DOI:
10.1039/C9RA10846G
(Paper)
RSC Adv., 2020,
10, 11557-11564
Exploration of a novel triazine-based covalent organic framework for solid-phase extraction of antibiotics†
Received
24th December 2019
, Accepted 2nd March 2020
First published on 20th March 2020
Abstract
Covalent organic frameworks (COFs) are a new class of porous materials with a large surface area and potential superiority in sample pretreatment. Herein a novel triazine-based COF named SCAU-1 was fabricated via a hydrothermal method, and SNW-1 was also prepared as a contrastive COF material. After characterization, SCAU-1 was used as solid-phase extraction (SPE) adsorbent to concentrate five sulfanilamides (SAs) and four tetracyclines (TCs). Several parameters were investigated in the extraction process, including the amount of adsorbent, the flow rate, the washing and elution solvent, the loading capacity and breakthrough volume. After detected by UPLC-MS/MS, the results revealed that SCAU-1 showed high extraction efficiency towards the selected antibiotics. According to the comparison with SNW-1, SCAU-1 showed better performance for the adsorption of TCs. While for SAs, the recoveries of SCAU-1 is close to that of SNW-1. The LODs are from 0.031 to 0.55 μg L−1, and the recoveries are from 88.0% to 93.4% for SCAU-1 with the RSDs ranging from 2.5% to 4.8%. The results demonstrated that SCAU-1 is a kind of promising adsorbent for the enrichment of organic pollutants.
Introduction
Antibiotics are extensively used in humans and animals for precaution and treatment of microbial infections due to their high efficiency, convenience and low-price.1,2 After the intake of antibiotics, a significant fraction is excreted with urine and feces and then enters the aquatic and terrestrial environment through multiple pathways, which will act on environmental organisms and pose a negative effect on human health and the ecosystem. For example, environmental antibiotics have an induction effect of drug resistance genes, which may result in an increase in drug resistance of the corresponding microorganisms.3–6 According to the literature, the pollution source of antibiotics is also the main pollution source of antibiotic resistance genes, which can be found in various water sources.7,8 Antibiotics in environmental water always exist at a low-level in complex water matrices, thus concentration and enrichment are needed before detection.9,10
For the enrichment of antibiotics in environmental water, solid-phase extraction (SPE) has been widely used for purification and concentration.11 Commercially available SPE cartridge including Oasis HLB,12 Hysphere C18-EC,13 and Bond Elut-ENV14 have been used for pre-concentration of antibiotics. In addition, some porous material such as MWCNTs,15 polystyrene porous nanofibers,16 molecularly imprinted polymer,17 porous covalent organonitridic frameworks (PCONFs) and porous aromatic frameworks (PAFs) are also used for the pretreatment of antibiotics.18,19 However, antibiotics differ greatly in hydrophilic and structure, thus high recoveries are difficult to get for various kinds. Therefore, there is considerable interest in developing new selective sorbents for extracting and isolating components from complicated matrices.
Covalent organic frameworks (COFs), an exciting new type of crystalline porous organic materials, are ingeniously constructed with organic building units via strong covalent bonds.20–24 Compared with metal organic frameworks (MOFs), COFs have been proved to be more stable in water and acidic media, and are metal-free in their structure. They always have good thermal stability, large surface area, and low density, which have diverse potential applications such as in catalysis,25 gas storage,26,27 and adsorptions.28 Furthermore, COFs have organic open pore structures as well as stacked π-electron systems, which make COFs ideal for sample pretreatment. Several researches have been focused on the exploration of COFs as SPE adsorbents or SPME layer for enriching organic or inorganic pollutants.29 Li et al. has used HL-COP as SPE absorbent for online analysis of trace Sudan dyes in food samples.30 Cai et al. adopted TpPa-1 as MSPE adsorbents for enriching polycyclic aromatic hydrocarbons (PAHs) from environmental water.31 Among the porous COFs, SNW-1, reported by Thomas in 2009,32 has proven to be an excellent adsorbent material, which has been applied for heavy metal removal,33 nitroaromatic explosives sensing and carbon dioxide capture.34,35 SNW-1 has also been reported as SPME coatings for selectively adsorbing polycyclic aromatic hydrocarbons (PAHs) and volatile fatty acids.36 And SNW-1@PAN electrospun nanofiber has been used as adsorbent for PT-SPE of five sulfonamides in meat samples.37 To widen the functionality of SNW-1, monomer designs are studied widely, such as changing the location of aldehyde group,32,38 or extending the size of triamine.39 However, altering the benzene ring of dialdehyde to heterocyclic ring may not only maintain the motif of SNW-1 but also provide more hydrogen bond donors, and such strategy is still rarely reported in the synthesis of COFs to our known.
Herein a novel porous COF, SCAU-1, was fabricated as SPE adsorbent. SNW-1 was also tested in the parallel experiment as a comparision material. Melamine are used in both two synthesis process, while 1,4-piperazinedicarboxaldehyde and terephthalaldehyde are employed for the synthesis of SCAU-1 and SNW-1, respectively. To study the adsorptivity of SCAU-1, two types of antibiotics in environmental water, including five sulfanilamides and four tetracyclines, are selected as analytes. The extraction results were determined by UPLC-MS/MS, and four kinds of real water (drinking water, river water, lake water and wastewater) and milk were also tested to validate the feasibility of the method.
Experimental
Reagents and materials
The chemical reagents employed were commercially available. Methanol (MeOH), acetonitrile (ACN), formic acid (FA) and water as mobile phase of LC-MS pure grade were purchased from Merck (Shanghai, China). Analytical grade solvents were purchased from Guangzhou Chemical Regent Factory (Guangzhou, China). Other reagents were all purchased from Aladin (Shanghai, China). The sieve plates for SPE column were made in polypropylene with the pore size of 20 μm.
The standard of nine single antibiotic, sulfadiazine (SDZ), sulfadimidine (SMZ), tetracycline (TC), sulfametoxydiazine (SME), oxytetracycline (OTC), sulfadoxine (SDO), chlortetracycline (CTC), sulfachloropyridazine (SCP), doxycycline (DC) were purchased from Sigma-Aldrich (Saint Louis, USA). The stock solution of the mixed standard were prepared as 10 mg L−1 in methanol/water (1
:
1, v/v), and stored at 4 °C in darkness. Different calibration solutions were prepared daily, by dilution of the stock solution with methanol/water (1
:
4, v/v).
Instruments
X-ray powder diffraction (XRPD) patterns were recorded on a Rigaku-D/max 2500 V X-ray diffractometer equipped with a source of Cu-Kα radiation (λ = 1.54178 Å) (Rigaku, Tokyo, Japan). The scanning electron micrographs (SEM) were obtained from a model S-3700 scanning electron microscope (Hitachi, Kyoto, Japan). The infrared spectrum (IR) and thermogravimetric analysis (TGA) were measured by using an EQUINOX 55 spectrometer (Bruker, Germany) and STA449C (NETZSCH, Selb, Germany), respectively. The BET surface area and pore distribution were tested by N2 adsorption on GEMINI VII 2390 (Micromeritics, Georgia, USA) under 77 K. Water samples flowed the SPE column by a vacuum pump (Yiheng, Shanghai, China).
Sample preparation
Four kinds of water samples with different matrices were collected, including drinking water, river water, lake water and wastewater. The river water, lake water and waste water were taken from the Pearl River, the Hongze Lake in Guangzhou and the water outlet of a livestock farm, respectively. The milk sample was bought from the local supermarket. All water samples were filtered with 0.45 μm filter membrane, and stored in brown bottles under 4 °C. For milk sample, 1.5 mL milk was acidified with 0.2 mL of 10% HClO4 to precipitate proteins. It was vortexed for 3 min and centrifuged at 10
000 rpm for 5 min. The supernatant was collected and diluted two times before sampling.
Synthesis of SCAU-1 and SNW-1
The synthesis process of the two COFs were carried out according to the literature with little modification.40 For SCAU-1, 0.365 g melamine and 0.535 g 1,4-piperazine-dicarboxaldehyde were mixed in DMSO (16 mL) and ultrasound for 15 min, then poured into a Teflon-line stainless steel vessel (25 mL). The vessel was heated to 180 °C and hold for 10 hours. After cooling to room temperature gradually, 0.55 g pale yellow powder was filtered (yield: 61%). The obtained powder was rinsed with dichloromethane and ethanol, respectively, and dried at 150 °C for 2 h in the vacuum oven.
The synthesis of SNW-1 was similar to the procedure of SCAU-1, of which 0.5 g terephthalaldehyde was used instead of 1,4-piperazinedicarboxaldehyde, and 0.45 g powder was finally obtained (yield: 52%).
The fabrication of COFs-SPE column and the extraction process
3 mL blank SPE columns were bought from Bona-Angel (Tianjin, China). The upper and under sieve plates were used to avoid the release of powder. Initially, the SPE column was washed with 2 mL methanol and 2 mL water, respectively. Then 50 mL water sample was added into the column under evacuation. After washing, the column was pumped as dry as possible and 2 mL solvent was added to elute the analytes. The elution was blow to dry under N2 atmosphere and resolved with 0.5 mL initial flow phase before determination.
The determination of SAs and TCs
The chromatographic separation and detection of nine antibiotics were carried out on a Waters Acquity UPLC system coupled to a Waters Xevo G2 Qtof mass spectrometer (Waters, USA) equipped with an ACQUITY UPLC®BEH C18 column (2.1 mm × 50 mm, 1.7 μm). The mobile phase composition and the gradient elution conditions are shown in Table S1 (ESI†). The column temperature was set at 40 °C, and the flow rate was 0.3 mL min−1. The volume of the sample injected was 2 μL. The mass spectrometry measurement of the compounds was performed in positive ion and multiple reaction monitoring (Tof MRM) mode by electrospray ionization. The detailed parameters were as follows: capillary voltage, 3000 V; desolvation gas temperature and flow rate, 350 °C and 650 L h−1; the source temperature, 120 °C; and the gas flow of the cone, 600 L h−1. The optimal mass spectrum parameters, the qualitative and quantitative ion of nine antibiotics are listed in Table S2 (ESI†).
Results and discussion
Characterization of the prepared COFs
The substance of this work was to synthesize a porous COF with high adsorptive capacity and strong π–π interaction, which may be applied in sample pretreatment. The design scheme was shown on Fig. 1a. By altering the phenyl ring to piperazine ring, N atoms may afford more lone pair electrons and polar forces with analytes. The prepared powder was confirmed by IR, PXRD, SEM, TGA and nitrogen adsorption isotherm measurements to observe the general physical property. From Fig. 1b and S1,† it can be seen that SCAU-1 has similar structure with SNW-1. In Fig. 1b, the substantial weakening of 2960 and 1697 cm−1 indicated that most –CHO groups have participated in the reaction. The strong peak at 3394 cm−1 corresponds to the stretching vibration of –NH– group. The peaks of 1560 and 1482 cm−1 are from the triazine rings, and the peak at 1340 and 1017 cm−1 represents the –C–N–C– stretching vibration of imides, which means that melamine has reacted with dialdehyde. The PXRD reveals SCAU-1 is amorphous material, which is close to the reported pattern of SNW-1 (Fig. S2†).30 The TGA curve gives the thermal stability of SCAU-1 (Fig. S4†), of which it can be seen that after removing the solvent molecules, the framework can maintain the motif until 400 °C. The FE-SEM characterization (Fig. 1c) showed that the morphology of SCAU-1 was uniform particles below fifty nanometers. However, nearly no particles of sorbents were found to be lost in the SPE process, which may be ascribed that agglomeration may occur when packing and the pores of sieve plates were partially blocked by the particles. After five cycles of SPE, the weight loss of sorbents were less than 5%.
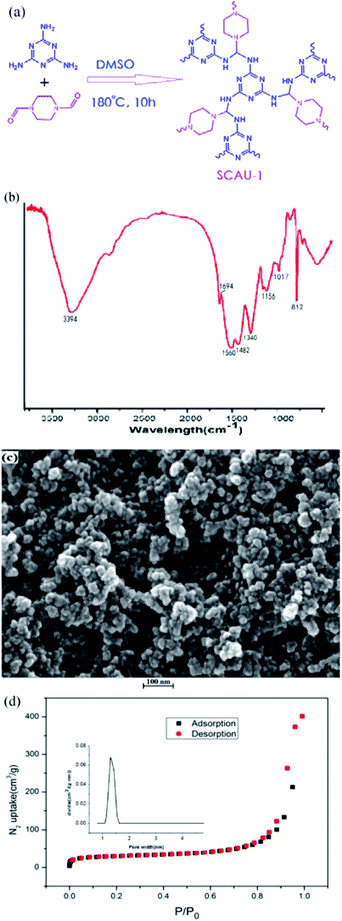 |
| Fig. 1 (a) The synthesis scheme of SCAU-1; (b) the FTIR spectrum of the prepared SCAU-1; (c) scanning electron micrograph of SCAU-1; (d) nitrogen adsorption/desorption at 77 K of the SCAU-1 powder. | |
The porosity of SCAU-1 and SNW-1 were characterized by N2 adsorption measurements at 77 K. The two COFs featured the type IV adsorption isotherm (Fig. 1d and S3†), which showed a steep uptake at low relative pressures and a nearly horizontal adsorption and desorption branches in the next section. The measured specific BET surface areas are 110.27 and 155.43 m2 g−1 for SCAU-1 and SNW-1, respectively. The BET surface area of SCAU-1 is smaller than the reported SNW-1,30 which may be ascribed that SCAU-1 possessed a lower degree of cross-linking and thus afforded more idle –NH2 groups. The remaining –NH2 groups may lead to the decrease of porosity and specific surface area. However, from the other prospective, abundant –NH2 groups can form H-bonds with the polar functional groups, which is beneficial for the adsorption of analytes. Compared with other porous materials with large BET surface, such as graphene, molecular sieve and carbon nanotube, COFs is more flexible to adjust the polarity and size of cavities by modifying the monomers, which may be superior on the selectivity for SPE process.
Optimization of SPE conditions
To investigate the SPE capacity of SCAU-1 towards antibiotics, a series of enrichment conditions were systematically optimized, including the amount of the adsorbent, the flow rate, the washing and elution solvent, the loading capacity and breakthrough volume. To shorten the loading time, the water volume was set to 50 mL except in the breakthrough volume test. The waste water sample was diluted two times by the blank water before extraction due to the high content of analytes and complex matrices.
To verify the adsorptivity of SCAU-1, the amount of sorbents were set at 20, 40, 60, 80 mg when spiked at 5 μg L−1. From Fig. 2a, it can be seen that the recoveries increased slightly before 60 mg and remain nearly stable after 60 mg, which meant that 60 mg is enough for SPE at this content level. Considering the specification of commercial SPE columns, 60 mg was chosen as the final dosage in the next experiment.
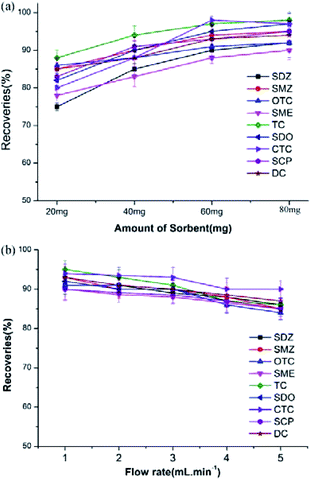 |
| Fig. 2 (a) Effect of the amount of sorbents on the recoveries of antibiotics (conditions: washing solvent, 2 mL water; elution solvent, 2 mL MeOH; spiked level, 5 μg L−1); (b) effect of flow rate on the recoveries of antibiotics (conditions: amount of sorbent, 60 mg; the other conditions were the same as above). | |
Considering the loading and adsorption time, different flow rates were investigated from 1 to 5 mL min−1. As seen from Fig. 2b, the recoveries slightly decreased when improving the flow rate from 1 to 3 mL min−1, and then occur relatively obvious decline. For overall consideration, 3 mL min−1 was selected as the optimum flow rate to ensure good extraction efficiency and shorten the sampling time.
To reduce the matrix effect, three kinds of washing solvents including water, water with 5% methanol, and water with 10% methanol were investigated in detail. Fig. 3a revealed that the recoveries inclined obviously with the increase of methanol content, which meant that the analytes can be easily eluted by methanol. Considering that the selected tic chromatography (EIC) of the waste water has few impurities peaks besides the analytes before 6.5 min, 2 mL water was used as washing solvent to avoid the decrease of recoveries (Fig. 3b).
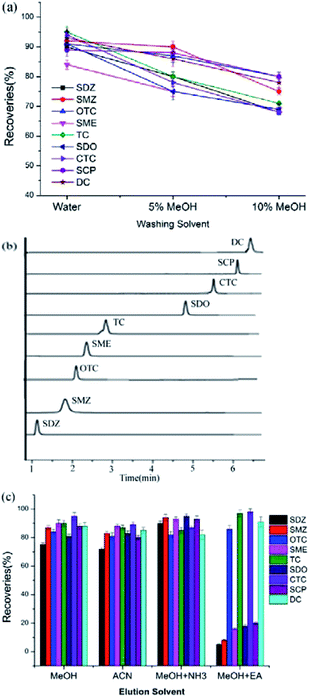 |
| Fig. 3 (a) Effect of washing solvent on the recoveries of antibiotics (conditions: amount of sorbent, 60 mg; elution solvent, 2 mL MeOH; spiked level, 5 μg L−1); (b) the selected ion chromatogram of the diluted waste water sample spiked at 5 μg L−1; (c) effect of elution solvent on the recoveries of antibiotics (conditions: amount of sorbent, 60 mg; washing solvent, 2 mL water; spiked level, 5 μg L−1). | |
To elute the analytes with different content completely, 2 mL methanol, methanol with 5% ammonia (MeOH + NH3), methanol with 5% acetic acid (MeOH + EA), and ACN were selected as elution solvents, respectively. Fig. 3c represents that five SAs can be easily eluted by methanol with 5% ammonia. This may be ascribed that the –NH2 group could interact with the hydroxyl group to form polar forces, and the OH− ion can also reduce the interaction between the sorbent and analytes. For TCs, methanol with 5% acetic acid has better performance than other solvents, due to the polar forces between H+ and phenolic hydroxyl group. To obtain the overall high recoveries, methanol with 5% ammonia was selected as the optimum elution solvent. After eluted with 2 mL preferential solvent, no target peaks can be found in the successive elution for real samples.
The loading capacity and breakthrough volume of the prepared SPE column were also studied. As presented from Fig. S5a,† when 50 mL water sample was spiked at 5, 20, 40 and 60 μg L−1 respectively, the recoveries differed little before 40 μg L−1, and then occur decline with the increase of concentration. Thus the loading capacity was close to 40 μg L− for 50 mL water volume. The breakthrough volume was also tested with the concentration of 1 μg L−1. From Fig. S5b,† it can be seen that the recoveries retained nearly stable before loading of 200 mL, and then slightly decreased, which meant that the breakthrough volume was approximate 200 mL.
Comparison of the extraction efficiency of the prepared COFs and commercial adsorbents
To evaluate the extraction efficiency of SCAU-1, the extraction performance of SCAU-1 was compared with SNW-1 and Waters Oasis HLB under the optimized conditions. The dimension of the three kinds of SPE columns are all 3 mL/60 mg to coincide the commercial specification. As shown in Fig. 4, the two COFs have similar performance and show better recoveries than the commercial HLB SPE columns. For TCs, SCAU-1 shows the best performance. While for SAs, SNW-1 is slightly advantageous than SCAU-1. The reason may lies that SNW-1 can afford strong π–π interaction with the phenyl rings of SAs, while for SCAU-1, the N atoms of the pyrazine ring can afford lone pair electrons and interact with the –OH groups of TCs through polar forces.
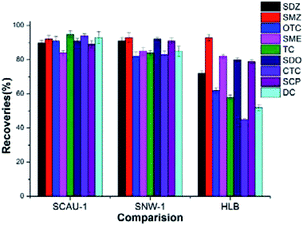 |
| Fig. 4 Extraction efficiency comparison of the prepared COFs with the commercial HLB (conditions: amount of sorbent, 60 mg; washing solvent, 2 mL water; elution solvent, 2 mL MeOH + NH3; spiked level, 5 μg L−1). | |
Analytical performance of the prepared COFs
The structure of the analytes and measurement conditions were listed in Table S1.† The limits of detection (LOD), correlation coefficients, and repeatability were determined under the optimized conditions (Table 1). The linearity range of the method was measured from 1 to 500 μg L−1. The correlation coefficients (R2) were found to be between 0.9983 and 0.9991. The LODs and LOQs were calculated according to KSb/m (K = 3 for LODs, 10 for LOQs), where Sb is the standard deviation of the blank samples and m is the slope of standard curve, respectively. The determined LODs were in the range of 0.031 to 0.55 μg L−1. The LODs for SAs and TCs obtained were lower or close to those reported results (Table 2). From the cost consideration, the expense of monomers adopted in SCAU-1 was relatively low, and the synthesis process was not complex, which favored for the real application in the future.
Table 1 Figures of merit for the established SPE method for the determination of antibiotics
Antibiotics |
Linear range (μg L−1) |
Correlation Coefficient (μg L−1) (R2) |
LODs |
Inter-day assay |
Reusability |
1 μg L−1 |
5 μg L−1 |
20 μg L−1 |
RSDa (%, n = 5) |
Recoveries% |
RSDa (%, n = 5) |
Recoveries% |
RSD (%, n = 3) |
Recoveries% |
RSD (%, n = 3) |
Recoveries% |
RSD (%, n = 3) |
Spiked level at 5 μg L−1. |
SDZ |
1–500 |
0.9987 |
0.031 |
3.9 |
91.2 |
3.4 |
89.1 |
2.3 |
90.5 |
2.5 |
91.0 |
3.4 |
SMZ |
1–500 |
0.9991 |
0.064 |
4.5 |
90.8 |
4.3 |
92.0 |
3.2 |
91.2 |
3.2 |
90.8 |
4.0 |
OTC |
2–500 |
0.9988 |
0.32 |
5.2 |
88.7 |
4.6 |
90.3 |
3.9 |
89.2 |
3.0 |
88.4 |
3.4 |
SME |
1–500 |
0.9991 |
0.43 |
5.0 |
88.9 |
4.8 |
89.6 |
4.3 |
88.0 |
3.5 |
88.2 |
4.6 |
TC |
2–500 |
0.9983 |
0.52 |
5.4 |
91.2 |
5.0 |
92.4 |
3.8 |
92.0 |
2.8 |
91.2 |
3.9 |
SDO |
1–500 |
0.9993 |
0.097 |
4.1 |
92.5 |
3.8 |
93.6 |
4.5 |
93.1 |
2.6 |
91.8 |
3.4 |
CTC |
2–500 |
0.9985 |
0.28 |
5.4 |
92.1 |
4.8 |
92.9 |
4.8 |
93.4 |
4.2 |
92.2 |
3.7 |
SCP |
1–500 |
0.9990 |
0.32 |
6.5 |
90.8 |
5.8 |
92.5 |
3.8 |
91.5 |
4.8 |
90.9 |
4.9 |
DC |
2–500 |
0.9985 |
0.55 |
7.2 |
92.1 |
6.3 |
93.1 |
4.8 |
92.8 |
4.4 |
90.5 |
4.2 |
Table 2 Comparison of the established method with the reported methods for analysis of water samples
SPE materials |
Analysis method |
Linear range (ng mL−1) |
Recoveries |
LODs (μg L−1) |
Ref. |
Covalent organonitridic frameworks |
LC-MS/MS |
0.0025–1 |
80–95.0 |
2–11 |
19 |
Oasis HLB |
LC-MS/MS |
2–1000 |
77.7–99.5 |
0.03–3 |
41 |
Polystyrene porous nanofibers |
LC-MS/MS |
0.125–12.5 |
0.0008–0.005 |
85.8–96.2 |
17 |
TiO2 nanotube arrays |
HPLC |
1–200 |
82.8–101.7 |
0.27–0.6 |
42 |
Magnetic molecularly imprinted polymers |
HPLC |
50–100 000 |
61.2–91.4 |
0.76–1.2 |
43 |
PIL@MPS@Fe3O4 NPs |
HPLC |
4–4000 |
83.5–103.0 |
0.2–1.0 |
44 |
Hybrid monolith PPY/GO/PVA cryogel |
HPLC |
0.1–100 |
85.5–99.0 |
0.10–0.2 |
45 |
TiO2-RG@SiO2@Fe3O4 nanoparticles |
HPLC |
1–200 |
90–108 |
0.2–0.5 |
46 |
Polypyrrole/SiO2/Fe3O4 nanoparticles |
HPLC |
0.3–200 |
86.7–99.7 |
0.3–1.0 |
47 |
Porphyrin-based Fe3O4@COFs |
HPLC |
1–500 |
95.7–107.3 |
0.2–1 |
48 |
SNW-1 |
LC-MS/MS |
1–500 |
81.9–93.3 |
0.031–0.55 |
This method |
SCAU-1 |
LC-MS/MS |
1–500 |
88.0–93.4 |
0.031–0.55 |
This method |
Three spiked levels were tested for the diluted wastewater, and the results were listed in Table 1 When spiked at 5 μg L−1, the inter-day assay (n = 5) were between 3.9% to 7.2%. The reusability were also tested for five cycles after absolute elution and balance of each cycle, and the results represented that the recoveries varied little during the experiment, which showed that the SPE column could be reused at least five times. The established method was also applied to five kinds of real samples (drinking water, river water, lake water, waste water and milk). The results were given in Table 3. The recoveries of the spiked milk were in the range of 82.0–88.2%, which meant that the established could be applied to other complicated matrices.
Table 3 Analytical results (mean ± SD, n = 3) for the determination of antibiotics in real samples
Sample |
Analytes (μg L−1) |
SDZ |
SMZ |
OTC |
SME |
TC |
SDO |
CTC |
SCP |
DC |
Drinking water |
ND |
ND |
ND |
ND |
ND |
ND |
ND |
ND |
ND |
River water (mean ± SD) |
ND |
5.8 ± 0.3 |
8.5 ± 0.5 |
ND |
4.3 ± 0.2 |
ND |
ND |
ND |
ND |
Lake water (mean ± SD) |
ND |
ND |
1.8 ± 0.1 |
ND |
2.6 ± 0.1 |
ND |
ND |
ND |
ND |
Waste water (mean ± SD) |
ND |
9.6 ± 0.5 |
12.0 ± 0.6 |
ND |
15.0 ± 0.7 |
ND |
12.4 ± 0.5 |
ND |
ND |
Milk (mean ± SD) |
ND |
ND |
ND |
ND |
ND |
ND |
ND |
ND |
ND |
Spiked milk (5 μg L−1, mean ± SD) |
4.08 ± 0.11 |
4.21 ± 0.12 |
4.14 ± 0.14 |
4.12 ± 0.10 |
4.22 ± 0.12 |
4.26 ± 0.15 |
4.35 ± 0.09 |
4.21 ± 0.18 |
4.33 ± 0.13 |
Conclusions
A novel porous COF and a contrast material, SCAU-1 and SNW-1 were prepared and successfully used as SPE sorbents to extract nine kinds of antibiotics from the environmental water and milk. Both two sorbents acquired high extraction performance and selectivity towards the analytes compared with the commercial SPE columns, of which SCAU-1 is superior for adsorbing TCs than SNW-1, while for SAs, the SPE performance of the two COFs are very close. However, from the synthesis prospect, the yield of SCAU-1 was higher than SNW-1. And through altering benzene ring to triazine ring, the extraction efficiency of polar analytes was improved. The design strategy can be extended to other preparation of COFs, and motivate the study of main skeleton modification of monomers. With the determination of UPLC-MS/MS, the established method has exhibited low LODs, wide linear ranges and good repeatability. Hence, SCAU-1 is a kind of promising material for the enrichment of organic pollutants with benzene rings.
Conflicts of interest
There are no conflicts to declare.
Acknowledgements
This work was supported by the Youth Teacher Award Foundation of South China Agricultural University [Provided by Guangdong Wen's Dahuanong Biotechnology Co., Ltd; Grant No. 5500-A17003], the National Natural Science Foundation of China (21707128), and Guangdong basic and applied basic research fund-Wen's joint fund project.
References
- I. Becheker, H. Berredjem, N. Boutefnouchet and M. J. Berredjem, Antibacterial activity of four sulfonamide derivatives against multidrug-resistant staphylococcus aureus, J. Chem. Pharm. Res., 2014, 6, 893–899 Search PubMed.
- L. L. Wang, J. Wu, Q. Wang, C. H. He, L. Zhou, J. Wang and Q. S. Pu, Rapid and sensitive determination of sulfonamide residues in milk and chicken muscle by microfluidic chip electrophoresis, J. Agric. Food Chem., 2012, 60, 1613–1618 CrossRef CAS PubMed.
- H. L. Qian, C. X. Yang, W. L. Wang, C. Yang and X. P. Yan, Advances in covalent organic frameworks in separation science, J. Chromatogr. A, 2018, 1542, 1–18 CrossRef CAS PubMed.
- Y. Z. Li, X. W. Wu, Z. Q. Li, S. X. Zhong, W. P. Wang, A. J. Wan and J. R. Chen, Fabrication of CoFe2O4-graphene nanocomposite and its application in the magnetic solid phase extraction of sulfonamides from milk samples, Talanta, 2015, 144, 1279–1286 CrossRef CAS PubMed.
- A. Bialk-Bielinska, S. Stolte, J. Arning, U. Uebers, A. Boschen, P. Stepnowski and M. Matzke, Ecotoxicity evaluation of selected sulphonamides, Chemosphere, 2011, 85, 928–933 CrossRef CAS PubMed.
- S. Goel, Emerging Micro-Pollutants in the Environment: Occurrence. Fate, and Distribution, 2015, vol. 1198, p. 19–42 Search PubMed.
- M. Qiao, G. G. Ying, A. C. Singer and Y. G. Zhu, Review of antibiotic resistance in China and its environment, Environ. Int., 2018, 110, 160–172 CrossRef CAS PubMed.
- I. D. Rafraf, I. Lekunberri, A. Sanchez-melsio and M. Aouni, Abundance of antibiotic resistance genes in five municipal wastewater treatment plants in the Monastir Governorate, Tunisia, Environ. Pollut., 2016, 219, 353–358 CrossRef CAS PubMed.
- Y. Li, Z. Li, W. Wang, S. Zhong, J. Chen and A. J. Wang, Miniaturization of self-assembled solid phase extraction based on graphene oxide/chitosan coupled with liquid chromatography for the determination of sulfonamide residues in egg and honey, J. Chromatogr. A, 2016, 1447, 17–25 CrossRef CAS PubMed.
- Z. Li, Y. Li, M. Qi, S. Zhong, W. Wang, A. J. Wang and J. Chen, Graphene-Fe3O4 as a magnetic solid-phase extraction sorbent coupled to capillary electrophoresis for the determination of sulfonamides in milk, J. Sep. Sci., 2016, 39, 3818–3826 CrossRef CAS PubMed.
- C. F. Poole, New trends in solid-phase extraction, Trends Anal. Chem., 2003, 22, 362–373 CrossRef CAS.
- M. J. García-Galán, T. Garrido, J. Fraile, A. Ginebreda, M. S. Díaz-Cruz and D. Barceló, Application of fully automated online solid phase extraction-liquid chromatography-electrospray-tandem mass spectrometry for the determination of sulfonamides and their acetylated metabolites in groundwater, Anal. Bioanal. Chem., 2011, 399, 795–806 CrossRef PubMed.
- M. J. García-Galán, M. S. Díaz-Cruz and D. Barceló, Determination of 19 sulfonamides in environmental water samples by automated on-line solid-phase extraction-liquid chromatography–tandem mass spectrometry (SPE-LC–MS/MS), Talanta, 2010, 81, 355–366 CrossRef PubMed.
- J. Han, Y. Wang, Y. Liu, Y. F. Li, Y. Lu, Y. S. Yan and L. Ni, Ionic liquid-salt aqueous two-phase extraction based on salting-out coupled with high-performance liquid chromatography for the determination of sulfonamides in water and food, Anal. Bioanal. Chem., 2013, 405, 1245–1255 CrossRef CAS PubMed.
- A. V. Herrera-Herrera, J. Hernández-Borges, M. M. Afonso, J. A. Palenzuela and M. Á. Rodríguez-Delgado, Comparison between magnetic and nonmagnetic multi-walled carbon nanotubes-dispersive solid phase extraction combined with ultra-high performance liquid chromatography for the determination of sulfonamide antibiotics in water samples, Talanta, 2013, 116, 695–703 CrossRef CAS PubMed.
- R. Chen, Y. Y. Yang, N. Wang, L. J. Hao, L. Li, X. Y. Guo, J. C. Zhang, Y. Z. Hu and W. Y. Shen, Application of packed porous nano fibers solid-phase extraction for the detection of sulfonamide residues from environmental water samples by ultra high performance liquid chromatography with mass spectrometry, J. Sep. Sci., 2015, 38, 749–756 CrossRef CAS PubMed.
- J. He, H. Z. Tang, L. Q. You, H. J. Zhan, J. Zhu and K. Lu, Fragment imprinted microspheres for the extraction of sulfonamides, Microchim. Acta, 2013, 180, 903–910 CrossRef CAS.
- G. J. Xu, B. B. Zhang, X. L. Wang, N. Li, Y. F. Zhao, L. Liu, J. M. Lin and R. S. Zhao, Porous covalent organonitridic frameworks for solid-phase extraction of sulfonamide antibiotics, Microchim. Acta, 2019, 186, 26–33 CrossRef PubMed.
- L. Chen, D. Yin, Z. C. Yang, W. D. Zhao, Y. L. Chen, W. F. Zhang and S. S. Zhang, J. Anal. Methods Chem., 2019, 2019, 1–13 Search PubMed.
- M. Čizmićv, S. Babić and M. Kaštelan-Macan, Multi-class determination of pharmaceuticals in wastewaters by solid-phase extraction and liquid chromatography tandem mass spectrometry with matrix effect study, Environ. Sci. Pollut. Res., 2017, 24, 20521–20539 CrossRef PubMed.
- S. Y. Ding and W. Wang, Covalent Organic Frameworks (COFs): From Design to Applications, Chem. Soc. Rev., 2013, 42, 548–568 RSC.
- X. Feng, X. Ding and D. Jiang, Covalent organic frameworks, Chem. Soc. Rev., 2012, 41, 6010–6022 RSC.
- A. P. Cote, A. I. Benin, N. W. Ockwig, M. O'Keeffe, A. J. Matzger and O. M. Yaghi, Porous, Crystalline, Covalent Organic Frameworks, Science, 2005, 310, 1166–1170 CrossRef CAS PubMed.
- S. Kandambeth, V. Venkatesh, D. B. Shinde, S. Kumari, A. Halder, S. Verma and R. Banerjee, Self-templated chemically stable hollow spherical covalent organic framework, Nat. Commun., 2015, 6, 6786–6795 CrossRef CAS PubMed.
- P. Zhang, Z. H. Weng, J. Guo and C. C. Wang, Solution-dispersible, colloidal, conjugated porous polymer networks with entrapped palladium nanocrystals for heterogeneous catalysis of the Suzuki–Miyaura coupling reaction, Chem. Mater., 2011, 23, 5243–5249 CrossRef CAS.
- R. Babarao and J. W. Jiang, Exceptionally high CO2 storage in covalent-organic frameworks: Atomistic simulation study, Energy Environ. Sci., 2008, 1, 139–143 RSC.
- D. P. Cao, J. H. Lan, W. Wang and B. Smit, Lithium-doped 3D covalent organic frameworks: high-capacity hydrogen storage materials, Angew. Chem., Int. Ed., 2009, 48, 4730–4733 CrossRef CAS PubMed.
- M. L. Chen, Y. L. Liu, X. W. Xing, X. Zhou, Y. Q. Feng and B. F. Yuan, Preparation of a hyper-cross-linked polymer monolithic column and its application to the sensitive determination of genomic DNA methylation, Chem.–Eur. J., 2013, 19, 1035–1041 CrossRef CAS PubMed.
- C. X. Yang and X. P. Yan, Application of covalent-organic frameworks in chromatography and sample pretreatment, Chin. J. Chromatogr., 2018, 36(11), 1075–1080 CrossRef CAS PubMed.
- C. J. Zhang, G. K. Li and Z. M. Zhang, A hydrazone covalent organic polymer based micro-solid phase extraction for online analysis of trace Sudan dyes in food samples, J. Chromatogr. A, 2015, 1419, 1–9 CrossRef CAS PubMed.
- S. J. He, T. Zeng, S. H. Wang, H. Y. Niu and Y. Q. Cai, Facile synthesis of magnetic covalent organic framework with three-dimensional bouquet-like structure for enhanced extraction of organic targets, ACS Appl. Mater. Interfaces, 2017, 9, 2959–2965 CrossRef CAS PubMed.
- M. G. Schwab, B. Fassbender, H. W. Spiess, A. Thomas, X. L. Feng and K. Müllen, Catalyst-free Preparation of Melamine-Based Microporous Polymer Networks through Schiff Base Chemistry, J. Am. Chem. Soc., 2009, 131, 7216–7217 CrossRef CAS PubMed.
- G. Yang, H. Han, C. Du, Z. Luo and Y. Wang, Facile synthesis of melamine-based porous polymer networks and their application for removal of aqueous mercury ions, Polymer, 2010, 51, 6193–6202 CrossRef CAS.
- W. Zhang, L. Qiu, Y. Yuan, A. Xie, Y. Shen and J. Zhu, Microwave-assisted synthesis of highly fluorescent nanoparticles of a melamine-based porous covalent organic framework for trace-level detection of nitroaromatic explosives, J. Hazard. Mater., 2012, 221, 147–154 CrossRef PubMed.
- C. Chen, J. Kim and W. Ahn, Efficient carbon dioxide capture over a nitrogen-rich carbon having a hierarchical micro-mesopore structure, Fuel, 2012, 95, 360–364 CrossRef CAS.
- J. L. Pan, S. Jia, G. K. Li and Y. L. Hu, Organic building block based microporous network SNW-1 coating fabricated by multilayer interbridging strategy for efficient enrichment of trace volatiles, Anal. Chem., 2015, 87, 3373–3381 CrossRef CAS PubMed.
- Z. M. Yan, B. Q. Hua, Q. Li, S. X. Zhang, J. Pang and C. H. Wu, Facile synthesis of covalent organic framework incorporated electrospun nanofiber and application to pipette tip solid phase extraction of sulfonamides in meat samples, J. Chromatogr. A, 2019, 1584, 33–41 CrossRef CAS PubMed.
- X. M. Wang, G. J. XU, X. L. Wang, X. Wang, X. L. Wang, R. S. Zhao and J. M. Lin, Melamine/o-phthalaldehyde covalent organic frameworks for solid phase extraction-liquid chromatography-tandem mass spectrometry analysis of sulfonamide antibiotics in environmental samples, Chin. J. Anal. Chem., 2018, 46(12), 1990–1996 CAS.
- Y. X. Li, H. N. Zhang, Y. T. Chen, L. Huang, Z. Lin and Z. W. Cai, Core-shell structured magnetic covalent organic framework nanocomposites for triclosan and triclocarban adsorption, ACS Appl. Mater. Interfaces, 2019, 11(25), 22492–22500 CrossRef CAS PubMed.
- F. S. Pan, M. D. Wang, H. Ding, Y. M. Song, W. D. Li, H. Wu, Z. Y. Jiang, B. Y. Wang and X. Z. Cao, Embedding Ag+@COFs within Pebax membrane to confer mass transport channels and facilitated transport sites for elevated desulfurization performance, J. Membr. Sci., 2018, 552, 1–12 CrossRef CAS.
- Y. F. Chai, Y. Q. Feng, Y. X. Zhang, M. X. Chen, R. Wang, M. M. Liu and Y. S. Wei, Optimization of an analytical method for the simultaneous determination of 24 antibiotics in swine wastewater, Environ. Chem., 2017, 36(10), 2147–2154 CAS.
- Q. Zhou and Z. Fang, Highly sensitive determination of sulfonamides in environmental water samples by sodium dodecylbenzene sulfonate enhanced microsolid phase extraction combined with high performance liquid chromatography, Talanta, 2015, 141, 170–174 CrossRef CAS PubMed.
- X. Xie, X. Liu, X. Pan, L. Chen and S. Wang, Surface-imprinted magnetic particles for highly selective sulfonamides recognition prepared by reversible addition fragmentation chain transfer polymerization, Anal. Bioanal. Chem., 2016, 408, 963–970 CrossRef CAS PubMed.
- H. Wu, Y. Shi, X. Guo, S. Zhao, J. Du, H. Jia, L. He and L. Du, Determination and removal of sulfonamides and quinolones from environmental water samples using magnetic adsorbents, J. Sep. Sci., 2016, 39, 4398–4407 CrossRef CAS PubMed.
- K. Chullasat, P. Nurerk, P. Kanatharana, P. Kueseng, T. Sukchuay and O. Bunkoed, Hybrid monolith sorbent of polypyrrole-coated graphene oxide incorporated into a polyvinyl alcohol cryogel for extraction and enrichment of sulfonamides from water samples, Anal. Chim. Acta, 2017, 961, 59–66 CrossRef CAS PubMed.
- M. Izanloo, A. Esrafili, M. Behbahani, M. Ghambarian and H. R. Sobhi, Trace quantification of selected sulfonamides in aqueous media by implementation of a new dispersive solid-phase extraction method using a nanomagnetic titanium dioxide graphene-based sorbent and HPLC-UV, J. Sep. Sci., 2018, 41, 910–917 CrossRef CAS PubMed.
- T. Sukchuay, P. Kanatharana, R. Wannapob, P. Thavarungkul and O. Bunkoed, Polypyrrole/silica/magnetite nanoparticles as a sorbent for
the extraction of sulfonamides from water samples, J. Sep. Sci., 2015, 38, 3921–3927 CrossRef CAS PubMed.
- J. Zhang, Z. P. Chen, S. Tang, X. G. Luo, J. B. Xi, Z. L. He, J. X. Yu and F. S. Wu, Fabrication of porphyrin-based magnetic covalent organic framework for effective extraction and enrichment of sulfonamides, Anal. Chim. Acta, 2019, 1089, 66–77 CrossRef CAS PubMed.
Footnote |
† Electronic supplementary information (ESI) available. See DOI: 10.1039/c9ra10846g |
|
This journal is © The Royal Society of Chemistry 2020 |
Click here to see how this site uses Cookies. View our privacy policy here.