DOI:
10.1039/D0RA00315H
(Paper)
RSC Adv., 2020,
10, 7764-7770
Mold resistance of bamboo after laccase-catalyzed attachment of thymol and proposed mechanism of attachment
Received
11th January 2020
, Accepted 10th February 2020
First published on 26th February 2020
Abstract
Laccase-catalyzed attachment of functional molecules onto the surface of bamboo represents an alternative, green approach to improve performance. Although treatment of bamboo with thymol improved resistance to mold, using laccase to fix the same concentration of thymol to the surface of the bamboo could increase both the antifungal activity and resistance to leaching. Leaching of thymol was reduced by as much as 48.4% when laccase was used in thymol fixation. To make clear the mechanisms of fixation, reaction of thymol catalyzed with laccase, was investigated using Fourier-transform infrared spectroscopy, 1H-NMR, high-resolution mass spectrometry and thermogravimetric analysis, respectively. Results show that thymol oligomer (L-thymol) was formed with ether linkages, which resist water leaching. Although further confirmatory studies are needed, it seems that ether linkages were the main connection of thymol to lignin. This study demonstrates that laccase catalysis is a promising strategy to functionalize the surface of bamboo in order to bestow new properties suitable for a wide range of applications.
1. Introduction
Bamboo, which grows faster than any woody plant,1 is a low-carbon, renewable, easy-to-grow resource. Because of its high strength, toughness, abrasion resistance and ability to withstand heat,2,3 bamboo is extremely versatile and has countless uses, ranging from basic tools to building materials.4 Bamboo is, however, susceptible to attack by mold-causing fungi,5–7 which is a particular problem in products such as chopsticks, cutting boards and packages that come into contact with food.8 Products that come into contact with food directly are washed frequently, which increases the likelihood of attack by mold fungi. Traditional wood preservatives, which included creosote, pentachlorophenol, iodopropynyl butylcarbamate, and chlorothalonil, are mostly highly toxic synthetic chemicals.9,10 Because of their negative effects on both the environment and human health, the application of many popular wood preservatives have now been phased out or have had their use restricted.11 This has led to intense efforts to find alternative, natural preservatives that are nontoxic and do not damage the environment.12,13 Thymol (2-isopropyl-5-methylphenol) as a naturally occurring antimicrobial phenol, is the principal component of thymol essential oil,14,15 which has been extracted in large scale. Studies have shown that thymol has broad-spectrum antifungal activity, and could potentially prevent the growth of molds on food and bamboo16–20 and it has been ratified by the United States Food and Drug Administration (FDA) as a safe food additive.21,22 The industrial production of food grade thymol can be extracted by means of supercritical CO2 fluid extraction (SFE) which is the most green and efficient technique to extract natural compounds.22,23 Although the possibility of using natural antifungal phenols, such as thymol, tannins, and eugenol,11 as wood preservatives has been explored, the high leachability of thymol decreases its effectiveness.24,25 Enzymatic functionalization of wood surfaces, either for aesthetic purposes or for preservation, has recently attracted much interest, both from academia and industry.26,27 Laccase (EC 1. 10. 3. 2) which belongs to the family of blue multi-copper oxidases and is found predominantly in white rot fungi, plays a major role in the degradation of lignin.28,29 Laccase can catalyze the oxidation of many phenols, anilines and their derivatives.30,31 Oxygen is needed for the reaction, and, since water is the only by-product (Fig. 1),32–34 laccase is considered to be a green and eco-friendly biocatalyst. Laccase-mediated attachment of natural phenols to the surface of bamboo has been proposed as an efficient way to circumvent leaching of antimicrobial phenolic compounds, without loss of efficacy.24 This study is intended to find a green and eco-friendly way to protect bamboo against mold, and to reduce leaching of thymol using laccase. The assay was schemed in Fig. 1. We also wanted to study the mechanism by which laccase fixes thymol to bamboo.
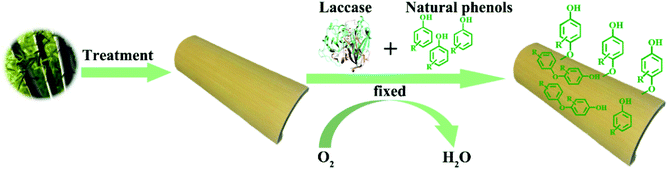 |
| Fig. 1 Schematic diagram of laccase-catalyzed fixation of natural antimicrobial phenol to bamboo. | |
2. Materials and methods
2.1 Materials
Samples of 5 year-old bamboo, species Phyllostachys pubescens, were collected from Xuan Cheng, An Hui province, China. The epidermis and endodermis of the culm were planed off and machined into blocks with the dimension of 50 mm (longitudinal) × 20 mm (tangential) × 5 mm (radial).
Aspergillus niger (A. niger) (isolation number: GDMCC 3.411) was used as the test fungus. The fungus was provided by Guangdong Institute of Microbiology (Guangzhou, Guangdong Province, China), and grown on potato dextrose agar (final pH, 5.6 ± 2) at 25–28 °C and 85 ± 5% relative humidity in culture medium as previously described.
2,2′-Azino-bis(3-ethylbenzothiazoline-6-sulfonic acid) (ABTS) and Trametes versicolor laccase (U ≥ 0.5 U mg−1, powder) were purchased from Sigma-Aldrich (Shanghai, China); analytical reagent thymol (purity > 99%), sodium acetate and glacial acetic acid were purchased from Sinopharm Chemical Reagent Co., Ltd. (Shanghai, China).
2.2 Determination of laccase activity
Laccase activity was determined by measuring the absorbance of ABTS at 420 nm using a UV-Vis spectrophotometer, (UV-2600, Shimadzu), with constant concentrations of laccase (0.5 mg mL−1) and ABTS (0.5 mmol L−1). Enzymatic activity (U) was defined as the amount of enzyme needed to catalyze the production of 1 μ mol of oxidized product per minute. All measurements were carried out in triplicate.35
The effects of pH, temperature and ethanol concentration on laccase activity were investigated in acetate/sodium acetate buffer system at a concentration of 2 mol L−1. Firstly, laccase activity was tested at designed temperature 25 °C and varying pH from 4 to 5.5. Next, the pH of the buffer was fixed at 4.5 and reaction temperature was varied from 25 to 45 °C. Finally, after the optimal reaction temperature and pH were selected as 4.5 and 40 °C, respectively, the effect of ethanol concentration on the activity of laccase was determined, which is designed as follows: 0%, 10%, 15%, 20% and 25% volume percentage in the buffer.
2.3 Impregnation of bamboo test blocks
Treatment solutions were prepared by dissolving thymol alone, or a combination of thymol and laccase, in a mixture of anhydrous ethanol and buffer solution and anhydrous ethanol has a volume fraction of 20%. The solution contained 0.22 wt% thymol was termed 0.22Thy. Thymol/laccase solutions, containing fixed amounts of laccase and ABTS (Table 1) and different amounts of thymol (0.10 wt%, 0.15 wt% and 0.22 wt%) were termed L-0.10Thy, L-0.15Thy and L-0.22Thy, respectively. Bamboo blocks were vacuum treated under 0.1 MPa and impregnated in the treatment solution for 24 h at 40 °C. The test samples were then removed from the treatment solution and vacuum dried at 40 °C, for 72 h.
Table 1 Conditions for processing test blocks
Sample |
Process |
Formulation of treatment solution |
Laccase (U mL−1) |
ABTS (mmol L−1) |
Thymol (wt%) |
Untreated |
— |
— |
— |
— |
0.22Thy |
Unleached |
0.06 |
0.5 |
0.22 |
L-0.10Thy |
0.1 |
L-0.15Thy |
0.15 |
L-0.22Thy |
0.22 |
0.22Thy/L |
Leached |
0.22 |
L-0.10Thy/L |
0.1 |
L-0.15Thy/L |
0.15 |
L-0.22Thy/L |
0.22 |
Retention of thymol in each block was calculated using the equation:
where:
m1 is the weight (g) of bamboo block before impregnation,
m2 is the weight (g) of bamboo block after impregnation,
m2 −
m1 is the weight (g) of treatment solution absorbed by block,
C is the concentration of thymol in treatment solution (g per 100 g),
H is the thickness of test block, (mm),
L is the length of test blocks, (mm),
W is the width of test block (mm).
2.4 Leaching of test blocks
Tests were carried out in accordance with AWPA E11-12 Standard Method of Accelerated Evaluation of Preservative Leaching.36 Test blocks impregnated with 0.22Thy, L-0.10Thy, L-0.15Thy and L-0.22Thy (Table 1) were completely submerged in deionized water. After 6, 24, 48 and thereafter at 48 hours intervals, the leachate was removed and replaced with an equal amount of fresh deionized water (50 mL per test block). Six replicates were carried out for each experiment. After leaching, the test blocks were removed from the leaching solution and vacuum dried at 40 °C for 3 days. After leaching, the test blocks were designated L-0.10Thy/L, L-0.15Thy/L and L-0.22Thy/L, respectively. The ratio of thymol leached from blocks was calculated as follows.
where: A is the total weight of thymol in leachate (mg), B is total weight of thymol in leached blocks (mg).
2.5 Mold resistance test
Mold resistance tests on treated bamboo samples were carried out according to the ASTM D4445-10 (2015) “Standard Test Method for Fungicides for Controlling Sapstain and Mold in Unseasoned Lumber (Laboratory Method)”.37 After inoculating bamboo specimens with 0.25 mL spore suspension, the Petri dishes were placed in the incubator and incubated at 25 °C for 4 weeks. The percentage of fungal growth on the block was visually estimated and scaled into 5 grades according to the standard methods. The Resisting efficiency (RE) of test blocks against A. niger was calculated as follows:
where: P1 is the percentage of the fungal growth on the test block, P0 is the percentage of the fungal growth on the untreated controls.
2.6 Fixation mechanism of anti-mold ingredient
It has been reported that laccase can catalyze phenols into polyphenols,38 which may improve both the leaching resistance and the antifungal effects. To explore the reaction of thymol, thymol (0.22 wt%) were allowed to react at 40 °C for 24 h, with laccase (2.0 mg mL−1) as catalyst and ABTS (0.5 mmol L−1) as the medium. The resulting mixture was centrifuged at 8000 rpm for 8 min and the supernatant was removed. The remaining solid was washed with distilled water three times to remove laccase and unreacted ABTS, followed by anhydrous ethanol washing to remove the excess thymol (Fig. 2). The product, termed L-thymol, was vacuum dried at 40 °C for 48 h. The yield of L-thymol was calculated as follows:
where: m0 is the initial weight of thymol added (g), m1 is the weight of L-thymol after reaction (g).
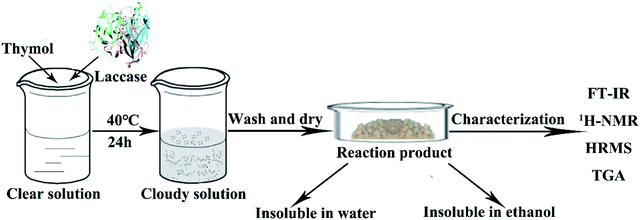 |
| Fig. 2 Preparation and characterization of laccase-catalyzed thymol reaction product. | |
2.7 Characterization
2.7.1 Fourier-transform infrared (FT-IR) spectroscopy. FT-IR spectra of the dried thymol and L-thymol were recorded using a 6700 FT-IR (Nicolet, USA). Spectra were recorded over the range 500–4000 cm−1, at a resolution of 8 cm−1 and with 32 scans.
2.7.2 Thermogravimetry/derivative thermogravimetry (TG/DTG). Thermogravimetric analysis was carried out under an atmosphere of nitrogen using a Sensys thermogravimetric analyzer (Setaram, France) over the temperature range 25–800 °C with a heating rate of 10 °C min−1.
2.7.3 1H-NMR spectroscopy. 1H-NMR spectra were recorded at 500 MHz using a Bruker AVANCE III 400 WB spectrometer (Bruker BioSpin AG, Fällanden, Switzerland). Chemical shifts were reported in ppm relative to residual deuterated solvent or to an internal standard (tetramethylsilane, TMS).
2.7.4 High-resolution mass spectrometry (HRMS). Compounds were detected on a Q-Exactive Orbitrap Mass Spectrometer (Thermo Fisher, CA, USA) equipped with a heated electrospray ionization probe. In addition, the mass spectrometry was performed in both positive and negative ionization modes.
3. Results and discussion
3.1 Enzyme activity
In previous studies, the laccase activity was measured in buffer solution, without organic solvents.39 Here, to achieve adequate solubility of thymol, we wanted to use a mixture of ethanol and buffer solution. Since enzymatic activity was expected to be different in the mixed solvent, we first measured laccase activity under different conditions in order to choose an appropriate level of enzyme activity for subsequent experiments. The effects of pH, temperature and proportion of ethanol in the mixed solvent on laccase activity are shown in Fig. 3.
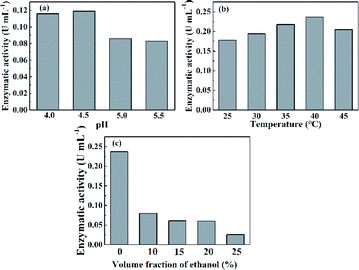 |
| Fig. 3 Effect of (a) pH, (b) temperature, and (c) volume fraction of ethanol on laccase activity. | |
When the pH of the reaction solution was increased from 4 to 4.5, laccase activity showed a slight upward trend (Fig. 3a), but when the pH was increased further, laccase activity was markedly reduced. The highest laccase activity, 0.119 U mL−1, was observed at pH 4.5, and this pH value was, therefore, chosen for further experiments.
Laccase activity increased steadily as the temperature was increased from 25 °C to 40 °C, and then decreased slightly when the temperature was further increased to 45 °C (Fig. 3b). The highest enzyme activity, 0.237 U mL−1 was achieved at a temperature of 40 °C, in agreement with a study by Chenghua Yu's.40
Addition of ethanol to the buffer markedly and concentration-dependently reduced laccase activity (Fig. 3c). Laccase activity, at 0.08 U mL−1, was highest with the lowest amount (10%) of added ethanol. When the amount of ethanol was increased to 15%, 20% and 25%, enzyme activity fell to 0.061 U mL−1, 0.06 U mL−1 and 0.026 U mL−1, respectively. In order to achieve a sufficiently high concentration of thymol and, at the same time, achieve adequate laccase activity, buffer containing 20% ethanol was chosen as the solvent.
Based on the experiments described above, the following conditions were chosen for the laccase-catalyzed reaction: pH, 4.5, temperature, 40 °C, volume fraction of ethanol, 20%.
3.2 Mold resistance and rate of leaching
Thymol, the main monoterpene phenol in essential oils extracted from some plants, has been shown to have significant antibacterial and antifungal activity.17 To explore the potential use of thymol in protection of bamboo against mold, and also to investigate the role of laccase catalysis in the fixation of thymol, tests were conducted using A. niger in accordance with AWPA E24-06 “Standard Method of Evaluating the Resistance of Wood Product Surfaces to Mold Growth”. Results in Fig. 4a showed that untreated bamboo blocks were all covered with mycelium on the 7th day, and the resisting efficiency declined rapidly to 0 (Fig. 4a). Bamboo blocks treated with thymol alone (0.22 wt%) restrained the growth of mold fungi to some extent, with slowed fungal growth. But, on the 20th day, the protective effect had disappeared and the resisting efficiency dropped to 0. Laccase-catalyzed treatment with thymol improved mold resistance, especially at thymol concentrations of 0.15 wt% and 0.22 wt% (Fig. 4a). Compared with treatment with thymol alone, laccase-catalyzed fixation of thymol greatly improved the ability of thymol to prevent fungal growth on the bamboo blocks.
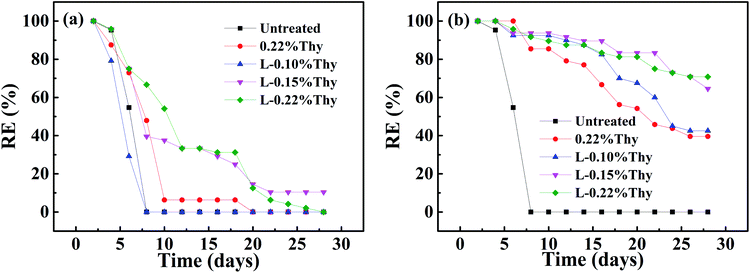 |
| Fig. 4 (a) Resisting efficiency against mold caused by A. niger, (b) resisting efficiency of different treatments after leaching. | |
Bamboo products, such as chopsticks, chopping boards and containers are frequently washed, which could lead to loss of antifungal agents, hence, the leaching experiments were conducted to determine whether the use of laccase catalysis during treatment with thymol protects the antifungal agent from leaching. After leaching for 14 days, thymol alone (0.22 wt%) inhibited the growth of mold fungi on the bamboo blocks, but after 28 days, the resisting efficiency was down to 39.6% (Fig. 4b). The use of laccase catalysis during treatment with thymol increased the duration of the antifungal effect, especially when the concentration of thymol reached 0.22wt%. Compared with 0.22Thy treatment of bamboo, the resisting efficiency of L-0.22Thy treatment increased by 31.2% on the 28th day. The enhanced antifungal effect is attributed to the reduced leaching of thymol from the bamboo. After treatment with 0.22Thy and L-0.22Thy, the rates of thymol leaching from bamboo were 75.2% and 26.8%, respectively (Table 2). Treatment of bamboo with thymol in the presence of laccase thus greatly enhanced both the antifungal effect and leaching resistance of the thymol, accordingly afforded better protection against mold.
Table 2 Retention of thymol and rate of leaching from treated blocks
Samples |
Retention (g m−2) |
Rate of leaching (%) |
0.22Thy |
194.6 |
75.2 |
L-0.22Thy |
214.5 |
26.8 |
3.3 Mechanism of improved antifungal activity and resistance to leaching
To understand the reaction of thymol that is catalyzed by laccase, and thus potentially explain the improved antifungal activity and leaching resistance, thymol was in vitro reacted with laccase in the presence of ABTS. The newly obtained product (termed L-thymol) was washed with water and ethanol in turn to remove the unreacted laccase and thymol. The amount of insoluble yield was 32%, which will be characterized by different methods.
3.3.1 Analysis by Fourier-transform infrared spectroscopy. According to the experimental results of leaching, treatment of bamboo with thymol in the presence of laccase greatly enhanced both the antifungal effect and leaching resistance of the thymol. It can be inferred that there must be some new binding forces which could reduce the rate of leaching. According to many literatures, the binding forces were probably resulted from the chemical combination of bamboo lignin and thymol,26,27 self-polymerization of thymol to form a more stable product,41–43 or both. Thymol, under the reaction of laccase catalytic system, therefore, may exist in bamboo in the following three forms: (1) primordial thymol, (2) thymol polymer, (3) a combination with lignin. Considering the steric hindrance of the chemical reaction and the content of phenolic lignin in bamboo,44 thymol self-polymerization is more likely. To elucidate the structure of L-thymol, and potentially the mechanism underlying the improved antifungal activity and resistance to leaching when bamboo is treated with thymol in the presence of laccase, the FT-IR spectra of thymol and L-thymol was analyzed (Fig. 5).
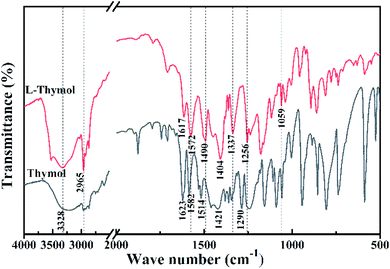 |
| Fig. 5 FT-IR of thymol (black) and L-thymol (red). | |
The peak at 2965 cm−1, attributed to the C–H stretching vibration of methyl groups,45 becomes sharper in L-thymol than in thymol, indicating a change that methyl group gets stronger. A change also occurred between 1256 cm−1 and 1059 cm−1, which is the C–O stretching vibrations of ether linkages.46 The presence of a phenolic C–O stretching vibration at 1337 cm−1 indicated that some of the phenolic hydroxyl groups had reacted to form ether bonds. The peaks attributed to the aromatic skeleton47 at 1617, 1572, 1490 and 1404 cm−1, were all smaller than the corresponding peaks in thymol, indicating that conjugation had increased. Additionally, the peaks attributed to the benzene ring skeleton of thymol, close to 1617 cm−1 and 1404 cm−1, also changed significantly, indicating changes in the skeleton of thymol.
3.3.2 Thermogravimetric analysis. The thermal behaviors of thymol and L-thymol were investigated using a thermo gravimetric analyzer. The decomposition temperature of thymol was 226 °C, while that of L-thymol was 358 °C (Fig. 6), indicating the presence of additional chemical bonds in L-thymol which increases the thermal stability of the product. Further speculation suggested that polymerization of thymol occurred as it had been reported in other phenolic chemicals.41
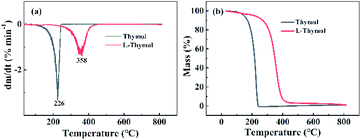 |
| Fig. 6 DTG mapping (a) and TG mapping (b) of thymol and L-thymol. | |
3.3.3 1H-NMR analysis. Analyses of the 1H-NMR spectra of thymol and L-thymol are shown in Fig. 7, 8 and Table 3. The peak at δ = 7.29 ppm is the residual solvent peak of CDCl3. Comparing peak area of thymol with those of L-thymol, the peaks attributed to R–CH3 (δ 1.30) and Ar–CH3 (δ 2.32) were almost unchanged, indicating that methyl groups in thymol are not involved in the reaction catalyzed by laccase. The integral integrated areas of Ar–H (δ 6.61, 6.78 and 7.14) and Ar–OH (δ 4.71) peaks in the L-thymol were smaller than the corresponding peaks in thymol, indicating that reaction occurred at the –OH groups of Ar–OH and Ar–H also participated in the reaction. Combining the results from FT-IR and TG with 1H-NMR, it is suggested that the reaction of thymol into L-thymol involves the formation of ether linkages.42
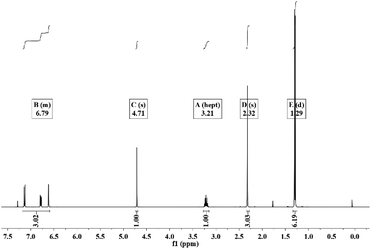 |
| Fig. 7 1H-NMR spectra of thymol. | |
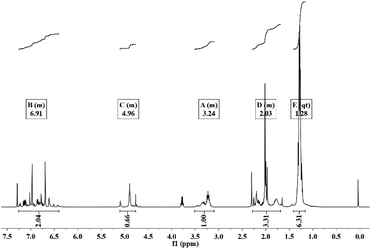 |
| Fig. 8 1H-NMR spectra of L-thymol. | |
Table 3 Chemical shifts and integrated areas of different types of hydrogen atom
Groups |
δ (ppm) |
Integrated area |
Thymol |
L-Thymol |
R–CH3 |
1.3 |
6.19 |
6.31 |
Ar–CH3 |
2.32 |
3.03 |
3.31 |
R3–CH |
3.21 |
1 |
1 |
Ar–OH |
4.71 |
1 |
0.66 |
ArH |
6.61, 6.78, 7.14 |
3.02 |
2.04 |
3.3.4 Analysis by high-resolution mass spectrometry. The high-resolution mass spectrum of L-thymol showed peaks at m/z 299.2 and 300.2 (Fig. 9). Since the molecular weight of thymol is 150.22, the reaction product is presumed to be a dimer.
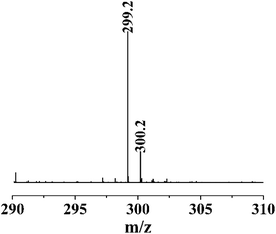 |
| Fig. 9 High-resolution mass spectrum of L-thymol. | |
Based on these analyses, a reaction scheme for the reaction of thymol catalyzed by laccase can be proposed (Fig. 10). Firstly, thymol was catalyzed by laccase to form phenoxy radicals.42 Two possible types of active phenoxy radicals were formed and began to initiate the polymerization of thymol. In addition, the laccase catalyzed polymerization of thymol is the main reason for the increase of leachability of thymol in bamboo.
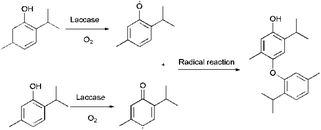 |
| Fig. 10 Mechanism of thymol dimerization catalyzed by laccase. | |
4. Conclusion
Bamboo treated with thymol in the presence of laccase had higher resistance to mold than bamboo treated with thymol alone, even after leaching, which indicated that there must be some new binding forces which could reduce the rate of leaching. Further studies on the mechanisms of thymol fixation showed that laccase is able to catalyze dimerization of thymol by formation of ether linkages. Based on the results, we speculate that thymol bonded by laccase to lignin in the bamboo in a similar way which will be revealed in the following work. The mechanism of oxidative attachment of thymol to bamboo using laccase is, however, not yet clear and this will be addressed in future studies.
Conflicts of interest
There are no conflicts to declare.
Acknowledgements
The authors acknowledge the funding support from Key Natural Science Foundation of Zhejiang Province (Grants No. LZ20C160004), the Natural Science Foundation of Zhejiang Province (Grants No. LQ20C160006), the Zhejiang Provincial Key Research & Development Project (Grants No. 2019C02037) and the Science and Technology Innovative Program for college students of Zhejiang Province (new-shoot Talents Program) (Grants No. 2019R412047).
References
- Z. Yuan, Y. Wen and N. S. Kapu, Bioresour. Technol., 2018, 247, 242–249 CrossRef CAS PubMed
. - V. Puri, P. Chakrabortty, S. Anand and S. Majumdar, J. Build. Eng., 2017, 9, 52–59 CrossRef
. - P. Dua, S. Satya, K. K. Pant, S. N. Naik and V. Kardam, Holz Roh- Werkst., 2016, 74, 1–4 CrossRef
. - Y. Okahisa, T. Yoshimura and Y. Imamura, J. Wood Sci., 2005, 51, 542–545 CrossRef CAS
. - F. Sun, B. Bao, L. Ma, A. Chen and X. Duan, J. Wood Sci., 2012, 58, 51–56 CrossRef CAS
. - T. Tang, O. Schmidt and W. Liese, J. Trop. For. Sci., 2012, 285–290 Search PubMed
. - X.-D. Huang, C.-Y. Hse and T. F. Shupe, BioResources, 2013, 9, 497–509 CrossRef
. - N. K. Prosper, S. Zhang, H. Wu, S. Yang, S. Li, F. Sun and B. Goodell, Wood Sci. Technol., 2018, 52, 619–635 CrossRef CAS
. - B. D. Mattos, B. L. Tardy, W. L. Magalhaes and O. J. Rojas, J. Controlled Release, 2017, 262, 139–150 CrossRef CAS PubMed
. - P. r. Annica, A. Gry and H. Ari, Holzforschung, 2010, 64, 645–651 Search PubMed
. - M. Rättö, A.-C. Ritschkoff and L. Viikari, Holzforschung, 2004, 58, 440–445 Search PubMed
. - T. Singh and A. P. Singh, Wood Sci. Technol., 2012, 46, 851–870 CrossRef CAS
. - C. Croitoru, C. Spirchez, A. Lunguleasa, D. Cristea and A. Pascu, Appl. Surf. Sci., 2018, 438, 114–126 CrossRef CAS
. - M. Asprea, I. Leto, M. C. Bergonzi and A. R. Bilia, LWT, 2017, 77, 497–502 CrossRef CAS
. - T. Zhong, Y. Liang, S. Jiang, L. Yang, Y. Shi, S. Guo and C. Zhang, RSC Adv., 2017, 7, 41610–41618 RSC
. - M. Behpour, S. Masoum and M. Meshki, RSC Adv., 2014, 4, 14270 RSC
. - S. Ribes, M. Ruiz-Rico, É. Pérez-Esteve, A. Fuentes, P. Talens, R. Martínez-Máñez and J. M. Barat, Food Control, 2017, 81, 181–188 CrossRef CAS
. - T. Bollenbach, Curr. Opin. Microbiol., 2015, 27, 1–9 CrossRef CAS PubMed
. - A. Ahmad, A. Khan, F. Akhtar, S. Yousuf, I. Xess, L. Khan and N. Manzoor, Eur. J. Clin. Microbiol. Infect. Dis., 2011, 30, 41–50 CrossRef CAS PubMed
. - K. Wang, S. Jiang, T. Pu, L. Fan, F. Su and M. Ye, Nat. Prod. Res., 2019, 33, 1423–1430 CrossRef CAS PubMed
. - N. F. S. Morsy, Ind. Crops Prod., 2020, 145, 1–7 CrossRef
. - S. Milovanovic, M. Stamenic, D. Markovic, J. Ivanovic and I. Zizovic, J. Supercrit. Fluids, 2015, 97, 107–115 CrossRef CAS
. - S. Abbaszadeh, A. Sharifzadeh, H. Shokri, A. Khosravi and A. Abbaszadeh, J. Mycol. Med., 2014, 24, e51–e56 CrossRef CAS PubMed
. - D. Filgueira, D. Moldes, C. Fuentealba and D. García, Ind. Crop. Prod., 2017, 103, 185–194 CrossRef CAS
. - M. Eikenes, G. Alfredsen, B. r. E. Christensen, H. Militz and H. Solheim, J. Wood Sci., 2005, 51, 387–394 CrossRef CAS
. - T. Kudanga, E. N. Prasetyo, J. Sipilä, G. S. Nyanhongo and G. M. Guebitz, Enzyme Microb. Technol., 2010, 46, 272–280 CrossRef CAS
. - K. Thakur, S. Kalia, B. S. Kaith, D. Pathania and A. Kumar, RSC Adv., 2015, 5, 76844–76851 RSC
. - M. Fernández-Fernández, M. Sanromán and D. Moldes, Wood Sci. Technol., 2014, 48, 151–160 CrossRef
. - V. Madhavi and S. Lele, BioResources, 2009, 4, 1694–1717 Search PubMed
. - J. Polak and A. Jarosz-Wilkolazka, Process Biochem., 2012, 47, 1295–1307 CrossRef CAS
. - J. Jiang, W. Ye, L. Liu, Z. Wang, Y. Fan, T. Saito and A. Isogai, Biomacromolecules, 2016, 18, 288–294 CrossRef PubMed
. - T. Kudanga, B. Nemadziva and M. Le Roes-Hill, Appl. Microbiol. Biotechnol., 2017, 101, 13–33 CrossRef CAS PubMed
. - S. Riva, Trends Biotechnol., 2006, 24, 219–226 CrossRef CAS PubMed
. - M. Sdahl, J. Conrad, C. Braunberger and U. Beifuss, RSC Adv., 2019, 9, 19549–19559 RSC
. - E. Değerli, S. Yangın and D. Cansaran-Duman, 3 Biotech, 2019, 9, 297 CrossRef PubMed
. - AWPA, American Wood Preservers Association, E 24-12, 2013.
- ASTM, American society for Testing and Materials, D4445-10, 2015.
- D. Filgueira, D. Moldes, C. Fuentealba and D. E. García, Ind. Crops Prod., 2017, 103, 185–194 CrossRef CAS
. - N. Aktaş and A. Tanyolaç, Bioresour. Technol., 2003, 87, 209–214 CrossRef
. - C. Yu, F. Wang, S. Fu, H. Liu and Q. Meng, J. Polym. Environ., 2017, 25, 1072–1079 CrossRef CAS
. - N. Mita, S. i. Tawaki, H. Uyama and S. Kobayashi, Macromol. Biosci., 2003, 3, 253–257 CrossRef CAS
. - S. Slagman, H. Zuilhof and M. C. Franssen, ChemBioChem, 2018, 19, 288–311 CrossRef CAS PubMed
. - J. Su, J. Fu, Q. Wang, C. Silva and A. Cavaco-Paulo, Crit. Rev. Biotechnol., 2018, 38 , 294–307 CrossRef CAS PubMed
. - R. Kaur, S. K. Uppal and P. Sharma, Sugar Tech., 2017, 675–680 CrossRef CAS
. - Q. Ma, D. Lin, Y. Yang and L. Wang, Food Hydrocolloids, 2016, 63, 677–684 CrossRef
. - J. Antoniou, F. Liu, H. Majeed, H. J. Qazi and F. Zhong, Carbohydr. Polym., 2014, 111, 359–365 CrossRef CAS PubMed
. - K. Wu, W. Ying, Z. Shi, H. Yang, Z. Zheng, J. Zhang and J. Yang, ACS Sustainable Chem. Eng., 2018, 6, 3853–3861 CrossRef CAS
.
|
This journal is © The Royal Society of Chemistry 2020 |
Click here to see how this site uses Cookies. View our privacy policy here.