DOI:
10.1039/C9RA10798C
(Paper)
RSC Adv., 2020,
10, 14794-14802
In vitro prebiotic activities of oligosaccharides from the by-products in Ganoderma lucidum spore polysaccharide extraction
Received
22nd December 2019
, Accepted 30th March 2020
First published on 14th April 2020
Abstract
Until recently, a variety of oligosaccharides from fruits, vegetables and mushrooms have demonstrated positive prebiotic effects. Ganoderma lucidum, a well-known traditional medicine and tonic in East Asia, has been utilized in the prevention and treatment of a broad range of illnesses. In this study, each of three oligosaccharides was obtained from the polysaccharide extraction by-products of sporoderm: the unbroken and broken spores of Ganoderma lucidum (UB-GLS, B-GLS). Their molecular weight distribution, monosaccharide composition and preliminary structures were analyzed using gel permeation chromatography (GPC), GC-MS, UV and FTIR, respectively. All of the oligosaccharides were found to exhibit prebiotic activities, evaluated by detecting growth stimulation on Lactobacillus in vitro. Among these, UB-O80 and B-O80 displayed the most significant effects (p < 0.05) in these groups, and UB-O80 showed higher resistance to hydrolysis by artificial human gastric juice compared with inulin, giving a maximum hydrolysis rate of 1.65%. Compared with inulin media, Lactobacillus also revealed high tolerance to lower pH levels and simulated gastric juices in UB-O80 and B-O80 media. Compared with a control in gut microbiota fermentation, the abundance of some beneficial bacteria increased and some harmful bacteria declined in the groups of UB-O80 and B-O80. In conclusion, the results suggest that GLS oligosaccharides could be exploited as promising prebiotics for the enhancement of human health.
1. Introduction
Nowadays, the gut microbiota is regarded as a “new organ” of the human body,1 whose cells are approximately tenfold greater in number than human cells. This biome colonizes in the intestinal tract, forms a mutual symbiotic relationship with the host, and plays an important role in the metabolism and immune systems. For instance, the intestinal flora of patients suffering from diabetes, hypertension and other metabolic diseases is quite different from that of healthy people.2 Intestinal microorganisms are normally divided into beneficial, harmful and neutral bacteria. Bifidobacterium and Lactobacillus are the two principal beneficial/probiotic bacteria of the intestinal microflora due to their health promoting properties; they make up about 25% of the total number of gut bacteria.3 Organic acids including lactic acids are produced by Lactobacilli fermentation, and acid-induced environment in the colon inhibit the growth of pathogens. These also include boosting immune function, improving digestion and eliminating fecal, and reducing the likelihood of irresistible bowel syndrome (IBS).4 Many studies confirm that the selective addition of certain substances to the diet is a valid approach to the dietary manipulation of the colonic microbiota, and substances are referred to as prebiotics.4
To date, some carbohydrates extracted from fruits, vegetables, mushrooms have been reported as being potential sources of natural prebiotics.5,6 Ganoderma lucidum (G. lucidum), a species belonging to the macro-fungi, has a long history of application as traditional medicine and tonic with its health-promoting properties in East Asia.7 Modern pharmacological studies have verified that G. lucidum is involved in anti-tumor, hypoglycemic, immunomodulation, liver protection, and other activities.8,9 It contains a variety of functional components, including polysaccharides, fatty acids, peptides, triterpenoids, organic germanium, adenosines and inorganic elements.10 The polysaccharides of G. lucidum can promote the growth of probiotics and alleviate colorectal cancer by altering of special gut bacteria.8,11 It helps reduce the abundance of potential pathogenic bacteria; additionally, G. lucidum polysaccharides have been found to significantly increase the numbers of several beneficial bacteria for anti-obesity SCFA-production in mice.12
The Ganoderma lucidum spores (GLS) contains all the genetic material and health-care effects of G. lucidum. They are the essential parts of G. lucidum and have been widely used for medicinal and functional food products in China. Nevertheless, their broken spores have been found to provide better absorption and therapeutic effects than unbroken spores, as the genetic material and biological composition were wrapped in two outer layer walls mainly consisting of chitin, which is extremely difficult for the alimentary tract to digest.13,14 In the alcohol precipitation process of edible fungus polysaccharide extraction, the resulting supernatant is rich in oligosaccharides components. It is generally directly discharged as waste, which not only affects the environment, but also produces waste resources. Studies confirmed that different soluble glucans extracted from oyster mushrooms can stimulate the growth of colon microorganisms.15 The fermentable fiber as well as oligosaccharides from mushrooms act as the prebiotics.16 However, there are still few studies on the prebiotics of GLS oligosaccharides, which do exist in the resulting supernatant of polysaccharide extractions.
In this study, each of the three GLS oligosaccharides were individually obtained from the supernatants of UB-GLS and B-GLS polysaccharide extraction by different ethanol concentration precipitation methods. The basic structure of the oligosaccharides was characterized, and the in vitro effects of GLS oligosaccharides on the proliferation of probiotics (Lactobacillus) were assessed by determination the changes in optical density (OD), artificial human gastric juice hydrolysis and their tolerance to lower pH levels in simulated gastric juice, the effects on gut microbiota were assessed by fermentation in vitro. This investigation might provide a promising way to avoid the vast production of by-products by the polysaccharide industry.
2. Materials and methods
2.1. Samples and chemicals
UB-GLS and B-GLS were provided by Shouxiangu Pharmaceutical Co. Ltd (Zhejiang, China). Inulin was purchased from Inbo Biotechnology Co. Ltd. Lactobacillus (Lactobacillus acidophilus) was purchased from the China Centre of Industrial Culture Collection (CICC, 6095) and was preserved by Microbiology Laboratory, Zhejiang University of Technology. It was cultured on MRS media and preserved for further use at 4 °C. Dialysis membranes (Mw cutoff = 100–500 Da) were obtained from Shanghai Yuanye Biotechnology Co. Ltd. Monosaccharide standards, TFA, NaBH4 and pepsin were purchased from Aladdin Chemistry Co. Ltd. Takara Ex Taq were purchased from Takara Bio Technology Co. Ltd. Other reagents were of analytical grade.
2.2. Extraction
Briefly, 200 g of GLS (UB-GLS, B-GLS) was soaked in 95% ethanol for 24 h, followed by forced air-drying at room temperature. They were then extracted by hot water bath at 90 °C for 3 h and centrifuged at 8000 × g for 15 min at 4 °C (CR21N; Hitachikoki, Japan). The supernatant was subsequently concentrated to a volume of one-fourth of its initial value, under reduced pressure and with a rotary evaporator at 50 °C, and then precipitated in sequence by 10-fold (v/v) 80%, 50%, 30% ethanol (final concentration v/v) at 4 °C for 24 h. The supernatant, UB-GLS and B-GLS oligosaccharides (UB-O30, UB-O50, UB-O80, B-O30, B-O50, B-O80) were collected by centrifuge at 8000 × g for 15 min and subjected to dialysis for 48 h at 16 °C. Finally, the retentate was concentrated and lyophilized with a freeze-dryer (Virtis BTP; Genevac, Britain) and stored in a desiccator until use. The sugar content was analyzed by the phenol-sulfuric method using glucose as the reference standard.17 The protein content was determined by using bovine serum albumin (BSA) as the standard to check its level of purity.18 The phenol content was quantified by the Folin–Ciocalteu method.19
2.3. Determination of molecular weight
The molecular weights (Mw) of the GLS oligosaccharides were determined by GPC (2695; Waters, USA) with an Agilent 1260 differential detector.6 The analytical system consisted of Ultra hydrogel 500 columns (7.8 × 300 mm; Waters, USA), Ultra hydrogel 250 columns (7.8 × 300 mm; Waters, USA), and Ultra hydrogel 120 columns (7.8 × 300 mm; Waters, USA), and connected in series by decreasing pore sizes. The mobile phase was 0.1 M in potassium nitrate and the flow rate was 1.0 mL min−1. The column temperature was fixed at 40 °C. Standards and samples were filtered through a 0.22 μm syringe filter and the injection volume was 40 μL. The molecular weight was calibrated with a series of polyethylene glycol standards. The Mws of the samples was determined by comparing the retention time with the standard curves.
2.4. Determination of monosaccharide composition
The monosaccharide composition was analyzed by GC-MS (Trace 1300-ISQ; Thermo, USA) with TG-5 chromatographic column.20–22 The sample (2 mg) was dissolved in 2 M TFA (6 mL), and hydrolyzed at 110 °C for 2 h. The TFA was completely removed by vaporizing it with methanol under reduced pressure. The residue was dissolved in distilled water (3 mL), and NaBH4 (25 mg) was added. After 3 h, the pH of solution was adjusted to 4.5 ± 0.2 either with 0.5 M hydrochloric acid or sodium hydroxide. The solution was evaporated to dryness by the addition of methanol. Acetic anhydride (4 mL) was added to the evaporation residue for acetylation at 100 °C for 1 h, and then the residue was evaporated to dryness level by the addition of toluene. It was dissolved in chloroform and added to distilled water to remove the water. The chloroform solution was filtered using an organic phase microfiltration membrane (0.22 μm) and analyzed by GC-MS. The carrier gas (helium) flow rate was at 1.0 mL min−1. The column was kept at 120 °C for 1 min, and then increased from 120 °C to 165 °C at 10 °C min−1, 1 min at 165 °C, from 165 °C to 169 °C at 1 °C min−1 and 0.5 min at 169 °C, 169 °C to 196 °C at 10 °C min−1 and 0.5 min at 196 °C, 196 °C to 200 °C at 1 °C min−1 and 1 min at 200 °C, from 200 °C to 240 °C at 10 °C min−1 and 5 min at 240 °C. The temperature of injector and detector were both set at 250 °C and the injection volume was 1.0 μL. Rhamnose, fucose, arabinose, xylose, mannose, glucose and galactose were used as monosaccharide standards. The qualitative analysis of sugar in the sample was determined by comparison to the retention time of standard sugar. The concentration of sugar in the sample was calculated by a comparison with the peak area of the standard curve of the respective sugar.
2.5. Fourier transform infrared (FTIR) and ultraviolet (UV) characterization of oligosaccharides
The structures of the GLS oligosaccharides were assayed by FTIR (Nicolet iS50; Thermo, USA). The samples were mixed with KBr (1
:
100 v/v), and pressed into tablets. The spectra were recorded from 400–4000 cm−1 with a resolution of 4 cm−1 at room temperature.1
For UV analysis, the sample was dissolved in distilled water and scanned from 200–500 nm using a UV-visible spectrophotometer (TU-1900; Pgeneral, China).
2.6. Prebiotic activity
The pH of the fermentation broth was measured with a pH meter, which was calibrated using buffers of pH 4.01, pH 6.86 and pH 9.18.
GLS oligosaccharides and positive prebiotic control (inulin) were used as the individual carbon sources for the growth of Lactobacillus in MRS medium, and the total sugar content was added to different mediums from extracts of the same level (1%, w/v). The fermentation broth was adjusted to pH 6.8 ± 0.1, either with 0.5 M hydrochloric acid or sodium hydroxide. The media were dispensed as 10 mL aliquots into test tubes with stoppers and then sterilized by autoclaving them at 121 °C for 30 min. After subculturing them twice in MRS medium at 37 °C for 24 h, the logarithmic phase culture of Lactobacillus was obtained by inoculating the MRS medium at 37 °C for 12 h. Then, 0.1 mL logarithmic culture of Lactobacillus was added to the sample liquid for fermentation. The growth of the probiotics was evaluated by increased turbidity after 0, 6, 12, 18, 24 and 48 h at 37 °C and measured by OD at 600 nm.23 The change of sugar concentration was analyzed by the phenol-sulfuric method after 0, 6, 12, 18, 24 and 48 h.18
2.7. The effects of artificial human gastric juice hydrolysis
Prebiotics must be able to withstand the digestive process before reaching the colon, and it is preferable for them to persist throughout the large intestine to also benefit its distal end. Therefore, the tolerance of prebiotics to gastric acid, mammalian enzymatic hydrolysis and gastrointestinal absorption were tested.24 The non-digestibility of the carbohydrates does not mean that they are completely indigestible. The digestibility of the GLS oligosaccharides were studied for gastric juice in comparison with inulin. Human gastric juice was simulated with a hydrochloric acid buffer containing (g L−1): NaCl, 8.0; KCl, 0.20; Na2HPO4·2H2O, 8.25; NaHPO4, 14.35; CaCl2·2H2O, 0.10; and MgCl2·6H2O, 0.18.4,6 The pH of the buffer was adjusted to 1.0, 2.0, 3.0, 4.0 and 5.0 using 5 M hydrochloric acid. The GLS oligosaccharides were dissolved in distilled water to give a 1.0% (the content of sugar, w/v) solution. Afterwards, 5 mL of buffer was added to the 5 mL of oligosaccharides solution at each different pH, and incubated in a water bath at 37 ± 1 °C for 6 h. Sampling were done periodically at 0, 0.5, 1, 2, 4 and 6 h. Reducing sugar concentration was determined by DNS25 and the total sugar content was determined by the phenol-sulphuric acid method.17 The hydrolysis rate of the sample was calculated according to the liberated amount of reducing sugar and the total sugar content, and presented as the following formula.
2.8. Tolerance tests of lower pH and simulated gastric juice
The composition of the gastroenteric environment tolerance test medium was similar to that of the fermentation medium. Acid buffers and simulated gastric juice were prepared according to the method reported by Huang.26 In the acid tolerance test, the pH of fermentation medium was adjusted to 1.5, 2.0, 2.5 and 3.0. Lactobacillus was incubated in different pH mediums. The growth rate of Lactobacillus was measured by changes in the OD value following anaerobic culture for 0, 2 and 4 h. In the simulated gastric juice tolerance test, Lactobacillus was incubated in mediums with 0.5% pepsin at pH 3.0.27 The growth of Lactobacillus was monitored by OD following anaerobic culture for 0, 1, 2, 4 and 6 h.
2.9. Fermentation in vitro
Fecal samples were collected from ten healthy human (five women, five men). All experiments were performed in accordance with the Guidelines of Biomedical Research Ethics Review Method Involving People (China), and approved by the Ethics Committee at Zhejiang University of Technology. Informed consents were obtained from human participants of this study. The basic medium contained the followings: 0.45 g per L KH2PO4; 0.45 g per L K2HPO4; 0.05 g per L NaCl; 0.064 g per L CaCl2·2H2O; 0.09 g per L MgSO4·7H2O; 10 g per L tryptone; 2.5 g per L yeast extract; 1.0 g per L l-cysteine; 2.0 mL per L heme; 200 μL per L vitamin. In order to assess the utilization of GLS oligosaccharides by the human fecal microbiome, 8.0 g L−1 each of UB-O80 and B-O80 were added into the growth medium as the sole carbon source. The pH value of fermentation medium was adjusted to 6.5, 5.0 mL of test medium was dispensed into a 10 mL-bottle under anaerobic conditions.28
Fecal samples (0.8 g) were homogenized with 8.0 mL of 0.1 M anaerobic phosphate-buffered saline (pH 7.0) using an automatic fecal homogenizer (Halo Biotechnology Co. LTD., Jiangsu, China) to make a 10% (w/v) slurries. Then 500 μL of slurries was added to the blank (control), UB-O80 and B-O80 medium, and incubated at 37 ± 1 °C for 24 h. Immediately afterward, 1.0 mL aliquots were removed for further analysis.
The removed aliquots were centrifuged, and the pellet was stored at −80 °C. The DNA of samples was extracted by DNA extraction kit (Q328520, Thermo, USA), and then the purity and concentration of the DNA was detected by agarose gel electrophoresis (HE-120, Tanon, China). Then an appropriate amount of sample was taken into the centrifuge tube, and the diluted genomic DNA was used as the template, the specific primers with Barcode and Takara Ex Taq high-fidelity enzyme were used for PCR amplification (580BR10905, Bio-Rad, USA) and sequencing according to the selection of sequencing area.12
2.10. Statistical analysis
All graphs were plotted using Graphpad Prism 6. The statistical analysis was performed with SPSS 20.0. One-way analysis of variance and Duncan's multiple range tests were used to determine the differences of diverse samples, and p < 0.05 was considered statistically significant.
3. Results and discussion
3.1. The preparation results of oligosaccharides
GLS oligosaccharides (UB-O30, UB-O50, UB-O80, B-O30, B-O50 and B-O80) were obtained from the by-products of the polysaccharide extracts from UB-GLS and B-GLS. The yields of the investigated oligosaccharides were shown in Table 1. It was found that the yields of the oligosaccharides decreased as the alcohol concentration increased (30% > 50% > 80%). Compared with different groups, under equal conditions, the results of B-GLS was significantly higher (p < 0.05) than that of UB-GLS, which is attributed to the higher carbohydrate dissolution rates of the species' broken as opposed to unbroken walls. The oligosaccharides possessed moisture levels of about 8.2–11.5%, a minor percentage of protein (2.6–5.2%) and polyphenol (1.8–3.4%); the total sugar content of the extracts was 67.3–75.7%.
Table 1 Yields of different oligosaccharides obtained from UB-GLS and B-GLS
Oligosaccharides |
Yieldb (%) |
UB-GLS |
B-GLS |
Different letters in the same column (A, B, C) or row (a, b) imply significant difference (P < 0.05). . |
30 |
1.83 ± 0.21Aaa |
2.34 ± 0.35Ab |
50 |
1.57 ± 0.34Ba |
1.96 ± 0.29Bb |
80 |
1.31 ± 0.27Ca |
1.64 ± 0.27Cb |
3.2. The molecular weight distribution of oligosaccharides
The Mw distribution of the GLS oligosaccharides was analyzed by GPC as shown in Fig. 1. The results revealed that the degree of GLS oligosaccharides polymerization was about 2–7, which was in nearly the same range as some fructooligosaccharides, xylooligosaccharide, mannan and galactan.6 Some prebiotic oligosaccharides from plants, such as honeysuckle, dragon fruit, dahlia, oat and onion, also contain oligosaccharides of comparable Mw range to those in our study.29 The results revealed that some the values of 30% alcohol extraction were greater than 50% and 80% in alcohol precipitation, which was attributed to the observation that the higher the alcohol concentration, the more easily the carbohydrates with larger molecular weight were precipitated by the alcohol. As a result, the lower molecular weight remain in the supernatants and the Mw of the extracts were more homogeneous.
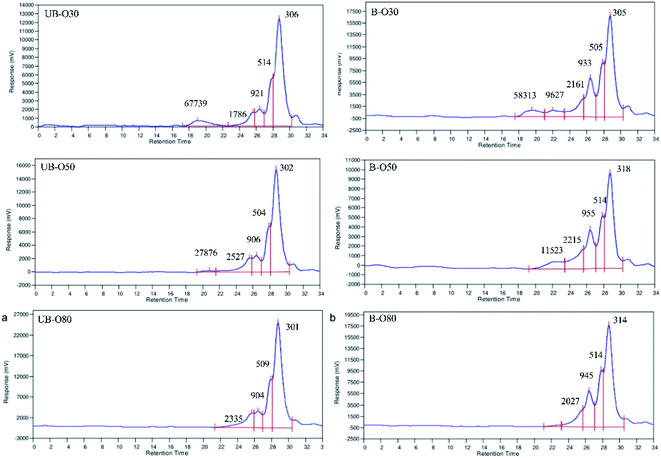 |
| Fig. 1 Molecular weight distributions of different GLS oligosaccharides in GPC profiles: (a) UB-GLS oligosaccharides; (b) B-GLS oligosaccharides. | |
3.3. The monosaccharide composition of oligosaccharides
Monosaccharide composition and molar ratio play an important role in oligosaccharide biological activity. The monosaccharide composition of the GLS oligosaccharides is shown in Table 2. Standard monosaccharide peaks in sequence were determined as being rhamnose, fucose, arabinose, xylose, mannose, glucose and galactose. The monosaccharide composition of the oligosaccharides was determined by comparison to the retention time of standard sugar. The results revealed that the monosaccharide composition of UB-GLS oligosaccharides was similar to that of B-GLS oligosaccharides, but the molar ratio of the UB-GLS oligosaccharides differs. Both UB-GLS and B-GLS oligosaccharides contained the monosaccharides of arabinose, mannose, glucose and galactose, where glucose was the most abundant. The monosaccharide composition of UB-O80 was arabinose, mannose, glucose and galactose in a molar ratio of 0.9
:
5.1
:
11.9
:
0.01, whereas the monosaccharide composition of B-O80 was arabinose, mannose, glucose and galactose in a molar ratio of 4.5
:
11.1
:
21.6
:
0.1. These were different from that the main monosaccharide composition and its molar ratio of GLS as reported in other literature. The polysaccharides of both broken and unbroken spores prepared by ultrasonic vibration or reflux consisted of rhamnose, arabinose, mannose, glucose, and galactose, although there was a small difference in their relative content.30 This difference was possibly attributed to variations in the growth environments of G. lucidum and the oligosaccharide preparation methods.
Table 2 Monosaccharide composition of different GLS oligosaccharides
Monosaccharide |
GLS oligosaccharides (in molar ratio) |
UB-O30 |
UB-O50 |
UB-O80 |
B-O30 |
B-O50 |
B-O80 |
Arabinose |
0.9 |
1.0 |
0.9 |
7.1 |
4.6 |
4.5 |
Mannose |
4.6 |
3.20 |
5.1 |
10.8 |
12.3 |
11.1 |
Glucose |
9.4 |
10.5 |
11.9 |
27.1 |
22.7 |
21.6 |
Galactose |
1.0 |
1.0 |
0.01 |
1.0 |
1.0 |
0.1 |
3.4. FTIR and UV spectrum analysis
FTIR is a non-destructive and rapid technique that can recognize different functional groups and is very sensitive to dissimilarities occurring in molecular structures. FTIR spectrum was used to identify the preliminary structures in the functional groups of the oligosaccharides based on peak vibration values in the regions of inferred radiation. The FTIR spectra (Fig. 2a and b) demonstrated that the graphs of the GLS oligosaccharides were basically consistent with typical carbohydrate vibrational bands.31 Peaks at around 3400 cm−1 were due to the hydroxyl tensile vibration modes, and the peaks at around 2936 cm−1 indicated the C–H stretch absorption of sugars. Bands between 1200–1500 cm−1 were mainly caused by C–OH bending and C–H deformation vibrations.32 The peaks at 950–1200 cm−1 were attributed to the stretching vibrations of heavy atoms C–C and C–O in the pyran ring.33,34 The band at 1040 cm−1 corresponded to the stretching vibration of C–O–C. The absorption peak at 540 cm−1 was characteristic of a pyran-type sugar ring. The occurrence of amide-based vibrations at 1640 cm−1 (due to Amide I) also indicated the presence of binding peptides in these oligosaccharides.35 The peaks at 1408 cm−1 might refer to phenols.36
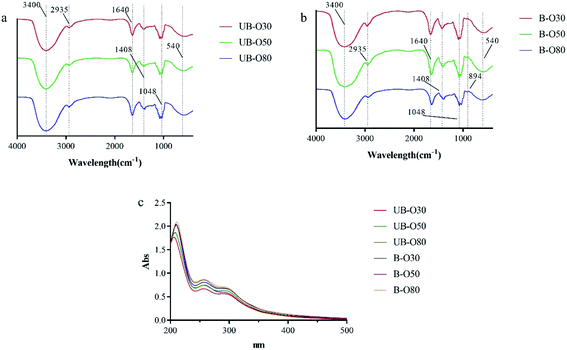 |
| Fig. 2 FTIR and UV spectra of different GLS oligosaccharides: (a) FTIR spectra of UB-GLS oligosaccharides; (b) FTIR spectra of B-GLS oligosaccharides; (c) UV spectra of UB-GLS and B-GLS oligosaccharides. | |
In carbohydrate analysis using FTIR spectra, α and β conformers can be clearly distinguished within the anomeric region vibrational bands since the α- and β-configurations are well separated in the 950 to 750 cm−1 regions, where 870–840 cm−1 corresponds to α conformers, while the β conformers lie at around 890 cm−1.31 As compared to UB-GLS, there is a significant change in bands of the (B-GLS) spectra. The weaker band found near this region (896 cm−1 in BSCP) was believed to indicate the presence of β-glucan, therefore, the band adjacent to 894 cm−1 was reported as being a β-glucosidic bond.31
UV spectra for GLS oligosaccharides (UB-O30, UB-O50, UB-O80, B-O30, B-O50 and B-O80) were shown in Fig. 2c. The absorption in 260–280 nm indicated that the crude oligosaccharides might contain some nucleic acids or protein, which was in coincidence with the results of FTIR. And the contents of the B-GLS oligosaccharides were higher than that of the UB-GLS oligosaccharides, which was attributed to the higher dissolution of genetic material and biological composition in B-GLS as compared to UB-GLS.
3.5. The growth of Lactobacillus
Lactobacillus is a probiotic microorganism that is beneficial to the functional food industry.37 As our previous work38 indicated that the trend of OD value seemed very consistent with that of viable cell count. So GLS oligosaccharides were used as the individual carbon source for the cultivation of Lactobacillus, and the growth of Lactobacillus (Fig. 3a and b) was recorded by changes of optical density (OD). It was found that all of the oligosaccharides, as comparable to inulin, had a significant effect on the genus' growth of Lactobacillus. Lactobacillus showed an outstanding growth rate of following 6 h of fermentation using UB-O30, UB-O50, UB-O80, B-O30, B-O50 and B-O80 as its carbon source, as opposed to inulin, which gave slower growth rates. The growth of Lactobacillus in UB-O30 and B-O30 mediums was slower after 24 h than that of inulin, and groups 80 was significantly higher (P < 0.05) than that of groups 50 and 30. Statistical significance was observed between the groups UB-GLS and B-GLS. Regarding growth rates, rapid increases were observed at approximately 6 h post-inoculation after which the groups entered a logarithmic growth period. They achieved a balance after 24 h post-inoculation. The change of sugar content during culture was shown in Fig. 3c and d, which was identical to the trend for Lactobacillus. It achieved a balance within 24 h. Our results were similar to those with low molecular weight carbohydrates extracted from fruits and vegetables, which could stimulate the growth of Lactobacilli and Bifidobacterium.39 Jackfruit seed oligosaccharides had been reported as stimulating the growth of Lactobacilli and Bifidobacterium. A similar result was observed on gluco-oligosaccharides produced by G. oxydans, this being an indicator that GLS oligosaccharides, the by-products of GLS polysaccharide extraction, possess good potential for exploitation as a prebiotic.
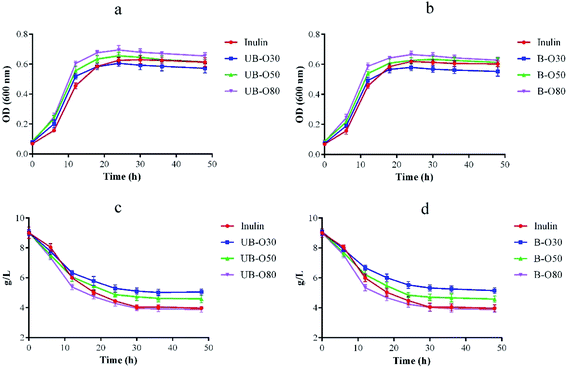 |
| Fig. 3 Growth of Lactobacillus (a and b) and changes in sugar concentration (c and d) over fermentation time as supplemented with different carbon sources. | |
3.6. Resistant to artificial human gastric juice hydrolysis
The non-digestibility characteristic of prebiotics ensure that they can reach the colon and persist throughout the large intestine, so that they can effectively stimulate certain native bacterial species (including probiotics) in the gut. The resistance of UB-O80 to hydrolysis in artificial human gastric juice was evaluated. As shown in Fig. 4, UB-O80 showed higher resistance to artificial human gastric juice. The percentage of hydrolysis increased with the decreased pH of the gastric juice. The degree of hydrolysis for UB-O80 at pH 1.0, 2.0, 3.0, 4.0 and 5.0 was 1.65%, 1.14%, 0.56%, 0.26% and 0.06%, respectively. It showed high acid resistance abilities compared to the reference prebiotic (inulin) at the same conditions, which gave maximum hydrolysis of 31.78%, 23.52%, 13.43%, 5.92% and 1.45% at pH 1, 2, 3, 4 and 5. Normally, food remains in the human stomach for about 3 h. Thus, when GLS oligosaccharides are ingested, more than 98% of them would reach the intestine. From the data point of view, UB-O80 was not sensitive to gastric juice, regardless of the incubation time. The data of UB-O80 were comparable to that for other oligosaccharides such as those from pitaya flesh, which were 96% resistant to artificial human gastric juice.5 Kojio-oligosaccharide displayed 100% resistance.40
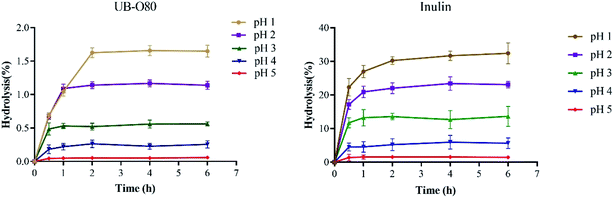 |
| Fig. 4 Resistance of UB-O80 and inulin to various artificial gastric juices: incubation for 6 h at 37 °C. | |
3.7. The effects of GLS oligosaccharides on probiotic growth at lower pH and in simulated gastric juice
The pH of the stomach can be as low as pH 1.5, but generally ranges from pH 2.5–3.5.41 While GLS oligosaccharides have beneficial effects on the proliferation rate of Lactobacillus, the growth of these bacteria can be affected by gastrointestinal conditions. This study aims to assess the transit tolerance of Lactobacillus in human gastric juice in vitro, and evaluate the effects of GLS oligosaccharides on the viability of Lactobacillus at lower pH and in simulated gastric juice.
The effect of GLS oligosaccharides (UB-O80, B-O80) on the tolerance of Lactobacillus to lower pH is revealed in Table 3. Lactobacillus showed a better growth rate following 2 h of fermentation at lower pH when using UB-O80 and B-O80 as their carbon source than with inulin, which indicates a slower growth rate with the latter. When simulated media were at pH 3.0, the viability of Lactobacillus remained at the highest level; the viability of Lactobacillus varied with pH (3.0 > 2.5 > 2.0 > 1.5), indicating that lower pH has a seriously inhibitory effect on the growth of Lactobacillus, such that UB-O80 and B-O80 offers a certain degree of protection against Lactobacillus' lower the pH intolerance.
Table 3 Growth rate of Lactobacillus over fermentation time as supplemented with different carbon sources at lower pH valuesa
Carbon sources |
Growth ratea |
Carbon sources |
Growth rate |
0–2 h |
2–4 h |
0–2 h |
2–4 h |
. |
pH 1.5 |
|
|
pH 2.0 |
|
|
Inulin |
0.018 |
0.002 |
Inulin |
0.028 |
0.012 |
UB-O80 |
0.034 |
0.014 |
UB-O80 |
0.051 |
0.035 |
B-O80 |
0.031 |
0.021 |
B-O80 |
0.068 |
0.029 |
pH 2.5 |
|
|
pH 3.0 |
|
|
Inulin |
0.086 |
0.043 |
Inulin |
0.131 |
0.073 |
UB-O80 |
0.114 |
0.712 |
UB-O80 |
0.168 |
0.117 |
B-O80 |
0.121 |
0.695 |
B-O80 |
0.174 |
0.123 |
The effect of GLS oligosaccharides (UB-O80, B-O80) on Lactobacillus' tolerance to simulated gastric juice (medium with pepsin) was shown in Fig. 5. It was found that the growth of Lactobacillus in simulated gastric juice containing the oligosaccharide of UB-O80 or B-O80 was higher than that inulin. However, the tolerance ability of Lactobacillus to the simulated gastric juice was not significantly different among UB-O80 and B-O80. When pH values were <3, inhibition of the action of protease caused by bacterial adhesion might contribute toward this phenomenon, comparable to behavior of resistant starch.27
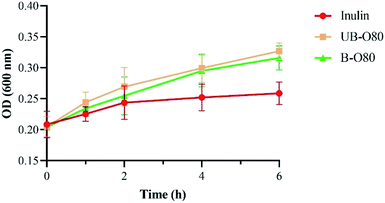 |
| Fig. 5 Tolerance of Lactobacillus in different carbon sources media containing simulated gastric juice. | |
Lactobacillus is an important probiotic and obligate anaerobe. The results of this study suggested that GLS oligosaccharides can significantly improve the tolerance ability of Lactobacillus to lower pH values and simulated gastric juice. GLS oligosaccharide can be considered an effective prebiotic in vivo.
3.8. Effects of GLS oligosaccharides on microbiome community composition
Among the gut microbiome community, 15 taxa with relative abundance were identified (Fig. 6). Families Enterobacteriaceae, Bacteroidaceae, Lachnospiraceae, Ruminococcaceae, Bifidobacteriaceae, Lactobacillaceae were dominating in the control group, and the abundance of these families was differentially modulated by oligosaccharides of UB-80 and B-80. After 24 h fermentation, the abundance of Ruminococcaceae, Bifidobacteriaceae and Lactobacillaceae increased in GLS oligosaccharides groups. Meanwhile, the abundance of Enterobacteriaceae and Lachnospiraceae reduced noticeable compared to the blank control. Study reported that Ruminococcaceae participated in the degradation of complex carbohydrates in the intestine,42 Bifidobacteriaceae and Lactobacillaceae possessed the abilities of reducing cholesterol, anti-tumor, delaying aging, antibacterial, anti-inflammatory and inhibiting pathogenic germ adhesion, laxative and other activities.43 While some of Lachnospiraceae could cause diabetes in sterile mice,44 and the abundance of Enterobacteriaceae increased in old age.45
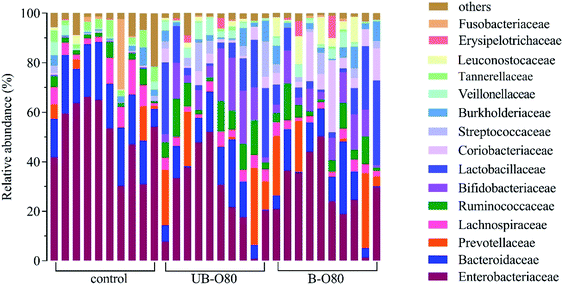 |
| Fig. 6 Gut microbiologic community bar chart on family level influenced by GLS oligosaccharides. | |
4. Conclusions
GLS oligosaccharides were obtained from the by-products of polysaccharide extraction. The degree of GLS oligosaccharides polymerization was in a range of 2–7. Both oligosaccharides of UB-GLS and B-GLS consisted of arabinose, mannose, glucose, and galactose, with their being little difference in their relative contents. The oligosaccharides showed some prebiotic properties, which included resistance to acid conditions in the simulated human stomach, partial artificial human gastric juice hydrolysis and the capacity to stimulate the growth of Lactobacillus. Compared to the blank control, the relative abundances of Bifidobacteriaceae and Lactobacillaceae were increased in the groups of GLS oligosaccharides addition in fermentation in vitro, while the abundances of Enterobacteriaceae and Lachnospiraceae was decreased. Thus, GLS oligosaccharides are potential sources of prebiotics such that they may be used as ingredients in functional foods and nutraceutical products to improve intestinal flora and immunity enhancement. It can also provide a feasible method for the high-value utilization of mushroom polysaccharide extraction by-products.
Conflicts of interest
The authors declare the absence of any competing financial interest.
Funding
This research was funded by the Key research and development projects of Zhejiang (No. 2018C02003 & No. 2019C02100).
References
- S. Li, M. Li, H. Yue, L. Zhou, L. Huang, Z. Du and K. Ding, Carbohyd. Polym., 2018, 186, 168–175 CrossRef CAS PubMed.
- M. Knip, Rev. Endocr. Metab. Dis., 2003, 4, 213–223 CrossRef CAS PubMed.
- P. Turn, M. Hamady, T. Yatsunenko, B. L. Cantarel, A. Duncan, R. E. Ley and M. Egholm, Nature, 2009, 7228, 457–480 Search PubMed.
- A. F. Azmi, S. Mustafa, D. M. Hashim and Y. A. Manap, Molecules, 2012, 17, 1635–1651 CrossRef CAS.
- F. M. N. A. Aida, M. Shuhaimi, M. Yazid and A. G. Maaruf, Trends Food Sci. Tech., 2009, 20, 567–575 CrossRef CAS.
- S. Wichienchot, M. Jatupornpipat and R. A. Rastall, Food Chem, 2010, 120, 850–857 CrossRef CAS.
- M. Y. Shaari and Y. Mohd, Effects of prebiotic activity of Ganoderma lucidum extracts on bifidobaciterium species and faecal microflora in vitro, University Putra Malaysia, Kuala Lumpur, 2012 Search PubMed.
- J. Luo, C. Zhang, R. Liu, L. Gao, S. Ou, L. Liu and X. Peng, J. Funct. Foods, 2018, 47, 127–135 CrossRef CAS.
- Y. Wang, K. Khoo, S. Chen, C. Lin, C. Wong and C. Lin, Bioorgan. Med. Chem., 2002, 10, 1057–1062 CrossRef CAS PubMed.
- S. Jong and J. M. Birmingham, Adv. Appl. Microbiol., 1992, 37, 101–134 CAS.
- S. Yamin, M. Shuhaimi, A. Arbakariya, A. B. Fatimah, A. K. Khalilah, O. Anas and A. M. Yazid, Int. Food Res. J., 2012, 19, 1199 CAS.
- I. Khan, G. Huang, X. Li, W. Leong, W. Xia and W. W. Hsiao, J. Funct. Foods, 2018, 41, 191–201 CrossRef CAS.
- L. C. Coleman, Bot. Gaz., 1927, 83, 48–60 CrossRef.
- X. Yang, H. Zhu, D. Zhao, J. Xu and Q. Kuang, Chin. Tradit. Herb. Drugs, 1997, 28, 721–723 CAS.
- A. Synytsya, K. Míčková, I. Jablonský, J. Spěváček, V. Erban and J. Čopíková, Carbohyd. Polym., 2009, 76, 548–556 CrossRef CAS.
- K. Manikandan, J. Food Protect., 1990, 53, 883–894 CrossRef PubMed.
- M. Dubois, K. A. Gilles, J. K. Hamilton, P. T. Rebers and F. Smith, Anal. Chem., 1956, 28, 350–356 CrossRef CAS.
- M. M. Bradford, Anal. Biochem., 1976, 72, 248–254 CrossRef CAS PubMed.
- X. Li, Q. Hu, S. Jiang, F. Li, J. Lin, L. Han, Y. Hong, W. Lu, Y. Chao and D. Chen, J. Saudi. Chem. Soc., 2015, 19, 454–460 CrossRef.
- E. Crowell and B. B. Burnett, Anal. Chem., 1967, 39, 121–124 CrossRef CAS.
- E. Ribechini, M. Zanaboni, A. M. R. Galletti, C. Antonetti, N. N. Nasso, E. Bonari and M. P. Colombini, J. Anal. Appl. Pyrol., 2012, 94, 223–229 CrossRef CAS.
- Q. Wu, H. Zhang, P. Wang and M. Chen, RSC Adv., 2017, 7, 45093–45100 RSC.
- S. R. Thambiraj, M. Phillips, S. R. Koyyalamudi and N. Reddy, Food Chem, 2018, 267, 319–328 CrossRef CAS PubMed.
- Y. Wang, Food Res. Int., 2009, 42, 8–12 CrossRef CAS.
- J. A. Robertson, P. Ryden, R. L. Botham, S. Reading, G. Gibson and S. G. Ring, LWT--Food Sci. Technol., 2001, 34, 567–573 CrossRef CAS.
- Y. Huang and M. C. Adams, Int. J. Food Microbiol., 2004, 91, 253–260 CrossRef PubMed.
- Y. Zhang, Y. Wang, B. D. Zheng, X. Lu and W. Zhuang, Food Funct, 2013, 4, 1609–1616 RSC.
- Q. Wu, W. Liu, H. Chen, Y. Yin, D. Y. Hongwei, X. Wang and L. Zhu, Anaerobe, 2017, 48, 206–214 CrossRef CAS PubMed.
- N. Benkeblia, S. Onodera and N. Shiomi, Food Chem, 2004, 87, 377–382 CrossRef CAS.
- X. Huang, H. Wu, F. Huang and X. Lin, Chin. Tradit. Herb. Drugs, 2006, 6, 813–816 Search PubMed.
- L. Yang and L. Zhang, Carbohyd. Polym., 2009, 76, 349–361 CrossRef CAS.
- A. Zhang, Y. Liu, N. Xiao, Y. Zhang and P. Sun, Food Chem, 2014, 146, 334–338 CrossRef CAS PubMed.
- J. Singthong, S. Ningsanond and S. Cui, Food Chem, 2009, 114, 1301–1307 CrossRef CAS.
- X. Xu, P. Chen, Y. Wang and L. Zhang, Carbohyd. Res., 2009, 344, 113–119 CrossRef CAS PubMed.
- A. Barth, Biochim. Biophys. Acta, Bioenerg., 2007, 1767, 1073–1101 CrossRef CAS PubMed.
- J. M. Veigas, M. S. Narayan and P. M. C Laxman, Food Chem, 2007, 105, 619–627 CrossRef CAS.
- H. M. Kakelar, A. Barzegari, S. Hanifian, J. Barar and Y. Omidi, Food Chem, 2019, 272, 709–714 CrossRef PubMed.
- M. Cai, S. Chen, Q. Ma, K. Yang and P. Sun, Food hydrocolloids, 2019, 95, 426–431 CrossRef CAS.
- R. Jovanovic, S. Kuzmanova and E. Winkelhausen, Int. J. Food Prop., 2014, 17, 949–965 CrossRef.
- W. H. Holzapfel, P. Haberer, J. Snel, U. Schillinger and J. H. H. Veld, Int. J. Food Microbiol., 1998, 41, 85–101 CrossRef CAS PubMed.
- W. E. V. Lankaputhra, Cult. Dairy Prod. J., 1995, 30, 2–7 CAS.
- M. Rajilić-Stojanović, Best Pract. Res., Clin. Gastroenterol., 2013, 27(1), 5–16 CrossRef PubMed.
- J. Yang, I. Martínez, J. Walter, A. Keshavarzian and D. J. Rose, Anaerobe, 2013, 23, 74–81 CrossRef CAS PubMed.
- K. Kameyama and K. Itoh, Microbes Environ, 2014, ME14054 Search PubMed.
- E. J. Woodmansey, J. Appl. Microbiol., 2007, 102, 1178–1186 CrossRef CAS PubMed.
|
This journal is © The Royal Society of Chemistry 2020 |
Click here to see how this site uses Cookies. View our privacy policy here.