DOI:
10.1039/C9RA10465H
(Paper)
RSC Adv., 2020,
10, 7321-7327
Amino acid sequences characterization and anti-inflammatory potency evaluation of Portulaca oleracea L. oligopeptides in macrophages
Received
12th December 2019
, Accepted 31st January 2020
First published on 19th February 2020
Abstract
The Portulaca oleracea L. oligopeptides are seldom explored because they are often present in a complex matrix. In the current study, eleven novel Portulaca oleracea L. oligopeptides (POPs) were isolated and their mino acid sequence identified. Further, the anti-inflammatory potency was explored in lipopolysaccharide (LPS)-induced RAW264.7 cells. Results showed that POP-1∼[EHGEYE] possessed excellent anti-inflammatory potency by attenuating the pro-inflammatory cytokine expression (TNF-α, NO, IL-1β); inhibiting iNOS and COX-2 expressions and regulating the MAPK, PI3K/Akt and NF-κB signaling pathways. This may be an important molecular mechanism of POPs in anti-inflammatory damage.
1 Introduction
Inflammation is a defense reaction to stimulation of various damages, which plays significant roles in disease development, such as asthma and atherosclerosis.1 It is now widely accepted that atherosclerosis is one of the leading causes of death in vascular diseases, and atherosclerosis has been recognized as a complex chronic inflammatory disease, in which continuous dyslipidemia and inflammation play a vital role.2 Macrophages produce many kinds of inflammatory mediators such as interleukin (IL-1β), tumor necrosis factor (TNF-α), nitric oxide (NO), and prostaglandins during the progress of inflammation. Further, the macrophages protect the body from external intruders through phagocytosis.3
Nuclear factor-kappa B (NF-κB) is primarily involved in inflammatory response. Extracellular stimulus, such as lipopolysaccharide (LPS), would induce the phosphorylation of the inhibitory sub-unit and dissociation from NF-κB. The active complex of NF-κB will bind to cognate DNA binding sites, and stimulates various inflammation-related genes.4 The chief upstream kinases that are implicated in the activation of NF-κB, include mitogen activated protein kinase (MAPK) and Akt. Both MAPKs and NF-κB play a crucial role in the regulation of pro-inflammatory cytokines.5 PI3K, a classical upstream kinase in mTOR pathway, has been implicated in various immune responses and inflammatory processes. Signaling pathways mediated by PI3K/Akt, MAPK and transcription factors such as activator NF-κB are the predominant cascades.6
Portulaca oleracea L. is a folk medicine, and is widely used to treat inflammation, cancer and aging diseases. Studies reported that Portulaca oleracea L. extracts can reduce the inflammation damage in macrophages under LPS stimuli. Poly-phenol derivatives are the major bio-active components in the Portulaca oleracea L., and mounting evidence has revealed that the poly-phenol derivatives can attenuate LPS-induced diseases, including acute lung injury, mastitis and endotoxemia in mice.7,8 However, the anti-inflammatory potency of the Portulaca oleracea L. oligopeptides (POPs) remain poorly explored. RAW264.7 macrophage cells, derived from Balb/c mice ascites, are commonly accepted as a tool to investigate the molecular mechanisms of macrophage involved in regulating immunity.9 Therefore, we isolated the POPs and explored the anti-inflammatory potency in RAW264.7 cells.
2 Material and methods
2.1 Chemicals and ingredients
Cell culture medium (DMEM), phosphate buffer solution (PBS) and fetal bovine serum (FBS) were purchased from GIBCO (Life Technologies, Grand Island, NY). Fresh plant of Portulaca oleracea L. was achieved from the South China Agricultural University (Guangzhou, China) and identified by Professor Wang (South China Agricultural University, Guangzhou, China). All the cell lines were obtained from the Cell Bank of the Chinese Academy of Sciences (Shanghai, China). The JC-1, CCK-8 kit (cell counting kit-8), TUNEL Apoptosis Detection Kit, Nuclear and Cytoplasmic Extraction Kit were purchased from Beyotime (Shanghai, China). All the kits were validated and used according to manufacturer's instructions. All the antibodies were purchased from Cell Signaling Technology (CST, Beverly, MA, USA). All the other chemicals were purchased from Sigma or Adamas (Sigma-Aldrich, St. Luis, MO) without any further purification.
2.2 POPs isolation and structural identification
The fresh Portulaca oleracea L. was extracted in 50% ethanol and ultrasound for 2 h, the extract was passed through filter paper. The supernatant was concentrated under reduced pressure with a vacuum rotary evaporator at 4 °C (EYELA, N-1000, Japan).
The extraction scheme of POPs is summarized in Fig. 1. Briefly, fresh Portulaca oleracea L. was defatted with iso-propanol and then centrifugated at 6000g for 15 min. The proteins with molecule weight over 1.5 kDa was removed by ultrafiltration with a 1.5 kDa cut off membrane. Oligopeptides with molecule weight less than 1.5 kDa were further analysed by hydrophobic chromatography, anion exchange chromatography, gel filtration chromatography, and RP-HPLC. Finally, eleven novel POPs were obtained and the purity is detected by HPLC (>97%). HPLC-ESI-MS was performed on a SCIEX X500R Q-TOF mass spectrometer (Framingham, U.S.A.) to analyze the amino acid sequences of POPs.
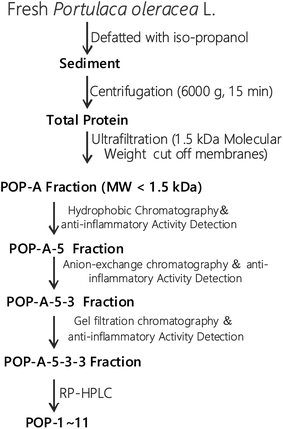 |
| Fig. 1 Extraction and separation processes of POPs. | |
2.3 Cell culture and cell viability
RAW264.7 cells were maintained in DMEM containing 10% (v/v) FBS, L-glutamine (2 mM), penicillin (100 U mL−1) and streptomycin (100 U mL−1) at 37 °C in a humidified atmosphere containing 5% CO2, the culture medium was changed every other day. To avoid the mortality induced by the POPs, the effects of POPs on of cell viabilities were determined using a cell counting kit-8 (CCK-8) method. The protective potencies of POPs were detected as follows: briefly, RAW264.7 cells were seeded in 96-well plates (4 × 105 cells per mL) and treated with POPs (10 μM) for 24 h, then treated with LPS (1 μg mL−1) for 12 h. After that, the cell viability was detected with CCK8 kit.
2.4 NO, PGE2, TNF-α, IL-6 and IL-1β production assays
RAW264.7 cells were seeded in 96-well plates (4 × 105 cells per mL) and treated with POP-1 10 and 50 μM for 24 h, respectively, then treated with LPS (1 μg mL−1) for 12 h. After that, the NO, PGE2, TNF-α, IL-6 and IL-1β levels were quantified using ELISA kits (Beyotime, Shanghai, China) according to the manufacturer's instructions.
2.5 Apoptosis and cell cycle arresting assays
The RAW264.7 cells were treated as the experimental design. After that, the cells were collected and fixed in 70% ethanol at −20 °C overnight. The fixed cells were washed twice with cold PBS and re-suspended in 1.0 mL of staining reagent PI for 0.5 h in dark at room temperature. As for the apoptosis detection, the cells were treated as the experimental design. After that, the apoptosis was determined with Annexin V-fluorescein isothiocyanate (annexin V-FITC) and Propidium Iodide (PI) labeling according to the manufacturer's instructions.
Both the cell cycle arresting and apoptosis were analyzed by flow cytometry (BD FACSCalibur, USA).
2.6 Western blotting
RAW264.7 cells were treated with the experimental design, then collected, washed, and lysed in the lysis buffer containing protease and phosphatase inhibitor cocktails and centrifuged at 1.3 × 104g for 15 min. Then, western blotting was performed. Total cell lysates were prepared by lysing the washed cell pellet directly in radioimmunoprecipitation (RIPA) buffer. The lysates were clarified by centrifugation at 13
000 × g for 15 min at 4 °C. The lysates were boiled and separated by 10% sodium dodecylsulfate (SDS)-polyacrylamide gels (PAGE), blotted onto a polyvinylidene fluoride (PVDF) membrane (Millipore, Bedford, MA, USA), and analyzed by western blotting with the antibodies. β-Actin and GAPDH were used for detect amount of proteins of each lane.
2.7 Statistical analysis
The data achieved from at least three independent experiments were listed as means ± SD, and were evaluated through analysis of variance (ANOVA), followed by Student's t-test. p < 0.05 were considered statistically significant. The analyses were performed with Origin 7.0 software (Origin Lab Corporation, Northampton, MA, USA).
3 Results
3.1 Isolation and identification of POPs
The extraction scheme of POPs is summarized in Fig. 1. Peptide fragmentation usually break at the amide bond under the ESI-MS/MS conditions when the collision energy is <200 eV. Therefore, the b and y ions are the major fragment ions under such a low energy. The POP-1 was analyzed by HPLC-ESI-MS for molecular mass determination. The ion fragment m/z 781.3146 is regarded as the [M + H2O + H]+ ion, while m/z 763.3050 is regarded as the [M + H]+ ion, m/z 616.2372 is regarded as the y5 ion, and m/z 599.2298 is regarded as the [y5-H2O + H]+ ion. The ion (m/z 453.1736) is the y4 ion, m/z 436.1660 is the [y4-H2O + H]+ ion, m/z 324.1306 is the y3 ion, and m/z 267.1086 is the y2 ion. The ion (m/z 249.0982) is the fragment [y2-H2O + H]+, the ion (m/z 221.1034) is the fragment [y2-COOH + H]+. On the basis of this, we concluded that the sequence of the peptide is EHGEYE (Fig. 2). Finally, we have successfully isolated eleven POPs and the amino acid sequences were identified by HPLC-ESI-MS analysis (Table 1). The purity of POPs was determined by HPLC (>97%), and were dissolved in PBS in a concentration of 10.0 mM as a stock solution and stored at −20 °C.
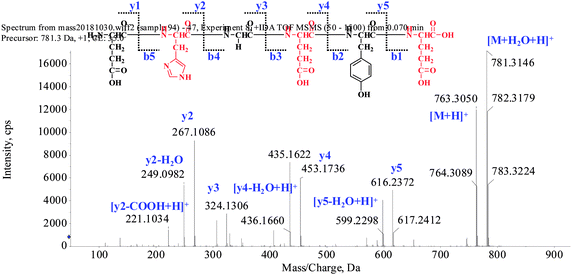 |
| Fig. 2 Structure and MS/MS spectra of POP-1. | |
Table 1 Amino acid sequences and protective activity of POPsa
No. |
Amino acid sequence |
Yield (%) |
Survival rate (%) |
RAW264.7 cells were seeded in 96-well plates and were treated with the POPs 10 μM for 24 h, then treated with LPS (1 μg mL−1) for 12 h. Ultimately, the cell viability was detected with CCK8 kit. |
POP-1 |
EHGEYE |
0.6 |
92.5 ± 6.85 |
POP-2 |
FSHTYV |
0.2 |
79.8 ± 6.03 |
POP-3 |
EGFHL |
0.3 |
84.2 ± 5.47 |
POP-4 |
HAGYSWA |
0.7 |
80.0 ± 4.26 |
POP-5 |
FRHALS |
0.4 |
87.1 ± 5.98 |
POP-6 |
FKEHGY |
0.5 |
89.7 ± 5.57 |
POP-7 |
FSHRGH |
0.4 |
83.6 ± 5.52 |
POP-8 |
EGHGF |
0.5 |
80.6 ± 6.19 |
POP-9 |
FSTHGG |
0.4 |
82.4 ± 5.54 |
POP-10 |
WEHKHA |
0.2 |
87.9 ± 4.60 |
POP-11 |
THAWSV |
0.3 |
79.8 ± 4.45 |
LPS |
— |
|
77.3 ± 7.29 |
Control |
— |
|
100 ± 5.11 |
Studies have identified that some natural products performed excellent anti-proliferative activity when utilized in anticancer efficacy.10 Therefore, we examined the cytotoxicity of POPs in RAW264.7 cells. All the POPs showed no cytotoxicity in RAW264.7 cells under 3.5 mM. So, further study was carried out to explore the anti-inflammatory potency of the POPs in RAW264.7 cells. Compared with the control group (cell viability was set as 100%), the cell viability was reduced by 22.7%, which revealed that the cells were suffered a serious injury under the LPS stimuli. Interestingly, the POPs performed excellent protective potency. POP-1, 5, 6 and 10 significantly attenuated the damages induced by LPS, and increased the cell viability by 15.2%, 9.80%, 12.4% and 10.6%, respectively. Of note, POP-1 significantly attenuated the inflammatory damage induced by the LPS. Hence, further study was performed to explore the underlying mechanism.
3.2 POP-1 attenuated the NO, PGE2 productions and suppressed iNOS, COX-2 expressions in LPS-induced RAW264.7 cells
LPS is the principal component of the outer membrane Gram-negative bacteria and is always used as an inducer of inflammation and oxidative damage in macrophages. NO, which is one of the major inflammatory mediators, is controlled by NO synthases (NOS), with inducible NOS (iNOS) markedly up-regulated in inflammatory disorders. Prostaglandin E2 (PGE-2) is a principal mediator of inflammation in diseases such as rheumatoid arthritis and osteoarthritis.11
To investigate the anti-inflammatory effects of POP-1, the levels of PGE-2 and NO were detected. As shown in Fig. 3A, compared with the control group, the NO level in LPS group was increased by 10.1 folds. However, compared with the LPS group, POP-1 treatments remarkably down-regulated the NO production by 20.5% and 71.1%, respectively. Moreover, POP-1 treatments down-regulated the LPS induced PGE-2 production by 24.5% and 75.5%, respectively.
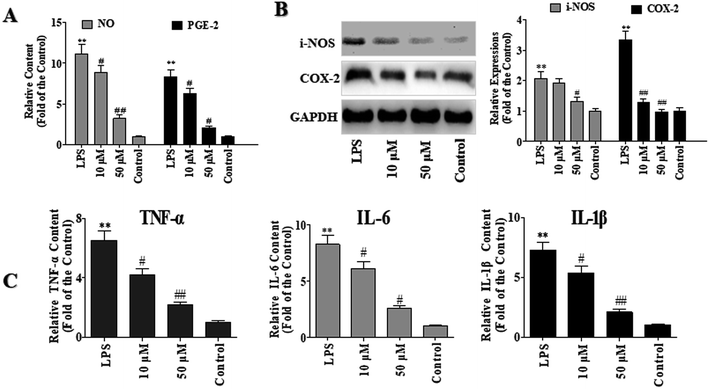 |
| Fig. 3 RAW264.7 cells were seeded in 6-well plates (4 × 106 cells per mL) and treated as experimental design. After that, the NO, PGE2, IL-6, TNF-α and IL-1β contents and the iNOS or COX-2 expressions were evaluated. GAPDH is used as the internal standard of process control. The values are expressed as means ± SD of triplicate tests. *p < 0.05 and **p < 0.01, vs. control, #p < 0.05 and ##p < 0.01, vs. LPS, indicate statistically significant. (A) Production of NO and PEG-2 with treatment of LPS and different concentration of POP-1. (B) Expressions of iNOS and COX-2 with treatment of LPS and different concentration of POP-1. (C) Expressions of TNF-α, IL-6, and IL-1β with treatment of LPS and different concentration of POP-1. | |
The iNOS and COX-2 are key enzymes of NO and PGE-2 production, respectively. In current study, the effects of POP-1 on LPS-induced iNOS and COX-2 expressions were explored, and the results were shown in Fig. 3B. The LPS markedly increased the iNOS and COX-2 expressions, whereas both the expression of iNOS and COX-2 were significantly down-regulated after the POP-1 treatments. This result is consistent with the NO and PGE2 levels reduction detected above. The results indicated that POP-1 may attenuate the iNOS and COX-2 expressions through the regulation of transcriptional mechanism.
3.3 Effect of POP-1 on TNF-α, IL-6 and IL-1β productions
The iNOS mediated NO production is linked with many pathophysiological conditions such as inflammation. In response to LPS stimuli, macrophages produce several cytokines such as TNF-α, IL-6, and IL-1β. Over production of inflammatory cytokines may disturb the balance between immunity and inflammatory responses which are association with abnormal physiological condition.12
To determine the potency of POP-1 on the TNF-α, IL-6, and IL-1β productions, RAW264.7 cells were induced with LPS, and treated with POP-1 as experimental design. After that, the contents of TNF-α, IL-6, and IL-1β in culture media were evaluated using ELISA kits.
As shown in Fig. 3C, compared to the control groups, the TNF-α, IL-6, and IL-1β levels were markedly increased by LPS stimuli by 5.53, 7.26 and 6.25 folds, respectively. However, the POP-1 markedly decreased the levels of TNF-α (66.6%, p < 0.05), IL-6 (69.1%, p < 0.05), and IL-1β (71.2%, p < 0.05) at 50 μM, respectively. Consistent with the results that POP-1 attenuated the LPS induced NO production and decreased the iNOS expression. Here, the results suggested that POP-1 performed the anti-inflammatory effect may by inhibiting iNOS mediated NO production, as well as TNF-α, IL-6, and IL-1β productions.
3.4 POP-1 attenuated the apoptosis and regained the cell cycle
Cell apoptosis may widespread during the development and represents a pre-lethal phase of programmed reaction to injury on a schedule set by various hormonal, nutritional, and macro-environmental factors.13 Flow cytometry using propidium iodide (PI) and Annexin-V as dyes to label viable and dead cells is one of the common techniques to study cell apoptosis/necrosis, therefore it was applied in current study and the results were list in Fig. 4A. Compared to the control group, the total apoptosis was increased by 33.4% by the LPS stimuli. It's suggested that LPS induced fatal damages to the cells. It is gratifying that after the POP-1 treatments, the total apoptosis were decreased by 16.6% and 20.8%, respectively. However, at the concentration of 10 μM, POP-1 was shown to enhance early apoptosis of LPS. POP-1 was shown to attenuate later apoptosis and total apoptosis of LPS both at concentration of 10 μM and 50 μM. Cell apoptosis provided visually evidence for the intracellular protective property of POP-1. Here, our studies display preliminary data related to the ability of POP-1 to attenuate the damages induced by LPS. The results revealed that POP-1 could efficiently attenuate the LPS induced cell apoptosis.
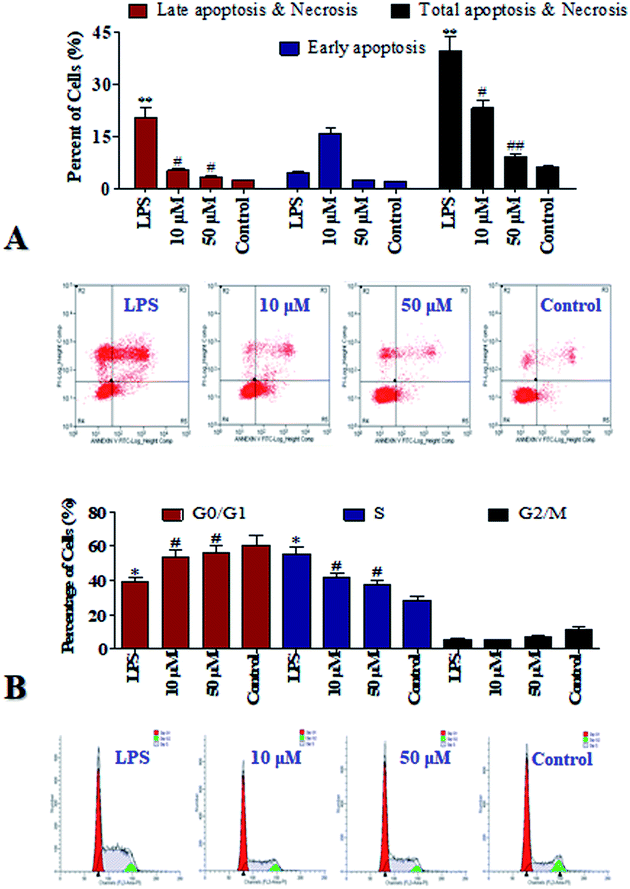 |
| Fig. 4 Apoptosis and cell cycle analysis of RAW264.7 cells under POP-1 treatments. RAW264.7 cells were treated as experimental design. (A) After the treatment, cells were stained with Annexin-V and PI. The apoptosis was explored by flow cytometry. The evaluation of apoptosis is via Annexin V: FITC Apoptosis Detection Kit followed the manufacture's protocol. Representative scatter diagrams. In each scatter diagrams, the abscissa represents the fluorescence intensity of the cells dyed by Annexin V; and the ordinate represents the fluorescence intensity of the cells dyed by PI. The lower left quadrant shows the viable cells, the upper left shows necrotic cells, the lower right shows the early apoptotic cells; while the upper right shows late apoptotic cells. (B) After the treatment, the cells were collected, fixed in 70% ethanol for 12 h, and stained with propidium iodide solution. G0/G1: quiescent state/growth phase; S: initiation of DNA replication; G2/M: biosynthesis/mitosis phases. The values are expressed as means ± SEM of triplicate tests. *p < 0.05 and **p < 0.01, vs. control, #p < 0.05 and ##p < 0.01, vs. LPS, indicate statistically significant difference. | |
Notably, according to the DNA content which regulates cell growth and replication, the cell cycle can be divided into three phases including: G0/G1, S and G2/M.12 To further investigate the mechanism of cell cycle in inflammation which influenced by LPS, the cell cycle phase distributions were detected, and the profiles were listed in Fig. 4B. Compared to the control group, an increase of 27.1% in the S population was observed in LPS group. So, RAW264.7 cells can be significantly blocked in S phase by LPS. Interestingly, with POP-1 treatments, the populations in S phase were decreased by 14.2% and 18.0%, respectively. Here comes the conclusion that the cell cycle arresting is also contributed to the protective potency of POP-1 in attenuating the damages by LPS stimuli. On the basis of that, the protective activity and involved mechanism were further explored.
3.5 Effects of POP-1 on NF-κB signaling pathway
To determine the role of NF-κB signaling pathway in POP-1 mediated inhibition of LPS-induced inflammatory response, we explored the activation of NF-κB in RAW264.7 cells. The results demonstrated that POP-1 altered the NF-κB signaling by suppressing the phosphorylation of p65, IKKα/β and IκBα (Fig. 5A). In addition, the POP-1 treatments also significantly blocked the degradation of LPS induced IκBα in the RAW264.7 cells.
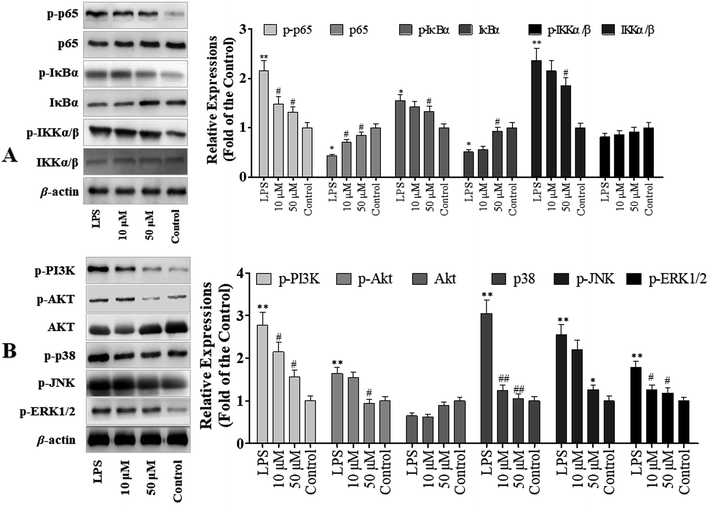 |
| Fig. 5 RAW264.7 cells were treated as experimental design. After that, the cell signaling proteins were explored by western blotting. The values are expressed as means ± SD of triplicate tests. *p < 0.05 and **p < 0.01, vs. control, #p < 0.05 and ##p < 0.01, vs. LPS, indicate statistically significant difference. β-Actin was used as an internal standard of process control and blot band densitometry was analyzed with ImageJ software. (A) Effects of POP-1 on NF-κB signaling pathway. (B) Effects of POP-1 on MAPKs and PI3K-Akt signaling molecules activation. | |
3.6 Effects of POP-1 on MAPKs and PI3K-Akt signaling molecules activation
Studies demonstrated that the activation of Akt and MAPKs signaling pathways is important to initiate the NF-κB signal transduction pathway.14 Hence, we explored the potency of POP-1 on phosphorylation of Akt. As showed in Fig. 5B, the LPS induction significantly up-regulated Akt phosphorylation, while POP-1 treatments suppressed it. Further, the effect of POP-1 on phosphorylation activity of three important MAPKs signaling molecules (JNK, ERK1/2, and p38) was also explored. The JNK, ERK1/2, and p38 phosphorylation levels in macrophages were significantly up-regulated by LPS induction, but POP-1 significantly suppressed the phosphorylation of JNK, ERK1/2, and p38, respectively.
4 Discussion
The Portulaca oleracea L. is an edible vegetable, and is widely used as a folk medicine in many countries. In current study, we isolated the Portulaca oleracea L. oligopeptides and explored their anti-inflammatory potency in LPS induced RAW264.7 cells.
Excessive generation of inflammatory mediators (NO and PGE2), and pro-inflammatory cytokines (IL-1β, IL-6 and TNF-α) work synergistically with other inflammatory mediators, play significant role in inducing the inflammatory processes. In addition, IL-1β, IL-6 and TNF-α are important inflammatory cytokines involved in inflammation-induced injuries. The NO is triggered by iNOS in macrophage and results in cellular damage at inflammatory sites. Likewise, COX2 catalyzes the production of PGE2 and ultimately induces fever at inflammatory sites. In current study, POP-1 suppressed iNOS and COX2 productions in LPS induced RAW264.7 cells. Moreover, POP-1 also suppressed the IL-1β, IL-6 and TNF-α secretion in LPS-stimulated RAW264.7 cells.
As a key transcription factor involved in inflammation, NF-κB activation can induce expressions of iNOS, COX2 and various inflammatory cytokines such as TNF-α. Under normal conditions, NF-κB is bound with IκB, an inhibitory subunit, and located in the cytoplasm as an inactive complex. When triggered by LPS-TLR4 signaling transduction, the Iκ-α is proteolytically degraded, which results in the translocation of NF-κB p65 substrate to nucleus and gene transcription. In current study, the results revealed that POP-1 significantly inhibited LPS-stimulated inflammation response in RAW264.7 cells by inhibiting NF-κB signaling pathway.
MAPKs are involved in various cellular functions under physiological and pathological conditions, and also play key roles in inflammatory reactions. MAPKs family has been shown to play important parts in LPS-stimulated expression of iNOS, COX2, and pro-inflammatory cytokines in activated RAW264.7 cells. Increasing evidence has revealed that some extracts of medicinal herbs could inhibit the expression of pro-inflammatory genes by regulating the phosphorylation of MAPK pathways. This study demonstrated that the levels of phosphorylation of ERK1/2, JNK and p38 MAPK were up-regulated in RAW264.7 cells following LPS-stimulation, but remarkably down-regulated by POP-1.
5 Conclusion
In conclusion, POP-1 showed excellent anti-inflammatory potency by inhibiting NO and pro-inflammatory cytokines production, and the anti-inflammatory role of POP-1 is primarily involved in NF-κB activation and MAPKs phosphorylation. These results suggest that POP-1 may be a potential anti-inflammatory agent. However, both animal and human studies should be designed in the further study while extrapolations cannot be made between cell culture studies to humans.
Author contributions
Shihui Chang and Liping Wang performed experiments, analyzed data, and review the manuscript. Ting Zhang wrote the manuscript. Yan Nie designed and supervised the study, and wrote the manuscript. Ruijie Liu and Lihua Ma provided intellectual input and aided in the experimental design. All authors read and approved the final version of the manuscript.
Conflicts of interest
The authors hereby declare that there are no conflicts of interest.
Acknowledgements
We thank Professor Chen for technical assistance as well as critical editing of the manuscript.
References
- P. Arner, Introduction: the inflammation orchestra in adipose tissue, J. Intern. Med., 2010, 262(4), 404–407 CrossRef PubMed.
- L. E. Wagenknecht, et al., A Comparison of Risk Factors for Calcified Atherosclerotic Plaque in the Coronary, Carotid, and Abdominal Aortic Arteries: The Diabetes Heart Study, Am. J. Epidemiol., 2007, 166(3), 340–347 CrossRef.
- M. C. Lovett, et al., Quantitative assessment of hsp70, IL-1β and TNF-α in the spinal cord of dogs with E40K SOD1 -associated degenerative myelopathy, Vet. J., 2014, 200(2), 312–317 CrossRef CAS PubMed.
- Y. S. Wang, et al., Ginsenoside compound K inhibits nuclear factor-kappa B by targeting Annexin A2, J. Ginseng Res., 2019, 43(3), 452–459 CrossRef PubMed.
- S. Cojocaru, V. Bârsan and A. Ceulemans, T11 target structure induced modulations of the pro-inflammatory and anti-infammatorycytokine expressions in experimental animals for glioma abrogation, Int. Immunopharmacol., 2015, 24(2), 198–207 CrossRef PubMed.
- F. Li, et al., Xanthohumol attenuates cisplatin-induced nephrotoxicity through inhibiting NF-κB and activating Nrf2 signaling pathways, Int. Immunopharmacol., 2018, 61, 277–282 CrossRef CAS PubMed.
- F. L. Li, et al., Preparation and antidiabetic activity of polysaccharide from Portulaca oleracea L., Afr. J. Biotechnol., 2009, 8(4), 569–573 CAS.
- K. Chan, et al., The analgesic and anti-inflammatory effects of Portulaca oleracea L. subsp. Sativa (Haw.) Celak, J. Ethnopharmacol., 2000, 73(3), 445–451 CrossRef CAS.
- H. J. Yoon, et al., Chitosan oligosaccharide (COS) inhibits LPS-induced inflammatory effects in RAW 264.7 macrophage cells, Biochem. Biophys. Res. Commun., 2007, 358(3), 954–959 CrossRef CAS.
- D. H. Han, J. Jinhyun and K. Jeonghee, Anti-proliferative and apoptosis induction activity of green tea polyphenols on human promyelocytic leukemia HL-60 cells, Anticancer Res., 2009, 29(4), 1417–1421 CAS.
- M. H. Tran, et al., Phenolic glycosides from Alangium salviifolium leaves with inhibitory activity on LPS-induced NO, PGE(2), and TNF-alpha production, Bioorg. Med. Chem. Lett., 2010, 40(48), 4389–4393 Search PubMed.
- G. Mazzarella, et al., Phenotypic features of alveolar monocytes/macrophages and IL-8 gene activation by IL-1 and TNF-α in asthmatic patients, Allergy, 2010, 55(s61), 36–41 Search PubMed.
- J. Li, et al., Analysis of Staphylococcus aureus cell viability, sublethal injury and death induced by synergistic combination of ultrasound and mild heat, Ultrason. Sonochem., 2017, 39, 101–110 CrossRef CAS.
- M. Xie, et al., MicroRNA-132 and microRNA-212 mediate doxorubicin resistance by down-regulating the PTEN-AKT/NF-κB signaling pathway in breast cancer, Biomed. Pharmacother., 2018, 102, 286–294 CrossRef CAS.
Footnote |
† Contributed equally to this work. |
|
This journal is © The Royal Society of Chemistry 2020 |
Click here to see how this site uses Cookies. View our privacy policy here.