DOI:
10.1039/D0RA00075B
(Paper)
RSC Adv., 2020,
10, 12053-12059
Cu(II) vitamin C tunes photocatalytic activity of TiO2 nanoparticles for visible light-driven aerobic oxidation of benzylic alcohols†
Received
3rd January 2020
, Accepted 15th March 2020
First published on 25th March 2020
Abstract
The incorporation of Cu(OAc)2 into ascorbic acid coated TiO2 nanoparticles easily provided a new heterogeneous visible-light active titania-based photocatalyst (TiO2-AA-Cu(II)) which was characterized by different techniques such as FT-IR, XPS, ICP-AES, TGA and TEM. A red-shift of the band-edge and a reduction of the band-gap (2.8 eV vs. 3.08 for TiO2) were demonstrated by UV-DRS and Tauc plots. The combination of the as-prepared TiO2-AA-Cu(II) nanoparticles with TEMPO and molecular oxygen (air) afforded an active catalytic system for the selective oxidation of diverse set of benzylic alcohols under solvent-free conditions. A photoassisted pathway was confirmed for oxidation reactions evidenced by good correlation between apparent quantum yield (AQY) and diffuse reflectance spectra (DRS) of the as-prepared nanohybrid. The spectral data and recycling experiments demonstrated the structural stability of the title copper photocatalyst during oxidation reactions.
1 Introduction
Transition metal ions are playing an important role in biological processes in the human body.1,2 They are found either at the active sites or as structural components of a good number of enzymes.3,4 Thus, study of the coordination chemistry of biologically important metal ions with bio-relevant ligands has been always an active research subject in bioinorganic chemistry. In this line of research, metal containing vitamins are increasingly becoming important particularly in drug design and nutrition, with the hope of improving and enriching the quality of existing vitamins, thereby serving as a better substitute as chemotherapeutic agents.5–7 In the search for novel metal complexes, that combine high activity with low toxicity, the study of metal complexes has continued to attract attention of some coordination chemists.8–10 This has also provided useful outlets for basic research in coordination chemistry. Among metal containing vitamins, the Cu(II) vitamin C complex attracted the most attention, because both Cu2+ and vitamin C are essential for the biosynthesis of the pigment melanin and are preferentially absorbed by the malignant melanoma tissues. There are also invaluable reports regarding the combination of Cu2+ and ascorbate (vitamin C) halt tumor growth in mice.11,12
As a part of our ongoing project, developing new visible light driven photocatalytic systems by surface modification of TiO2 nanoparticles by vitamins–metal complexes,13–15 in the present study, a novel heterogeneous nanostructured bio-photocatalyst is prepared by incorporation of simple and easily available Cu(OAc)2 compound into ascorbic acid-coated TiO2 nanoparticles under ultrasonic agitation. Copper–vitamin C complex supported on TiO2 nanoparticles is explored as an active photocatalyst for the selective oxidation of benzylic alcohols via ideal green process, i.e. using molecular oxygen in air as oxidant and be free of any solvent under visible light irradiation (Scheme 1). Spectral data, leaching experiment and recycling test proved the stability of the as-prepared nanohybrid making it competent catalyst for practical goals.
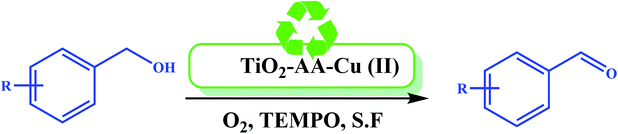 |
| Scheme 1 Aerobic oxidation of benzyl alcohols in the presence of TiO2-AA-Cu(II) nanohybrid. | |
2. Experimental
Note: general remarks and step by step preparation of TiO2-AA-Cu(II) nanohybrid catalyst are given in ESI.†
2.1. General procedure for aerobic oxidation of benzylic alcohols catalyzed by TiO2-AA-Cu(II) nanohybrid
To a mixture of benzyl alcohols (0.125 mmol) and TiO2-AA-Cu(II) nanohybrid (3 mg) was added TEMPO (0.0192 mmol) and the reaction mixture was stirred at 70 °C under air, visible light using a full spectrum CFL bulb (40 W) and solvent free conditions for the required time. The reaction progress and the yields of the products were monitored by GC. The isolated products were obtained by plate chromatography eluted with n-hexane/ethylacetate (10
:
3).
3. Result and discussion
3.1. Synthesis and characterization of TiO2-AA-Cu(II) nanohybrid
First, TiO2 and TiO2-AA nanoparticles were synthesized and purified according to our previous report16 (for experimental details, see the ESI†). For fabrication of TiO2-AA-Cu(II) nanohybrid, 0.2 g of TiO2-AA is dispersed in 5 mL deionized (DI) water through sonication for 5 min. Then, 1.0 mmol Cu(OAc)2 dissolved in ethanol is dropped into the suspension under ultrasonic agitation at room temperature for 20 min. The as-obtained mixture is refluxed for 12 h (Scheme S1†). Afterwards, the obtained product is centrifuged, washed with ethanol and dried at 100 °C for 12 h under vacuum. The Cu content of as-prepared catalyst was 1.23% based on ICP-AES analysis.
TEM images of nanohybrid exhibited a spherical morphology with an average size of 10–15 nm (Fig. S1†).
Comparative FT-IR spectra of TiO2, TiO2-AA and TiO2-AA-Cu(II) nanohybrid depicted in Fig. 1, confirms the complexation of Cu(II) with AA coated TiO2. As depicted in Fig. 1, all of the samples show a broad peak at around 3000 cm−1 corresponding to the hydroxyl groups. Broad peak at 1625 cm−1 corresponds to the surface adsorbed water. The bands in the region of 500–750 cm−1 are attributed to the stretching vibrations of Ti–O groups.17 The coating of AA on TiO2 (Fig. 1b) is substantiated by C–O stretching vibration of Ti–O–C appeared in the range of 927 and 984 cm−1.18 The strong peaks at about 1411 and 1560 cm−1 are attributed to the acetate group. The appearance of a band at 563 cm−1 in Fig. 1c is rationalized to the stretching vibration of Cu–O bond.19
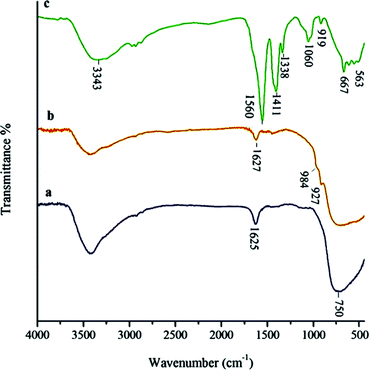 |
| Fig. 1 FT-IR spectra of (a) nanostructure TiO2 (b) TiO2-AA (c) TiO2-AA-Cu(II) nanohybrid. | |
The survey XPS spectrum of TiO2-AA-Cu(II) nanohybrid reveals the presence of Ti 2p, Cu 2p, O 1s and C elements (Fig. 2). Two broad peaks at 458 and 464 eV are attributed to the Ti 2p3/2 and Ti 2p1/2, respectively.20 The peak at 531 eV is assigned to the O 1s.21 Also, the signals at 935.32 and 955.28 eV correspond to 2p3/2 and 2p1/2 of Cu(II), respectively.22–24
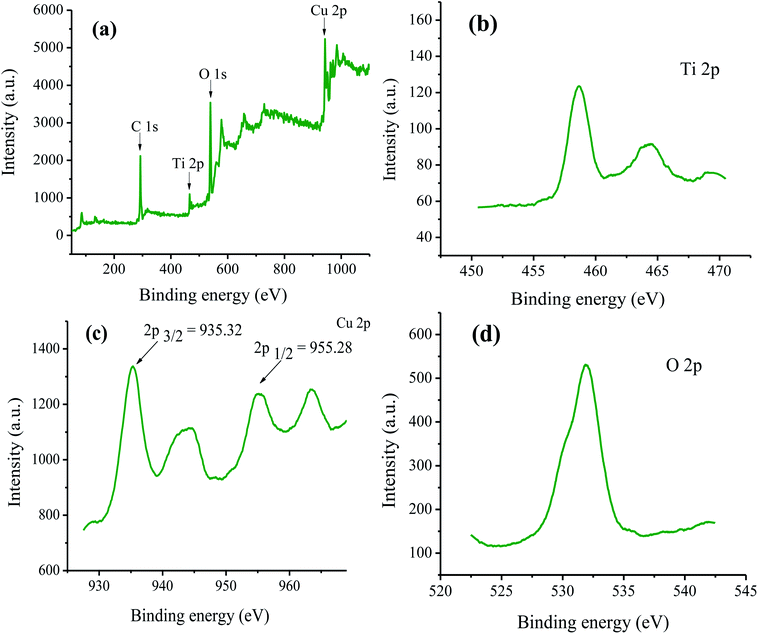 |
| Fig. 2 XPS spectra of TiO2-AA-Cu(II) nanohybrid (a) wide scan, (b) Ti 2p, (c) Cu 2p, and (d) O 1s. | |
TGA curve of catalyst (Fig. S2†) demonstrates its thermostability up to 200 °C and the organic parts decomposed completely at 678 °C.
Tauc plot, (αhν)2 vs. (hν), was used to determine the optical bandgap. As shown in Fig. 3, the band gap of TiO2 (3.08 eV) reduced gradually during modification with AA (TiO2-AA, 3.0 eV) and complexation with Cu(II) (Cu-AA-Cu(II), 2.8 eV) making it a visible-light sensitive photocatalyst.
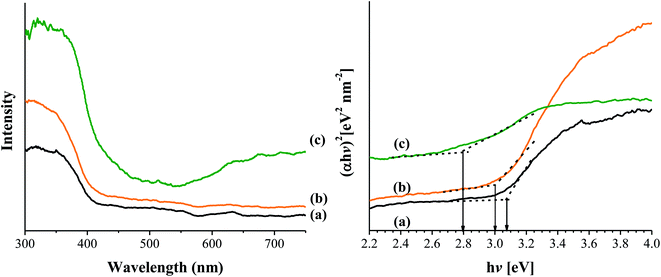 |
| Fig. 3 UV-Vis spectra and DRS of (a) bare TiO2 NPs, (b) TiO2-AA and (c) TiO2-AA-Cu(II) nanohybrid. | |
3.2. Photo-induced aerobic oxidation of benzylic alcohols
Oxidation of 4-chlorobenzyl alcohol (0.125 mmol) in the presence of TiO2-AA-Cu(II)/TEMPO photocatalytic system was chosen as a model reaction. A series of experiments was conducted to optimize the effect of solvent, amount of catalyst and TEMPO, temperature, and nature of oxidants (Fig. S3 and S4†).
According to optimization experiments the reaction proceeds more effective in the absence of any solvent (Fig S3a†). A temperature variation study showed that the conversion steadily increases from 50 to 90% as the temperature increases from 25 to 70 °C with maintaining the selectivity (Fig S3b†). A survey of the results shown in Fig. S3c† revealed that a catalyst loading of 3 mg (0.4 mol% based on Cu) leads to the desired yield of product. Also, we found that TEMPO is essential to trigger the reaction and access to the highest catalytic performance (Fig S3d†). When air was replaced by pure O2 as well as commercially available oxidants, less activity and selectivity were observed (Fig. S3e†). The parent materials such as TiO2, TiO2-AA, AA, Cu(OAc)2 and also ZnO were inferior under optimized conditions (Fig. S4†). Thus, title catalytic aerobic procedure exhibits the highest efficiency under visible light irradiation, using a benzyl alcohol
:
TEMPO molar ratio of 6.5 (0.125
:
0.0192) and 3 mg (0.4 mol% based on Cu) catalyst under solvent free conditions at 70 °C.
Next, we subjected various benzylic alcohols to the reaction conditions to assess the scope of the method. Our data summarized in Table 1 demonstrated the high efficiency of the title photocatalytic system for the visible light-induced aerobic oxidation of structurally and electronically different benzyl alcohols. However, the oxidation performance affected by electronic properties of substrates. Electron-withdrawing groups on the phenyl rings of alcohols retarded the reaction (Table 1, entries 8 and 9). Both primary and secondary benzylic alcohols delivered the corresponding carbonyl compounds in good to excellent yields. It should be mentioned that no trace of ester or benzoic acid was observed resulting from over oxidation of secondary and primary alcohols, respectively. To demonstrate the chemoselectivity of the method, 4-methylsulfanyl benzyl alcohol carrying oxidative sensitive sulfur atom was subjected to the oxidation procedure. The related carbonyl compound was formed as major product (85%) accompanied with 15% of the pertinent sulfoxide (4-methanesulfinyl-phenyl)-methanol resulting from sulfide group oxidation (Table 1, entry 14). It should be noted that the photocatalytic system was unable to oxidize the aliphatic alcohols and heterocyclic alcohols under different conditions.
Table 1 Oxidation of benzylic alcohols in the presence of TiO2-AA-Cu(II)a
Entry |
Alcohol |
Productb |
Isolated yieldc (%) |
Reaction condition: 0.125 mmol alcohol, TEMPO (0.0192 mmol), cat. (0.003 g) and the reactions were run under solvent free conditions at 70 °C for 2 h under air and visible light (CFL, 40 W). The products were identified by comparison with authentic sample retention times of GC analysis and NMR spectra. The selectivity of products were >99% based on GC analysis. 15% of (4-methanesulfinyl-phenyl)-methanol was obtained. |
1 |
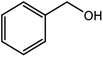 |
 |
96 |
2 |
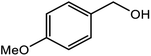 |
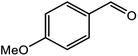 |
96 |
3 |
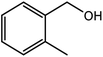 |
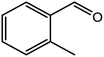 |
94 |
4 |
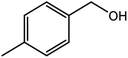 |
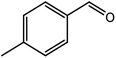 |
96 |
5 |
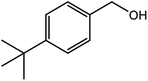 |
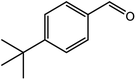 |
89 |
6 |
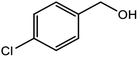 |
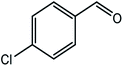 |
95 |
7 |
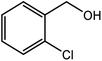 |
 |
94 |
8 |
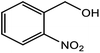 |
 |
60 (3 h) |
9 |
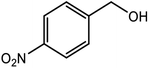 |
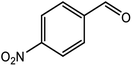 |
68 (3 h) |
10 |
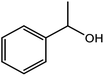 |
 |
98 |
11 |
 |
 |
68 (5 h) |
12 |
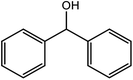 |
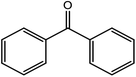 |
78 (5 h) |
13 |
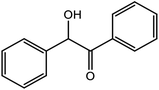 |
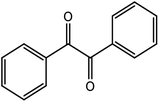 |
85 |
14d |
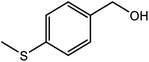 |
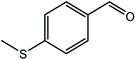 |
85 (6 h) |
3.3. Photocatalytic activity
The photocatalytic activity of TiO2-AA-Cu(II) nanohybrid was investigated by the oxidation of 4-chlorobenzyl alcohol under air as oxidant. The visible-light source for the photocatalytic reaction was a 40 W CFL bulb (400 to 800 nm) equipped with different cut-off filters. A reaction subjected on the full spectrum irradiation (without any cut-off filters) gives 98% 4-chlorobenzaldehyde (GC yield). Nevertheless, the product yield decreased to 70, 62, and 49%, by employing 450–800, 550–800, and 600–800 nm filters, respectively. The contributions of the photoassisted pathway by deducting the thermal contribution (product formed at dark; 30%) are 41, 11, 19 and 27% for 400–450, 450–550, 550–600 and 600–800 nm, respectively (Fig. 4). These results are consistent with the UV-visible absorption spectrum and band gap value of 2.8 eV of TiO2-AA-Cu(II) nanohybrid and demonstrate the highest visible-light photocatalytic performance of catalyst at 400–450 nm (Fig. 4).
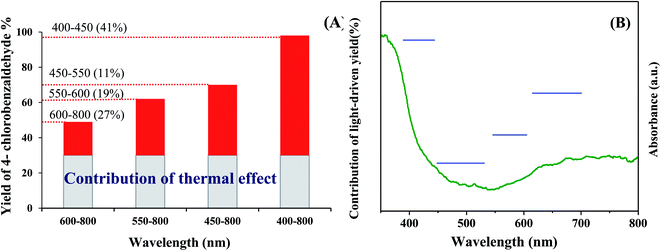 |
| Fig. 4 Dependence of 4-chloro benzaldehyde yield on the irradiation wavelength (A), and the action spectrum of the photocatalytic reaction, in which the light driven conversion is plotted against the irradiation wavelength (B). | |
More evidence for the photocatalytic activity of as-prepared catalyst was obtained by apparent quantum yield for 4-chlorobenzaldehyde formation (AQY) calculated with the following equation: [AQY (%) = (%) Yvis − Ydark ×2/(photon number entered into the reaction vessel) × 100].25 Fig. 5 clearly shows a good correlation between AQY and diffuse reflectance spectrum (DRS) of the TiO2-AA-Cu(II) nanohybrid confirming once again a photoassisted pathway for title oxidation reactions.
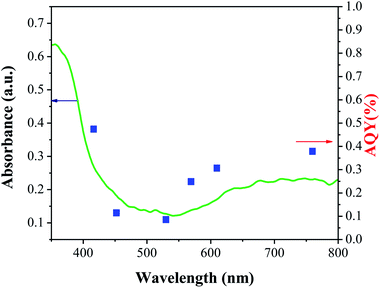 |
| Fig. 5 Photocatalytic action spectrum for synthesis of 4-chlorobenzaldehyde using TiO2-AA-Cu(II) photocatalyst. | |
3.4. Catalyst recovery and reuse
The stability and reusability of TiO2-AA-Cu(II) nanohybrid were assessed during 4 runs (Fig. S5†). At the end of each run, 2 mL ethanol as a green solvent was added, followed by centrifugation to isolate the residual catalyst. The catalyst was dried under vacuum and reused for next runs. The negligible loss of activity was observed during 4 runs revealed the structural stability of the catalyst. More evidences for this important issue were obtained by monitoring the FT-IR spectra of catalyst during 4 cycles (Fig. S6†) as well as lack of copper in filtrate based on leaching hot filtration test combined with ICP-AES analysis.
Table 2 lists some aerobic methods based on Cu catalysts for oxidation of benzyl alcohol in comparison with the present catalytic system. The obvious advantages of our photochemical method including a visible light-induced catalyst with low dosage and high recyclability, desired reaction time, high product yield and excellent selectivity and particularly environmentally benign conditions used in this work (solvent-free condition under air and visible light irradiation) qualify all requirements of an efficient catalytic system for applied goals.
Table 2 Comparison of oxidative activity of TiO2-AA-Cu(II) nanohybrid with other Cu based catalysts in the oxidation of benzyl alcohol
Entry |
Catalyst |
Catalyst (mol%) |
Conditions |
Time (h) |
Yield (%) |
Ref. |
(3-Aminopropyl) triethoxysilane. (S)-1-(3,5-Dichloro-2-hydroxybenzyl) pyrrolidine-2-carboxylic acid. 4-Dimethylaminopyridine. Polystyrene. Triazine-based polyethyleneamine dendrimer. N-Allyl-o-hydroxyacetophenoniminato. Picolinoyl chloride. N-Methylimidazole. |
1 |
TiO2-AA-Cu(II) |
0.003 g (0.4 mol%) |
70 °C/s.f/TEMPO/vis. light/air |
2 |
96 |
This work |
2 |
SBA@APa-LProb-CuOAc |
1 |
50 °C/toluene/TEMPO/Cs2CO3/O2 |
9 |
90 |
26 |
3 |
Salophen-copper(II) |
2 |
rt/MeCN/TBHP/NaOH/air |
24 |
97 |
27 |
4 |
CuCl |
5 |
rt/DMAPc/TEMPO/H2O/air |
10 |
94 |
28 |
5 |
PSd-PEG-TD2e Cu(OAc)2 |
20 |
80 °C/heptane/TEMPO/air |
24 |
80 |
29 |
6 |
[Cu(Lallyl)2]f |
0.025 mmol |
25 °C/toluene/TEMPO/air |
18 |
97 |
30 |
7 |
CuBr/UiO-66-NH-PCg |
5 |
rt/CH3CN/TEMPO/NMIh/air |
9 |
86 |
31 |
8 |
[MCM-41-bpy-CuI] |
5 |
50 °C/EtOH/TEMPO/NH3/air |
26 |
92 |
32 |
9 |
CuO-rectorite |
31 mg |
50 °C/H2O/K2CO3/TEMPO/O2 |
24 |
77.8 |
33 |
10 |
(NH4)4[CuMo6O18(OH)6] |
1 |
60 °C/MeCN : H2O/NaCl/O2 |
20 |
87 |
34 |
11 |
Cu–Mn oxide/C |
10 wt% |
80 °C/CH2Cl2/TEMPO/O2 |
2.5 |
96.9 |
35 |
12 |
CuFe2O4 |
10 |
100 °C/H2O/TEMPO/O2 |
24 |
79 |
36 |
13 |
CuBr |
5 |
rt/CH3OH/L-proline/Na2CO3/TEMPO/air |
6 |
92 |
37 |
4 Conclusion
In conclusion, an efficient and clean aerobic method for visible light-driven photooxidation of benzylic alcohols over TiO2-AA-Cu(II) nanohybrid in combination with TEMPO under solvent-free condition was developed. The catalyst showed desired recyclability and no copper leaching was observed during reaction, which demonstrates its potential for industrial application. Good correlation between apparent quantum yield (AQY) and diffuse reflectance spectrum (DRS) of the as-prepared nanohybrid confirmed a photoassisted pathway for the title oxidation reactions. The use of air as oxidant and visible light as a safe light source under solvent-free condition in the presence of a reusable and selective bio-relevant oxidation catalyst offer an ideal green protocol, making it more appropriate for applied goals.
Conflicts of interest
The authors declare no conflict of interest.
Acknowledgements
Support for this work by Research Council of University of Birjand and ‘‘Iran national Science Foundation’’ (Grant No. 96013225) is highly appreciated.
References
- W. W. Zhao, J. J. Xu and H. Y. Chen, Analyst, 2016, 141, 4262–4271 RSC .
- S. Kapoor, D. Brazete, I. C. Pereira, G. Bhatia, M. Kaur, L. F. Santos and J. M. Ferreira, J. Non-Cryst. Solids, 2019, 506, 98–108 CrossRef CAS .
- C. A. Denard, H. Huang, M. J. Bartlett, L. Lu, Y. Tan, H. Zhao and J. F. Hartwig, Angew. Chem., Int. Ed., 2014, 53, 465–469 CrossRef CAS PubMed.
- J. M. Palomo and M. Filice, Biotechnol. Adv., 2015, 33, 605–613 CrossRef CAS PubMed.
- D. A. Kose, B. Zumreoglu-Karan, C. Unaleroglu, O. Sahin and O. Buyukgungor, J. Coord. Chem., 2006, 59, 2125–2133 CrossRef CAS .
- A. E. Fazary, Y.-H. Ju, A. Q. Rajhi, A. S. Alshihri, M. Y. Alfaifi, M. A. Alshehri, K. A. Saleh, S. E. I. Elbehairi, K. F. Fawy and H. S. M. Abd-Rabboh, Open Chem., 2016, 14, 287–298 CAS.
- P. Brandão and S. Guieu, in Mol. Nutr., Elsevier, 2020, pp. 33–49 Search PubMed.
- A. Pettenuzzo, R. Pigot and L. Ronconi, Eur. J. Inorg. Chem., 2017, 2017, 1625–1638 CrossRef CAS.
- B. Annaraj and M. A. Neelakantan, Eur. J. Med. Chem., 2015, 102, 1–8 CrossRef CAS PubMed.
- A. K. Renfrew, Metallomics, 2014, 6, 1324–1335 RSC.
- B. Zümreoglu-Karan, Coord. Chem. Rev., 2006, 250, 2295–2307 CrossRef.
- Y.-D. Park, Y.-J. Lyou, H.-S. Hahn, M.-J. Hahn and J.-M. Yang, J. Biomol. Struct. Dyn., 2006, 24, 131–138 CrossRef CAS PubMed.
- F. Feizpour, M. Jafarpour and A. Rezaeifard, Catal. Lett., 2018, 148, 30–40 CrossRef CAS.
- F. Feizpour, M. Jafarpour and A. Rezaeifard, Catal. Lett., 2019, 149, 1595–1610 CrossRef CAS.
- E. Rezapour, M. Jafarpour and A. Rezaeifard, Catal. Lett., 2018, 148, 3165–3177 CrossRef CAS.
- M. Jafarpour, F. Feizpour and A. Rezaeifard, RSC Adv., 2016, 6, 54649–54660 RSC.
- G. Li, B. Wang, W. Q. Xu, Y. Han and Q. Sun, Dyes Pigm., 2018, 155, 265–275 CrossRef CAS.
- Y. Ou, J.-D. Lin, H.-M. Zou and D.-W. Liao, J. Mol. Catal. A: Chem., 2005, 241, 59–64 CrossRef CAS.
- Z. Ansari, A. Saha, S. S. Singha and K. Sen, J. Photochem. Photobiol., A, 2018, 367, 200–211 CrossRef CAS.
- S. Mathew, P. Ganguly, S. Rhatigan, V. Kumaravel, C. Byrne, S. Hinder, J. Bartlett, M. Nolan and S. Pillai, Appl. Sci., 2018, 8, 2067 CrossRef CAS.
- Q. Hu, J. Huang, G. Li, Y. Jiang, H. Lan, W. Guo and Y. Cao, Appl. Surf. Sci., 2016, 382, 170–177 CrossRef CAS.
- T. Zheng, T. Wang, R. Ma, W. Liu, F. Cui and W. Sun, Sci. Total Environ., 2019, 650, 1412–1418 CrossRef CAS PubMed.
- J. Kim, T. Kang, H. Kim, H. J. Shin and S.-G. Oh, J. Ind. Eng. Chem., 2019, 77, 273–279 CrossRef CAS.
- S. E. Allen, R. R. Walvoord, R. Padilla-Salinas and M. C. Kozlowski, Chem. Rev., 2013, 113, 6234–6458 CrossRef CAS PubMed .
- Y. Sugano, Y. Shiraishi, D. Tsukamoto, S. Ichikawa, S. Tanaka and T. Hirai, Angew. Chem., Int. Ed., 2013, 52, 5295–5299 CrossRef CAS PubMed.
- I. Saberikia, E. Safaei, B. Karimi and Y.-I. Lee, ChemistrySelect, 2017, 2, 11164–11171 CrossRef CAS.
- T. Chen and C. Cai, Synth. Commun., 2015, 45, 1334–1341 CrossRef CAS.
- G. Zhang, C. Yang, E. Liu, L. Li, J. A. Golen and A. L. Rheingold, RSC Adv., 2014, 4, 61907–61911 RSC.
- S. Pan, S. Yan, T. Osako and Y. Uozumi, Synlett, 2018, 29, 1152–1156 CrossRef CAS.
- M. del Mar Conejo, P. Ávila, E. Álvarez and A. Galindo, Inorg. Chim. Acta, 2017, 455, 638–644 CrossRef.
- X. Du, Y. Luan, F. Yang, D. Ramella and X. Shu, New J. Chem., 2017, 41, 4400–4405 RSC.
- H. Zhao, Q. Chen, L. Wei, Y. Jiang and M. Cai, Tetrahedron, 2015, 71, 8725–8731 CrossRef CAS.
- W. Liu, J. Yang and J. Cai, Res. Chem. Intermed., 2019, 45, 549–561 CrossRef CAS.
- Y. Wei, Z. Wei, S. Ru, Q. Zhao, H. Yu and G. Zhang, Green Chem., 2019, 21, 4069–4075 RSC.
- G. Yang, W. Zhu, P. Zhang, H. Xue, W. Wang, J. Tian and M. Song, Adv. Synth. Catal., 2008, 350, 542–546 CrossRef CAS.
- X. Zhu, D. Yang, W. Wei, M. Jiang, L. Li, X. Zhu, J. You and H. Wang, RSC Adv., 2014, 4, 64930–64935 RSC.
- G. Zhang, X. Han, Y. Luan, Y. Wang, X. Wen and C. Ding, Chem. Commun., 2013, 49, 7908–7910 RSC.
Footnote |
† Electronic supplementary information (ESI) available. See DOI: 10.1039/d0ra00075b |
|
This journal is © The Royal Society of Chemistry 2020 |
Click here to see how this site uses Cookies. View our privacy policy here.