DOI:
10.1039/C9RA10964A
(Paper)
RSC Adv., 2020,
10, 8348-8351
Iodine-catalyzed convergent aerobic dehydro-aromatization toward benzazoles and benzazines†
Received
27th December 2019
, Accepted 15th February 2020
First published on 26th February 2020
Abstract
An iodine-catalyzed aerobic dehydro-aromatization has been developed, providing straightforward and efficient access to various benzoazoles and benzoazines. The present transition-metal-free protocol enables the dehydro-aromatization of tetrahydrobenzazoles and tetrahydroquinolines with molecular oxygen as the green oxidant, along with some other N-heterocycles. Hence, a broad range of heteroaromatic compounds are generated in moderate to good yields under facile reaction conditions.
Introduction
Benzazoles and benzazines have unique pharmacological and biological activities and thus have many applications in natural products and pharmaceutical drugs.1–3 As a result, chemical researchers have been actively seeking new methodologies to synthesize these compounds. In recent years, dehydrogenative aromatization has emerged as a direct and efficient approach to access heteroaromatic compounds, especially N-heterocycles. This strategy produces a series of benzazines with high value4 and continues to be an attractive and significant research object in organic synthesis.
The direct dehydro-aromatization methods were generally achieved by using stoichiometric oxidants, such as SeO2, DDQ, o-iodoxybenzoic acid (IBX) and sulfur (Scheme 1a).5,6 Recently, transition metal catalysts combined with oxygen as the sole sacrificial reagent were used to enable oxidative dehydrogenative aromatization, such as Au, Pt, Pd, Ru, Fe, and Co.7–9 On the other hand, in view of the atom-economy requirement and potential application for hydrogen storage, Ir, Ru, Fe and Co catalyst were used for the acceptorless dehydrogenation reactions of N-heterocycles.10–12 However, due to the involvement of transition metals and other specialized catalysts or amounts of oxidants, these dehydrogenation protocols are often expensive or not environmentally friendly. In recent years, Lewis acids,13 photoredox catalysis,14 electrocatalysis15 and graphene oxide (GO)16 were used for the same goal (Scheme 1b). In addition, potassium tert-butoxide17 and elemental sulfur18 also could promote the dehydrogenation process. However, most of these methods are mainly suitable for dehydro-aromatization of N-heterocycles. Hence, developing a convergent catalytic system which can be employed for dehydro-aromatization of both carbocycles and N-heterocycles to afford a diversity of heteroaromatic compounds is highly desirable.
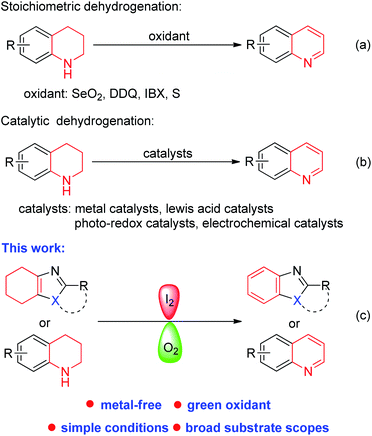 |
| Scheme 1 Kinds of dehydrogenative aromatization reactions. | |
Our group has been focusing on synthesizing aromatic heterocyclic compounds, using cyclohexanones as the aryl source via a dehydrogenative aromatization sequence.19,20 In our recent research, cyclohexanones coupled with amines to form tetrahydrobenzimidazoles, which could not be dehydrogenated to produce heteroaromatic compounds under these conditions.21 As a follow up study, herein, we describe an iodine catalyzed dehydro-aromatization reaction using molecular oxygen as the green oxidant toward various benzazoles and benzazines (Scheme 1c).
Results and discussion
We commenced our studies by choosing 2-phenyl-4,5,6,7-tetrahydrobenzo[d]thiazole (1a) as the model substrate. The desired product 4a was afforded in 36% in the presence of KI and oxygen (Table 1, entry 1). Then, a series of iodide-containing reagents such as NaI, NIS, elemental iodine, ICl and NaIO4 were screened (entries 2–6).22 The best reaction yield was achieved when elemental iodine was added as the catalyst (entry 4). A control experiment showed that trace of desired product was obtained in the absence of iodine reagent (entry 7). Subsequently, several solvents were tested. All of them did not give a higher yield (entries 8–13). The yield was up to 79% when o-dichlorobenzene (o-DCB) and toluene were used as the mixed solvent (entry 14). When the reaction atmosphere was changed to air, the reaction yield dramatically decreased (entry 15). A lower yield was obtained when the reaction was performed at 140 °C (entry 16).
Table 1 Optimization reaction conditionsa

|
Entry |
Catalyst |
Solvent |
Yieldb (%) |
Reaction conditions: 1a (0.2 mmol), catalyst (20 mol%), solvent (0.8 mL), 160 °C, under O2 (sealed tube), 30 h. GC yield. o-DCB/toluene (0.8 mL/0.2 mL). Under air. At 140 °C. Isolated yield. o-DCB:o-dichlorobenzene, ND: not detected. |
1 |
KI |
o-DCB |
36% |
2 |
NaI |
o-DCB |
39% |
3 |
NIS |
o-DCB |
52% |
4 |
I2 |
o-DCB |
71% |
5 |
NaIO4 |
o-DCB |
68% |
6 |
ICl |
o-DCB |
67% |
7 |
— |
o-DCB |
Trace |
8 |
I2 |
Chlorobenzene |
51% |
9 |
I2 |
Toluene |
55% |
10 |
I2 |
1,4-Dioxane |
23% |
11 |
I2 |
DMSO |
Trace |
12 |
I2 |
NMP |
ND |
13 |
I2 |
DMA |
ND |
14c |
I2 |
o-DCB/toluene |
79% (75%)f |
15c,d |
I2 |
o-DCB/toluene |
48% |
16c,e |
I2 |
o-DCB/toluene |
53% |
With the optimized conditions in hand, we explored the scope of this dehydrogenative aromatization reaction. The model reaction afforded the target product 4a in 75% isolated yield, and 68% isolated yield could be achieved in a gram-scale reaction. The corresponding products were obtained in moderate to good yields, when 2-phenyl-4,5,6,7-tetrahydrobenzo[d]thiazole substrates bearing with various substituents, such as alkyl, aryl, methoxy and halogen (4b–4i). Bulky 1-(naphthalen-1-yl)-4,5,6,7-tetrahydrobenzo[d]thiazole and 2-(naphthalen-1-yl)-4,5,6,7-tetrahydrobenzo[d]thiazole could also react well to afford the target products in good yields (4j, 4k). The steric effect of groups was not obvious on the reaction and the corresponding products were obtained in 62–74% yields when the carbocycle with an substituent (4l–4n). The desired product 4o could be obtained in 56% yield when 1-benzyl-2-phenyl-4,5,6,7-tetrahydro-1H-benzo[d]imida-zole was used as the substrate. Regrettably, 2-phenyl-4,5,6,7-tetrahydrobenzo[d]oxazole could not be dehydrogenated under the standard condition (4p). Subsequently, we investigated the dehydrogenation of tetracycle-fused system. Benzo[d]benzo[4,5]imidazo[2,1-b]thiazole 5a could be smoothly generated in 71% yield under the standard conditions when 7,8,9,10-tetrahydrobenzo[d]benzo[4,5]imida-zo[2,1-b]thiazole was used as the substrate. The substrates bearing a substituent such as methyl and halogen on the benzene ring afforded the corresponding product in 63–70% yields (5b–5d). The dehydrogenative products were gave in 64–71% yields when carbocycle was decorated by an alkyl or aryl substituent (5e, 5f) (Tables 2 and 3).
Table 2 Dehydro-aromatization of tetrahydrobenzazolesa
Conditions: 1 or 2 (0.2 mmol), I2 (20 mol%), o-DCB/toluene (0.8 mL/0.2 mL), 160 °C, 30 h, under O2 (sealed tube), isolated yield. 6 mmol scale. |
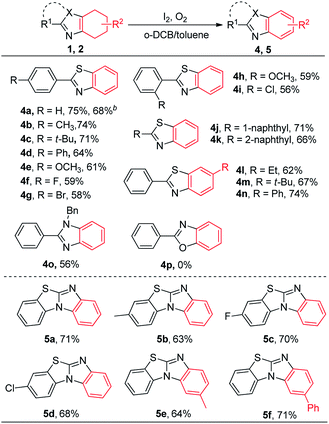 |
Table 3 Dehydro-aromatization of N-heterocyclesa
Conditions: 3 (0.2 mmol), I2 (20 mol%), o-DCB/toluene (0.8 mL/0.2 mL), 160 °C, 30 h, under O2, isolated yield. |
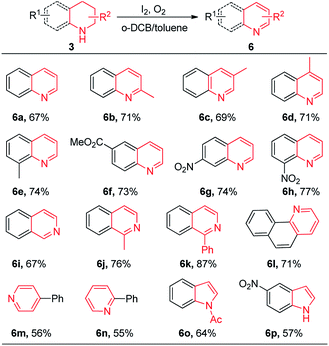 |
To further extend the scope of the substrates, we tested a variety of N-heterocycles under the standard reaction condition. Fortunately, most of them could give the desired products in moderate to good yields. Quinoline 6a was generated in 67% yield when 1,2,3,4-tetrahydroquinoline was used as the substrate. Tetrahydroquinolines with a methyl located at 2-, 3-, 4-, or 8-position could afford the corresponding dehydrogenation products in good yields (6b–6e). Strong electron-withdrawing groups, such as ester and nitro groups, attached at tetrahydroquinoline substrates were well tolerated to give the target products (6f–6h). The dehydrogenation reactions of 1,2,3,4-tetrahydroisoquinoline smoothly proceeded to give the corresponding isoquinoline products in 67–87% yields (6i–6k). Benzo[h]quinoline 6l was produced in 71% yield. In addition, 4-phenylpiperidine and 2-phenylpiperidine could be oxidized to form pyridine products in moderate yields under standard conditions (6m, 6n). Furthermore, indole products could be obtained in good yields when 1-(indolin-1-yl)ethanone and 5-nitroindoline were used (6o, 6p).
On the basis of relevant literatures,23 a possible reaction mechanism is illustrated in Scheme 2. Initially, nitrogen-centered radical A is formed via single-electron oxidation process from 1a in the presence of elemental iodide, and elemental iodide converts into iodine ion. Tautomerization of A produces intermediate B. Subsequent single-electron oxidation and deprotonation of B generates intermediate C, which can be further transformed into intermediate D via a deprotonation. Meanwhile, the second single-electron oxidative dehydrogenation of D affords the intermediate G, followed by a deprotonation to provide the desired product 4a. The iodide anion is oxidized to form iodine in the presence of oxygen.
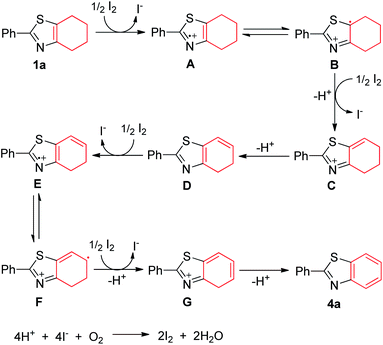 |
| Scheme 2 Possible reaction mechanism. | |
Conclusions
In conclusion, we have developed a direct dehydro-aromatization of tetrahydrobenzazoles and N-heterocycles, providing a straightforward and efficient approach to a variety of benzazoles and benzazines. The present protocol is achieved by use of elemental iodine as the catalyst and molecular oxygen as the green oxidant, which could produce a broad range of products in moderate to good yields under facile transition-metal-free conditions.
Conflicts of interest
There are no conflicts to declare.
Acknowledgements
We are grateful for financial support from the National Natural Science Foundation of China (21871226, 21572194, 21372187), the Collaborative Innovation Center of New Chemical Technologies for Environmental Benignity and Efficient Resource Utilization.
Notes and references
-
(a) M. O. Chaney, P. V. Demarco, N. D. Jones and J. L. J. Occolowitz, J. Am. Chem. Soc., 1974, 96, 1932 CrossRef CAS PubMed;
(b) M. K. Sahoo, G. Jaiswal, J. Rana and E. Balaraman, Chem.–Eur. J., 2017, 23, 14167 CrossRef CAS PubMed;
(c) O. Afzal, S. Kumar, M. R. Haider, M. R. Ali, R. Kumar, M. Jaggi and S. Bawa, Eur. J. Med. Chem., 2015, 97, 871 CrossRef CAS PubMed.
-
(a) N. Kumar, M. Alamgir, D. St and C. Black, Heterocycl. Chem., 2007, 9, 87 Search PubMed;
(b) M. E. Doyle and J. M. Egan, Pharmacol. Rev., 2003, 55, 105 CrossRef CAS PubMed;
(c) S. Meidute-Abaraviciene, H. Mosen, I. Lundquist and A. Salehi, Acta Physiol., 2009, 195, 375 CrossRef CAS PubMed.
-
(a) D. K. Kahlon, T. A. Lansdell, J. S. Fisk and J. Tepe, Bioorg. Med. Chem., 2009, 17, 3093 CrossRef CAS PubMed;
(b) F. Touzeau, A. Arrault, G. Guillaumet, E. Scalbert, B. Pfeiffer, M.-C. Rettori, P. Renard and J.-Y. Merour, J. Med. Chem., 2003, 46, 1962 CrossRef CAS PubMed;
(c) A. Masajtis-Zagajewska, J. Majer and M. Nowicki, Hypertens. Res., 2010, 33, 348 CrossRef CAS PubMed.
- E. Vitaku, D. T. Smith and J. T. Njardarson, J. Med. Chem., 2014, 57, 10257 CrossRef CAS PubMed.
-
(a) J. M. Humphrey, Y. Liao, A. Ali, T. Rein, Y. L. Wong, H. J. Chen, A. K. Courtney and S. F. Martin, J. Am. Chem. Soc., 2002, 124, 8584 CrossRef CAS PubMed;
(b) K. C. Nicolaou, C. J. N. Manthison and T. Montagnon, J. Am. Chem. Soc., 2004, 126, 5192 CrossRef CAS PubMed.
-
(a) M. Zhao, L. Bi, W. Wang, C. Wang, M. Baudy-Floćh, J. Ju and S. Peng, Bioorg. Med. Chem., 2006, 14, 6998 CrossRef CAS PubMed;
(b) M. Cain, O. Campos, F. Guzman and J. M. Cook, J. Am. Chem. Soc., 1983, 105, 907 CrossRef CAS;
(c) K. C. Nicolaou, C. J. N. Mathison and T. Montagnon, Angew. Chem., Int. Ed., 2003, 42, 4077 CrossRef CAS PubMed.
-
(a) M. H. So, Y. Liu, C. M. Ho and C. M. Che, Chem.–Asian J., 2009, 4, 1551 CrossRef CAS PubMed;
(b) D. V. Jawale, E. Gravel, N. Shah, V. Dauvois, H. Li, I. N. Namboothiri and E. Doris, Chem.–Eur. J., 2015, 21, 7039 CrossRef CAS PubMed;
(c) D. Ge, L. Hu, J. Wang, X. Li, F. Qi, J. Lu, X. Cao and H. Gu, ChemCatChem, 2013, 5, 2183 CrossRef CAS.
-
(a) S. Furukawa, A. Suga and T. Komatsu, Chem. Commun., 2014, 50, 3277 RSC;
(b) A. E. Wendlandt and S. S. Stahl, J. Am. Chem. Soc., 2014, 136, 11910 CrossRef CAS PubMed.
-
(a) X. Cui, Y. Li, S. Bachmann, M. Scalone, A.-E. Surkus, K. Junge, C. Topf and M. Beller, J. Am. Chem. Soc., 2015, 137, 10652 CrossRef CAS PubMed;
(b) A. E. Wendlandt and S. S. Stahl, J. Am. Chem. Soc., 2014, 136, 506 CrossRef CAS PubMed;
(c) A. V. Iosub and S. S. Stahl, Org. Lett., 2015, 17, 4404 CrossRef CAS PubMed.
-
(a) R. Yamaguchi, C. Ikeda, Y. Takahashi and K. Fujita, J. Am. Chem. Soc., 2009, 131, 8410 CrossRef CAS PubMed;
(b) K. Fujita, Y. Tanaka, M. Kobayashi and R. Yamaguchi, J. Am. Chem. Soc., 2014, 136, 4829 CrossRef CAS PubMed;
(c) J. Wu, D. Talwar, S. Johnston, M. Yan and J. Xiao, Angew. Chem., Int. Ed., 2013, 52, 6983 CrossRef CAS PubMed;
(d) R. Xu, S. Chakraborty, H. Yuan and W. D. Jones, ACS Catal., 2015, 5, 6350 CrossRef CAS.
-
(a) S. Muthaiah and S. H. Hong, Adv. Synth. Catal., 2012, 354, 3045 CrossRef CAS;
(b) S. Chakraborty, W. W. Brennessel and W. D. Jones, J. Am. Chem. Soc., 2014, 136, 8564 CrossRef CAS PubMed;
(c) G. Jaiswal, V. G. Landge, D. Jagadeesan and E. Balaraman, Nat. Commun., 2017, 8, 2147 CrossRef PubMed.
-
(a) R. H. Crabtree, ACS Sustainable Chem. Eng., 2017, 5, 4491 CrossRef CAS;
(b) Y. H. Han, Z. Y. Wang, R. R. Xu, W. Zhang, W. X. Chen, L. R. Zheng, J. Zhang, J. Luo, K. L. Wu, Y. Q. Zhu, C. Chen, Q. Peng, Q. Liu, P. Hu, D. S. Wang and Y. Li, Angew. Chem., Int. Ed., 2018, 57, 11262 CrossRef CAS PubMed;
(c) C. Deraedt, R. Ye, W. T. Ralston and F. D. Toste, J. Am. Chem. Soc., 2017, 139, 18084 CrossRef CAS PubMed.
-
(a) A. F. G. Maier, S. Tussing, T. Schneider, U. Flörke, Z.-W. Qu, S. Grimme and J. Paradies, Angew. Chem., Int. Ed., 2016, 55, 12219 CrossRef CAS PubMed;
(b) M. Kojima and M. Kanai, Angew. Chem., Int. Ed., 2016, 55, 12224 CrossRef CAS PubMed.
-
(a) S. Kato, Y. Saga, M. Kojima, H. Fuse, S. Matsunaga, A. Fukatsu, M. Kondo, S. Masaoka and M. Kanai, J. Am. Chem. Soc., 2017, 139, 2204 CrossRef CAS PubMed;
(b) K.-H. He, F.-F. Tan, C.-Z. Zhou, G.-J. Zhou, X.-L. Yang and Y. Li, Angew. Chem., Int. Ed., 2017, 56, 3080 CrossRef CAS PubMed;
(c) M. F. Zheng, J. L. Shi, T. Yuan and X. C. Wang, Angew. Chem., Int. Ed., 2018, 57, 5487 CrossRef CAS PubMed;
(d) S. Chen, Q. Wan and A. K. Badu-Tawiah, Angew. Chem., Int. Ed., 2016, 55, 9345 CrossRef CAS PubMed;
(e) F. Su, S. C. Mathew, L. Mçhlmann, M. Antonietti, X. Wang and S. Blechert, Angew. Chem., Int. Ed., 2011, 50, 657 CrossRef CAS.
- Y. Wu, H. Yi and A. Lei, ACS Catal., 2018, 8, 1192 CrossRef CAS.
- J. Y. Zhang, S. Y. Chen, F. F. Chen, W. S. Xu, G.-J. Deng and H. Gong, Adv. Synth. Catal., 2017, 359, 2358 CrossRef CAS.
- T. T. Liu, K. K. Wu, L. D. Wang and Z. K. Yu, Adv. Synth. Catal., 2019, 361, 3958 CrossRef CAS.
- T. B. Nguyen and P. Retailleau, Adv. Synth. Catal., 2018, 360, 2389 CrossRef CAS.
-
(a) X. X. Cao, X. F. Cheng, Y. Bai, S. W. Liu and G.-J. Deng, Green Chem., 2014, 16, 4644 RSC;
(b) Y. F. Liao, Y. Peng, H. G. Qi, G.-J. Deng, H. Gong and C.-J. Li, Chem. Commun., 2015, 51, 1031 RSC;
(c) J. J. Chen, G. Z. Li, Y. J. Xie, Y. F. Liao, F. H. Xiao and G.-J. Deng, Org. Lett., 2015, 17, 5870 CrossRef CAS PubMed.
-
(a) J. Wu, Y. J. Xie, X. G. Chen and G.-J. Deng, Adv. Synth. Catal., 2016, 358, 3206 CrossRef CAS;
(b) Y. J. Xie, J. Wu, X. G. Che, Y. Chen, H. W. Huang and G.-J. Deng, Green Chem., 2016, 18, 667 RSC;
(c) S. P. Chen, L. R. Wang, J. Zhang, Z. R. Hao, H. W. Huang and G.-J. Deng, J. Org. Chem., 2017, 82, 11182 CrossRef CAS PubMed.
-
(a) Y. J. Xie, X. G. Chen, Z. Wang, H. W. Huang, B. Yi and G.-J. Deng, Green Chem., 2017, 19, 4294 RSC;
(b) X. G. Chen, Z. Wang, H. W. Huang and G.-J. Deng, Adv. Synth. Catal., 2018, 360, 4017 CrossRef CAS.
-
(a) Y. Su, X. J. Zhou, C. L. He, W. Zhang, X. Ling and X. Xiao, J. Org. Chem., 2016, 81, 4981 CrossRef CAS PubMed;
(b) S. Tang, K. Liu, Y. Long, X. T. Qi, Y. Lan and A. W. Lei, Chem. Commun., 2015, 51, 8769 RSC;
(c) H. Cao, J. W. Yuan, C. Liu, X. Q. Hu and A. W. Lei, RSC Adv., 2015, 5, 41493 RSC;
(d) W.-H. Bao, M. He, J.-T. Wang, X. Peng, M. Sung, Z. L. Tang, S. Jiang, Z. Cao and W.-M. He, J. Org. Chem., 2019, 84, 6065 CrossRef CAS PubMed.
-
(a) V. Humne, Y. Dangat, K. Vanka and P. Lokhande, Org. Biomol. Chem., 2014, 12, 4832 RSC;
(b) J. Zhao, H. Huang, W. Wu, H. Chen and H. Jiang, Org. Lett., 2013, 15, 2604 CrossRef CAS PubMed;
(c) S. P. Chen, Y. X. Li, P. H. Ni, B. C. Yang, H. W. Huang and G.-J. Deng, J. Org. Chem., 2017, 82, 2935 CrossRef CAS PubMed.
Footnote |
† Electronic supplementary information (ESI) available. See DOI: 10.1039/c9ra10964a |
|
This journal is © The Royal Society of Chemistry 2020 |