DOI:
10.1039/C9RA10446A
(Paper)
RSC Adv., 2020,
10, 10368-10381
Lactobacillus plantarum KFY02 enhances the relieving effect of gardenoside on montmorillonite induced constipation in mice
Received
12th December 2019
, Accepted 12th February 2020
First published on 11th March 2020
Abstract
Lactobacillus plantarum KFY02 (KFY02), isolated from naturally fermented milk yoghurt in Korla, Xinjiang, Northwest of China, showed gardenoside action for the intestinal regulation of constipated mice. Comparatively, the effects of KFY02 (0.5 × 108 CFU kg−1, by body weight (BW)), gardenoside (50 mg kg−1, BW), and KFY02 (0.5 × 108 CFU kg−1, BW) + gardenoside (50 mg kg−1, BW) on intestinal regulation in mice with montmorillonite-induced constipation were also studied. Enzyme linked immunoassay, hemotoxylin and eosin (H&E) staining, quantitative polymerase chain reaction (qPCR) assay and high performance liquid chromatography (HPLC) analysis were used for the study. Compared with the model group, KFY02 + genipin (combined group) increased the propelling rate of activated carbon in the small intestines of mice and accelerated the discharge of the first black stool in mice. At the same time, the combination group reduced the levels of motilin (MTL), substance P (SP) and endothelin-1 (ET-1) in the serum, and increased the somatostatin (SS), vasoactive intestinal peptide (VIP), acetylcholinesterase (AchE) and gastrin (Gastrin) levels in the serum, which made these parameters close to those of the normal group. Using qPCR analysis, it was observed that the combined group up-regulated the mRNA expression of endothelial nitric oxide synthase (eNOS), stem cell factor (SCF), stem cell factor receptor (c-Kit), glutathione (GSH), catalase and manganese-superoxide dismutase (Mn-SOD) and down-regulated the expression of inducible nitric oxide synthase (iNOS) and transient receptor potential vanilloid receptor 1 (TRPV1). The combination increased the Bacteroides and Akkermansia abundances and decreased the Firmicutes abundance in the feces of the constipated mice and decreased the Firmicutes/Bacteroides ratio. The expression of the above genes was similar to that of the normal group. The results indicate that KFY02 produced β-glucosidase to hydrolyze the gardenoside glycosidic bond to produce genipin and can effectively promote the regulation of gastrointestinal hormones and intestinal peristalsis and reduce oxidative tissue damage in constipated mice. This study also confirmed that KFY02 has similar relieving effects to gardenoside for constipation in mice.
1. Introduction
Xinjiang is the largest provincial administration of China; it is located in the northwest and represents one-sixth of the landmass of the country. Its geographical environment provides advantages for the livestock industry, and local nomadic families have a recorded history of traditional dairy production. Fermented dairy products are rich in lactic acid bacteria (LAB) with beneficial probiotic characteristics becoming increasingly popular worldwide.1 They are usually produced by natural fermentation of the whole milk using the microorganisms contained in the previously fermented yoghurt. After thousands of years of domestication, these traditional dairy products retain many of the lactic acid bacteria and hence the unique flavor of dairy products.2,3
Lactic acid bacteria, which are widely distributed in nature, are safe and edible microorganisms and are the main intestinal probiotics, usually existing in a balanced state with harmful bacteria in the intestines. If the number of harmful bacteria in the intestine increases and the probiotics decrease, dyspepsia and dysfunction of the digestive tract will occur.4 According to the existing research, probiotic strains can promote health through different mechanisms and it is primarily colonized in the gut and supports a healthy digestive tract and balanced immune system by inhibiting the potential pathogens and producing useful metabolites or enzymes.5,6 Chronic diarrhea, constipation, bloating, indigestion and other symptoms are related to an imbalance of lactic acid bacteria in the intestine.7,8
Gardenoside, extracted from gardenia of the Rubiaceae family, has been reported to possess anti-inflammatory, anti-oxidative, and anti-diabetic properties, to alleviate soft tissue damage and possesses other pharmacological effects.9–11 Studies have shown that the combination of probiotics and gardenoside can enhance its physiological activity and promote the absorption of gardenoside in the body to achieve better disease prevention and treatment effects. The mechanism for the microbial transformation of gardenoside is to ferment gardenoside using a β-glucosidase-producing bacterium, and β-glucosidase can break the chemical bond of gardenoside to produce genipin.12 Qian et al.13 demonstrated that the Lactobacillus casei strain Shirota can enhance the anti-proliferative effect of gardenoside on human oral squamous cell carcinoma HSC-3 cells using in vitro cell experiments. Kim et al.14 used the β-glucosidase produced by Lactobacillus antri (rBGLa) to hydrolyze gardenoside to a smaller molecule of genipin and expressed it in E. coli to increase the content of genipin. Genipin has significant effects on the digestive system, cardiovascular system, and central nervous system diseases, and also has certain anti-inflammatory, antihypertensive, and laxative effects, and can be used to treat soft tissue damage and liver diseases.15 In our previous experiment, our team isolated the lactic acid bacterium KFY02, from Xinjiang naturally fermented yogurt and used it to ferment gardenoside, gardenoside and genipin were detected using high performance liquid chromatography in this study. The beneficial microorganisms and gardenoside in L. plantarum can be used to benefit the intestinal tract.
Montmorillonite powder is an over the counter (OTC) drug used for treating diarrhea. Moderate intake can alleviate diarrhea, and a high dosage can cover the surface of the digestive tract and combine with the mucosal proteins of the digestive tract to reduce the moisture content in the digestive tract, reducing the frequency of gastrointestinal movements, prolonging the retention time of the intestinal contents, reducing the amount and frequency of bowel movements, and increasing stool hardness leading to constipation.16,17 Therefore, montmorillonite powder was used in this study to induce constipation in mice to evaluate the effects of KFY02 in combination with gardenoside on intestinal regulation and laxative effects.
2. Materials and methods
2.1. Materials
Gardenoside with more than 98% purity was purchased from the Sigma-Aldrich Chemical Co. (St. Louis, MO, USA). The experimental strain was KFY02 isolated from the traditional fermented milk yoghurt in Korla of Xinjiang, and deposited in the China General Microbiological Culture Collection Center (CGMCC, Beijing, China) with an access code CGMCC no. 15638. Trizol reagent was purchased from Gene Copoeia Inc. (Invitrogen, Carlsbad, CA, USA). Oligo (Dt) primer, Ribolock RNase Inhibitor, dNTP mix, 5× reaction buffer, Revert Aid M-mu/v RT, SYBR Green PCR Master Mix, and polymerase chain reaction primer (Table 1) were obtained from Thermo Fisher Scientific (Waltham, MA, USA). Motilin (MTL), somatostatin (SS), substance P (SP), vasoactive intestinal peptide (VIP), endothelin (ET-1), acetylcholinesterase (AchE), and gastrin (Gastrin) enzyme-linked immunosorbent assay (ELISA) kits were purchased from Beijing Chenglin Biotechnology Co., Ltd. (Beijing, China). The total superoxide dismutase (T-SOD), catalase (CAT), malondialdehyde (MDA), glutathione (GSH) and the glutathione peroxidase (GSH-PX) kit were obtained from Nanjing Institute of Bioengineering (Nanjing, Jiangsu, China). All other chemicals were of reagent grade.
Table 1 The oligonucleotide primers used for qRT-PCR
Gene name |
Primer sequence |
SCF |
F:5′-GAATCTCCGAAGAGGCCAGAA-3′ |
R:5′-GCTGCAACAGGGGGTAACAT-3′ |
c-Kit |
F:5′-GCAGGTTGTCCAACTTATTGAGA-3′ |
R:5′-GCAGTTTGCCAAGTTGGAGT-3′ |
GSH1 |
F:5′-GGGTGAAGCACAAGAAAGAAGG-3′ |
R:5′-TTGGCTGAGGAGCGAAGA-3′ |
Catalase |
F:5′-GGAGGCGGGAACCCAATAG-3′ |
R:5′-GTGTGCCATCTCGTCAGTGAA-3′ |
Cu/Zn-SOD |
F:5′-GAAGAGAGGCATGTTGGAGA-3′ |
R:5′-CCAATTACACCACGAGCCAA-3′ |
Mn-SOD |
F:5′-CAGACCTGCCTTACGACTATGG-3′ |
R:5′-CTCGGTGGCGTTGAGATTGTT-3′ |
nNOS |
F:5′-GAG AGG ATT CTG AAG ATG AGG-3′ |
R:5′-TTG CTA ATG AGG GAG TTG TTC-3′ |
iNOS |
F:5′-GTTCTCAGCCCAACAATACAAGA-3′ |
R:5′-GTGGACGGGTCGATGTCAC-3′ |
eNOS |
F:5′-TCAGCCATCACAGTGTTCCC-3′ |
R:5′-ATAGCCCGCATAGCGTATCAG-3′ |
TRPV1 |
F:5′-AGCGAGTTCAAAGACCCAGA-3′ |
R:5′-TTCTCCACCAAGAGGGTCAC-3′ |
GDNF |
F:5′-GGGGTATGGAGAAGTTGGCTAG-3′ |
R:5′-CTATGAGAATGCTGCCGAAAA-3′ |
Firmicutes |
F:5′-TGAAACTYAAAGGAATTGACG-3′ |
R:5′-ACCATGCACCACCTGTC-3′ |
Akkermansia |
F:5′-GGAGATTACTGCCCTGGCTCCTA-3′ |
R:5′-CACTCATCGTACTCCTGCTTGTTGCTG-3′ |
Bacteroides |
F:5′-CRAACAGAATTAGATACCCT-3′ |
R:5′-GGTAAGGTTCCTCGCGTAT-3′ |
GAPDH |
F:5′-AGGTCGGTGTGAACGGATTTG-3′ |
R:5′-GGGGTCGTTGATGGCAACA-3′ |
2.2. Ethics statement
Fifty SPF 6 week-old male Kunming mice were provided from the Center of Experimental Animals of Chongqing Medical University (Chongqing, China), and the Animal license number was SYXK 2018-0003. This study was approved by the Ethics Committee of Chongqing Collaborative Innovation Center for Functional Food (201903003B). The mice were housed in an air-conditioned room with the laboratory temperature maintained at 25 ± 1 °C and at a relative humidity of 50 ± 5%, with a 12/12 hour light/dark cycle and provided with food and water ad libitum.
2.3. In vitro resistance test for lactic acid bacteria
2.3.1. Determination of lactic acid bacteria tolerance in artificial gastric juice. An artificial gastric juice was prepared: 0.2% NaCl, 0.35% pepsin, the pH was adjusted to 3.0 with 1 mol L−1 HCl, and the cells were sterilized by filtration. 5 mL of the culture medium containing the bacteria was taken, and the cells were obtained by centrifugation at 3000 rpm for 10 min. These were washed twice with sterile saline and resuspended in 5 mL of normal saline, mixed with the bacterial suspension with artificial gastric juice in a volume ratio of 1
:
9, then shaken well and incubated in a constant temperature incubator for 3 h at 37 °C. The viable count was determined by plate count at 0 and 3 h, respectively, and the survival rate was calculated according to eqn (1):18 |
 | (1) |
2.3.2. Determination of growth efficiency in lactic acid bacteria bile salts. The bacterial suspension was inoculated with 2% of the inoculum in MRS-THIO medium (0.2% sodium thioglycolate in MRS medium) containing 0.0% and 0.3% bovine bile salt. Using a blank medium (uninoculated MRS-THIO medium) as a control, the samples were placed in a constant temperature incubator for 24 h at 37 °C. The optical density (OD) values of the above-mentioned different concentrations of the culture medium were measured at 600 nm by fluorescence spectrophotometer (Evolution 220, Thermo Fisher Scientific, Waltham, MA, USA), and the tolerance of the strain to the bile salt was calculated according to eqn (2):19 |
 | (2) |
2.3.3. Determination of the surface hydrophobicity of lactic acid bacteria. Determination of the surface hydrophobicity of the strains using BATH (carbon hydrocarbon compound adhesion method): 5 mL of the culture medium containing the bacteria was removed, centrifuged at 3000 rpm for 10 min, and the supernatant medium was discarded to collect the cells, and then washed twice with 5 mL of phosphate buffered saline (PBS) buffer. Using PBS buffer as a blank control, the cell concentration was adjusted with PBS buffer to make the OD560 nm value about 1.00 (A0). 4 mL of the adjusted bacterial concentration was placed in a 10 mL sterile centrifuge tube, and 0.8 mL of xylene was added, it was then shaken on a vortex for 30 s, and paused for 10 s. After repeating twice, the mixture was allowed to stand for 5 to 10 min, and the lower aqueous phase was removed. The OD560 nm value (A) was determined, and PBS buffer was used as a blank control. The hydrophobicity rate was calculated according to eqn (3):20 |
 | (3) |
2.3.4 HPLC for the transformation experiment of gardenoside to genipin by KFY02. Gardenoside and KFY02 were mixed in a 5 mL MRS medium, the concentration of gardenoside was adjusted to 2 mg mL−1, and the concentration of KFY02 was adjusted to 0.5 × 108 CFU mL−1. The mixture was incubated at 37 °C for 0 and 24 h, the filtrate was filtered through a 0.22 μm filter, and the gardenoside and KFY02 filtrates were obtained at 0 and 24 h, respectively. A concentration of 2 mg mL−1 of gardenoside and a genipin standard stock solution was prepared using a chromatographic grade methanol solution. The HPLC conditions were as follows: C18 column (2.1 mm × 50 mm, 1.7 μm); column temperature: 25 °C; mobile phase: 35–65% methanol–water: flow rate: 0.6 mL min; detection wavelength: 238 nm; loading volume: 2 μL (Ultimate3000; Thermo Fisher Scientific, Inc., Waltham, MA, USA).
2.3.5. Animal experiments. Fifty 6 week-old male mice were subcultured for 1 week and then grouped into a normal group, model group, KFY02 group, gardenoside group and KFY02 + gardenoside group (combination group), with 10 mice per group. Each mouse was intragastrically treated daily, 0.2 mL of pure water was administered to the normal and model groups, 50 mg kg−1 of gardenoside was administered to the gardenoside group, the KFY02 group was administered 0.5 × 108 CFU kg−1 KFY02, and the combination group were intragastrically administered with 0.5 × 108 CFU kg−1 KFY02 and 50 mg kg−1 gardenoside continuous gavage for 9 d. Constipation was induced in the mice from the 7th day to the 9th day. The normal group did not receive any treatment and the other four groups of mice were given a daily dose of 30 mg kg−1 montmorillonite. After the end of the constipation induction on the 9th day, the mice were starved for 24 h, then all of the mice were intragastrically administrated with 10% activated carbon 0.1 mL/10 g (BW) of ice water, and the mice in each group were divided into two groups. Five mice were observed to record the time of the first black stool, and the other five mice had blood taken from the eyeball after 25 min of oral administration of activated carbon iced water. After dissecting the mouse, the small intestine from the pylorus to the ileocecal region was removed, the total length of the small intestine and the distance the activated carbon had advanced along the small intestine were determined and with reference to the method previously published by Xu et al.,21 the propelling rate of activated carbon in the small intestine was calculated according to eqn (4). During the experiment, the body weight changes of the mice were recorded. Furthermore, 2–4 fresh stools were collected and weighed per mouse every day, and the dry weight was obtained by drying fresh feces in an oven at 70 °C for 48 h. The moisture content of the mouse feces was determined using eqn (5). |
 | (4) |
|
 | (5) |
2.3.6. Mouse serum index detection. The blood from the mice were centrifuged at 4 °C, 4000 rpm for 10 min, and the upper layer of serum was removed. The SS, VIP, AchE, Gastrin, MTL, SP, and ET-1 kit instructions were followed to determine the levels of the mouse SS, VIP, AchE, Gastrin, MTL, SP and ET-1.
2.3.7. Detection of T-SOD, CAT, MDA, GSH and GSH-PX in serum. According to the kit instructions, the blood of the mice was centrifuged at 4 °C, 4000 rpm for 10 min, and the upper layer of serum was removed to determine the content of the following substances in the serum: T-SOD, CAT, MDA, GSH and GSH-PX.
2.3.8. Small bowel histological examination. The mouse was dissected and the 2 cm small intestine was placed in a centrifuge tube containing 10% formalin solution for 24 h. It was then dehydrated in 95% ethanol, and then placed in xylene to displace the alcohol in the tissue to make the small intestine tissue transparent, and then embedded in paraffin. Then, the wax block was sliced using a microtome and stained with hematoxylin and eosin (H&E) to prepare a small intestinal pathological section of the mouse, which was observed under a biological microscope (BX43; Olympus, Tokyo, Japan).
2.3.9. RT-qPCR assay. The small intestine tissue and feces were pulverized using a biological sample homogenizer, and total RNA in the tissue and microorganisms from the feces were extracted with TRIzol reagent. Then, the concentration and purity of the RNA were measured using an ultra-micro spectrophotometer. When the ratio of OD260/OD280 was in the range of 2.0 to 2.2, the RNA was transcribed into cDNA according to the reverse transcription kit method to obtain a cDNA template. The cDNA concentration was determined using a ultra-micro spectrophotometer. 1 μL of the 1000 ng μL−1 cDNA template, 10 μL of SYBR Green PCR Master Mix, 1 μL of forward and reverse primers, and 7 μL of sterile distilled water were mixed. The quantification of relative mRNA concentrations were detected by quantitative reverse transcription polymerase chain reaction (qRT-PCR) using a Real-Time PCR System (StepOnePlus Real-Time PCR System; Thermo Fisher Scientific, Inc., Waltham, MA, USA). The procedure was carried out at 95 °C for 60 s, then at 95 °C for 15 s; 55 °C for 30 s; 72 °C for 35 s for 40 cycles; finally at 95 °C for 30 s; 55 °C for 35 s to carry out testing, and each reaction was performed four times. The relative primers are listed in Table 1. GAPDH was used as the reference gene. The relative expression level of the gene was calculated using the 2−ΔΔCt method.22,23
2.4. Data analysis
The serum and histological assays for each mouse were averaged after three parallel experiments, and then analyzed using SPSS 19.0 statistical software. The one-way analysis of variation (ANOVA) method was used to analyze whether the data for each group had a significant difference at the P < 0.05 level.
3. Results and analysis
3.1. Morphological characteristics of KFY02
As shown in Fig. 1, the colony color of KFY02 on the MRS medium was milky white, round, with neat edges, and had a low protrusion, and a smooth and moist surface. After gram staining, it was observed under the microscope that KFY02 was purple in the form of uniform short rods and no buds, which is consistent with the morphological characteristics of lactic acid bacteria.
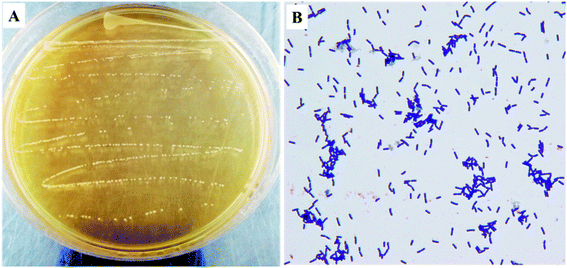 |
| Fig. 1 The morphological characteristics of KFY02: (A) colony morphology; and (B) gram stain results. | |
3.2. Biological barrier resistance and surface hydrophobicity of KFY02
In order to determine whether probiotics can survive and colonize the gastrointestinal tract, screening was performed using artificial gastric juice and bile salt tolerance tests to ensure that probiotics exert their probiotic functions on the human body. The pH value of human gastric juice was generally maintained at about 3.0, and the bile salt concentration was generally between 0.03–0.3%; the residence time of food in this environment is relatively short, usually 1–3 hours. To evaluate the in vitro probiotic properties of KFY02, we measured the survival rate of artificial gastric juice 3.0 and the growth efficiency of 0.3% bile salt. The results showed that KFY02 was almost unaffected by artificial gastric juice and its survival rate was 93.51%. The growth rate was 28.37% in 0.3% bile salt and its surface hydrophobicity was 30.52%. Based on the good bio-barrier resistance and surface hydrophobicity of KFY02, it is preliminarily considered to have potential benefits, and its probiotic characteristics were further evaluated using animal experiments.
3.3. High-performance liquid chromatography assay
The precisely absorbed sample solution (2 μL) was injected into the liquid chromatograph, and run according to the procedure described in the method, and the chromatogram was recorded. The results are shown in Fig. 2. The KFY02 was incubated with the genipin mixture for 0 h (Fig. 2B) and at 37 °C for 24 h (Fig. 2C). It can be seen that there is only a single peak for gardenoside in Fig. 2B. Two peaks appeared in Fig. 2C after processing, and the peak time corresponded to the peak time of gardenoside in the standard figure (5.703 min and the peak time of genipin was 8.690 min). It can be seen that gardenoside can be partially converted to genipin by KFY02.
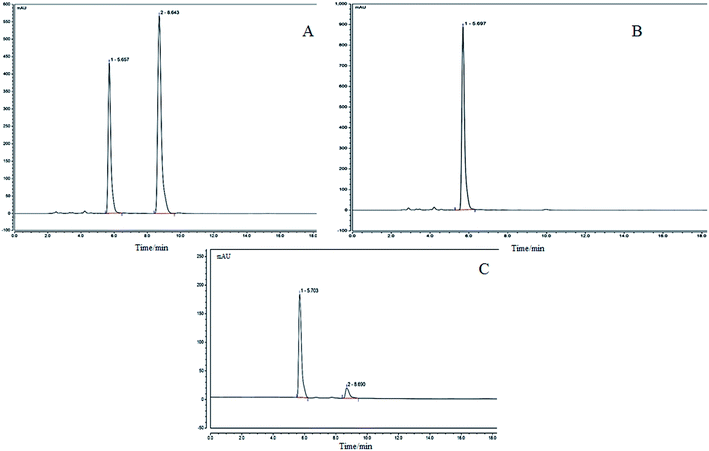 |
| Fig. 2 HPLC for the transformation experiment of gardenoside to genipin by KFY02. (A) Genipin and genipin standards: (1) gardenoside; (2) genipin; (B) gardenoside and KFY02 incubation for 0 h; and (C) gardenoside and KFY02 incubation for 24 h. | |
3.4 Effects of KFY02 and gardenoside on the body weights of mice
The effect of KFY02 and gardenoside on the body weight of constipated mice is shown in Fig. 3. There was no significant difference in the body weight between the normal group and the other groups. However, from the overall trend, the body weight of the normal group was increased during the entire experiment, while the body weight of the model group decreased after 2 d of induction. The body weight changes in the KFY02 group, gardenoside group and combination group were consistent, and the body weight of mice increased linearly from 1 to 6 d. The body weight of mice at 7–9 days was stable, indicating that KFY02 and gardenoside could interfere. The rate of the weight gain in mice was reduced, but the difference between the intervention groups was small.
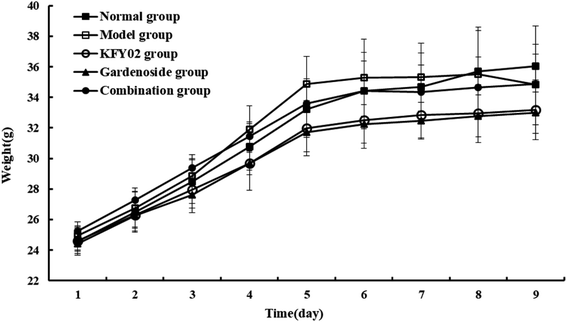 |
| Fig. 3 Effects of KFY02 and gardenoside on the body weights of mice. | |
3.5. Effects of KFY02 and gardenoside on fecal parameters in a mouse model of constipation
Constipation can affect the normal intestinal peristalsis of a patient, resulting in a lower than normal frequency of bowel movements. During the induction of constipation in mice, observation of the discharge time of the first black stool, the intestinal propulsion speed and fecal water content can be used to estimate the intestinal function and determine the degree of constipation. Therefore, we used these three visual indicators to assess the constipation status of mice. Fecal water content is an important indicator for evaluating the effectiveness of constipation models. Constipation patients are affected by fecal stem segments, and a higher water content is often accompanied by the improvement of fecal peristalsis. As shown in Fig. 4, in the first 6 d of the experiment, there was no significant difference in the moisture content of the feces between the groups. After the constipation was induced from the seventh day, except for the normal group, the moisture content of the feces of the other groups was different. On the last day, the model group had a significantly lower fecal water content than the normal group, indicating successful modeling. The water content of feces in the KFY02 group and the gardenoside group was improved compared with the model group. The trend for the water content in the feces of the combined group was similar to that in the normal group, which proved that the combination of KFY02 and gardenoside has the effect of preventing and alleviating constipation.
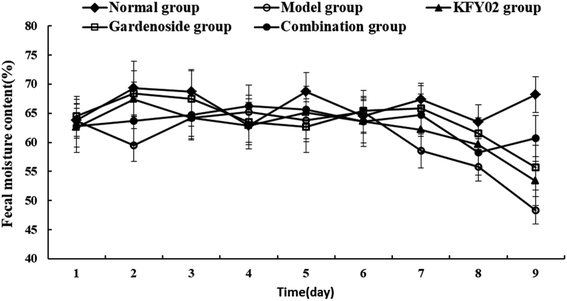 |
| Fig. 4 Effects of KFY02 and gardenoside on the moisture content of mice feces. | |
In order to determine the effect of KFY02 and gardenoside on defecation in model mice, the time of the first black stool after the last administration was recorded. The longer this takes, the more serious the constipation. As shown in Fig. 5, the defecation time for the model group was 179.8 min, which was significantly longer than that of the normal group (P < 0.05). The defecation time of the KFY02 group and the gardenoside group was 147.16 and 130.8 min, respectively, which was significantly shorter than that of the model group (P < 0.05). At the same time, the defecation time of KFY02 combined with gardenoside was similar to that of the normal group, indicating that the combination of KFY02 and gardenoside has a mutual promoting effect and can effectively inhibit constipation.
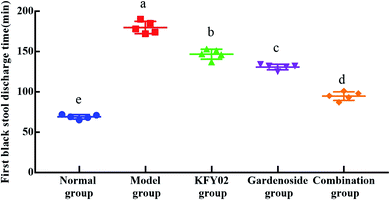 |
| Fig. 5 Discharge times of the first black stool. | |
As shown in Fig. 6 and Table 2, the rate of advancement of activated carbon in the intestinal tract of mice was measured and calculated by intragastric administration of 10% activated carbon for 25 min. In the normal group, the activated carbon almost completely passed through the small intestine, and the propulsion rate was 99.32 ± 1.36%, which was significantly different from the model group (P < 0.05). The small intestine propulsion rates of the KFY02 group and the gardenoside group were 71.53 ± 2.44% and 71.30 ± 3.12%, respectively, while the combined group small intestine propulsion rate was 86.02 ± 2.52%, which was significantly higher than the model group and close to that of the normal group propulsion rate. This indicated that genipin produced by the combination of KFY02 and gardenoside can enhance the medicinal value of gardenoside, which can promote intestinal peristalsis and food advancement, thereby alleviating constipation.
 |
| Fig. 6 Effects of KFY02 and gardenoside on the propellant rate of activated carbon. | |
Table 2 Effects of KFY02 and genipin on the propellant rate of activated carbona
Item |
Normal |
Model |
KFY02 |
Gardenoside |
Combination |
Values presented are the mean ± SD. In the same column, values with different letters in the same column are significantly different (P < 0.05) according to Duncan's multi-range test. Normal: normal mice; model: mice treated with 30 mg kg−1 montmorillonite; KFY02: mice treated with 30 mg kg−1 montmorillonite and KFY02 at a dose of 0.5 × 108 CFU kg−1 per day; gardenoside: mice treated with 50 mg kg−1 gardenoside; combination group: mice treated with 30 mg kg−1 montmorillonite and 0.5 × 108 CFU kg−1 KFY02 + 50 mg kg−1 gardenoside per day. |
Intestinal length (cm) |
56.9 ± 3.01a |
59 ± 2.83a |
55.92 ± 2.06a |
56.8 ± 3.59a |
55.8 ± 2.48a |
Intestinal transit length (cm) |
56.5 ± 2.83a |
36.5 ± 2.41d |
40.8 ± 1.96c |
40.5 ± 2.27c |
48 ± 1.83b |
Intestinal transit ratio (%) |
99.32 ± 1.36a |
61.86 ± 1.35d |
71.53 ± 2.44c |
71.30 ± 3.12c |
86.02 ± 2.52b |
3.6. Histopathological analysis of the small intestine
Studies have shown that damage to the small intestinal villi affects the intestinal tissue to varying degrees, and intestinal peristalsis and slowing of the peristalsis can cause constipation; thus, the small intestinal villus integrity is important for evaluating constipation.23 As shown in Fig. 7, the small intestine villi of the normal group were neat and uniform, without damage or wrinkling. The small intestine villi of the model group were severely ruptured and atrophied, and the goblet cells were incomplete. The appearance of the small intestine villi in the normal group, KFY02 group, gardenoside group and combination group were significantly improved compared with the model group. Although the small intestine villi of the combined group demonstrated contraction and rupture, it was significantly improved compared with the model group, which was arranged in a similar way to the normal group.
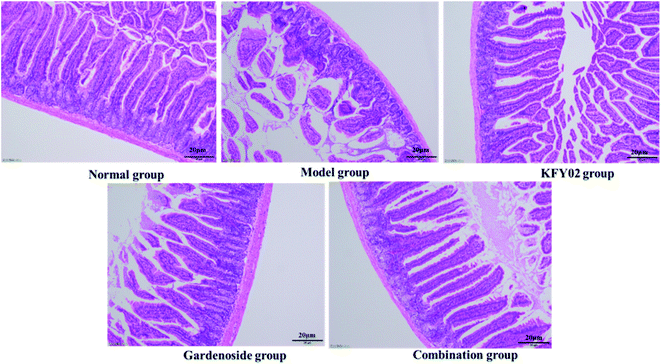 |
| Fig. 7 Observation of histopathology sections of the small intestine. | |
3.7. Effects of KFY02 and gardenoside on serum factors
The serum MTL, Gastrin, ET-1, SS, AchE, SP and VIP levels were detected using an ELISA kit. The results are shown in Table 3, the gastrointestinal motility related to the constipated mice induced by diphenoxylate hydrochloride factors such as AchE, MTL, Gastrin, VIP, and SP were significantly (P < 0.05) decreased. The levels of AchE, MTL, Gastrin, VIP and SP in the normal group were significantly higher than those in the other four groups, and the ET-1 and SS levels were the lowest in the normal group. After intervention with KFY02 and gardenoside serum AchE, MTL, Gastrin, VIP, and SP levels increased significantly, and ET-1 and SS levels were decreased. We noted that KFY02 and gardenoside alone can effectively regulate these serum biomarkers, but the combination of KFY02 and genipin was more effective in preventing constipation, and the levels of indicators were similar to those in the normal group.
Table 3 Effects of KFY02 and gardenoside on serum factorsa
Item |
Normal |
Model |
KFY02 |
Gardenoside |
Combination |
Values presented are the mean ± SD. In the same column, values with different letters in the same column are significantly different (P < 0.05) according to Duncan's multi-range test. Normal: normal mice; model: mice treated with 30 mg kg−1 montmorillonite; KFY02: mice treated with 30 mg kg−1 montmorillonite and KFY02 at a dose of 0.5 × 108 CFU kg−1 per day; gardenoside: mice treated with 50 mg kg−1 gardenoside; combination group: mice treated with 30 mg kg−1 montmorillonite and 0.5 × 108 CFU kg−1 KFY02 + 50 mg kg−1 gardenoside per day. |
AchE |
142.11 ± 16.39a |
69.91 ± 2.45d |
91.83 ± 4.21cd |
103.92 ± 12.13bc |
124.25 ± 24.03ab |
ET-1 |
439.07 ± 41.31d |
604.78 ± 25.19a |
528.77 ± 17.80bc |
493.29 ± 16.35b |
493.30 ± 3.70c |
Gastrin |
12.56 ± 0.98a |
7.68 ± 0.37d |
9.62 ± 0.62c |
10.15 ± 0.50bc |
11.27 ± 0.73b |
MTL |
122.63 ± 0.76a |
86.30 ± 2.05d |
103.68 ± 1.88c |
109.68 ± 6.30bc |
114.98 ± 1.33ab |
SP |
57.72 ± 6.57a |
17.25 ± 1.44e |
24.34 ± 2.46d |
33.65 ± 3.2c |
48.14 ± 2.13b |
SS |
1.02 ± 0.12e |
3.28 ± 0.22a |
2.17 ± 0.09b |
1.81 ± 0.13c |
1.48 ± 0.05d |
VIP |
25.46 ± 3.23a |
9.58 ± 0.29d |
11.47 ± 0.44cd |
15.00 ± 0.73bc |
17.18 ± 0.69b |
3.8. Detection of T-SOD, CAT, MDA, GSH and GSH-PX in serum
As shown in Table 4, T-SOD, CAT, GSH and GSH-PX levels in mice serum were highest in the normal group, while the MDA level was the lowest. The T-SOD, CAT, GSH and GSH-PX levels after constipation induced by difenoxol hydrochloride decreased, while the MDA level increased. KFY02, gardenoside, KFY02 and gardenoside joint action could significantly (P < 0.05) inhibit the decrease in the T-SOD, CAT, GSH and GSH-PX levels and increase the MDA level in serum in the constipated mice induced by difenoxol hydrochloride. The effect of KFY02 and gardenoside work similarly, and can reduce the content of MDA in the serum of constipated mice and increase the enzyme activity of SOD, CAT and GSH-PX.
Table 4 Content of T-SOD, CAT, MDA, GSH and GSH-PX in seruma
Group |
CAT (U mL−1) |
GSH (mol L−1) |
GSH-PX (mol L−1) |
MDA (nmol mL−1) |
T-SOD (U mL−1) |
Values presented are the mean ± SD. In the same column, values with different letters in the same column are significantly different (P < 0.05) according to Duncan's multi-range test. Normal: normal mice; model: mice treated with 30 mg kg−1 montmorillonite; KFY02: mice treated with 30 mg kg−1 montmorillonite and KFY02 at a dose of 0.5 × 108 CFU kg−1 per day; gardenoside: mice treated with 50 mg kg−1 gardenoside; combination group: mice treated with 30 mg kg−1 montmorillonite and 0.5 × 108 CFU kg−1 KFY02 + 50 mg kg−1 gardenoside per day. |
Normal |
4.41 ± 0.28a |
0.73 ± 0.06a |
133.90 ± 8.18a |
3.04 ± 0.59e |
641.42 ± 66.13a |
Model |
0.79 ± 0.09d |
0.26 ± 0.3d |
29.92 ± 8.74d |
17.21 ± 0.98a |
314.44 ± 58.69c |
KFY02 |
2.41 ± 0.35c |
0.45 ± 0.07c |
69.24 ± 4.70c |
12.12 ± 0.55b |
448.01 ± 27.43b |
Gardenoside |
2.74 ± 0.31c |
0.46 ± 0.06c |
80.31 ± 7.22c |
8.79 ± 0.57c |
385.76 ± 89.19bc |
Combination |
3.28 ± 0.12b |
0.62 ± 0.06b |
118.33 ± 9.04b |
6.51 ± 0.44d |
489.96 ± 3.76b |
3.9 mRNA expression levels of Cu/Zn-SOD, Mn-SOD, CAT, GSH-PX and GSH in the small intestinal tissue
Fig. 8 shows that the messenger RNA (mRNA) expressions of cuprozinc-superoxide dismutase (Cu/Zn-SOD), manganese superoxide dismutase (Mn-SOD), CAT, GSH-PX and GSH in the small intestines of the mice in the normal group were the strongest, whereas those in the model group were the weakest. The KFY02 and gardenoside significantly (P < 0.05) increased the expressions of Cu/Zn-SOD, Mn-SOD, CAT, GSH-PX and GSH in the small intestines of the constipated mice. Nevertheless, the effect of the combination was the strongest.
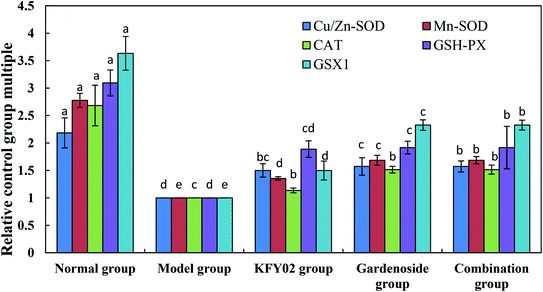 |
| Fig. 8 mRNA expression levels of Cu/Zn-SOD, Mn-SOD, CAT, GSH-PX and GSH in the small intestinal tissue of mice. Values presented are the mean ± standard deviation (N = 10/group). a–eMean values with different letters in the same bars differ significantly (P < 0.05) by Duncan's multiple-range test. CAT, catalase; Cu/Zn-SOD, cuprozinc-superoxide dismutase; Mn-SOD, manganese superoxide dismutase; mRNA, messenger RNA. | |
3.10. mRNA expression levels of TRPV-1, c-Kit, GDNF and SCF in the small intestinal tissue
Fig. 9 shows that the mRNA expressions of c-Kit, GDNF and the stem cell factor (SCF) in the small intestines of the constipated mice (model group) were significantly (P < 0.05) lower than those of the other groups. However, the relative expression of TRPV-1 in the normal group was significantly (P < 0.05) higher than that in the other groups. The KFY02, gardenoside and combination groups upregulated the expressions of c-Kit, GDNF and SCF in the small intestines of the constipated mice, and downgraded the expressions of TRPV-1, and the effects of the combination were greater than those of KFY02 and gardenoside alone.
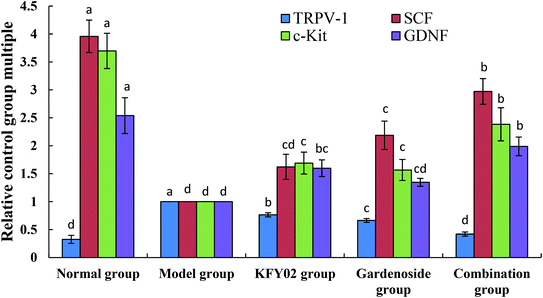 |
| Fig. 9 mRNA expression levels of c-Kit and SCF in the small intestinal tissue of mice. Values presented are the mean ± standard deviation (N = 10/group). a–d Mean values with different letters in the same bars differ significantly (P < 0.05) by Duncan's multiple-range test. SCF: stem cell factor. | |
3.11. mRNA expression levels of nNOS, eNOS, and iNOS in the small intestinal tissue
The mRNA expressions of neuronal nitric oxide synthase (nNOS) and endothelial nitric oxide synthase (eNOS) were the strongest in the small intestines of the normal mice, whereas nNOS expression was the weakest (Fig. 10). Experimental constipation weakened the nNOS and eNOS expressions in the small intestines, whereas the inducible nitric oxide synthase (iNOS) expression was enhanced. KFY02, gardenoside and the combination group inhibited the constipation-induced nNOS, weakened the eNOS expression, and enhanced the iNOS expression. The effects of the combination of gardenoside and KFY02 were better than those of gardenoside and KFY02 alone.
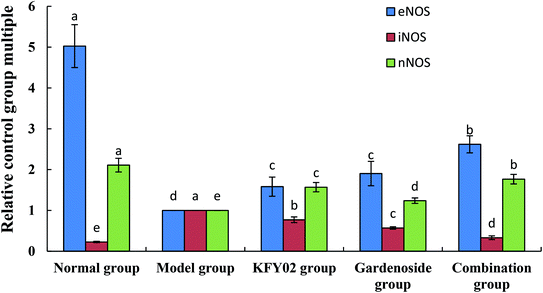 |
| Fig. 10 mRNA expression levels of nNOS, eNOS, and iNOS in the small intestinal tissue of mice. Values presented are the mean ± standard deviation (N = 10/group). a–eMean values with different letters in the same bars differ significantly (P < 0.05) by Duncan's multiple-range test. | |
3.12. Expression of microbial mRNA in mouse feces
Fig. 11 shows that the Bacteroides and Akkermansia abundance in the normal mouse feces were the highest, whereas the Firmicutes levels was the lowest, and the Firmicutes/Bacteroides ratio was significantly lower than that of the other groups (P < 0.05). The effects of the combination group showed that, compared with the model group, the fecal levels of Firmicutes in the KFY02, gardenoside, and combination treated mice decreased, and the levels of Bacteroides and Akkermansia increased, and the Firmicutes/Bacteroides ratio significantly decreased (P < 0.05). Nevertheless, the combination decreased the fecal level of Firmicutes in the constipated mice, increased the levels of Bacteroides and Akkermansia, and decreased the Firmicutes/Bacteroides ratio by significantly more than KFY02 and gardenoside, making the fecal microbial status of the combination mice close to that of the normal mice.
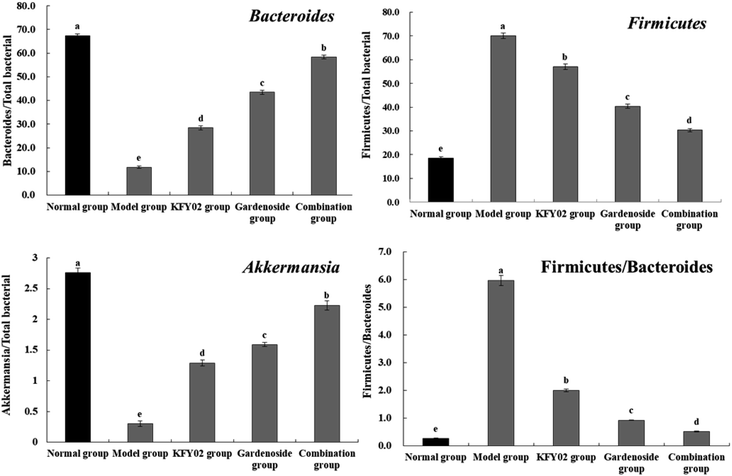 |
| Fig. 11 mRNA expression of microorganisms in the mouse feces. Values presented are the mean ± standard deviation (N = 10/group). a–eMean values with different letters in the same bars differ significantly (P < 0.05) by Duncan's multiple-range test. | |
4. Discussion
Lactic acid bacteria are well known probiotics that have a good resistance to stomach acid and bile salts and can enter the intestinal tract with more viable bacteria and play a probiotic role.24 KFY02 has shown potential for this in this study, such as a good acid resistance, bile salt resistance and an in vivo colonization ability. Gardenoside has been reported to have anti-inflammatory, anti-oxidative, and anti-diabetic properties, alleviate soft tissue damage and possess other pharmacological effects. However, its effect on the gastrointestinal tract is not obvious. Therefore, in this study, we used KFY02 to perform the conversion experiment of gardenoside, which confirmed that KFY02 is able to convert gardenoside to genipin. More studies have shown that probiotics and genipin increase the content of short-chain fatty acids in the intestine, which in turn promotes intestinal movement and defecation, and may play a role in preventing or treating constipation in humans and animals.10,25–27
Constipation is one of the most common gastrointestinal diseases. The main clinical symptoms are infrequent gastrointestinal motility and difficulty in defecation. Studies have confirmed that more severe degrees of constipation significantly reduce the fecal quantity, weight, water content, time to discharge the first black stool, and the activated carbon propulsion rate in experimentally constipated mice.28,29 In this study, KFY02 in combination with gardenoside inhibited the effects of constipation in mice. KFY02 and gardenoside significantly reduced the body weight, fecal water content, time of the first defecation and propellant speed of activated carbon in constipated mice. The results of this experiment are consistent with the results previously published by Yu-Xuan, who studied D-tagatose by regulating the composition of the gut microbiota and thus relieving constipation.30
Clinical studies have shown changes in the neurotransmitter levels in some patients with constipation, including excitatory peptide neurotransmitters MTL, gas, AChE, SP, VIP, inhibitory peptide neurotransmitters ET, and SS. MTL is an important indicator of gastrointestinal motility, which can promote the movement of various parts of the gastrointestinal tract, and the decrease in the MTL release will reduce the frequency of gastrointestinal motility.31 Gastrin is an important gastrointestinal hormone that has a significant impact on the gastrointestinal tract. It can enhance the secretion of gastric juice, stimulate gastrointestinal motility, promote pyloric sphincter relaxation, and accelerate gastric emptying.32 Acetylcholine is currently recognized as improving gastrointestinal motility by receptor binding, it plays an important role in intestinal motility, and is one of the classic neurotransmitters affecting the intestinal wall. Overall, elevated AChE is positively correlated with the acetylcholine ester (ACh) levels.33 SP is an excitatory transmitter of gastrointestinal motor neurons. It promotes the contraction of smooth muscles in the gastrointestinal tract, stimulates the secretion of water and electrolytes in the small intestine and colon mucosa, and promotes gastrointestinal motility.34 VIP stimulates intestinal peristalsis and promotes gastrointestinal motility.35 ET maintains normal intestinal function by maintaining vascular tone and cardiovascular stability.36 SS inhibits the release of gastrointestinal hormones and reduces the gastric emptying speed and smooth muscle contraction, thereby accelerating constipation.37 The results of this study showed that the levels of MTL, Gas, AChE, SP and VIP in the model group were significantly lower than those in the normal group, while the levels of ET and SS were higher than those in the normal group. The combination of KFY02 and gardenoside can help constipated mice restore these neurotransmitter levels to normal and help relieve constipation.
A clinical study has shown that the elderly have different degrees of constipation associated with an increase in age. The proportion of constipation in the elderly is 25–32%; this percentage increases with age. It has been shown that constipation is directly related to the oxidative aging of the body.38 The main clinical symptoms of constipation are infrequent gastrointestinal motility and difficulty in defecating resulting in the long-term accumulation of feces in the intestine, which directly affects the small intestine tissues. Human constipation can cause a range of abnormalities, including significant oxidative stress. SOD, CAT, GSH-PX, MDA and GSH are indicators of significant changes after oxidative stress,39,40 Cu/Zn-SOD and Mn-SOD are usually present in humans and vertebrates (rats, pigs, cattle, etc.). Fe-SOD, the other metal ion superoxide dismutase, is mainly found in the chloroplasts of plant cells, but not in animal cells. Cu/Zn-SOD and Mn-SOD activities of constipated patients are lower than those of non-constipated patients, which may be due to the long-term retention of stools, irritation and intestinal inflammation in patients.41 Therefore, respiratory bursts during polymorphonuclear neutrophils and other phagocytic foreign bodies can produce large amounts of free radicals. With the aggravation of constipation, oxygen free radical reactions and lipid peroxidation are further initiated.42 CAT, GSH and GSH-PX in the body will decrease, but the content of the lipid peroxidation product MDA will increase.43 The regulation of SOD and CAT is the main mechanism of the antioxidant enzymes. SOD can convert harmful superoxide radicals into hydrogen peroxide. Although hydrogen peroxide is a harmful reactive oxygen species, CAT, as an important antioxidant enzyme in the body, can break down H2O2 into harmless water,44 inhibit oxidative stress, and then form an antioxidant chain to reduce intestinal damage caused by constipation. GSH-PX is a peroxolytic enzyme. Its active center is selenocysteine, which can catalyze GSH to GSSG and reduce toxic peroxides to non-toxic hydroxyl compounds, thereby protecting the structure and function of cell membranes and interference and damage from oxides.45 Glutathione can help maintain normal immune system function, and has antioxidant effects and integrated detoxification.46 Genipin not only has significant effects on digestive diseases, but also has anti-inflammatory and reduced lipid peroxidation functions.47 Our research shows that KFY02 and gardenoside can enter the intestine, improve the intestinal microecology, reduce the MDA content in the sera and small intestines of constipated mice, and increase the expression levels of Mn-SOD, Cu/Zn-SOD, GSH and GSH-PX, and the combined intervention of KFY02 and gardenoside have a better effect on regulating oxidation-related expression and improving constipation.
Another study showed that Cajal bodies (ICC), which have an abnormal number of mesenchymal cells, can cause changes in the cell morphology and network structure, which can slow down colonic peristalsis and induce constipation, which may be the basic pathogenesis of slow transit constipation.48,49 c-Kit is one of the specific markers of ICC, and is a factor in maintaining ICC proliferation and growth under normal conditions,50 SCF is a natural ligand of the c-Kit receptor.51 Studies have found that the concentration of SCF is very important to the cultivation of the ICC. Without SCF, the cultivation of ICC cannot survive. A further study on animals with constipation found that with reduced amounts of ICC in the small intestine of mice with constipation, c-Kit and SCF expression levels will decline.52 In our study, KFY02 and gardenoside inhibited the decrease of c-Kit and SCF expression in the small intestine of constipated mice, but the effect of the combination was more obvious. It can increase c-Kit by increasing the amount of ICC in the mice, thus relieving constipation.
TRPV1 has a close relationship with bowel movement and absorption. Activated TRPV1 can trigger the release of the neurotransmitter, which leads to disorders in the movement of the small intestine.53,54 The increasing TRPV1 expression is a striking phenomenon of intestinal damage.55 GDNF can regulate ganglion cells, restore ganglion function, repair damaged intestines, and effectively inhibit constipation.56 The results of this study also confirmed that the combination group played a role in ameliorating constipation in mice by up-regulating the expression of GDNF in mouse small intestine tissues, down-regulating the expression of TRPV1, protecting the function of the small intestine, and thereby enhancing the intestinal activity.
Studies have shown that constipation has a certain relationship with the enteric nervous system. NO is a major inhibitory neurotransmitter in the enteric nervous system, it can cause smooth muscle relaxation and weaken gastrointestinal movement. The increase in nitric oxide synthase (NOS)-positive fibers will lead to an increase in the NO content, which affects intestinal function and leads to constipation.57 NOS is the only rate-limiting enzyme in the process of NO synthesis. Changes in its activity can directly affect the production of nitric oxide and its biological effects. There are three different subtypes of NOS, namely NOS1 (nNOS), NOS2 (iNOS), and NOS3 (eNOS).58 Under normal physiological conditions, NO in vascular endothelial cells is mainly derived from eNOS, and its main role is to regulate normal physiological functions. The expression of nNOS was significantly reduced in the small intestine of constipated animals. In the resting state, iNOS is not expressed, but can cause a large amount of iNOS and NO production under various pathological conditions.59,60 Therefore, controlling the expression of NOS can effectively reduce the content of NO, which is a feasible method of controlling constipation. By regulating the expression of TRPV1, GDNF, and NOS, the normal expression of these genes in the intestine can relieve constipation, which is one of the mechanisms by which lactic acid bacteria play a role in inhibiting constipation.61 In this study, we found that the combination of KFY02 and gardenoside can significantly increase the expression of eNOS and nNOS in the small intestine, down-regulate the expression of iNOS, and relieve constipation.
Previous studies suggest that intestinal diseases are closely related to intestinal flora, and regulating the intestinal flora can reduce the impact of intestinal diseases.62 Slow transit constipation is the most common intestinal disease, it can cause intestinal flora imbalance and induce the production of harmful bacteria flagellated Gram-negative bacteria and the pathogenic microorganism Helicobacter pylori.63 Anaerobic bacteria dominates the intestinal flora of healthy people. At the level of the phylum, Firmicutes and Bacteroidetes are dominant. Bacteroidetes include three major types of bacteria: Bacteroidia, Flavobacteriia, and Sphingobacteriia. Bacteroidia is mostly found in the intestines of humans or animals. It is a common bacterium in the intestine, but can sometimes become a pathogen. In feces, Bacteroides are the main microbial species.64 Intestinal flora imbalance is mainly manifested by a decrease in the abundance of bacteria (such as Firmicutes and Lactobacillus), an increase in the abundance of beneficial bacteria (such as Bacteroides), and an increase in the content of Akkermansia. Akkermansia bacteria is a Gram-negative anaerobic bacterium, which can increase the number of intestinal goblet cells, increase the number of Treg cells, and reduce the expression of proinflammatory factors to protect the intestinal barrier function.65–67 Adjusting the proportion of these microorganisms in the intestine can improve the intestinal environment and thus relieve constipation. Here we find that the combined treatment of KFY02 and gardenoside has an effect on the richness and diversity of fecal microbial communities in mice, and helps to improve the status of intestinal microorganisms. In mice with constipation, KFY02, gardenoside, and genipin work synergistically to help prevent constipation.
This study represents the first time that the effects of KFY02 and gardenoside on the treatment of constipation have been evaluated. Combination treatment has a better effect on improving constipation in mice, and the effect is better than KFY02 and genipin alone. KFY02, gardenoside, and combination intervention groups could alleviate constipation to a certain extent, but in the combination group, KFY02 converted gardenoside to genipin, which had more significant prevention and treatment effects on intestinal diseases and was easier to absorb and utilize, therefore the active substance content could be increased to promote the inhibitory effects of gardenoside on constipation. In addition, the combination of KFY02 and gardenoside improved the intestinal microecology of mice, improved the intestinal environment, enhanced intestinal water retention and increased the water content of feces, thereby relieving constipation. KFY02 is a β-glucosidase-producing bacterium that plays an important role in the conversion between gardenoside and genipin, and has a better effects for the relief of constipation. The development and mechanism of KFY02 in functional foods requires further research.
Conflicts of interest
No conflicts of interests are related to this article.
Acknowledgements
This work was supported by the Foundation of Chongqing Science and Technology Bureau (Grants No: cstc2017shms-kjfp80011; cstc2017shms-kjfp80053), the Venture and Innovation Support Program for Chongqing Overseas Returnees (project number: cx2018095) and the Fundamental Research Funds for the Central Universities (No. XDJK2017B039, Southwest University), China. All authors have read and approved the final manuscript.
References
- Y. Hor, L. Lew, A. S. Lau, J. Ong, L. Chuah, Y. Lee, S. Choi, F. Rashid, N. Wahid, Z. Sun, L. Kwok, H. Zhang and M. Liong, J. Funct. Foods, 2018, 44, 235–245 CrossRef CAS.
- C. H. Hu, L. Q. Ren, Y. Zhou and B. C. Ye, Food Sci. Nutr., 2019, 7, 1997–2005 CrossRef CAS PubMed.
- L. Mo, H. Jin, L. Pan, Q. Hou, C. Li, I. Darima, H. Zhang and J. Yu, Food Biotechnol., 2019, 33, 174–192 CrossRef CAS.
- T. G. Elgin, S. L. Kern and S. J. McElroy, Clin. Ther., 2016, 38, 706–715 CrossRef CAS PubMed.
- C. Hill, F. Guarner, G. Reid, G. R. Gibson, D. J. Merenstein, B. Pot, L. Morelli, R. B. Canani, H. J. Flint, S. Salminen, P. C. Calder and M. E. Sanders, Nat. Rev. Gastroenterol. Hepatol., 2014, 11, 506–514 CrossRef PubMed.
- Z. Zhang, J. Lv, L. Pan and Y. Zhang, Appl. Microbiol. Biotechnol., 2018, 102, 8135–8143 CrossRef CAS PubMed.
- N. Sansotta, D. G. Peroni, S. Romano, A. Rugiano, P. Vuilleumier, G. Baviera and I. S. M. C. Italian, Society Of Pediatric Allergy, Curr. Opin. Pediatr., 2019, 31, 661–669 CrossRef PubMed.
- D. Barnes and A. M. Yeh, Nutr. Clin. Pract., 2015, 30, 747–759 CrossRef CAS PubMed.
- D. Shen, D. Zhao, X. Yang, J. Zhang, H. He and C. Yu, Eur. J. Pharmacol., 2019, 864, 172728 CrossRef PubMed.
- S. Habtemariam and G. Lentini, Biomedicines, 2018, 6, 39 CrossRef PubMed.
- J. Yuan, J. Zhang, J. Cao, G. Wang and H. Bai, Cell. Mol. Neurobiol., 2019 DOI:10.1007/s10571-019-00749-6.
- Y. S. Kim, C. Lee and J. Y. Ma, AMB Express, 2017, 7, 64, DOI:10.1186/s13568-017-0360-y.
- Y. Qian, J. Song, P. Sun, R. Yi, H. Liu, X. Feng, K. Park and X. Zhao, Molecules, 2018, 23, 1069 CrossRef PubMed.
- Y. S. Kim, C. J. Lee and J. Y. Ma, AMB Expr., 2017, 1, 2191–0855, DOI:10.1186/s13568-017-0360-y.
- M. J. Jin, I. S. Kim, D. Kim and H. H. Yoo, J. Agric. Food Chem., 2014, 62, 9632–9636 CrossRef CAS PubMed.
- G. Pérez-Gaxiola, C. A. Cuello-García, I. D. Florez and V. M. Pérez-Pico, Cochrane Database of Systematic Reviews, 2018, 4 DOI:10.1002/14651858.CD011526.pub2.
- H. Lichtenstein, Fortschr. Med., 1991, 109, 528 CAS.
- H. Suo, X. Zhao, Y. Qian, G. Li, Z. Liu, J. Xie and J. Li, Int. J. Mol. Sci., 2014, 15, 21875–21895 CrossRef CAS PubMed.
- Y. Qian, H. Suo, M. Du, X. Zhao, J. Li, G. J. Li, J. L. Song and Z. Liu, Exp. Ther. Med., 2015, 9, 272–278 CrossRef PubMed.
- J. Zhang, X. Zhou, B. Chen, X. Long, J. Mu, Y. Pan, J. Le Song, X. Zhao and Z. Yang, Appl. Sci., 2018, 8, 1–15 Search PubMed.
- J. Xu, X. Zhou, C. Chen, Q. Deng, Q. Huang, J. Yang, N. Yang and F. Huang, BMC Complementary Altern. Med., 2012, 12, 14 CAS.
- M. N. Fitch, D. Phillippi, Y. Zhang, J. Lucero, R. S. Pandey, J. Liu, J. Brower, M. S. Allen, M. J. Campen, J. D. McDonald and A. K. Lund, Environ. Res., 2019, 108913 Search PubMed.
- R. Yi, P. Peng, J. Zhang, M. Du, L. Lan, Y. Qian, J. Zhou and X. Zhao, J. Med. Food, 2019, 1–14 Search PubMed.
- X. Zhao, Y. Qian, G. Li, R. Yi, K. Park and J. Song, J. Dairy Sci., 2019, 102, 26–36 CrossRef CAS PubMed.
- F. Indrio, A. Di Mauro, G. Riezzo, E. Civardi, C. Intini, L. Corvaglia, E. Ballardini, M. Bisceglia, M. Cinquetti, E. Brazzoduro, A. Del Vecchio, S. Tafuri and R. Francavilla, JAMA Pediatr., 2014, 168, 228 CrossRef PubMed.
- M. M. Tabbers and M. A. Benninga, Clinical Evidence, 2015 Search PubMed.
- S. Kim, S. C. Choi, K. S. Park, M. I. Park, J. E. Shin, T. H. Lee, K. W. Jung, H. S. Koo and S. Myung, Journal of Neurogastroenterology and Motility, 2015, 21, 111–120 CrossRef PubMed.
- H. Su, J. Chen, S. Miao, K. Deng, J. Liu, S. Zeng, B. Zheng and X. Lu, Food Chem. Toxicol., 2019, 134, 110838 CrossRef CAS PubMed.
- R. Mukai, O. Handa, Y. Naito, S. Takayama, Y. Suyama, C. Ushiroda, A. Majima, Y. Hirai, K. Mizushima, T. Okayama, K. Katada, K. Kamada, K. Uchiyama, T. Ishikawa, T. Takagi and Y. Itoh, Dig. Dis. Sci., 2019 DOI:10.1007/s10620-019-05954-3.
- Y. Liang, P. Wen, Y. Wang, D. OuYang, D. Wang, Y. Chen, Y. Song, J. Deng, Y. Sun and H. Wang, Int. J. Mol. Sci., 2019, 20, 5721 CrossRef PubMed.
- J. Chen, D. Li, Y. Chen, H. Tao, Z. Pu, J. Zhang, Y. Tan, X. Shi, S. Yue, G. Zhou, Y. Tang and J. Duan, J. Ethnopharmacol., 2019, 238, 111868 CrossRef CAS PubMed.
- X. Xin, K. Zheng, Y. Niu, M. Song and W. Kang, Open Chem., 2018, 16, 155–162 CAS.
- Y. X. Liang, P. Wen, Y. Wang, D. M. OuYang, D. Wang, Y. Z. Chen, Y. Song, J. Deng, Y. M. Sun and H. Wang, Int. J. Mol. Sci., 2019, 20, 5721 CrossRef PubMed.
- Z. Zhuang, M. Chen, J. Niu, N. Qu, B. Ji, X. Duan, Z. Liu, X. Liu, Y. Wang and B. Zhao, Molecules, 2019, 24, 3813 CrossRef PubMed.
- L. Cong, L. Duan, W. Su, S. Hao and D. Li, Med. Sci. Monit., 2019, 25, 5028–5035 CrossRef CAS PubMed.
- X. Liu, S. Chen, Q. Yan, Y. Li and Z. Jiang, J. Funct. Foods, 2019, 57, 399–407 CrossRef CAS.
- J. Chen, D. Li, Y. Chen, H. Tao, Z. Pu, J. Zhang, Y. Tan, X. Shi, S. Yue, G. Zhou, Y. Tang and J. Duan, J. Ethnopharmacol., 2019, 238, 111868 CrossRef CAS PubMed.
- B. Liu, X. Xiao, X. Zhou, J. Zhou, L. Lan, X. Long, Y. Pan, M. Du and X. Zhao, Food Sci. Nutr., 2019, 7, 2068–2082 CrossRef CAS PubMed.
- N. Xu, W. Fan, X. Zhou, Y. Liu, P. Ma, S. Qi and B. Gu, Metab. Brain Dis., 2018, 33, 1625–1633 CrossRef CAS PubMed.
- J. Zhang, X. Zhou, B. Chen, X. Long, J. Mu, Y. Pan, J. Song, X. Zhao and Z. Yang, Appl. Sci., 2018, 8, 1498 CrossRef.
- K. Rtibi, D. Grami, S. Selmi, M. Amri, H. Sebai and L. Marzouki, Toxicol. Rep., 2017, 4, 221–225 CrossRef CAS PubMed.
- N. M. Roudsari, N. Lashgari, S. Momtaz, M. H. Farzaei, A. M. Marques and A. H. Abdolghaffari, Daru, J. Pharm. Sci., 2019 DOI:10.1007/s40199-019-00284-1.
- R. Mukai, O. Handa, Y. Naito, S. Takayama, Y. Suyama, C. Ushiroda, A. Majima, Y. Hirai, K. Mizushima, T. Okayama, K. Katada, K. Kamada, K. Uchiyama, T. Ishikawa, T. Takagi and Y. Itoh, Dig. Dis. Sci., 2019 DOI:10.1007/s10620-019-05954-3.
- M. Khushtar, H. H. Siddiqui, R. K. Dixit, M. S. Khan, D. Iqbal and M. A. Rahman, Eur. J. Integr. Med., 2016, 8, 546–551 CrossRef.
- Y. Zhou, F. Tan, C. Li, W. Li, W. Liao, Q. Li, G. Qin, W. Liu and X. Zhao, Antioxidants, 2019, 8, 524 CrossRef CAS PubMed.
- F. Han, C. Qi, Z. Lin, Y. Jia, L. Yang, J. Lu, X. Wang, E. Li, J. G. Qin and L. Chen, Aquaculture, 2020, 514, 734504 CrossRef.
- J. Wei, Q. Man, C. Ding, Y. Hu, M. Liu, H. Li, F. Guo, Y. Zhang, D. Li, L. Song, H. Yang and S. Tang, J. Proteome Res., 2019, 18, 3821–3830 CrossRef CAS PubMed.
- J. Kim and B. J. Kim, Can. J. Physiol. Pharmacol., 2019 DOI:10.1139/cjpp-2019-0452.
- J. Kim, J. Park, M. Kang, H. Choi, S. Bae, Y. Choi, Y. Lee, S. Seo, J. Hong and D. Hwang, Molecules, 2019, 24, 896 CrossRef PubMed.
- P. P. K. M. Gamage, B. A. Patel, M. S. Yeoman, R. N. Ranson and M. J. Saffrey, J. Cell. Mol. Med., 2018, 22, 5160–5164 CrossRef CAS PubMed.
- J. Yin, Y. Liang, D. Wang, Z. Yan, H. Yin, D. Wu and Q. Su, Int. J. Mol. Med., 2017, 41, 649–658 Search PubMed.
- L. Chen, J. Zhang, H. Suo, W. Wang, H. Wang, Y. Zhang, Q. Hu, X. Zhao and J. Li, Foods, 2019, 86, DOI:10.3390/foods8030086.
- B. Liu, X. Yang, L. Guo, J. Zhang, X. Zhou, R. Yi and X. Zhao, J. Food Qual., 2019, 1–13 Search PubMed.
- X. Li, Y. Liu, W. Guan, Y. Xia, Y. Zhou, B. Yang and H. Kuang, J. Ethnopharmacol., 2019, 240 Search PubMed.
- F. Li, H. Zhou, X. Zhou, R. Yi, J. Mu, X. Zhao and W. Liu, Appl. Sci., 2019, 9, 159 CrossRef CAS.
- L. Chen, J. Zhang, H. Suo, W. Wang, H. Wang, Y. Zhang, Q. Hu, X. Zhao and J. Li, Foods, 2019, 8, 86 CrossRef CAS PubMed.
- R. Tomita, S. Igarashi, S. Fujisaki and K. Tanjoh, Hepatogastroenterology, 2007, 54, 1662–1666 CAS.
- Y. Ishizuka, M. Yoshida, K. Ambe, J. Sasaki, N. Sugihara and H. Watanabe, Bull. Tokyo Dent. Coll., 2019 DOI:10.2209/tdcpublication.2019-0003.
- S. Givvimani, C. Munjal, N. Narayanan, F. Aqil, G. Tyagi, N. Metreveli and S. C. Tyagi, Am. J. Physiol.: Gastrointest. Liver Physiol., 2012, 303, G281–G290 CrossRef CAS PubMed.
- Y. L. J. Vissers, M. M. Hallemeesch, P. B. Soeters, W. H. Lamers and N. E. P. Deutz, Am. J. Physiol.: Gastrointest. Liver Physiol., 2004, 286, G747–G751 CrossRef CAS PubMed.
- V. Shah, G. Lyford, G. Gores and G. Farrugia, Gastroenterology, 2004, 126, 903–913 CrossRef CAS PubMed.
- T. Hu, P. Wen, H. Fu, G. Lin, S. Liao and Y. Zou, Food Funct., 2019, 1, 1513–1528 RSC.
- C. Liu, X. Tang, J. Yu, H. Zhang and X. Li, J. Funct. Foods, 2017, 38, 110–118 CrossRef.
- J. Round and S. Mazmanian, Nat. Rev. Immunol., 2009, 9, 313–323 CrossRef CAS PubMed.
- F. Li, T. Zhang, Y. He, W. Gu, X. Yang, R. Zhao and J. Yu, J. Ethnopharmacol., 2020, 247, 112232 CrossRef PubMed.
- Y. Wang, A. Li, L. Zhang, M. Waqas, K. Mehmood, M. Iqbal, C. Muyou, Z. Li, Y. Lian, S. Sizhu and J. Li, Microb. Pathog., 2019, 137, 103760 CrossRef CAS PubMed.
- M. Dao, A. Everard, J. Aron-Wisnewsky, N. Sokolovska, E. Prifti, E. Verger, B. Kayser, F. Levenez, J. Chilloux, L. Hoyles, M. Dumas, S. Rizkalla, J. Dore, P. Cani and K. Clement, Gut, 2016, 65, 426–436 CrossRef CAS PubMed.
|
This journal is © The Royal Society of Chemistry 2020 |
Click here to see how this site uses Cookies. View our privacy policy here.