DOI:
10.1039/D0RA00356E
(Paper)
RSC Adv., 2020,
10, 10113-10117
Three-component reaction of azulene, aryl glyoxal and 1,3-dicarbonyl compound for the synthesis of various azulene derivatives†
Received
13th January 2020
, Accepted 27th February 2020
First published on 10th March 2020
Abstract
A three-component reaction of an azulene, an aryl glyoxal and a 1,3-dicarbonyl compound has been elaborated to access a series of azulene derivatives. Some of these azulene-containing adducts were further subjected to post-MCR transformations to assemble azulene–heterocycle conjugates.
Introduction
Azulene is a bicyclic aromatic hydrocarbon with a deep blue colour and a dipole moment of about 1.08 D.1 Such properties are in striking contrast with those of the isomeric naphthalene that is colourless and has a dipole moment of 0 D. The polarity of azulene and in turn the appearance of the blue colour can be explained by the charge-separated resonance structure in which the bicyclic core of azulene is regarded as a fusion of 6 π-electron cyclopentadienyl anion and 6 π-electron tropylium cation.
Owing to the unique structural and photophysical properties of the azulene core, a number of azulene-based advanced organic materials2 has been developed targeting the applications in sensors,3 bioimaging,4 non-linear optics (NLO),5 optoelectronics,6 molecular electronics7 and so on. Furthermore, azulene derivatives have been successfully incorporated in solar cells8 and organic field-effect transistors (OFETs)8c,d,9 demonstrating high potential for further exploration in this type of devices.
Consequently, this sparked a growing interest in the development of novel synthetic methodologies for azulene construction10 and functionalization11 with a special emphasis being given to the assembly of azulene-fused heterocycles,12 azulene–heterocycle conjugates13 and azulene-containing polymers.8a,c,d,14
Several recent methodologies for azulene functionalization involve one-pot and/or multicomponent approaches.15 On the other hand, in recent years, a number of multicomponent transformations have been developed based on the ability of aryl glyoxals to react with 1,3-dicarbonyl compounds and additional nucleophiles resulting in the formation of structurally diverse (heterocyclic) adducts.16 We decided to take an advantage of this strategy towards the synthesis of azulene derivatives through exploration of the nucleophilic potential of the five-membered ring of azulene core.
Results and discussion
Knowing that the treatment of an aryl glyoxal 2 with a 1,3-dicarbonyl compound 3 results in the Knoevenagel condensation,16 we envisaged that the presence of an azulene 1 would trigger the Michael addition of 1 onto the Knoevenagel adduct A. A subsequent proton transfer in the intermediate B would produce the desired azulene derivative 4 (Scheme 1). After conducting a brief screening of the reaction conditions (see ESI†), we were pleased to find that such a three-component transformation could be successfully accomplished at the elevated temperature of 80 °C using isopropanol as a solvent.
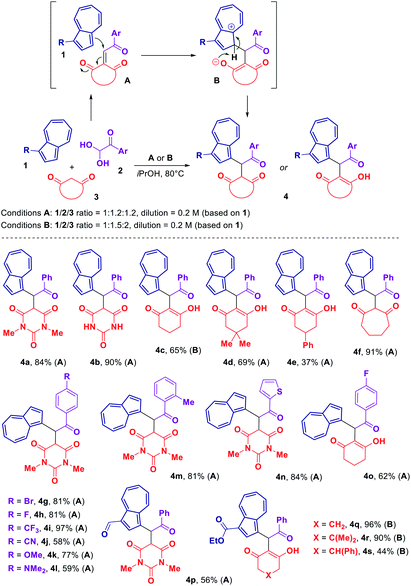 |
| Scheme 1 Scope of the three-component reaction of azulene 1, aryl glyoxal monohydrate 2 and 1,3-dicarbonyl compound 3. | |
The scope of the resulting process is outlined in Scheme 1. In order to evaluate the reactivity of a 1,3-dicarbonyl component 3, several barbituric acid derivatives and cyclic 1,3-diketones were reacted with unsubstituted azulene and phenyl glyoxal monohydrate resulting in the formation of products 4a–f with the yields ranging from 37% to 91%. Interestingly, according to NMR analysis, products 4a, b, f derived from either barbituric acids or cycloheptane-1,3-dione were observed in a keto form in solution (CDCl3 for 4a and 4f, [D6]DMSO for 4b). In contrast, products 4c–e obtained using various cyclohexane-1,3-diones were observed in a enolized form, with the compound 4e existing as a mixture of two interconvertible diastereomeric enol forms.
With respect to an aryl glyoxal component 2, a number of variously substituted phenyl glyoxal monohydrates along with a heteroaromatic thiophen-2-yl glyoxal monohydrate have been tested allowing to acquire an array of azulene-containing adducts 4g–o (Scheme 1). It was found that the presence of either electron-withdrawing or electron-donating substituent in the phenyl ring of glyoxal could be well tolerated.
Blocking one of the azulene's reactive positions with an electron-withdrawing group did not shut down the reactivity of the azulene core towards our transformation. Thus, we were able to prepare a series of 1,3-disubstituted azulene derivatives 4p–s starting from either azulene-1-carbaldehyde or ethyl azulene-1-carboxylate.
Considering that some of the obtained azulene derivatives, such as for example 4c and 4d comprised a 1,4-diketo unit, we decided to probe their reactivity in the condensations with nitrogen nucleophiles towards the formation of azulene–heterocycle conjugates. To our delight, reacting 4c and 4d with hydrazine monohydrate in methanol at rt produced azulene–tetrahydrocinnoline conjugates 5a and 5b in high yields of 94% and 93%, respectively (Scheme 2). Encouraged by these results, we went on exploring the potential of our 1,4-diketones in a Paal–Knorr synthesis of pyrroles.17 Gratifyingly, the treatment of 4c, 4d and 4o with aniline in isopropanol at 80 °C allowed to prepare azulene–dihydroindol-4-one conjugates 7a–c in moderate to good yields (Scheme 3). The molecular structure of representative azulene–dihydroindol-4-one derivative 7b has been resolved through the X-ray crystallographic analysis (Fig. 1, see ESI† for details). The above synthetic strategy was also found to be amenable to a variation of an amine component 6. Examining different aromatic and benzylic amines in the reactions with 4c or 4d delivered expected azulene-substituted dihydroindol-4-ones 7c–h in up to 93% yield (Scheme 3).
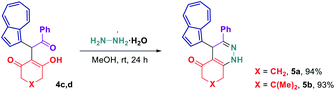 |
| Scheme 2 Synthesis of azulene–tetrahydrocinnolin-5-one conjugates 5. | |
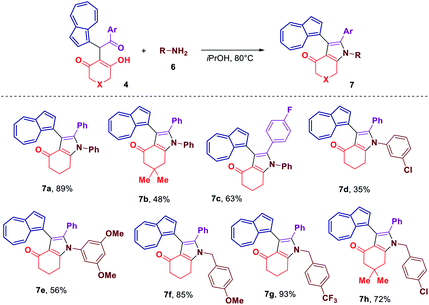 |
| Scheme 3 Synthesis of azulene–dihydroindol-4-one conjugates 7. | |
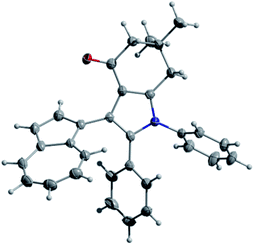 |
| Fig. 1 Molecular structure of 7b, showing thermal displacement ellipsoids at the 50% probability level. The dimethyl formamide (DMF) molecule acquired during the crystallization process and present in the crystal packing is not shown. | |
In an attempt to streamline the access towards azulene–heterocycle conjugates, we have conducted a one-pot synthesis of compounds 7a (Scheme 4). Reacting azulene (1a), phenyl glyoxal monohydrate (2a) and cyclohexane-1,3-dione (3c) in isopropanol at 80 °C for 1 h lead to the formation of acyclic adduct 4c. Once the formation of 4c was confirmed by the TLC analysis, the aniline (6a) was added and the reaction was continued for another 8 h allowing to obtain the desired azulene-substituted dihydroindol-4-one 7a in 51% overall yield.
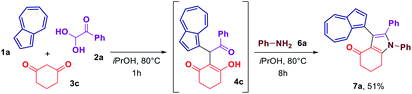 |
| Scheme 4 One-pot synthesis of azulene–dihydroindol-4-one conjugate 7a. | |
The optical properties of all acquired azulene derivatives 4, 5 and 7 have been assessed by measuring their UV/Vis absorption in dichloromethane and in methanol (both at c ≅ 5 × 10−6 M, see ESI†).‡ The UV/Vis absorption spectra of representative azulene-containing products 4d, 4r, 5b and 7b are shown in Fig. 2. Similarly to most of simple azulene derivatives, all prepared compounds 4, 5 and 7 were characterized by a strong absorbance in the UV region and a relatively weak absorbance in the visible region, with the latter being responsible for the colouration of their solutions.
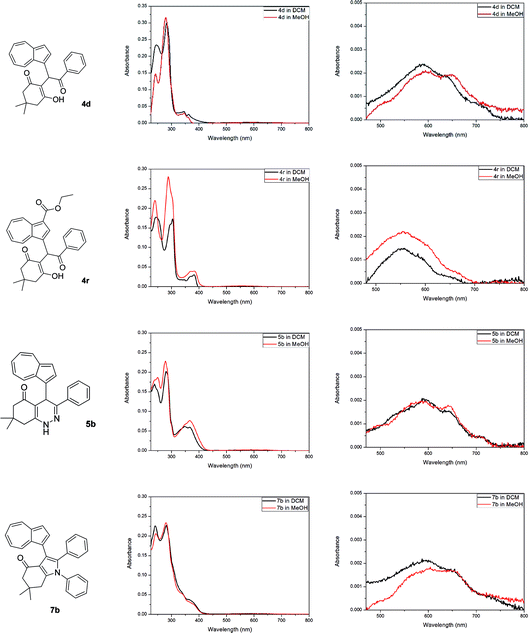 |
| Fig. 2 UV/Vis absorption spectra of 4d, 4r, 5b and 7b measured in dichloromethane and in methanol (both at c ≅ 5 × 10−6 M, left column); magnified visible region of UV/Vis absorption spectra of 4d, 4r, 5b and 7b (right column). | |
Conclusions
In conclusion, we have developed a novel multicomponent protocol for the azulene derivatization through the reaction with an aryl glyoxal and a 1,3-dicarbonyl compound. The scope of the process has been briefly explored resulting in generation of a small set of branched azulene-containing adducts. Some of these adducts could be further upgraded into azulene–heterocycle conjugates through the post-MCR condensations with nitrogen nucleophiles. Collectively, these methodologies provide a straightforward access to three distinct types of azulene derivatives.
Conflicts of interest
There are no conflicts to declare.
Acknowledgements
This work was supported by the start-up fund from Soochow University (grant Q410900714), National Natural Science Foundation of China (grant 21650110445), Natural Science Foundation of Jiangsu Province of China (grant BK20160310), the Priority Academic Program Development of Jiangsu Higher Education Institutions (PAPD), the Project of Scientific and Technologic Infrastructure of Suzhou (grant SZS201708) and the Social Policy Grant of Nazarbayev University.
Notes and references
-
(a) D. M. Lemal and G. D. Goldman, J. Chem. Educ., 1988, 65, 923 CrossRef CAS;
(b) A. G. Anderson Jr and B. M. Steckler, J. Am. Chem. Soc., 1959, 81, 4941 CrossRef.
-
(a) A. E. Ion, A. Dogaru, S. Shova, A. M. Madalan, O. Akintola, S. Ionescu, M. Voicescu, S. Nica, A. Buchholz, W. Plass and M. Andruh, CrystEngComm, 2018, 20, 4463 RSC;
(b) F. Wang, T. T. Lin, C. He, H. Chi, T. Tang and Y.-H. Lai, J. Mater. Chem., 2012, 22, 10448 RSC;
(c) K. Kurotobi, K. S. Kim, S. B. Noh, D. Kim and A. Osuka, Angew. Chem., Int. Ed., 2006, 45, 3944 CrossRef CAS PubMed.
-
(a) T. Tang, T. Lin, F. Erden, F. Wang and C. He, J. Mater. Chem. C, 2018, 6, 5153 RSC;
(b) E. H. Ghazvini Zadeh, A. W. Woodward, D. Richardson, M. V. Bondar and K. D. Belfield, Eur. J. Org. Chem., 2015, 2271 CrossRef CAS;
(c) E. Amir, R. J. Amir, L. M. Campos and C. J. Hawker, J. Am. Chem. Soc., 2011, 133, 10046 CrossRef CAS PubMed;
(d) H. Salman, Y. Abraham, S. Tal, S. Meltzman, M. Kapon, N. Tessler, S. Speiser and Y. Eichen, Eur. J. Org. Chem., 2005, 2207 CrossRef CAS.
-
(a) P. M. Gosavi, Y. S. Moroz and I. V. Korendovych, Chem. Commun., 2015, 51, 5347 RSC;
(b) Y. S. Moroz, W. Binder, P. Nygren, G. A. Caputod and I. V. Korendovych, Chem. Commun., 2013, 49, 490 RSC;
(c) W. Pham, R. Weissleder and C.-H. Tung, Angew. Chem., Int. Ed., 2002, 41, 3659 CrossRef CAS;
(d) L. C. Murfin, M. Weber, S. J. Park, W. T. Kim, C. M. Lopez-Alled, C. L. McMullin, F. Pradaux-Caggiano, C. L. Lyall, G. Kociok-Köhn, J. Wenk, S. D. Bull, J. Yoon, H. M. Kim, T. D. James and S. E. Lewis, J. Am. Chem. Soc., 2019, 141, 19389 CrossRef CAS PubMed.
-
(a) L. Cristian, I. Sasaki, P. G. Lacroix, B. Donnadieu, I. Asselberghs, K. Clays and A. C. Razus, Chem. Mater., 2004, 16, 3543 CrossRef CAS;
(b) P. G. Lacroix, I. Malfant, G. Iftime, A. C. Razus, K. Nakatani and J. A. Delaire, Chem.–Eur. J., 2000, 6, 2599 CrossRef CAS PubMed.
-
(a) T. Shoji, K. Miura, T. Araki, A. Maruyama, A. Ohta, R. Sekiguchi, S. Ito and T. Okujima, J. Org. Chem., 2018, 83, 6690 CrossRef CAS PubMed;
(b) T. Shoji and S. Ito, Chem.–Eur. J., 2017, 23, 16696 CrossRef CAS PubMed;
(c) H. Xin and X. Gao, ChemPlusChem, 2017, 82, 945 CrossRef CAS PubMed;
(d) J.-X. Dong and H.-L. Zhang, Chin. Chem. Lett., 2016, 27, 1097 CrossRef CAS.
-
(a) G. Yang, S. Sangtarash, Z. Liu, X. Li, H. Sadeghi, Z. Tan, R. Li, J. Zheng, X. Dong, J. Liu, Y. Yang, J. Shi, Z. Xiao, G. Zhang, C. Lambert, W. Hong and D. Zhang, Chem. Sci., 2017, 8, 7505 RSC;
(b) F. Schwarz, M. Koch, G. Kastlunger, H. Berke, R. Stadler, K. Venkatesan and E. Lörtscher, Angew. Chem., Int. Ed., 2016, 55, 11781 CrossRef CAS PubMed.
-
(a) E. Puodziukynaite, H.-W. Wang, J. Lawrence, A. J. Wise, T. P. Russell, M. D. Barnes and T. Emrick, J. Am. Chem. Soc., 2014, 136, 11043 CrossRef CAS PubMed;
(b) H. Nishimura, N. Ishida, A. Shimazaki, A. Wakamiya, A. Saeki, L. T. Scott and Y. Murata, J. Am. Chem. Soc., 2015, 137, 15656 CrossRef CAS PubMed;
(c) J. Yao, Z. Cai, Z. Liu, C. Yu, H. Luo, Y. Yang, S. Yang, G. Zhang and D. Zhang, Macromolecules, 2015, 48, 2039 CrossRef CAS;
(d) H. Xin, C. Ge, X. Jiao, X. Yang, K. Rundel, C. R. McNeill and X. Gao, Angew. Chem., Int. Ed., 2018, 57, 1322 CrossRef CAS PubMed.
-
(a) E. C. P. Smits, S. Setayesh, T. D. Anthopoulos, M. Buechel, W. Nijssen, R. Coehoorn, P. W. M. Blom, B. de Boer and D. M. de Leeuw, Adv. Mater., 2007, 19, 734 CrossRef CAS;
(b) P. H. Wöbkenberg, J. G. Labram, J.-M. Swiecicki, K. Parkhomenko, D. Sredojevic, J.-P. Gisselbrecht, D. M. de Leeuw, D. D. C. Bradley, J.-P. Djukic and T. D. Anthopoulos, J. Mater. Chem., 2010, 20, 3673 RSC;
(c) Y. Yamaguchi, Y. Maruya, H. Katagiri, K.-i. Nakayama and Y. Ohba, Org. Lett., 2012, 14, 2316 CrossRef CAS PubMed;
(d) Y. Yamaguchi, K. Ogawa, K.-i. Nakayama, Y. Ohba and H. Katagiri, J. Am. Chem. Soc., 2013, 135, 19095 CrossRef CAS PubMed;
(e) Y. Yamaguchi, M. Takubo, K. Ogawa, K.-i. Nakayama, T. Koganezawa and H. Katagiri, J. Am. Chem. Soc., 2016, 138, 11335 CrossRef CAS PubMed;
(f) H. Xin, C. Ge, L. Fu, X. Yang and X. Gao, Chin. J. Org. Chem., 2017, 37, 711 CrossRef CAS;
(g) H. Xin, C. Ge, X. Yang, H. Gao, X. Yang and X. Gao, Chem. Sci., 2016, 7, 6701–6705 RSC;
(h) H. Xin, J. Li, C. Ge, X. Yang, T. Xue and X. Gao, Mater. Chem. Front., 2018, 2, 975 RSC.
-
(a) D. D. Nolting, M. Nickels, R. Price, J. C. Gore and W. Pham, Nat. Protoc., 2009, 4, 1113 CrossRef CAS PubMed;
(b) N. R. Kumar, A. R. Agrawal and S. S. Zade, Chem.–Eur. J., 2019, 25, 14064 CrossRef CAS PubMed;
(c) H. Langhals and M. Eberspächer, Synthesis, 2018, 50, 1862 CrossRef CAS;
(d) V. Claus, M. Schukin, S. Harrer, M. Rudolph, F. Rominger, A. M. Asiri, J. Xie and A. S. K. Hashmi, Angew. Chem., Int. Ed., 2018, 57, 12966 CrossRef CAS PubMed.
-
(a) M. Fujinaga, K. Suetake, K. Gyoji, T. Murafuji, K. Kurotobi and Y. Sugihara, Synthesis, 2008, 3745 CAS;
(b) J. Dubovik and A. Bredihhin, Synthesis, 2015, 47, 538 CAS;
(c) A. Székely, Á. Péter, K. Aradi, G. L. Tolnai and Z. Novák, Org. Lett., 2017, 19, 954 CrossRef PubMed;
(d) T. Shoji, T. Araki, N. Iida, Y. Kobayashi, A. Ohta, R. Sekiguchi, S. Ito, S. Mori, T. Okujima and M. Yasunami, Eur. J. Org. Chem., 2018, 1145 CrossRef CAS;
(e) X. Shi, A. Sasmal, J.-F. Soulé and H. Doucet, Chem.–Asian J., 2018, 13, 143 CrossRef CAS PubMed.
-
(a) C. Kogawa, A. Fujiwara, R. Sekiguchi, T. Shoji, J. Kawakami, M. Okazaki and S. Ito, Tetrahedron, 2018, 74, 7018 CrossRef CAS;
(b) T. Shoji, T. Araki, N. Iida, K. Miura, A. Ohta, R. Sekiguchi, S. Ito and T. Okujima, Org. Chem. Front., 2019, 6, 195 RSC;
(c) T. Shoji, K. Miura, A. Ohta, R. Sekiguchi, S. Ito, Y. Endo, T. Nagahata, S. Mori and T. Okujima, Org. Chem. Front., 2019, 6, 2801 RSC;
(d) H. Xin, J. Li, X. Yang and X. Gao, J. Org. Chem., 2020, 85, 70 CrossRef PubMed.
-
(a) A. C. Razus, L. Birzan, A. Corbu, O. Zaharia and C. Enache, ARKIVOC, 2006,(xii), 121 Search PubMed;
(b) T. Shoji, S. Takagaki, M. Tanaka, T. Araki, S. Sugiyama, R. Sekiguchi, A. Ohta, S. Ito and T. Okujima, Heterocycles, 2017, 94, 1870 CrossRef CAS;
(c) T. Shoji, M. Tanaka, S. Takagaki, K. Miura, A. Ohta, R. Sekiguchi, S. Ito, S. Mori and T. Okujima, Org. Biomol. Chem., 2018, 16, 480 RSC.
-
(a) F. Wang, Y.-H. Lai and M.-Y. Han, Macromolecules, 2004, 37, 3222 CrossRef CAS;
(b) X. Wang, J. K.-P. Ng, P. Jia, T. Lin, C. M. Cho, J. Xu, X. Lu and C. He, Macromolecules, 2009, 42, 5534 CrossRef CAS;
(c) K. Tsurui, M. Murai, S.-Y. Ku, C. J. Hawker and M. J. Robb, Adv. Funct. Mater., 2014, 24, 7338 CrossRef CAS.
-
(a) N. Takenaga, K. Fukazawa, M. Maruko and K. Sato, Heterocycles, 2015, 90, 113 CrossRef CAS;
(b) C. F. Gers, J. Rosellen, E. Merkul and T. J. J. Müller, Beilstein J. Org. Chem., 2011, 7, 1173 CrossRef CAS PubMed.
-
(a) J. Khalafy, M. Rimaz, M. Ezzati and R. H. Prager, Bull. Korean Chem. Soc., 2012, 33, 2890 CrossRef CAS;
(b) G.-H. Ma, X.-J. Tu, Y. Ning, B. Jiang and S.-J. Tu, ACS Comb. Sci., 2014, 16, 281 CrossRef CAS PubMed;
(c) V. A. Peshkov, A. A. Peshkov, O. P. Pereshivko, K. Van Hecke, L. L. Zamigaylo, E. V. Van der Eycken and N. Yu. Gorobets, ACS Comb. Sci., 2014, 16, 535 CrossRef CAS PubMed;
(d) H. Wei, B. Li, G. Wang, K. Van Hecke, O. P. Pereshivko and V. A. Peshkov, Synthesis, 2016, 48, 1734 CrossRef CAS;
(e) X. Yang, L. Zheng, Z. Chen and W. Zhong, Synth. Commun., 2018, 48, 929 CrossRef CAS;
(f) X. Chang, X. Zhang and Z. Chen, Org. Biomol. Chem., 2018, 16, 4279 RSC;
(g) M. Saroha and J. M. Khurana, New J. Chem., 2019, 43, 8644 RSC;
(h) V. G. Melekhina, V. S. Mityanov, B. V. Lichitsky, A. N. Komogortsev, A. N. Fakhrutdinov, E. D. Daeva and M. M. Krayushkin, Tetrahedron Lett., 2019, 60, 1745 CrossRef CAS;
(i) B. V. Lichitsky, A. D. Tretyakov, A. N. Komogortsev, V. S. Mityanov, A. A. Dudinov and M. M. Krayushkin, Chem. Heterocycl. Compd., 2019, 55, 156 CrossRef CAS.
- A. Balakrishna, A. Aguiara, P. J. M. Sobral, M. Y. Wani, J. Almeida e Silva and A. J. F. N. Sobral, Catal. Rev.: Sci. Eng., 2019, 61, 84 CrossRef CAS.
Footnotes |
† Electronic supplementary information (ESI) available: Detailed experimental procedures, products characterization, X-ray crystallographic data and copies of UV/vis absorption and NMR spectra. CCDC 1974555. For ESI and crystallographic data in CIF or other electronic format see DOI: 10.1039/d0ra00356e |
‡ UV/Vis absorption of 7b was measured only in methanol due to poor solubility in dichloromethane. |
|
This journal is © The Royal Society of Chemistry 2020 |
Click here to see how this site uses Cookies. View our privacy policy here.