DOI:
10.1039/D0RA00693A
(Paper)
RSC Adv., 2020,
10, 12360-12364
Bifunctional phase-transfer catalysts for synthesis of 2-oxazolidinones from isocyanates and epoxides†
Received
22nd January 2020
, Accepted 19th March 2020
First published on 26th March 2020
Abstract
A series of bifunctional phase-transfer catalysts (PTCs) were synthesized to catalyze the [3 + 2] coupling reaction of isocyanates and epoxides to afford 2-oxazolidinones in good to high yields (up to 92% yield) using PhCl as a solvent at 100 °C within 12 h. These bifunctional PTCs were easily prepared from commercially available tertiary-primary diamines and isocyanates (or isothiocyanates, mono-squaramides, respectively) in two simple steps with good modularity and demonstrated high efficiency (2.5 mol% catalyst-loading). The synergistic interaction of the quaternary ammonium salt center and hydrogen-bond donor group in the catalyst with the substrate is crucial to this atom-economic reaction.
1 Introduction
2-Oxazolidinones and derivatives are important nitrogen and oxygen containing heterocycles present in many chemicals with different biological and pharmacologic activities,1–3 such as Linezolid and Tedizolid as antibiotics (Fig. 1) to treat infections caused by Gram-positive bacteria that are resistant to other antibiotics.4,5 Much attention has been paid by synthetic organic chemists to find facile methods to 2-oxazolidinones. There are many synthetic routes to 2-oxazolidinones, and these methods can be divided into two types including phosgene-involved and non-phosgene involved routes. The synthesis of 2-oxazolidinones from phosgene and vicinal amino-alcohols is not recommended, even if the yield is excellent, but its toxicity is extremely high.6,7 Non-phosgene involved routes to 2-oxazolidinones are investigated extensively in recent years.8–10 Among them, the ring-opening of aziridines by CO2 and “one-pot” reaction of primary amines with epoxides and CO2 are straightforward accesses to 2-oxazolidinones.11–15 The [3 + 2] coupling of isocyanates with epoxides is also an atom-economic process to provide 2-oxazolidinones, and the pioneering work on this coupling was established by Speranza and Peppel in 1958 through phase-transfer catalysis with a high temperature up to 200 °C.16 After that, several metallic catalysts have been found to catalyze this [3 + 2] coupling reaction,17–23 but still with unsatisfactory results because of harsh conditions (e.g., high catalyst-loadings, high temperature, large amount of epoxides, or with a dropwise addition of isocyanates). In 2017, Toda and colleagues24 have discovered an elegant route to 2-oxazolidinones with high yields by using tetraarylphosphonium salts (TAPS) as an organocatalyst. However, organocatalyzed [3 + 2] coupling of isocyanates and epoxides to 2-oxazolidinones is still a challenge. Herein, we presented a group of bifunctional phase-transfer catalysts (Bif-PTCs) for synthesis of 2-oxazolidinones in good yields and high efficiency (Fig. 2).
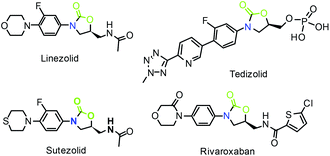 |
| Fig. 1 Pharmaceutics containing 2-oxazolidinone motif. | |
 |
| Fig. 2 Phase-transfer catalysts for synthesis of 2-oxazolidinones. | |
2 Results and discussion
2.1. The preparation of bifunctional phase-transfer catalysts (Bif-PTCs)
The synthesis and applications of bifunctional phase-transfer catalysts are very attractive to academies and industries for their high efficiency and environmental sustainability.25–29 These Bif-PTCs usually contain both one quaternary onium salt center and at least one hydrogen-bonding donor group in their structures. The hydrogen-bonding donor groups include hydroxyl (–OH), (thio)urea and squaramide. Recently, we have reported catalytic asymmetric amination of β-nitrostyrenes by chiral Bif-PTCs in high yields and enantioselectivties.30 We have also developed a group of Bif-PTCs for catalyzed cycloaddition of CO2 with epoxides to produce cyclic carbonates in good to excellent yields.31 For our continuous effort on the synthesis and applications of Bif-PTCs, we have prepared a series of quaternary ammonium salts with one hydrogen-bonding donor for catalyzed [3 + 2] coupling of isocyanates and epoxides to 2-oxazolidinones. From commercially available tertiary-primary diamines, Bif-PTC-1–10 were prepared in moderate to good yields through 2–4 steps. These catalysts featured by simple preparation, high modularity and good stability. There are only two simple steps including coupling reaction of tertiary-primary diamines with isocyanates and following quaternization to afford urea-containing Bif-PTCs (Bif-PTC-1–7) in good yield. In the synthetic process of thiourea-containing Bif-PTCs (Bif-PTC-8 and 9), Boc-protection and deprotection were used to avoid S-alkylation in quaternization by benzyl bromide.32 Squaramide-containing Bif-PTC-10 can be easily prepared from coupling of mono-ester of squaramide with 2-(pyrrolidin-1-yl)ethan-1-amine and then quaternization by benzyl bromide. The structures of these Bif-PTCs are listed in Fig. 3, and all Bif-PTCs are characterized by their NMR and HRMS.31
 |
| Fig. 3 The chemical structures of Bif-PTC-1–10. | |
2.2. The [3 + 2] coupling of isocyanates and epoxides by Bif-PTCs
Inspired by Toda and colleagues' work, a free O–H of TAPS plays a role as hydrogen-bonding donor in activation of epoxides, these above prepared Bif-PTCs also contain free N–H in their structures, we deduced that these Bif-PTCs could be used as organocatalysts for synthesis of 2-oxazolidinones from the coupling of epoxides and isocyanates.
At first, we chose the coupling of phenyl glycidyl ether 1a and p-chlorobenzene isocyante 2a as a model reaction for optimization of reaction conditions, and the results are shown in Table 1. It was found that without catalyst leads to no reaction (entry 1 vs. 3). When TBAB was used as catalyst, 2-oxazolidinone 3aa was obtained in very low yield with the recovery of the substrate epoxide 1a (entry 2). Bif-PTCs with electron-donating groups at phenyl ring (such as para-Me or 3,5-diMe, Bif-PTC-3, 4, 6 and 7) have shown low catalytic activities to this [3 + 2] cycloaddition and produced 3aa in low yields (entries 5, 6, 8 and 9, 38–45%). The catalysts with a urea group demonstrate better performance than catalysts with a thiourea or squaramide group in this [3 + 2] coupling reaction (entry 3 vs. entries 10–12). Bif-PTC-1 is the best catalyst to provide 2-oxazolidinone 3aa in 85% yield under standard reaction conditions. Instead of PhCl as solvent, when the coupling of 1a and 2a were carried out in toluene, NMP or 1,4-dioxane, the yield of 3aa is decreased significantly (entry 3 vs. entries 13–15).
Table 1 The screening of coupling reaction conditions for 2-oxazolidinone
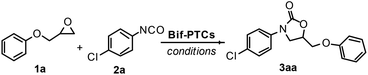
|
Entrya |
Bif-PTCs |
Solvent |
Temp. |
Time |
Yieldc (%) |
0.2 mmol of 1a and 0.21 mmol of 2a in 2 mL PhCl were stirred under inert atmosphere. TBAB = (n-Bu)4N+Br−. Isolated yield based on 1a. NMP = 1-methylpyrrolidin-2-one. Bif-OC-1 is the precursor (without quaternization) of Bif-PTC-1. |
1 |
None |
PhCl |
100 °C |
12 h |
0 |
2 |
TBABb (2.5 mol%) |
PhCl |
100 °C |
12 h |
12 |
3 |
Bif-PTC-1 (2.5 mol%) |
PhCl |
100 °C |
12 h |
85 |
4 |
Bif-PTC-2 (2.5 mol%) |
PhCl |
100 °C |
12 h |
84 |
5 |
Bif-PTC-3 (2.5 mol%) |
PhCl |
100 °C |
12 h |
45 |
6 |
Bif-PTC-4 (2.5 mol%) |
PhCl |
100 °C |
12 h |
40 |
7 |
Bif-PTC-5 (2.5 mol%) |
PhCl |
100 °C |
12 h |
80 |
8 |
Bif-PTC-6 (2.5 mol%) |
PhCl |
100 °C |
12 h |
46 |
9 |
Bif-PTC-7 (2.5 mol%) |
PhCl |
100 °C |
12 h |
38 |
10 |
Bif-PTC-8 (2.5 mol%) |
PhCl |
100 °C |
12 h |
24 |
11 |
Bif-PTC-9 (2.5 mol%) |
PhCl |
100 °C |
12 h |
26 |
12 |
Bif-PTC-10 (2.5 mol%) |
PhCl |
100 °C |
12 h |
36 |
13 |
Bif-PTC-1 (2.5 mol%) |
Toluene |
100 °C |
12 h |
70 |
14 |
Bif-PTC-1 (2.5 mol%) |
NMPd |
100 °C |
12 h |
Trace |
15 |
Bif-PTC-1 (2.5 mol%) |
1,4-Dioxane |
100 °C |
12 h |
32 |
16 |
Bif-PTC-1 (5 mol%) |
PhCl |
100 °C |
12 h |
87 |
17 |
Bif-PTC-1 (10 mol%) |
PhCl |
100 °C |
12 h |
92 |
18 |
Bif-PTC-1 (2.5 mol%) |
PhCl |
r.t. |
12 h |
22 |
19 |
Bif-PTC-1 (2.5 mol%) |
PhCl |
60 °C |
12 h |
45 |
20 |
Bif-OC-1e (2.5 mol%) |
PhCl |
100 °C |
12 h |
0 |
21 |
Bif-OC-1/TBAB (2.5 mol%) |
PhCl |
100 °C |
12 h |
75 |
22 |
Bif-OC-1/TBAF (2.5 mol%) |
PhCl |
100 °C |
12 h |
0 |
23 |
Bif-OC-1/TBAI (2.5 mol%) |
PhCl |
100 °C |
12 h |
72 |
24 |
Bif-PTC-1 (2.5 mol%) |
PhCl |
100 °C |
8 h |
56 |
25 |
Bif-PTC-1 (2.5 mol%) |
PhCl |
100 °C |
4 h |
33 |
Increase of catalyst-loading from 2.5 mol% to 5 mol% and 10 mol%, the yield of 3aa is promoted slightly (entry 3 vs. entries 16 and 17). When the reaction temperature was set at 60 °C and room temperature (r.t.), the yield of 3aa was dropped to 45% and 22%, respectively (entry 3 vs. entries 18 and 19). By using Bif-OC-1 (the precursor of Bif-PTC-1, without quaternization) as a catalyst, the corresponding 2-oxazolidin-one 3aa was not obtained through the coupling process (entry 20). However, when the catalytic amount of Bif-OC-1 and TBAB were used, 3aa was obtained in 75% yield (entry 21). Switching TBAB to TBAF, no 3aa is found by TLC checking (entry 22), and replacing TBAB with TBAI, the yield of 3aa is 72% (entry 23). This may due to the better nucleophilicity and leaving property of Br− than F−. The dual role of Br− in the catalytic cycle is a good nucleophile for ring-opening of epoxide and also a good leaving group during the cyclization. The combined use of TBAB and urea with electron-withdrawing group (such as CF3) is alternative to the coupling of epoxides and isocyanates to form 2-oxazolidinones. The decrease of reaction time resulted in the decrease of yields of 3aa (entries 24 and 25). Based on these above screening, the optimal conditions are listed as follows: 2.5 mol% of Bif-PTC-1 as catalyst, PhCl as solvent, the reaction mixture was stirred at 100 °C for 12 h. With the optimal reaction conditions in hand, various isocyanates (1b–f) and epoxides (2b–f) were used as substrates to produce a number of 2-oxazolidinones (3aa–fc) in moderate to good yields. The scope of substrates was shown in Fig. 4. It was found that arylisocyanates contain one electron-donating group (p-Me) can afford corresponding 2-oxazolidinones in high to excellent yields (examples 3ab, 3bb, 3cb, 3db and 3eb vs. the others). The glycidol-derivated epoxides provide 2-oxazolidinones in better yields than styrene oxides (3fc vs. 3ga), it may due to the electron-withdrawing effect of aryl rings.
 |
| Fig. 4 The Bif-PTC-1 catalyzed synthesis of 2-oxazolidinones. | |
A plausible mechanism for Bif-PTCs catalyzed [3 + 2] coupling of isocyanate and epoxide is proposed in Fig. 5. At the beginning, the epoxide 1 is activated by Bif-PTC-1 through hydrogen-bonding interaction between two N–H and O atom to form intermediate A, which undergoes nucleophilic attack from Br− to give intermediate B, intermediate B attacks isocyanate 2 to yield intermediate C, the intramolecular ring-closing of intermediate C provides 2-oxazolidinone 3 with a release of Bif-PTC-1 to take apart in the next catalytic cycle. It was found that isocyanates and epoxides are less soluble in PhCl, so the PTCs may play a role as a substrate-to-solvent transfer agent. In addition, Br− in PTCs as a nucleophile attacks epoxide to make ring-opening process in this catalytic cycle. In order to show the application of this above [3 + 2] coupling reaction, a intermediate 6 of a potent antibiotic Linezolid was directly prepared in 53% yield by the coupling of epoxide 4 and isocyanate 5 under standard reaction conditions (Scheme 1).
 |
| Fig. 5 The plausible catalytic cycle for Bif-PTCs catalyzed [3 + 2] coupling reaction. | |
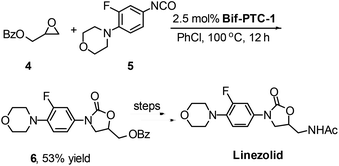 |
| Scheme 1 The preparation of a intermediate for Linezolid. | |
3 Experimental
3.1. General
1H NMR and 13C NMR spectra were measured in CDCl3, solution on a Bruker AV-400 spectrometer using TMS as an internal reference. Coupling constant (J) values are given in Hz. Multiplicities are designated by the following abbreviations: s, singlet; d, doublet; t, triplet; q, quartet; br, broad; m, multiplet. High-resolution mass spectra (HRMS) were performed on a Bruker microTOF-Q II Mass Spectrometer with ES ionization (ESI). All commercially available reagents were used as received. Thin-layer chromatography on silica (with GF254) was used to monitor all reactions. Products were purified by flash column chromatography on silica gel purchased from Qingdao Haiyang Chemical Co., Ltd. The preparation and characterization data of all bifunctional phase-transfer catalysts (Bif-PTCs) were found in our previous report.31
3.2. Typical procedure for the synthesis of 2-isoxazolidinones 3
0.20 mmol of epoxide 1, 2.7 mg of Bif-PTC-1 (2.5 mol%), and 0.21 mmol of isocyanate 2 in 2.0 mL PhCl was stirred for 12 h at 100 °C under inert atmosphere. The solvent was evaporated under reduced pressure and the residue was purified by a flash column chromatography (petroleum ether: ethyl acetate = 2
:
1 to 1
:
3) to yield corresponding 2-oxazolidinones 3. The characterization data of products were found in ESI.†
4 Conclusions
In conclusion, 2-oxazolidinones have been prepared through [3 + 2] coupling reactions of isocyanates and epoxides in the presence of 2.5 mol% bifunctional phase-transfer catalysts (Bif-PTCs) in good to excellent yields. These hydrogen-bonding donor containing Bif-PTCs can be easily prepared from commercially available tertiary-primary diamines and iso(thio)cyanates or mono-squaramide. Furthermore, an intermediate of Linezolid was also obtained in 53% yield by this simple manipulation. The synthesis of 2-oxazolidinone-containing bioactive molecules by using this strategy are underway in our laboratory.
Conflicts of interest
There are no conflicts to declare.
Acknowledgements
We thank the National Natural Science Foundation of China (21372259 and 81503028) for financial support.
Notes and references
- M. Baumann, I. R. Baxendale, S. V. Ley and N. Nikzad Nikbin, Beilstein J. Org. Chem., 2011, 7, 442 CrossRef CAS PubMed.
- D. J. P. Pinto, J. M. Smallheer, D. L. Cheney, R. M. Knabb and R. R. Wexle, J. Med. Chem., 2010, 53, 6243 CrossRef CAS PubMed.
- H. Suzuki, I. Utsunomiya, K. Shudo, T. Fujimura, M. Tsuji, I. Kato, T. Aoki, A. Ino and T. Iwaki, ACS Med. Chem. Lett., 2013, 4, 1074 CrossRef CAS PubMed.
- M. F. Gordeev and Z. Y. Yuan, J. Med. Chem., 2014, 57, 4487 CrossRef CAS.
- S. J. Brickner, M. R. Barbachyn, D. K. Hutchinson and P. R. Manninen, J. Med. Chem., 2008, 51, 1981 CrossRef CAS PubMed.
- K. C. Murdock, J. Org. Chem., 1968, 33, 1367 CrossRef CAS.
- D. Crich, K. Ranganathan, S. Rumthao and M. Shirai, J. Org. Chem., 2003, 68, 2034 CrossRef CAS PubMed.
- M. Zhou, X. Zheng, Y. Wang, D. Yuan and Y. Yao, ChemCatChem, 2019, 11, 5783 CrossRef CAS.
- Y. Xie, C. Lu, B. Zhao, Q. Wang and Y. Yao, J. Org. Chem., 2019, 84, 1951 CrossRef CAS PubMed.
- S. Arshadi, A. Banaei, S. Ebrahimias, A. Monfared and E. Vessally, RSC Adv., 2019, 9, 19465 RSC.
- X. Wang, W. Gao, Z. Niu, L. Wojtas, J. A. Perman, Y. Chen, Z. Li, B. Aguila and S. Ma, Chem. Commun., 2018, 54, 1170 RSC.
- B. Xu, P. Wang, M. Lv, D. Yuan and Y. Yao, ChemCatChem, 2016, 8, 2466 CrossRef CAS.
- B. Wang, Z. Luo, E. H. M. Elageed, S. Wu, Y. Zhang, X. Wu, F. Xia, G. Zhang and G. Gao, ChemCatChem, 2016, 8, 830 CrossRef CAS.
- U. R. Seo and Y. K. Chung, Green Chem., 2017, 19, 803 RSC.
- M. Lv, P. Wang, D. Yuan and Y. Yao, ChemCatChem, 2017, 9, 4451 CrossRef CAS.
- G. P. Speranza and W. J. Peppel, J. Org. Chem., 1958, 23, 1922 CrossRef CAS.
- J. E. Herweh, T. A. Foglia and D. Swern, J. Org. Chem., 1968, 33, 4029 CrossRef CAS.
- I. Shibata, A. Baba, H. Iwasaki and H. Matsuda, J. Org. Chem., 1986, 51, 2177 CrossRef CAS.
- T. Baronsky, C. Beattie, R. W. Harrington, R. Irfan, M. North, J. G. Osende and C. Young, ACS Catal., 2013, 3, 790 CrossRef CAS.
- C. Beattie and M. North, RSC Adv., 2014, 4, 31345 RSC.
- P. Wang, J. Qin, D. Yuan, Y. Wang and Y. Yao, ChemCatChem, 2015, 7, 1145 CrossRef CAS.
- J. Castro-Osma, A. Earlam, A. Lara-Sánchez, A. Otero and M. North, ChemCatChem, 2016, 8, 2100 CrossRef CAS.
- X. Wu, J. Mason and M. North, Chem.–Eur. J., 2017, 23, 12937 CrossRef CAS PubMed.
- Y. Toda, S. Gomyou, S. Tanaka, Y. Komiyama, A. Kikuchi and H. Suga, Org. Lett., 2017, 19, 5786 CrossRef CAS PubMed.
- H. Wang, Catalysts, 2019, 9, 244 CrossRef.
- N. Lu, Y. Fang, Y. Gao, Z. Wei, J. Cao, D. Liang, Y. Lin and H. Duan, J. Org. Chem., 2018, 83, 1486 CrossRef CAS PubMed.
- Y. Liu, J. Wang, Z. Wei, J. Cao, D. Liang, Y. Lin and H. Duan, Org. Lett., 2019, 21, 5719 CrossRef CAS.
- S. Paria, Q. Kang, M. Hatanaka and K. Maruoka, ACS Catal., 2019, 9, 78 CrossRef CAS.
- R. Craig, M. Litvajov, S. A. Cronin and S. J. Connon, Chem. Commun., 2018, 54, 10108 RSC.
- J. Z. hu, D. Cui, Y. Li, J. He, W. Chen and P. Wang, Org. Biomol. Chem., 2018, 16, 3012 RSC.
- Y. Li, D. Cui, J. Zhu, P. Huang, Z. Tian, Y. Jia and P. Wang, Green Chem., 2019, 11, 5231 RSC.
- J. Novacek and M. Waser, Eur. J. Org. Chem., 2014, 802 CrossRef CAS.
Footnotes |
† Electronic supplementary information (ESI) available. See DOI: 10.1039/d0ra00693a |
‡ Co-first authors. These two authors have the same contribution to this work. |
|
This journal is © The Royal Society of Chemistry 2020 |
Click here to see how this site uses Cookies. View our privacy policy here.