DOI:
10.1039/C9RA10555G
(Paper)
RSC Adv., 2020,
10, 12599-12603
Visible light-mediated photocatalytic oxidative cleavage of activated alkynes via hydroamination: a direct approach to oxamates†
Received
15th December 2019
, Accepted 26th February 2020
First published on 27th March 2020
Abstract
The direct oxidative cleavage of activated alkynes via hydroamination has been described using organic photocatalyst under visible-light irradiation at room temperature. In this reaction, the single electron oxidation of an in situ formed enamine followed by radical coupling with an oxidant finally delivers the oxamate. The key features of this photocatalytic reaction are the mild reaction conditions, metal-free organic dye as a photocatalyst, and TBHP playing a dual role as “O” source and for the regeneration of the photocatalyst.
Introduction
C–C bond cleavage reactions have been paid considerable attention to, owing to the potential utility of resulting functionalized intermediates in organic transformations and medicinal chemistry.1 Other than organic synthesis, one also finds C–C bond cleavage applications in photocatalytic degradation leading to environment remediation and further in generation of fine chemicals from biomass based organic materials. In the past decades, various strategies for C–C single, double and triple bond breakage have been developed.2 Among C–C bond cleavage reactions, although the cleavage of the C–C triple bond has been extensively studied, still it remains a challenging issue in contemporary organic synthesis due to its large bond dissociation energy.3 In recent years, several proficient transition-metal-catalysed reactions have been demonstrated for C–C triple bond cleavages.4 To circumvent the use of precious transition-metal catalysts, recently metal-free approaches have become more attractive and provide potential building blocks such as amides, nitriles, acids, and esters from C–C triple bond cleavage.5 Despite the progress made, metal-free methods with economic and readily available reagents are still highly desirable and remain a challenge. In this context, recently, visible-light induced photocatalysis has emanated as an enabling platform to access new chemical transformations under mild conditions.6 However, the complete C–C triple bond cleavages under photocatalysis are scarce. Recently, Lee research group has developed the C–C triple bond cleavage strategy to synthesize azulen-1-yl ketones via N-sulfonyl enamides under photocatalysis (Scheme 1a).7 Enamines have gained significant attention in oxidative C–C bond cleavages.8 In this regard, Wang and co-workers have presented the oxidative cleavage of electron rich enamines to amides under Ru catalyzed visible-light irradiation (Scheme 1b).9 To the best of our knowledge, visible light induced organic photocatalyzed oxidative cleavage of C–C triple bond via hydroamination leading to oxamates has not been reported (Scheme 1c).
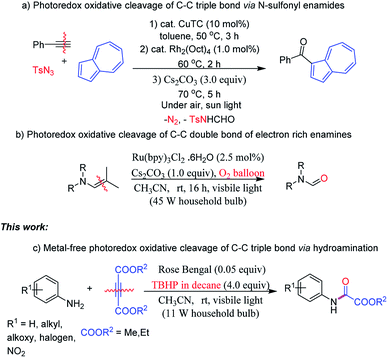 |
| Scheme 1 Selected examples for photocatalysis oxidative cleavage of C–C double and triple bonds. | |
In recent years, alkynes as appealing substrates have pinched great attention in the synthesis of heterocycles and building blocks as well.8c,d,10 With our continuing interests in developing sustainable methods leading to potential intermediates and heterocycles of bio relevance under metal-free catalysis8c,d,11 from the amines and electron deficient alkynes as key starting materials, herein we report visible-light induced Rose Bengal photocatalyzed C–C triple bond oxidative cleavage.
Organic molecules containing amide (peptide bond) α to ester functionality are important in the synthesis of bioactive molecules.12 In this context, oxamates are well known as versatile building blocks for the synthesis of functionalized organic molecules, biologically active molecules and natural products.13 Consequently, few methods have been developed for the construction of oxamates and their analogues using metal or metal-free catalysts.14 Traditional methods involved the coupling of amines with precursors such as monoesters of oxalyl chloride or diester of oxalic acid in generating oxamates.14 However, the monoesters of oxalyl chlorides are thermally unstable and require in stoichiometric amounts, and moreover, the preparation methods are laborious and involve the distillation process of the reaction mixture. Although alternative methods documented in the literature,14 the use of a dehydrating agent, the high pressure of CO/O2, expensive metal catalyst, limited substrate scope, and difficulty in separation of catalyst from product and its reuse are significant drawbacks. Very recently, oxamates could also be successfully synthesized via C–C and C
C bonds of acetoacetamides and enamines by employing metal catalyst and harsh reaction conditions respectively.15 However, the development of sustainable methods toward the synthesis of oxamates is highly desirable.
Results and discussion
In this sense, we investigated visible-light-mediated C–C triple bond cleavage by using aniline 1a and diethyl acetylenedicarboxylate 2a as model substrates in the presence of 4 equiv. of aqueous tertiary butyl hydrogen peroxide (aq. TBHP) as the oxidizing agent, 5 mol% Rose Bengal in DMF at room temperature under irradiation with 11 W CFL household bulb, pleasantly which afforded the desired product ethyl 2-oxo-2-(phenylamino)acetate 3aa with 22% yield after 48 h (Table 1, entry 1). Change of solvent from DMF to CH3CN improved the yield to 58% (Table 1, entry 2). Inspired by this result, we screened other oxidants (Table 1, entries 3–7) and TBHP in decane was found to be most effective (82%, Table 1, entry 5). To further improve the yield, we then investigated the reaction with various photocatalysts such as eosin Y, riboflavin, rhodamine B, Ru(bpy)3Cl2 and Ru(bpy)3Cl2·H2O. Although the two Ru based photocatalysts and Rose Bengal were found to be sufficient for C–C triple bond cleavage (Table 1, entries 11–13 and 5), The low cost, easy accessible and metal-free nature of Rose Bengal16 inspired us to further optimize the reaction conditions with this organophotocatalyst. Next, we have screened the reaction by varying the equiv. of Rose Bengal photocatalyst, and 0.05 equiv. was found to be optimum (Table 1, entries 14–16). We were pleased to find oxamate 3aa in 81% yield with 0.05 equiv. of Rose Bengal, 4 equiv. of TBHP (in decane) and 11 W CFL bulb as a light source in CH3CN solvent (Table 1, entry 14). The reaction did not proceed either in the absence of photocatalyst or visible light, indicating that both components are crucial for C–C triple bond cleavage (entries 17 and 18). Endeavors to use sunlight instead of 11 W CFL bulb gave a very poor yield of 3aa (entry 19). Other CFL bulbs such as 5 W and 15 W did not improve the yield (entries 20 and 21). The use of bases such as DIPEA and Cs2CO3 were not successful (entries 22 and 23). Among solvents examined (entries 24–27), CH3CN showed that it is the most suitable solvent for the present transformation (entry 14).
Table 1 Optimization of reaction conditions for the synthesis of 3aaa

|
Entry |
Photocatalyst (equiv.) |
Oxidant (equiv.) |
Solvent |
Yieldb (%) |
Reaction conditions: 1a (0.53 mmol), 2a (0.53 mmol), solvent (1.5 mL), rt (27 °C), 11 W CFL household bulb, for 48 h. Isolated yields after silica-gel column chromatography. Reaction mixture was degassed before providing O2 atmosphere (balloon). Reaction mixture was degassed before providing N2 atmosphere. Reaction is performed on presynthesized enamine. Reaction carried out in dark. Reaction performed under sunlight. 5 W household bulb used. 15 W household bulb used. DIPEA (di isopropyl ethylamine) (1.0 equiv.) used. Cs2CO3 (1.0 equiv.) used. TBHP (5.0–6.0 M) in decane. DTBP = di-tert-butyl peroxide, TBP = tert-butyl peroxybenzoate. |
1 |
Rose Bengal (0.05) |
aq. TBHP (4.0) |
DMF |
22 |
2 |
Rose Bengal (0.05) |
aq. TBHP (4.0) |
CH3CN |
58 |
3 |
Rose Bengal (0.05) |
O2 balloon |
CH3CN |
40c |
4 |
Rose Bengal (0.05) |
TBHP in decane (4.0) |
CH3CN |
55d |
5 |
Rose Bengal (0.05) |
TBHP in decane (4.0) |
CH3CN |
82 |
6 |
Rose Bengal (0.05) |
DTBP (4.0) |
CH3CN |
39 |
7 |
Rose Bengal (0.05) |
TBP (4.0) |
CH3CN |
16 |
8 |
Eosin Y (0.05) |
TBHP in decane (4.0) |
CH3CN |
68 |
9 |
Riboflavin (0.05) |
TBHP in decane (4.0) |
CH3CN |
62 |
10 |
Rhodamine B (0.05) |
TBHP in decane (4.0) |
CH3CN |
45 |
11 |
Ru(bpy)3Cl2 (0.05) |
TBHP in decane (4.0) |
CH3CN |
83 |
12 |
Ru(bpy)3Cl2·6H2O (0.05) |
TBHP in decane (4.0) |
CH3CN |
80 |
13 |
Ru(bpy)3Cl2·6H2O (0.05) |
O2 balloon |
CH3CN |
43 |
14 |
Rose Bengal (0.05) |
TBHP in decane (4.0) |
CH3CN |
81, 80e |
15 |
Rose Bengal (0.025) |
TBHP in decane (4.0) |
CH3CN |
75 |
16 |
Rose Bengal (0.15) |
TBHP in decane (4.0) |
CH3CN |
82 |
17 |
— |
TBHP in decane (4.0) |
CH3CN |
Nd |
18 |
Rose Bengal (0.05) |
TBHP in decane (4.0) |
CH3CN |
Tracef |
19 |
Rose Bengal (0.05) |
TBHP in decane (4.0) |
CH3CN |
21g |
20 |
Rose Bengal (0.05) |
TBHP in decane (4.0) |
CH3CN |
71h |
21 |
Rose Bengal (0.05) |
TBHP in decane (4.0) |
CH3CN |
78i |
22 |
Rose Bengal (0.05) |
TBHP in decane (4.0) |
CH3CN |
82j |
23 |
Rose Bengal (0.05) |
TBHP in decane (4.0) |
CH3CN |
82k |
24 |
Rose Bengal (0.05) |
TBHP in decane (4.0) |
DCE |
71 |
25 |
Rose Bengal (0.05) |
TBHP in decane (4.0) |
DMSO |
14 |
26 |
Rose Bengal (0.05) |
TBHP in decane (4.0) |
DCM |
56 |
27 |
Rose Bengal (0.05) |
TBHP in decane (4.0) |
DMA |
43 |
After optimization of the reaction conditions, we next tested the broad range of anilines 1. Anilines 1 containing electron-neutral and electron-rich functional groups were well participated in oxidative C–C triple bond cleavage to afford the corresponding oxamates (3aa, 3ab, 3ba, 3bb & 3da, Table 2) with very good yields. Weak electron withdrawing groups like halogens substituted anilines reacted well, providing the corresponding products (3ea, 3eb, 3fa, 3fb, 3ga, 3ha, 3ia, 3ja & 3ka) with good yields. Slightly lower yields were observed for electron-withdrawing nitro-substituted anilines, this may be due to less formation of enamine or hydroamination product (3la & 3lb). We sought to further explore this oxidative C–C triple bond cleavage reaction with aliphatic amines. The low yields of (3ma & 3nb) in case of aliphatic amines, could be due to the less stabilization of radical cation (II) and imminium ion (III) when compared to other aromatic amines. Furthermore, other diverse in situ generated enamines failed to participate in oxidative C–C cleavage, due to failure in the formation of radical cation intermediate (II) and imminium ion (III) in case of (3ac & 3ad) wherein we have –CH3 and –Ph in the ‘α’ position of enamine, which might not facilitate oxidation and t-BuO–O˙ radical addition, thus indicating CO2Et group is crucial in the ‘α’ position of enamine. Alternatively, more EWG like CF3 might also not favor the oxidation of enamine to intermediate (II) in the presence of Rose Bengal catalyst.
Table 2 Substrates scope with respect to aniline and alkynesa,b

|
Reaction conditions: 1 (1.0 mmol), 2 (1.0 mmol), Rose Bengal (5 mol%), TBHP in decane (4.0 equiv.), 11 W CFL bulb, CH3CN (1.5 mL), rt, for 48 h. Isolated yields after silica-gel column chromatography. |
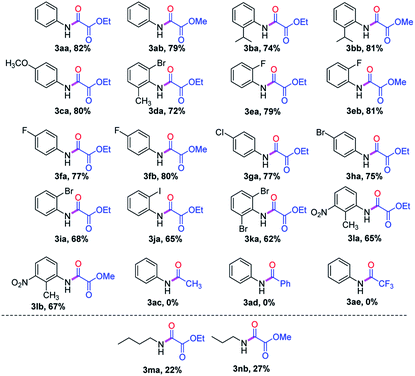 |
Based on our observations and literature reports7,9 a plausible mechanism for the visible light Rose Bengal photocatalyzed C–C triple bond oxidative cleavage is illustrated in Scheme 2. The visible-light-excited Rose Bengal (RB*) would undergo oxidative quenching by hydroamination product I to afford Rose Bengal radical anion (RB˙−) and radical cation II.
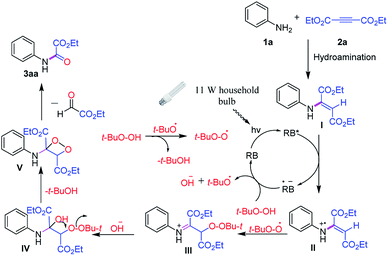 |
| Scheme 2 Plausible reaction mechanism. | |
Rose Bengal radical anion upon transfer of an electron to t-BuO–OH would provide the tertiary butoxide radical, hydroxide anion and complete the photocatalysis cycle by the generation of Rose Bengal. On the other hand, radical cation II would undergo addition reaction simultaneously by tertiary butyl peroxide radical and hydroxide anion to form intermediate IV. Upon intramolecular cyclization by giving up a t-BuOH, 1,2 dioxetane V would be formed which further undergoes fragmentation to give the desired product 3aa.
Conclusion
We have developed a visible light driven Rose Bengal photocatalysis oxidative cleavage of C–C triple bond through hydroamination for the direct synthesis of oxamates from amines and electron deficient alkynes as a key starting material. The key features of this transformation are the mild conditions, metal-free organic dye as a photocatalyst, TBHP playing a dual role as “O” source and for the regeneration of photocatalyst, and a tandem hydroamination-oxidative C–C triple bond cleavage, thus enabling the preparation of oxamate derivatives. Notably, the present method offers a wide range of oxamate derivatives as of potential building blocks which are difficult to synthesize using previously developed methods.
Conflicts of interest
There are no conflicts to declare.
Acknowledgements
We gratefully acknowledge the Council of Scientific and Industrial Research (02(0297)/17/EMR-II) and the Department of science and Technology-science and Engineering Research Board (DST-SERB- EMR/ 2016/ 000952) New Delhi, India, and Indian Institute of Technology Hyderabad (IITH) for financial support. NRK and MO thanks DST-SERB, AVM thanks UGC, SA thanks CSIR, New Delhi, India for the award of research fellowship.
Notes and references
-
(a) L. Gu and H. Zhang, RSC Adv., 2015, 5, 690 RSC;
(b) C. Qin, P. Feng, Y. Ou, T. Shen, T. Wang and N. Jiao, Angew. Chem., Int. Ed., 2013, 52, 7850 CrossRef CAS PubMed;
(c) N. Okamoto, T. Sueda, H. Minami, Y. Miwa and R. Yanada, Org. Lett., 2015, 17, 1336 CrossRef CAS PubMed;
(d) C. Qin, Y. Su, T. Shen, X. Shi and N. Jiao, Angew. Chem., Int. Ed., 2016, 55, 350 CrossRef CAS PubMed.
- For C–C bond cleavages:
(a) Y. J. Park, J.-W. Park and C.-H. Jun, Acc. Chem. Res., 2008, 41, 222 CrossRef CAS PubMed;
(b) A. Masarwa and I. Marek, Chem.–Eur. J., 2010, 16, 9712 CrossRef CAS PubMed;
(c) M. Murakami and T. Matsuda, Chem. Commun., 2011, 47, 1100 RSC;
(d) L. Souillart and N. Cramer, Chem. Rev., 2015, 115, 9410 CrossRef CAS PubMed;
(e) C. Tang and N. Jiao, Angew. Chem., Int. Ed., 2014, 53, 6528 CrossRef CAS PubMed;
(f) Q. Gao, S. Liu, X. Wu, J. Zhang and A. Wu, Org. Lett., 2015, 17, 2960 CrossRef CAS PubMed;
(g) W. Zhou, W. Fan, Q. Jiang, Y.-F. Liang and N. Jiao, Org. Lett., 2015, 17, 2542 CrossRef CAS PubMed;
(h) C. Zhang, P. Feng and N. Jiao, J. Am. Chem. Soc., 2013, 135, 15257 CrossRef CAS PubMed;
(i) F. Chen, T. Wang and N. Jiao, Chem. Rev., 2014, 114, 8613 CrossRef CAS PubMed.
- M. Tobisu and N. Chatani, Chem. Soc. Rev., 2008, 37, 300 RSC.
- For C–C triple bond cleavages:
(a) T. Shen, Y. Zhang, Y.-F. Liang and N. Jiao, J. Am. Chem. Soc., 2016, 138, 13147 CrossRef CAS PubMed;
(b) U. Dutta, D. W. Lupton and D. Maiti, Org. Lett., 2016, 18, 860 CrossRef CAS PubMed;
(c) R. Chinchilla and C. Najera, Chem. Rev., 2014, 114, 1783 CrossRef CAS PubMed;
(d) Z. Long, Y. Yang and J. You, Org. Lett., 2017, 19, 2781 CrossRef CAS PubMed;
(e) W. Wu and H. Jiang, Acc. Chem. Res., 2012, 45, 1736 CrossRef CAS PubMed;
(f) W.-B. Sheng, Q. Jiang, W.-P. Luo and C.-C. Guo, J. Org. Chem., 2013, 78, 5691 CrossRef CAS PubMed;
(g) N. Okamoto, M. Ishikura and R. Yanada, Org. Lett., 2013, 15, 2571 CrossRef CAS PubMed;
(h) Q. Jiang, A. Zhao, B. Xu, J. Jia, X. Liu and C. Guo, J. Org. Chem., 2014, 79, 2709 CrossRef CAS PubMed;
(i) H. Li, W.-J. Hao, M. Wang, X. Qin, S.-J. Tu, P. Zhou, G. Li, J. Wang and B. Jiang, Org. Lett., 2018, 20, 4362 CrossRef CAS PubMed;
(j) J. Ni, Y. Jiang, Z. An and R. Yan, Org. Lett., 2018, 20, 1534 CrossRef CAS PubMed.
- For the metal-free cleavage of the C–C triple bond, see:
(a) R. M. Moriarty, R. Penmasta, A. K. Awasthi and I. Prakash, J. Org. Chem., 1988, 53, 6124 CrossRef CAS;
(b) A. Banerjee, B. Hazra, A. Bhattacharya, S. Banerjee, G. C. Banerjee and S. Sengupta, Synthesis, 1989, 1989, 765 CrossRef;
(c) K. Miyamoto, Y. Sei, K. Yamaguchi and M. Ochiai, J. Am. Chem. Soc., 2009, 131, 1382 CrossRef CAS PubMed;
(d) K. A. Aravinda Kumar, V. Venkateswarlu, R. A. Vishwakarma and S. D. Sawant, Synthesis, 2015, 47, 3161 CrossRef CAS;
(e) T. Yamaguchi, T. Nobuta, Y. Kudo, S. Hirashima, N. Tada, T. Miura and A. Itoh, Synlett, 2013, 24, 607 CrossRef CAS;
(f) P. Sathyanarayana, A. Upare, O. Ravi, P. R. Muktapuram and S. R. Bathula, RSC Adv., 2016, 6, 22749 RSC;
(g) N. Okamoto, M. Ishikura and R. Yanada, Org. Lett., 2013, 15, 2571 CrossRef CAS PubMed;
(h) U. Dutta, D. W. Lupton and D. Maiti, Org. Lett., 2016, 18, 860 CrossRef CAS PubMed;
(i) Y. G. Lin and Q. L. Song, Eur. J. Org. Chem., 2016, 3056 CrossRef CAS;
(j) G. Majji, S. Guin, S. K. Rout, A. Behera and B. K. Patel, Chem. Commun., 2014, 50, 12193 RSC;
(k) Q. Jiang, A. Zhao, B. Xu, J. Jia, X. Liu and C. C. Guo, J. Org. Chem., 2014, 79, 2709 CrossRef CAS PubMed;
(l) S. Khamarui, R. Maiti and D. K. Maiti, Chem. Commun., 2015, 51, 384 RSC.
- For selected recent examples using organic photoredox catalysts, see:
(a) W.-L. Guo, L.-Q. Wang, Y.-N. Wang, J.-R. Chen and W.-J. Xiao, Angew. Chem. Int. Ed., 2015, 54, 2265 (Angew. Chem., 2015, 127, 2293) CrossRef CAS PubMed;
(b) T. Xiao, L. Li, G. Lin, Q. Wang, P. Zhang, Z. Mao and L. Zhou, Green Chem., 2014, 16, 2418 RSC;
(c) D. J. Wilger, N. J. Gesmundo and D. A. Nicewicz, Chem. Sci., 2013, 4, 3160 RSC;
(d) D. P. Hari, P. Schroll and B. Koenig, J. Am. Chem. Soc., 2012, 134, 2958 CrossRef CAS PubMed;
(e) M. Neumann, S. Fuldner, B. Konig and K. Zeitler, Angew. Chem. Int. Ed., 2011, 50, 951 (Angew. Chem., 2011, 123, 981) CrossRef CAS PubMed;
(f) Y. Pan, S. Wang, C. W. Kee, E. Dubuisson, Y. Yang, K. P. Loh and C.-H. Tan, Green Chem., 2011, 13, 3341 RSC;
(g) Y. Pan, C. W. Kee, L. Chen and C.-H. Tan, Green Chem., 2011, 13, 2682 RSC;
(h) X. Lang, X. Chen and J. Zhao, Chem. Soc. Rev., 2014, 43, 473–486 RSC;
(i) W. Ma, C. Chen, H. Ji and J. Zhao, Acc. Chem. Res., 2014, 47(2), 355–363 CrossRef PubMed;
(j) X. Lang, J. Zhao and X. Chen, Chem. Soc. Rev., 2016, 45, 3026–3038 RSC.
- S. Park, W. H. Jeon, W.-S. Yong and P. H. Lee, Org. Lett., 2015, 17, 5060 CrossRef CAS PubMed.
-
(a) H. Sun, C. Yang, F. Gao, Z. Li and W. Xia, Org. Lett., 2013, 15, 624 CrossRef CAS PubMed;
(b) T. Shen, Y. Zhang, Y.-F. Liang and N. Jiao, J. Am. Chem. Soc., 2016, 138, 13147 CrossRef CAS PubMed;
(c) A. Sagar, S. Vidyacharan, A. H. Shinde and D. S. Sharada, Org. Biomol. Chem., 2016, 14, 4018–4022 RSC;
(d) A. Sagar, V. N. Babu, A. Dey and D. S. Sharada, Tetrahedron Lett., 2015, 56, 2710 CrossRef CAS.
- J. Li, S. Cai, J. Chen, Y. Zhao and D. Z. Wang, Synlett, 2014, 25, 1626 CrossRef.
-
(a) J. Sun, G. Zheng, T. Xiong, Q. Zhang, J. Zhao, Y. Li and Q. Zhang, ACS Catal., 2016, 6, 3674 CrossRef CAS;
(b) G. Zheng, Y. Li, J. Han, T. Xiong and Q. Zhang, Nat. Commun., 2015, 6, 7011 CrossRef CAS PubMed.
-
(a) S. Arepally, V. N. Babu and M. Bakhadoss, Org. Lett., 2017, 19, 5014–5017 CrossRef CAS PubMed;
(b) S. Arepally, V. N. Babu, A. Polu and D. S. Sharada, Eur. J. Org. Chem., 2018, 5700 CrossRef CAS;
(c) S. Vidyacharan, A. Sagar and D. S. Sharada, Org. Biomol. Chem., 2015, 13, 7614 RSC;
(d) S. Arepally, A. Chamuah, N. Katta and D. S. Sharada, Eur. J. Org. Chem., 2019, 7, 1542 CrossRef;
(e) V. N. Babu, A. Murugan, N. Katta, D. Suman and D. S. Sharada, J. Org. Chem., 2019, 84, 6631 CrossRef CAS PubMed.
-
(a) C. J. Dinsmore and D. C. Beshore, Tetrahedron, 2002, 58, 3297 CrossRef CAS;
(b) L. C. Dias and M. A. B. Ferreira, J. Org. Chem., 2012, 77, 4046–4062 CrossRef CAS PubMed;
(c) J. H. Sellstedt, C. J. Guinosso, A. J. Begany, S. C. Bell and M. Rosenthale, J. Med. Chem., 1975, 18, 926 CrossRef CAS PubMed;
(d) J. B. Wright, C. M. Hall and H. G. N. Johnson, J. Med. Chem., 1978, 21, 930 CrossRef CAS PubMed;
(e) D. H. Klaubert, J. H. Sellstedt, C. J. Guinosso, R. J. Capetola and S. C. Bell, J. Med. Chem., 1981, 24, 742 CrossRef CAS PubMed;
(f) K. D. Hargrave, F. K. Hess and J. T. Oliver, J. Med. Chem., 1983, 26, 1158 CrossRef CAS PubMed;
(g) S. Choi, A. Pradhan, N. L. Hammond, A. G. Chittiboyina, B. L. Tekwani and M. A. Avery, J. Med. Chem., 2007, 50, 3841 CrossRef CAS PubMed;
(h) N. N. Polushin, Tetrahedron Lett., 1996, 37, 3231 CrossRef CAS.
-
(a) Z. Liu, Q. Lei, Y. Li, L. Xiong, H. Song and Q. Wang, J. Agric. Food Chem., 2011, 59, 12543 CrossRef CAS PubMed;
(b) C. Palmer, N. A. Morra, A. C. Stevens, B. Bajtos, B. P. Machin and B. L. Pagenkopf, Org. Lett., 2009, 11, 5614 CrossRef CAS PubMed;
(c) Y. Xu, M. McLaughlin, E. N. Bolton and R. A. Reamer, J. Org. Chem., 2010, 75, 8666 CrossRef CAS PubMed;
(d) J. W. Lynn and J. English Jr, J. Am. Chem. Soc., 1951, 73, 4284 CrossRef CAS.
-
(a) S. Lchimurahashi, Y. Mitsue and K. Ike, J. Chem. Soc., Chem. Commun., 1987, 125–127 RSC;
(b) S. T. Gadge and B. M. Bhanage, J. Org. Chem., 2013, 78, 6793 CrossRef CAS PubMed;
(c) S. T. Gadge, E. N. Kusumawati, K. Harada, T. Sasaki, D. N. Hamane and B. M. Bhanage, J. Mol. Catal., 2015, 400, 170 CrossRef CAS.
-
(a) Z. Zhang, X. Gao, H. Yu, G. Zhang and J. Liu, Adv. Synth. Catal., 2018, 360, 3406 CrossRef CAS;
(b) M. Adib, R. Pashazadeh, S. J. A. Gohari and F. Shahsavari, Synlett, 2017, 28, 1481 CrossRef CAS.
- S. Sharma and A. Sharma, Org. Biomol. Chem., 2019, 17, 4384 RSC.
Footnote |
† Electronic supplementary information (ESI) available. See DOI: 10.1039/c9ra10555g |
|
This journal is © The Royal Society of Chemistry 2020 |
Click here to see how this site uses Cookies. View our privacy policy here.