and 34.0 °C respectively. Similarly, decrease in MFFT was observed for latex 2 with HES-BC and HES-EEH (Fig. 4). The extent of reduction in MFFT for HES based RCAs is relatively lesser in comparison to the corresponding analogues BC and EEH, possibly due to both inherent physical characteristics (like Tg) of new HES based compounds and difference in molar concentration while using same wt% of HES coalescing agents. To confirm this, MFFT was measured by dispersing same moles of HES-BC (equivalent to 2 wt% of BC) with latex 2 and found to be 22.3 ± 0.6 °C. All the above MFFT studies primarily indicated that HES based compounds were dispersible with commercial waterborne lattices and the film formation was occurred in the presence of HES based compounds with reduction in MFFT values of the original latices.
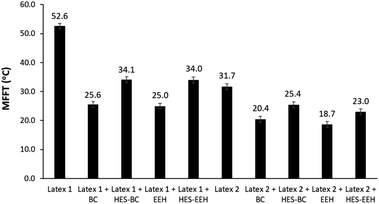 |
| Fig. 4 Minimum film formation temperature (MFFT) of commercial latices in the absence and presence of 2 wt% of CAs (BC-OH and EEH-OH) and HES based RCAs (HES-BC and EEH-BC). Average MFFT values are given from three independent measurements. | |
Studies on HES-VS equilibrium
The above observation encouraged to evaluate the usefulness of HES compounds as RCA for coating applications. In the process of HES reaction with latex or other additives, the formation of vinylsulfone (VS) is crucial. To evaluate the formation of VS from HES based compounds, we carried out set of experiments under basic conditions at which majority of coatings are formulated. HES-BC and HES-EEH were independently dissolved in acetone17 and dispersed uniformly in aqueous solution of sodium bicarbonate (0.1 M, pH ∼ 8.5) by vigorous stirring. The contents were discharged to Petri dish, allowed to evaporate at room temperature and the dry content was collected for investigation. Analysis of HES-BC dispersed contents by high pressure liquid chromatography (HPLC) equipped with mass spectrometer (MS) indicated (Fig. 5) the formation of VS1 (357.2 Da, M+) and HES-BC-VS1 adduct (731.2 Da, M+). HES-BC-VS1 adduct was presumably formed by the addition of HES-BC to VS1 by Michael type (Scheme 2) addition. When HES-EEH was subject to equilibration of VS2 (387.0 Da, M+) and HES-EEH-VS2 [755.0 Da, (M++Na)] were observed (ESI, Fig. S4 and S5†). To gain more understanding, the progress of HES-VS equilibrium was monitored by quantifying the unreacted HES using 2,6-difluorobenzaldehyde as an internal standard. The decrease in concentration of HES-BC and the formation of HES-VS adducts are evident (Fig. 6a). Both VS1 and HES-BC-VS1 adduct were isolated and characterized by NMR and high resolution mass spectroscopy. To our knowledge, VS intermediates have not been isolated and characterized in such HES-VS dynamic equilibrium reactions. In order to verify that the VS formation takes place only during the drying process as well as under basic conditions, the following two control experiments were performed; (i) when HES-BC was stirred in aqueous solution of sodium bicarbonate (0.1 M, pH ∼ 8.5) in a closed vial, no formation of VS was observed even after 4 days; (ii) when water was used instead of basic sodium bicarbonate solution, no VS1 formation was observed after drying. HES-BC was taken for further reactivity studies with monomers and latex, as HES-BC more readily (Fig. 6) forms VS1 than HES-EEH to VS2 (depletion of ∼70% for HES-BC vs. ∼57% for HES-EEH) under identical drying conditions. For broader applicability, the equilibrium studies also performed in the presence of benzyltrimethylammonium hydroxide (triton-B), an organic base under similar basic (pH ∼ 9.0) conditions for HES-BC. The formation of VS1 and HES-BC-VS1 was observed with 62% depletion of HES-BC (Fig. S6, ESI†) under identical duration and conditions. The formation of HES-BC-VS1 adduct strongly encouraged us to test the reactivity of HES with vinyl monomers and latex.
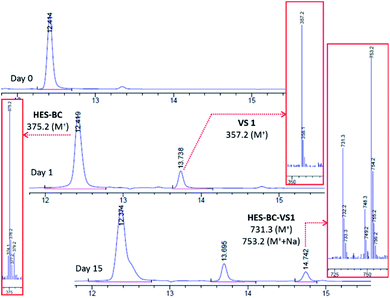 |
| Fig. 5 HPLC-MS traces of HES-BC and VS1 equilibrium and subsequent formation of HES-BC-VS1 adduct. | |
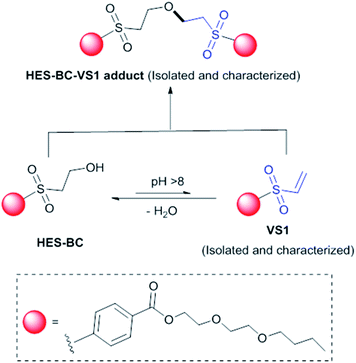 |
| Scheme 2 HES-VS equilibrium under basic conditions. Both VS1 and HES-BC-VS1 adduct were isolated and characterized by NMR and mass spectroscopy. | |
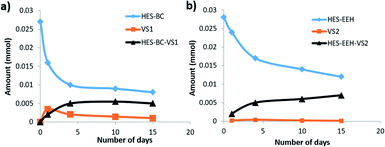 |
| Fig. 6 Rate of consumption of HES-BC (a) and HES-EEH (b) during equilibration under basic conditions. The progress of formation of HES-BC-VS1 and HES-EEH-VS2 adducts is provided. | |
Reactivity of HES/VS with monomers and latex
The reactivity of HES-BC with hydroxyl and amine containing monomers such as 2-hydroxyethyl methacrylate (HEMA) and tert-butylaminoethyl methyacrylate (TBAEMA) was evaluated (Scheme 3). The monomers were dispersed with HES-BC in aqueous solution of sodium bicarbonate (pH ∼ 8.5) or triton-B (0.1 M, pH ∼ 9.0) and allowed to dry in Petri dishs at ambient temperature. Mass analysing of the dry residue containing HEMA confirmed the presence of HES-BC [374.1 Da (M+)], VS1 [357.2 Da (M+)] and HES-BC-HEMA [509.2 Da (M++Na)] adduct (Fig. S8, ESI†). Conversely, only HES-BC and HES-BC-TBAEMA [542.3 Da (M+)] adducts were present in TBAEMA containing dry residue (Fig. S9, ESI†). This might be attributed to the higher reactivity TBAEMA with VS1 compared to HEMA. The formation of HES-BC-VS1 adduct [753.3, Da (M++Na)] was not observed in both dispersed mixtures, possibly due to the presence of excess HEMA and TBAEMA. All the above observations affirm that HES-BC has the tendency to form VS1 under basic conditions and further react with nucleophiles conveniently.
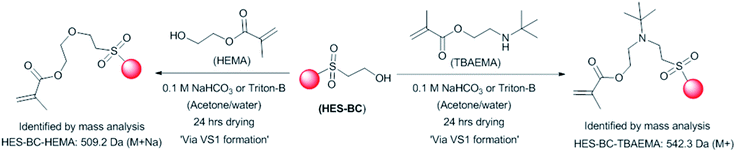 |
| Scheme 3 Reactivity of HES-BC with 2-hydroxyethyl methacrylate (HEMA) and tert-butylaminoethyl methyacrylate (TBAEMA) via VS1. | |
Finally, we evaluated the application of HES-BC as RCA towards coating application by synthesizing –OH containing latex18 using HEMA as one of the monomers. Polymer latex containing approximately 5 mol% hydroxyl groups was synthesized (Fig. 7) via an emulsion polymerization of methyl methacrylate (MMA), n-butyl acrylate (nBA) and 2-hydroxyethyl methacrylate (HEMA) at 65 °C, using ammonium persulphate initiator and sodium dodecyl sulphate surfactant under nitrogen atmosphere. After 30 min, pH was adjusted to ≈9.0 using NaHCO3 solution (5 wt%). Poly(MMA-co-nBA-co-2HEMA) latex of 32.7 wt% solid content and uniform particle size (Zave(DLS) = 62.0 nm, PDI = 0.09) was obtained. To assess the reactivity of HES-BC with poly(MMA-co-nBA-co-2HEMA) latex, HES-BC was dispersed directly with the latex. In another independent experiment, triton-B (0.05 wt% with respect to HES-BC) was added during dispersion. The dispersed content was taken into a Petri dish and dried at atmosphere. Sample was taken for analysis after 1, 4, 7, 10 and 15 days. The latex film was washed with diethyl ether and the ether soluble fraction containing unreacted HES-BC was analysed and quantified by NMR. About 84% and 79% depletion of HES-BC was observed after 10 days respectively in the absence and presence of triton-B (Fig. 8). Analysis of ether insoluble residue19 by NMR indicated the appearance of new broad peaks at 7.61 ppm and 7.36 ppm (Fig. 9a). These broad peaks were assigned to aromatic protons of poly(MMA-co-nBA-co-2HEMA) latex bound HES-BC (HES-BC-poly(MMA-co-nBA-co-2HEMA) latex, Fig. 9a). The peaks at 8.09 ppm and 7.91 ppm correspond to aromatic protons of unreacted HES-BC (Fig. 9a). The observed up-field chemical shift for aromatic protons after reaction with latex is possibly due to extensive hyperconjugation present in the polymeric structure of the latex. Further, the peaks of HES-BC-latex were confirmed by synthesizing VS1 independently and its subsequent reaction with poly(MMA-co-nBA-co-2HEMA) latex. The characteristic broad signals of aromatic protons for HES-BC-latex was found to be similar (Fig. 9b) for the residue obtained by the reaction of either with HES-BC or VS1. Thus, the incorporation of HES-BC to poly(MMA-co-nBA-co-2HEMA) latex was confirmed and the potential application of HES-BC as reactive coalescing agent is verified.
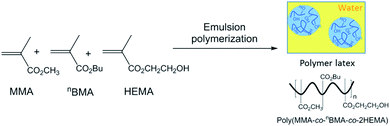 |
| Fig. 7 Latex synthesis by emulsion polymerization. | |
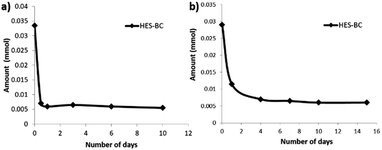 |
| Fig. 8 Rate of consumption of HES-BC in the absence (a) and presence (b) of triton-B during the film formation with latex. | |
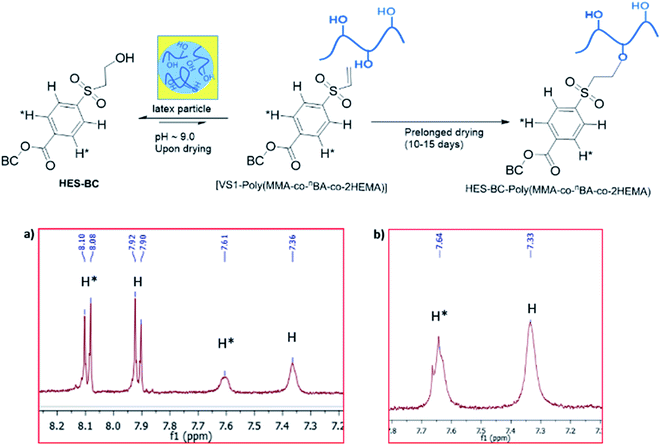 |
| Fig. 9 Schematic representation of the reaction of HES-BC with poly(MMA-co-nBA-co-2HEMA) latex through HES-VS equilibrium dynamics. Characteristic broad peaks observed for aromatic protons of HES-BC-latex are given. (a) 1H NMR spectrum of HES-BC-Latex obtained through HES-VS equilibrium dynamics; aromatic signals of unreacted HES-BC and HES-BC-Latex are respectively observed at 8.09/7.91 ppm and 7.61/7.36 ppm; (b) 1H NMR spectrum of HES-BC-poly(MMA-co-nBA-co-2HEMA) obtained directly by the reaction of VS1 with poly(MMA-co-nBA-co-2HEMA). | |
Experimental
General information on methods used for various analysis and spectral characterization are provided in the ESI.† All chemicals were purchased from commercial suppliers and used without further purification. Commercial latex 1 and latex 2 were received as gift sample respectively from BASF and Nippon.
Synthetic procedures
Synthesis of 2. To a solution of NaOH (4.57 g, 114.1 mmol) in 60 mL of water was added 4-mercaptobenzoic acid (8.00 g, 51.9 mmol) and stirred for 45 min at 80 °C till a clear solution was obtained. To this solution 2-bromoethanol (6.68 g, 53.4 mmol) was added and reaction mixture continued to stir for another 2 h. The reaction mixture was cooled and 1 N HCl solution was added slowly. White solid of 4-((2-hydroxyethyl)thio)benzoic acid (2) formed was filtered and dried to give the desired product. White solid (10.0 g, 97%); 1H NMR (400 MHz, D2O) δ 8.04–7.69 (m, 2H, ArH), 7.58–7.34 (m, 2H, ArH), 3.81 (td, J = 6.2, 0.6 Hz, 2H, CH2), 3.25 (t, J = 6.2 Hz, 2H, CH2); 13C NMR (101 MHz, D2O) δ 130.15, 127.19, 59.62, 33.85; HRMS (ESI): 199.0427 (M + H)+.
Synthesis of 3. Imidazole (1.48 g, 21.7 mmol) and TBDMSCl (2.01 g, 13.3 mmol) were added to 2 (2.4 g, 12.1 mmol) in dichloromethane (100 mL) and stirred at room temperature. After 16 h, the reaction mixture was filtered. The filtrate was concentrated and washed twice with hexanes to give the desired product 3. Light brown solid (3.0 g, 81%); 1H NMR (400 MHz, CDCl3) δ 7.99 (d, J = 8.5 Hz, 2H, ArH), 7.36 (d, J = 8.5 Hz, 2H, ArH), 3.86 (t, J = 6.8 Hz, 2H, CH2), 3.16 (t, J = 6.8 Hz, 2H, CH2), 0.89 (s, 9H, CH3), 0.06 (s, 6H, CH3); 13C NMR (101 MHz, CDCl3) δ 171.04, 144.69, 130.43, 126.50, 62.01, 34.56, 25.85, 18.31, −5.32; HRMS (ESI): 313.1288 (M + H)+.
General procedure for the synthesis of 4a–4d
To compound 3 (6.00 g, 19.2 mmol, 1.0 equiv.) in anhydrous DMF (20 mL), N-(3-dimethylaminopropyl)-N′-ethylcarbodiimide hydrochloride (EDC·HCl, 3.69 g, 19.2 mmol, 1.0 equiv.), 4-dimethylamino pyridine, (DMAP, 1.17 g, 9.6 mmol, 0.5 equiv.) and butyl carbitol (4.68 g, 28.8 mmol, 1.5 equiv.) were added sequentially at 0 °C under argon and stirred for 48 h at room temperature. Reaction mixture was diluted with water and extracted with EtOAc. The organic layer was washed with water twice, dried over Na2SO4 and concentrated. The colourless oil obtained was purified by column chromatography in EtOAc/hexanes (1/5 v/v) to give the desired product 4a as colourless oil (5.0 g, 57%). 1H NMR (400 MHz, CDCl3) δ 7.89 (dd, J = 8.5, 1.6 Hz, 2H, ArH), 7.27 (dd, J = 8.6, 1.7 Hz, 2H, ArH), 4.45–4.36 (m, 2H, CH2), 3.84–3.73 (m, 4H, CH2), 3.63 (dd, J = 6.1, 3.6 Hz, 2H, CH2), 3.58–3.50 (m, 2H, CH2), 3.41 (t, J = 6.6 Hz, 2H, CH2), 3.08 (t, J = 6.8 Hz, 2H, CH2), 1.54–1.46 (m, 2H, CH2), 1.36–1.24 (m, 2H, CH2), 0.86–0.82 (m, 12H, CH3), 0.05 (s, 6H, CH3); 13C NMR (101 MHz, CDCl3) δ 166.21, 143.84, 130.03, 126.56, 71.25, 70.73, 69.25, 64.09, 62.00, 34.64, 31.72, 25.64, 19.26, 18.30, 13.88, 5.33; HRMS (ESI): 457.2447 (M + H)+.
Compounds 4b, 4c and 4d were synthesized from compound 3 respectively utilizing EEH, TMPD and PPH, following the procedure used for the synthesis of 4a.
4b. Compound 4b was synthesized from 3 (5.00 g, 16.0 mmol) and ethylene glycol-2-ethylhexyl ether (EEH, 3.35 g, 8.00 mmol). Colourless oil (5.00 g, 66%); 1H NMR (400 MHz, CDCl3) δ 7.88 (d, J = 8.6 Hz, 2H, ArH), 7.27 (d, J = 8.6 Hz, 2H, ArH), 4.49–4.26 (m, 2H, CH2) 3.79 (t, J = 6.9 Hz, 2H, CH2), 3.75–3.56 (m, 2H, CH2), 3.34 (d, J = 5.9 Hz, 2H, CH2), 3.08 (t, J = 6.9 Hz, 2H, CH2), 1.48–1.41 (m, 1H, CH), 1.37–1.07 (m, 8H, CH2), 0.84 (s, 9H, CH3), 0.83–0.75 (m, 6H, CH3), −0.00 (s, 6H, CH3); 13C NMR (101 MHz, CDCl3) δ 166.22, 143.77, 139.47, 129.99, 126.95, 126.57, 74.26, 68.84, 64.08, 62.01, 39.61, 34.64, 30.55, 29.08, 25.84, 23.83, 23.07, 18.30, 14.05, 11.05, −5.33; HRMS (ESI): 469.2817 (M + H)+.
4c. Compound 3 (4.80 g, 15.3 mmol) and 2,2,4-trimethylpentane-1,3-diol (TMPD, 3.37 g, 23.0 mmol) afford 4c as colourless oil (3.30 g, 48%); 1H NMR (400 MHz, CDCl3) δ 7.86 (dd, J = 8.4, 1.5 Hz, 2H, ArH), 7.45–7.20 (m, 2H, ArH), 4.30, 3.95 (dd, J = 10.9, 1.3 Hz, 2H, CH2), 3.88–3.68 (m, 2H, CH2), 3.30 (dd, J = 2.6, 1.3 Hz, 1H, CH), 3.19–2.96 (m, 2H, CH2), 1.92 (ddt, J = 9.1, 6.8, 4.6 Hz, 1H), 1.05–0.88 (m, 12H), 0.83 (s, 9H), −0.00 (s, 6H); 13C NMR (101 MHz, CDCl3) δ 166.42, 144.03, 129.87, 126.88, 126.67, 79.36, 71.84, 62.03, 39.62, 34.66, 28.74, 25.84, 23.55, 22.13, 20.50, 18.29, 16.79, −5.33; HRMS (ESI): 441.2492 (M + H)+.
4d. Compound 4d was obtained from 1-phenoxypropan-2-ol (PPH, 5.00 g, 16.0 mmol) and 3 (3.60 g, 24.0 mmol) and isolated as white solid (5.0 g, 69%). 4d found to exist as rotamers; 1H NMR (400 MHz, CDCl3) δ 7.83 (dd, J = 18.7, 8.6 Hz, 2H, ArH), 7.37–7.07 (m, 4H, ArH), 7.00–6.80 (m, 3H, ArH), 5.64–5.22 (m, 1H, CH), 4.69 (td, J = 6.3, 4.4 Hz, 1H, CH), 4.55–4.18, 4.22–3.89 (m, 2H, CH2), 3.78 (t, J = 6.8 Hz, 2H, CH2), 3.07 (d, J = 0.9 Hz, 2H, CH2), 1.69–1.19 (m, 3H, CH3), 0.83 (d, J = 0.8 Hz, 9H, CH3), −0.00 (d, J = 1.0 Hz, 6H, CH3); 13C NMR (101 MHz, CDCl3) δ 166.07, 165.72, 158.70, 157.90, 144.05, 143.83, 130.01, 129.54, 129.47, 127.06, 126.57, 126.54, 121.26, 121.09, 116.27, 114.78, 72.00, 70.16, 69.50, 67.39, 62.00, 35.43, 34.66, 34.61, 31.87, 31.57, 25.85, 18.30, 17.03, 16.84, 14.08, −5.32; HRMS (ESI): 447.2027 (M + H)+.
General procedure for the synthesis of HES compounds
A solution containing 1
:
1 mixture of acetic acid and water (50 mL) was added to 4a (4.0 g, 8.9 mmol) followed by 25 mL of H2O2 (30% solution) at 0 °C. After the addition was complete, the reaction mixture was heated to 50 °C for 15 hours and quenched with saturated NaHCO3 at room temperature. The contents were extracted with EtOAc and concentrated under vacuum to give the crude product. Analytically pure product was obtained by column chromatography in DCM/MeOH (9/1 v/v) to afford 2.3 g (68%) of HES-BC as colourless oil; 1H NMR (400 MHz, CDCl3) δ 8.16 (d, J = 8.5 Hz, 2H, ArH), 7.92 (d, J = 8.4 Hz, 2H, ArH), 4.58–4.19 (m, 2H, CH2), 4.06–3.82 (m, 2H, CH2), 3.86–3.68 (m, 2H, CH2), 3.65–3.55 (m, 2H, CH2), 3.54–3.46 (m, 2H, CH2), 3.36 (t, J = 6.7 Hz, 2H, CH2), 3.33–3.20 (m, 2H, CH2), 1.51–1.36 (m, 2H, CH2), 1.30–1.17 (m, 2H, CH2), 0.80 (t, J = 7.4 Hz, 3H, CH3); 13C NMR δ 164.85, 142.94, 135.23, 130.66, 128.06, 71.27, 70.73, 70.10, 69.00, 64.92, 58.31, 56.24, 31.69, 19.25, 13.87; HRMS (ESI): 375.1482 (M + H)+.
HES-EEH, HES-TMPD and HES-PPH were synthesized from 4b, 4c and 4d respectively adopting the above general procedure.
HES-EEH. Colourless oil (4.00 g, 74%) was isolated from 4b (5.00 g, 10.6 mmol); 1H NMR (400 MHz, CDCl3) δ 8.18 (d, J = 8.4 Hz, 2H, ArH), 7.95 (d, J = 8.4 Hz, 2H, ArH), 4.55–4.26 (m, 2H, CH2), 4.07–3.84 (m, 2H, CH2), 3.80–3.56 (m, 2H, CH2), 3.32 (dd, J = 5.9, 1.7 Hz, 4H, CH2), 1.45 (dq, J = 8.5, 5.9, 4.3 Hz, 1H, CH), 1.39–1.03 (m, 8H, CH2), 0.80 (t, J = 7.4 Hz, 6H, CH3); 13C NMR (101 MHz, CDCl3) δ 164.83, 142.92, 135.31, 130.61, 128.06, 74.28, 68.60, 64.91, 58.31, 56.25, 39.58, 30.53, 29.06, 23.83, 23.05, 14.04, 11.04; HRMS (ESI): 387.1848 (M + H)+.
HES-TMPD. White solid (2.0 g, 74%) was obtained from 4c (3.30 g, 7.50 mmol); 1H NMR (400 MHz, CDCl3) δ 8.21 (d, J = 8.4 Hz, 2H, ArH), 8.01 (d, J = 8.4 Hz, 2H, ArH), 4.37, 4.10 (d, J = 10.9 Hz, 2H, CH2), 4.05–3.98 (m, 2H, CH2), 3.42–3.22 (m, 3H, CH2, CH), 1.97 (pd, J = 6.9, 2.5 Hz, 1H, CH), 1.27–0.67 (m, 12H, CH3); 13C NMR (101 MHz, CDCl3) δ 175.57, 165.02, 143.00, 135.35, 130.64, 128.28, 81.86, 79.41, 72.62, 69.87, 58.30, 56.23, 39.61, 28.78, 23.49, 22.14, 20.34, 16.77; HRMS (ESI): 376.1784 (M + H)+.
HES-PPH. The product existed as a mixture of rotamers. White solid (2.00 g, 74%) was isolated from 4d (3.38 g, 7.50 mmol); 1H NMR (400 MHz, CDCl3) δ 8.19–7.98 (m, 2H, ArH), 7.89 (dd, J = 8.5, 6.9 Hz, 2H, ArH), 7.24–7.10 (m, 2H), 6.95–6.73 (m, 3H, ArH), 5.44 (pd, J = 6.4, 4.2 Hz, 1H, CH), 4.92–4.53 (m, 1H, CH), 4.47–4.35, 4.16–4.00 (m, 2H, CH2), 3.96–3.83 (m, 2H, CH2), 3.34–3.20 (m, 2H, CH2), 1.38 (dd, J = 32.5, 6.4 Hz, 3H); 13C NMR (101 MHz, CDCl3) δ 164.72, 164.40, 158.55, 157.80, 143.06, 142.95, 135.39, 134.92, 130.63, 129.62, 129.55, 128.08, 128.05, 121.29, 116.24, 114.75, 71.80, 70.80, 69.99, 68.31, 58.30, 58.28, 56.21, 16.88, 16.67; HRMS (ESI): 365.1064 (M + H)+.
General procedure for HES-VS equilibrium studies
To acetone (1.0 mL) solution of HES-BC (10 mg, 0.027 mmol), 1 mL of 0.1 M NaHCO3 solution (for experiments with triton-B, 1 mL of diluted solution of triton-B with pH ∼ 9.0 was used instead) was added. The mixture was homogenized by constant stirring before transferring to a Petri dish quantitatively, and allowed to dry at 25 °C. For reliable quantification, the experiments were conducted in parallel using four Petri dishes for analysing the samples on various days (1, 4, 10 and 15 days). The dried contents in the Petri dish was suspended in CDCl3 (1 mL) containing 2,6-difluorobenzaldehyde (3.26 mg, 0.023 mmol) as internal standard for quantifying the amount of HES-BS, VS1 and HES-BC-VS1. The mixture was filtered using the micro filter and submitted for NMR analysis. The experiment was conducted in duplicate.
In a separate experiment, the contents in four Petri dishs were combined after 4 days and concentrated. VS1 and HES-BC-VS1 adduct were isolated from the mixture by semi preparative TLC using 10% ethyl acetate in hexanes.
VS1. 1H NMR (400 MHz, chloroform-d) δ 8.19–8.11 (m, 2H), 7.94–7.86 (m, 2H), 6.60 (dd, J = 16.5, 9.7 Hz, 1H), 6.45 (d, J = 16.6 Hz, 1H), 6.04 (d, J = 9.7 Hz, 1H), 4.49–4.41 (m, 2H), 3.82–3.74 (m, 2H), 3.65–3.58 (m, 2H), 3.56–3.49 (m, 2H), 3.39 (t, J = 6.7 Hz, 2H), 1.53–1.42 (m, 2H), 1.39–1.22 (m, 2H), 1.19 (t, J = 7.1 Hz, 2H); 13C NMR (101 MHz, chloroform-d) δ 171.25, 165.00, 143.43, 137.82, 134.77, 130.64, 129.10, 127.96, 71.30, 70.72, 70.09, 69.01, 64.88, 60.45, 31.69, 21.12, 19.28, 14.22, 13.96; HRMS (ESI): 357.1373 (M + H)+.
HES-BC-VS1. 1H NMR (400 MHz, chloroform-d) δ 8.23–8.02 (m, 1H), 7.99–7.68 (m, 1H), 4.62–4.35 (m, 1H), 3.86–3.75 (m, 1H), 3.73–3.59 (m, 3H), 3.58–3.48 (m, 2H), 3.39 (t, J = 6.7 Hz, 2H), 3.15 (t, J = 6.1 Hz, 1H), 1.52–1.42 (m, 1H), 1.36–1.19 (m, 2H), 0.83 (t, J = 7.4 Hz, 2H); 13C NMR (101 MHz, CDCl3) δ 164.88, 143.54, 134.98, 130.44, 128.02, 71.27, 70.73, 70.10, 69.01, 64.95, 64.18, 55.80, 31.69, 19.26, 13.92; HRMS (ESI): 731.2769 (M + H)+.
Synthesis of poly(MMA-co-nBA-co-2HEMA) latex
In a 250 mL glass jacketed reactor was charged with 144.2 g of distilled water, 2.14 g of sodium dodecyl sulphate, and 1.03 g of ammonium persulfate. The solution was purged with argon for 30 min at room temperature. The monomer mixture (nBA, MMA and 2HEMA: 33.5 g, 42.4 g and 4.65 g respectively) was degassed with argon for 30 min at room temperature and added via syringe for 3 h (≈30 mL h−1). The glass reactor was connected to Lauda water heater and the reaction was continued for 6 h at 65 °C. Finally, the reaction mixture was heated to 80 °C for 1.5 h to complete the polymerization reaction and no fouling was observed in the reaction. After the reaction, the latex sample pH was adjusted to ≈9.0 using NaHCO3 solution (aq, 5 wt%). The latex particle size was measured using Malvern DLS instrument (Zave = 62.0 nm, PDI = 0.09) (latex total solid content = 37.2 wt%). MFFT of the latex was measured thrice using Rhopoint instrument. MFFT of poly(MMA-co-nBA-co-2HEMA) latex: 18.5 ± 0.2 °C. MFFT of poly(MMA-co-nBA-co-2HEMA) latex after dispersing HES-BC: 10.4 ± 0.4 °C.
Reactivity of HES-BC with latex
HES-BC (65.1 mg, 0.17 mmol) was dissolved in 6 mL of acetone. Acetone (1.0 mL) containing HES-BC (0.028 mmol) was added to 5 different vials having poly(MMA-co-nBA-co-2HEMA) latex (1.0 mL) solution. The contents were stirred for homogenization and allowed to evaporate in a Petri dish independently and analysed on various days (1, 4, 7, 10 and 15). The film obtained was taken in diethyl ether, washed thrice with same solvent after sonicating each time for 5 min. The ether washings were collected, concentrated and dissolved in CDCl3 for NMR analysis. The unreacted HES-BC was quantified by adding known amount of internal standard (2,6-difluorobenzaldehyde). The latex film was dried after washing, collected and dissolved in THF-D8 for NMR analysis.
Conclusions
A series of 2-hydroxyethylsulfone (HES) analogues of commercial coalescing agents were synthesized and their application as reactive coalescing agents (RCAs) towards VOC-free waterborne coating was assessed. The HES based RCAs demonstrated to be stable during storage under basic aqueous conditions and in the presence of hydroxyl containing latex. The reaction of HESs with latex was found to take place only during film formation after drying process. Detailed equilibrium studies revealed that RCAs readily forms corresponding vinyl sulfones (VS) during drying process, which befitted as good Michael acceptors for the addition of nucleophilic functional groups present with the latex polymer. The formation of VS intermediate was isolated and confirmed by spectroscopic methods. Thus, the results presented in the article established one of the potential applications of hydroxyethyl sulfone based compounds towards developing environmentally benign VOC free waterborne coating formulations. Detailed understanding on coalescence and film formation by microscopy or Förster Resonance Energy Transfer (FRET) analysis, suitability and performance of these promising HES based RCAs in real coatings are topics for our future study.
Conflicts of interest
There are no conflicts to declare.
Acknowledgements
This work was funded by the Agency for Science, Technology and Research (A*STAR), Singapore under ‘Environmentally friendly speciality products’ programme (Science and Engineering Research Council grant no. 152 80 00044). Prof. Alexander M. Van Herk, programme manager, Institute of Chemical and Engineering Sciences, Singapore has provided meaningful insights on the present work during meetings.
Notes and references
- W. Arendt, R. Hanes and E. McBride, Paints & Coatings Industry, Sept 2012, https://www.pcimag.com/articles/96764-advances-in-low-voc-coalescent-technology- Search PubMed.
- H. Frommelius, A. Natesh, H.-G. Schulte, S. Shah and V. Shah, Paints & Coatings Industry, Jan 2009, https://www.pcimag.com/articles/88856-low-odor-voc-free-renewable-coalescents-for-latexes-used-in-very-low-voc-paints Search PubMed.
-
(a) J. L. Keddie and A. F. Routh, Fundamentals of latex film formation: Processes and properties, Springer, 2010 CrossRef
;
(b) P. A. Steward, J. Hearn and M. C. Wilkinson, Adv. Colloid Interface Sci., 2000, 86, 195–267 CrossRef CAS PubMed
;
(c) S. Zohrehvand and K. te Nijenhuis, Colloid Polym. Sci., 2005, 283, 1305–1312 CrossRef CAS
. -
(a) M. Kobayashi, Y. Rharbi and M. A. Winnik, Macromolecules, 2001, 34, 1855–1863 CrossRef CAS
;
(b) M. Soleimani, J. C. Haley, D. Majonis, G. Guerin, W. Lau and M. A. Winnik, J. Am. Chem. Soc., 2011, 133, 11299–11307 CrossRef CAS PubMed
. -
(a) J. L. Keddie, P. Meredith, R. A. L. Jones and A. M. Donald, Macromolecules, 1995, 28, 2673–2682 CrossRef CAS
;
(b) F. T. Carter, R. M. Kowalczyk, I. Millichamp, M. Chainey and J. L. Keddie, Langmuir, 2014, 30, 9672–9681 CrossRef CAS PubMed
. - https://www.coatingsworld.com/issues/2017-09-01/view_market-research/kusumgar-nerlfi-amp-growney-publish-third-global-p.
- https://www.pcimag.com/articles/83117-formulating-solutions-meeting-voc-regulations-with-coalescents.
- M. Klähn, R. Krishnan, J. M. Phang, F. C. H. Lim, A. M. van Herk and S. Jana, Polymer, 2019, 179, 121635 CrossRef
. - W. D. Weir, Low odor reactive coalescent, US Pat., application no. US6509494B1, 2003
. - J. T. Maddox, M. D. Clark and R. L. Engan, Waterborne coating compositions that include 2,2,4-trimethyl-3-oxopentanoate esters as reactive coalescents, International patent application no. WO 2012/082512Al, 2012
. - M. Lahtinen, E. Glad, S. Koskimies, F. Sundholm and K. Rissanen, J. Appl. Polym. Sci., 2003, 87, 610 CrossRef CAS
. - J. V. Barbosa, E. Veludo, J. Moniz, F. D. Magalhães and M. M. S. M. Bastos, Eur. J. Lipid Sci. Technol., 2012, 114, 1175 CrossRef CAS
. -
(a) M. Teyssot, M. Fayolle, C. Philouze and C. Dupuy, Eur. J. Org. Chem., 2003, 54 CrossRef CAS
;
(b) S. Strasser, C. Wappl and C. Slugovc, Polym. Chem., 2017, 8, 1797 RSC
;
(c) C. F. Nising and S. Bräse, Chem. Soc. Rev., 2008, 37, 1218 RSC
. -
(a) D. C. Meadows and J. Gervay-Hague, Med. Res. Rev., 2006, 26, 793 CrossRef CAS
;
(b) N. S. Simpkins, Tetrahedron, 1990, 46, 6951 CrossRef CAS
;
(c) B. D. Mather, K. Viswanathan, K. M. Miller and T. E. Long, Prog. Polym. Sci., 2006, 31, 487 CrossRef CAS
. - J. Morales-Sanfrutos, J. Lopez-Jaramillo, M. Ortega-Mũnoz, A. Megia-Fernandez, F. Perez-Balderas, F. Hernandez-Mateo and F. Santoyo-Gonzalez, Org. Biomol. Chem., 2010, 8, 667 RSC
. - D. J. Berrisford, P. A. Lovell, N. R. Sulimanab and A. Whiting, Chem. Commun., 2005, 5904 RSC
. - HES can be dispersed directly in aqueous sodium bicarbonate solution or latex. As the quantity of HES used for equilibrium or reactivity studies is low, HESs were dissolved in acetone for convenience and easy handling of viscous HES compounds.
- We have not used commercial latex for reactivity studies as its monomer composition and –OH content, if any, are not known. However, for MFFT measurements (Fig. 4) we have used commercial latex for convenience and to prove suitability for wider application.
- To eliminate the physical adsorption of HES-BC and/or VS1 to the latex, for each analysis, collected samples was taken in diethyl ether and sonicated for 5 mins and the process was repeated for three times.
Footnote |
† Electronic supplementary information (ESI) available: For HPLC analysis of reaction mixture, mass analysis of intermediates and spectral data for all the compounds. See DOI: 10.1039/d0ra00753f |
|
This journal is © The Royal Society of Chemistry 2020 |
Click here to see how this site uses Cookies. View our privacy policy here.