DOI:
10.1039/C9RA10347C
(Paper)
RSC Adv., 2020,
10, 13223-13231
Xanthohumol from Humulus lupulus L. potentiates the killing of Mycobacterium tuberculosis and mitigates liver toxicity by the combination of isoniazid in mouse tuberculosis models
Received
10th December 2019
, Accepted 22nd March 2020
First published on 1st April 2020
Abstract
Anti-tuberculosis drug induced hepatotoxicity is the main problem in tuberculosis patients. Xanthohumol, a major prenyl chalcone present in hops, has diverse biological activities including antibacterial and hepatoprotective activities. The present research aimed to investigate the combined effect of xanthohumol with isoniazid against Mycobacterium tuberculosis-infected mice. The liver damage was induced by treatment with isoniazid daily for 8 weeks. During the experiment, the uninfected group and the normal control group received an equal volume of saline, the xanthohumol group received an equal volume of xanthohumol only, and the isoniazid group received an equal volume of isoniazid only. The combination therapy group received not only isoniazid but also the corresponding xanthohumol. Experimental results showed that isoniazid combined with xanthohumol resulted in the lowest lung and spleen colony-forming unit counts compared to other groups. Furthermore, other positive outcomes implied that isoniazid combined with xanthohumol obviously alleviated anti-tuberculosis drug induced liver damage as indicated by the declined levels of ALT, AST, ALP, bilirubin and MDA and the increased levels of SOD, GSH-Px and ATPases. The study of the mechanisms underlying the hepatoprotective activity showed that xanthohumol was able to activate the antioxidative defense system and protect the hepatocellular membrane. The combination of isoniazid and xanthohumol had more effective bacteriostatic and hepatoprotective activities on Mycobacterium tuberculosis-infected mice than isoniazid alone. In conclusion, xanthohumol has the potential to be an effective adjuvant in tuberculosis treatment.
Introduction
Tuberculosis (TB) is caused by Mycobacterium tuberculosis (Mtb), which remains a major global health problem with over a million deaths, more than 9 million new TB cases and about two billion people are infected in worldwide due to this disease.1 The World Health Organization (WHO) has proposed a deadline to terminate the TB epidemic by 2035. However, the main obstacle to reach this goal requires long term treatment for TB and a minimum of six months of chemotherapy to achieve a cure. Moreover, long-term chemotherapy is often accompanied by drug–drug interactions, severe liver injuries, the emergence of multi-drug resistance and infection relapse.2 Presently, the WHO recommends the administration of rifampicin and isoniazid (RIF + INH) or isoniazid (INH), the common effective treatment prescribed against TB. Unfortunately, two common anti-TB drugs (RIF/INH) can cause severe hepatic damage through a series of complicated mechanisms. Hence, TB treatment remains an obstacle due to the high rate of hepatotoxicity.3 Peroxidation of the endogenous product has been pointed out to be an important factor in the hepatotoxic action of RIF and INH. Anti-TB drugs mediated oxidative damage is usually attributed to the formation of excess reactive oxygen species (ROS), which serve as stimulators of lipid peroxidation and injury the cell membrane.4 Oxidative stress in the hepatocyte generated the change of mitochondrial permeability and induces hepatotoxicity.5 Therefore, a decrease in the duration of TB treatment is a vital issue and the development of novelty strategies that could reduce the hepatic toxic damage of TB treatment is a main goal.6
Laboratory and epidemiological researches suggest that dietary factors play a crucial role in the prevention and development of human diseases,7 including coronary heart disease, cancer, and inflammatory diseases. Hops, the female inflorescence of the hop plant (Humulus lupus L.) have long served as a flavoring agent to add aroma and bitterness to beer in the brewing industry.8 Hops are used for thousands of years to treat restlessness, insomnia, and dyspepsia in China. Modern pharmacological researches have indicated that the alcoholic extracts of hops are clinically used to treat acute bacterial dysentery, pulmonary tuberculosis, and silicosis with positive effects.8 Xanthohumol (XN) is the principal prenylated flavonoid in the hops. Recently, XN has been attracted interest because of its potential health benefits, including hepatoprotective effects,9 antiviral,10 and antibacterial activities.11 XN possesses capable of both inhibiting the production of excess ROS in vivo and suppressing peroxidation in vitro.12 Moreover, XN also assuaged liver damage and modulated oxidative reaction in the hepatitis C virus-infected Tupaia belangeri.13 Based on these researches, it would be of great interest to investigate whether XN possesses potential hepatoprotective effects on anti-TB drug-induced hepatotoxicity.
To verify our hypothesis, this research was carried out to evaluate the anti-TB activity and protective efficacy of XN in combination with INH in a murine of Mtb infection.
Material and methods
Drugs
Isoniazid and xanthohumol (purity, >98%) were purchased from Sigma (St. Louis, USA) and dissolved in dimethyl sulfoxide (Sigma, the concentration did not exceed 0.1% v/v). All other chemicals were purchased from Sinopharm Chemical Reagent Co., Ltd.
Mycobacteria and animals
Mycobacterium tuberculosis (Mtb) strain H37Rv (purchased from the Beijing Biological Product Institute, China) was cultured and grown on Lowenstein–Jensen (L–J) medium. After four weeks, bacterial colonies were obtained and homogenized in 0.03% (v/v) Tween 80 saline (Sigma) and then stored at −25 °C until use. Colony-forming units (CFUs) were calculated by plating serial dilutions on L–J medium. Pathogen-free female BALB/c mice (six weeks old and 20 ± 2 g of weight) were purchased from Zhejiang Academy of Medical Science. The mice were maintained under constant temperature and humidity with a 12 h light/dark cycles. All mice were free to access sterile water and basal diets allowed seven days of acclimation before commencing experimental researches. All animal experiment was approved by the Animal Care Use Committee of the Tongji University Shanghai Affiliated Pulmonary Hospital (no. K19-061Y) and researches were consistent with the Guide for the Care and Use of Experimental Animals (2nd edn, vol. 1, 1993, and vol. 2, 1984, available from the Canadian Council on Animal Care).
Bacterial infections and XN/INH intervention
Pathogen-free female BALB/c mice were infected with Mtb via the intranasal route with 5 × 105 CFU per mL in 20 μL volumes per mouse. Then, all mice were randomly divided into five groups (described in Table 2): uninfected group, Mtb uninfected mice and no treatment; control group, Mtb infected mice and no treatment, XN group, Mtb infected and treated with XN (10 mg kg−1); INH group, Mtb infected and treated with INH (10 mg kg−1); and XN + INH group, Mtb infected and treated with XN + INH (combination of 10 mg kg−1 XN and 10 mg kg−1 INH). Seven days after infection, mice from the five groups were treated with XN and/or INH by esophageal gavage once daily for eight weeks, the uninfected group and the normal control group received an equal volume of saline, and mice in treatment groups received an equal volume of drugs. At a specific time, mice were sacrificed and their lung and spleen were obtained and homogenized in phosphate-buffered saline (PBS). Then, the organ lysates were serial diluted in PBS and plated on Middlebrook 7H11 plates (Sigma-Aldrich, USA) to get CFUs per organ. Bacterial colony formations were calculated at the end of treatment. During the experimental period, the weights of the animal were recorded daily. After the last treatment, the mice were left to fast for 12 h and sacrificed, trunk blood collected and liver tissue obtained for biochemical analysis. The trunk blood was centrifuged at 4000 rpm for 8 min at 4 °C. Then, the separated serum was collected and stored at −25 °C for further biochemical analysis. The liver tissue was quickly collected, washed with PBS, wiped by filter paper and weighed. Thereafter, the liver tissue was homogenized with cold PBS and the homogenate was centrifuged at 4000 rpm for 10 min at 4 °C. The supernatant was collected and stored at −25 °C for further analysis.
Table 1 Additive activity between XN and INH against TB H37Rv
Compound a |
Compound b |
Combinationb |
FICc |
Interpretation |
Name |
MICa (μg mL−1) |
Name |
MICb (μg mL−1) |
MIC minimum inhibitory concentration for alone. MIC of samples in combination. The fractional inhibitory concentration (FIC) = FICa + FICb. |
XN |
36.12 |
INH |
0.028 |
5.6/0.019 |
0.83 |
Additive |
RIF |
0.268 |
12.64/0.199 |
1.09 |
Indifferent |
Streptomycin |
0.62 |
21.06/0.47 |
1.34 |
Indifferent |
Table 2 Experimental design performed in anti-tuberculosis study
Group |
Drug combination or alone |
No. of mice sacrificed at indicated time pointa |
Total no. of mice |
W−1 |
W0 |
W2 |
W4 |
W8 |
W, week. |
Uninfected |
Untreated |
|
|
|
|
10 |
10 |
Control |
Untreated |
10 |
10 |
10 |
10 |
10 |
50 |
Single drug |
XN |
10 |
10 |
10 |
10 |
10 |
50 |
Single drug |
INH |
10 |
10 |
10 |
10 |
10 |
50 |
Two-drug combinations |
XN + INH |
10 |
10 |
10 |
10 |
10 |
50 |
Total no. of mice |
|
40 |
40 |
40 |
40 |
50 |
210 |
Biochemical assays
The biochemical tests for the measurement of aspartate aminotransferase (AST), alanine aminotransferase (ALT), alkaline phosphatase (ALP), bilirubin, superoxide dismutase (SOD), glutathione peroxidase (GSH-Px) and malondialdehyde (MDA) with the commercial assay kits on the basis of the manufacturer's instructions. The assay kit was purchased from Nanjing Jiancheng Bioengineering Institute (Nanjing, China). The liver index was counted according to the equation:
Liver index (%) = mice liver weight/mice weight × 100% |
Determination of adenosine triphosphatase (ATPase) activity
The activities of Na+/K+, Mg2+, and Ca2+ ATPases were measured with the commercial assay kit according to the manufacturer's instructions. The assay kit was purchased from Nanjing Jiancheng Bioengineering Institute (Nanjing, China). The activity of ATPases in hepatic tissue homogenates is defined as μmoles of inorganic phosphorous liberated per h per g tissue.
In vitro synergistic antimycobacterial experiment
The effect of combinations of the XN with INH against TB H37Rv was determined using the checkerboard assay with minor modifications.14 To investigate the synergistic effects of XN combined with INH, 96-well microtiter plates were used containing continuous two-fold dilutions of XN (0.625–40 μg mL−1) along the vertical orientation, and streptomycin (0.25–16 μg mL−1), RIF (0.07–10 μg mL−1) or INH (0.00375–0.24 μg mL−1) along the horizontal orientation. All assays were carried out in triplicate. Fractional inhibitory concentration (FIC) was calculated by the following equation:
FICa = MICa in combination with (b)/MICa alone |
FICb = MICb in combination with (a)/MICb alone |
The FIC was interpreted as follows: FIC ≤ 0.5 = synergistic; >0.5–1.0 = additive; >1.0–≤4.00 = indifferent and >4.0 = antagonistic.15
Time kill assay
The time-kill curve method was carried out to measure the rate of killing of M. tuberculosis H37Rv within 24 h of treatment by XN or INH alone and in combination with MIC. Briefly, the drugs alone or in combination were suspended in CAMHB followed by inoculation of M. tuberculosis suspension (1.5 × 108) and incubated at 37 °C. 1 mL of sample was collected at specified time intervals of 0, 1, 4, 8, 16 and 24 h of incubation period and then diluted with PBS. 100 L of dilution was inoculated on Middlebrook 7H11 agar. All plates were incubated for 24 h at 37 °C. Then, the CFU counting was performed. Antagonism was defined as a ≥2
log10 CFU per mL increase in counts. Indifference or additive was defined as a <2
log10 CFU per mL decrease or increase in counts compared with the most active single drug alone. The synergistic effect was defined as a ≥2
log10 CFU per mL decrease in counts between the most active drug and the combination at 24 h.16
Histopathological evaluation
Small pieces of liver and lung tissue were fixed in 10% formaldehyde and subsequently embedded in paraffin. The paraffin-embedded liver and lung tissues were cut into thin sections (4–5 μm) and then stained using Hematoxylin and Eosin, and examined using the optical microscope for pathological evaluation.
Subacute toxicity study of XN
Female mice were randomly divided into five groups (groups 1, 2, 3, 4 and 5), eight mice in each group. Group 1 is used as a control group and received tap water as a vehicle, groups 2, 3, 4 and 5 mice received XN at doses of 10, 20, 40 and 80 mg kg−1 b.w. by esophageal gavage daily for three weeks, respectively. After the last treatment, the mice were left to fast for 12 h and sacrificed, trunk blood collected and liver tissue obtained for biochemical analysis.
Stability of XN in vitro
The stability study of XN was performed according to the previous report. Briefly, XN was incubated with simulated intestinal (pH = 7.2) and gastric (pH = 2) fluids under continuous vibration at 37 °C for 2 h in the water bath.17 Then, 10 mmol L−1 of XN stock solution was added to a 100 μmol L−1 of XN. Aliquots of samples were obtained and assayed by the HPLC method. The HPLC analysis was carried out on an Agilent 1260 system equipped with a C18 LiChrospher column (250 mm × 4.6 mm, 5 μm), and a quaternary pumps. Detection was at 290 and 370 nm. The mobile phase consisted of 0.1% trifluoroacetic acid (A) and methanol (B) was applied as follows: 90% (A) to 10% (A) for 25 min.
The data statistical analysis
All experimental results were reported as the means ± SD and the experiment were replicated twice. The differences between groups were estimated by one-way ANOVA and using SPSS software (version 16.0); P < 0.05 was usually considered as statistically significant.
Results
Bacterial numbers in the lungs and spleens after administration of XN and/or INH
The numbers of bacteria were measured in the lungs and spleens of mice each week after infection with Mtb H37Rv. As displayed in Fig. 1A and B, the lungs and spleens bacterial CFU began to increase and the difference in bacterial CFU was not significant at one-week post-infection. However, one week later, after administration with XN and/or INH, the bacteria CFU counts were different among the four groups. Interestingly, after two weeks of treatments, the lungs and spleens bacteria CFU counts of INH and XN + INH group began to decline, while the bacteria CFU counts of the control group always increased. After eight weeks of treatments, bacteria CFU counts in mice lungs and spleens administrated with INH alone and XN + INH were significantly declined compared to the control group and XN alone group (P < 0.01). More important, the XN + INH group displayed the lowest bacteria CFU counts, which implied that XN could improve the bactericidal activity of INH by combination therapy.
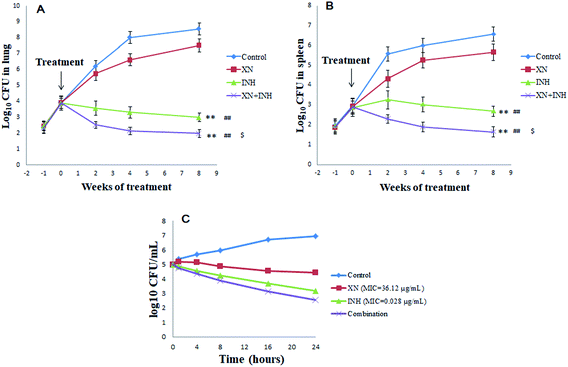 |
| Fig. 1 The colony-forming units (CFU) in mice spleens (A) and lungs (B). BALB/c mice were infected with Mycobacterium tuberculosis H37Rv strain for one week followed by 8 weeks of administration of XN, INH, or the combination of both. The bacterial CFU were declined compared to the control without drug treatment. **P < 0.01 (vs. XN alone group), ##P < 0.01 (vs. control group), $P < 0.05 (vs. INH group). Time-kill curves of XN and INH used alone and in combination against Mtb (C). | |
Additive effects between the XN and INH
In the present study, the first-line anti-TB drug (INH, RIF or streptomycin) was combined with XN to investigate possible additive effects against Mtb. Table 1 displayed additive effects of XN mixed with INH, RIF or streptomycin against the strain H37Rv. The combination of XN with INH reduced the MIC of both compounds against H37Rv-TB. FIC value was 0.83, indicative of an additive effect against Mtb. However, the RIF or streptomycin effect was not improved by XN (FIC = 1.09 and 1.34, respectively).
As shown in Fig. 1C, the time-kill curve of the XN + INH combination validated the results of the checkerboard microdilution experiment which obtained an FIC index of 0.83 for the XN + INH combination. Time-killing findings indicated a decline in the colony counts of Mtb in the combination of XN and INH. In addition, the decrease in colony counts for XN + INH combination was <2
log10 CFU per mL, compared with the INH alone as the most active drug, which indicated an additive effect against Mtb.
Effects of XN + INH on liver index
All data of the liver index in different groups were shown in Fig. 2A. After infection with Mtb H37Rv, resulting in obviously increased the liver index (4.7 ± 0.36 g/100 g) in the control group, compared with that (3.0 ± 0.24 g/100 g) in the uninfected group (P < 0.01). Obviously, the liver index in the INH group was significantly higher (P < 0.01) than that in the control group. However, the liver index of the XN + INH group was lower than the INH group (P < 0.01), and there was no obvious difference compared with the XN + INH group and the uninfected group. The result implied that the hepatic intumescence induced by INH had been mitigated after oral administration the XN + INH.
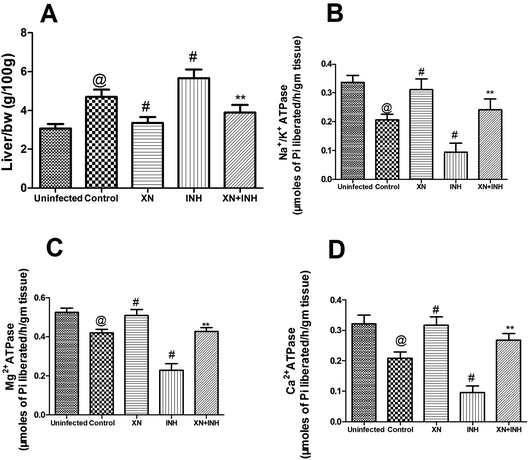 |
| Fig. 2 Effect of XN treatment on the liver index (A) Na+/K+ ATPases (B), Mg2+ ATPases (C) and Ca2+ ATPases (D) in liver tissue. | |
Effect of XN + INH treatment on activities of ATPases
As showed in Fig. 2B–D, after infection with Mtb H37Rv, resulting in obviously decreased the ATPases (Na+/K+ ATPases, 0.20 ± 0.01 μmoles of Pi liberated per h per g tissue; Mg2+ ATPases, 0.42 ± 0.01 μmoles of Pi liberated per h per g tissue; Ca2+ ATPases, 0.20 ± 0.02 μmoles of Pi liberated per h per g tissue) in control group, compared with those (Na+/K+ ATPases, 0.33 ± 0.02 μmoles of Pi liberated per h per g tissue; Mg2+ ATPases, 0.52 ± 0.02 μmoles of Pi liberated per h per g tissue; Ca2+ ATPases, 0.32 ± 0.02 μmoles of Pi liberated per h per g tissue) in uninfected group (P < 0.01). In addition, the activities of Na+/K+, Mg2+, and Ca2+ ATPases were significantly decreased in the INH alone group when compared with the control group (P < 0.01), indicating that administration with INH could induce the damage of the hepatocellular membrane. However, this reduction of the activities of ATPases was obviously assuaged in mice treated XN simultaneously with INH, indicating the XN + INH possesses the capacity towards the recovery of hepatocellular membrane instability. In addition, XN administration alone also improved the activities of above ATPases in liver tissue compared to the control group (P < 0.01).
Effect of XN + INH treatment on liver function markers
Our research was carried out to investigate the hepatoprotective effect of XN + INH in TB treatment. Generally, evaluation of the extent of liver damage was based on the analysis of serum ALT, AST, ALP, and total bilirubin. As showed in Fig. 3, after infection with Mtb H37Rv, resulting in obviously increased the liver function indexes (ALT, 44.2 ± 3.44 U L−1; AST, 76.32 ± 3.59 U L−1; ALP, 91.61 ± 2.9 U L−1; bilirubin, 0.53 ± 0.07 mg dL−1) in control group, compared with those (ALT, 25.74 ± 3.1 U L−1; AST, 56.16 ± 3.46 U L−1; ALP, 73.6 ± 3.48 U L−1; bilirubin, 0.37 ± 0.03 mg dL−1) in uninfected group (P < 0.01). In addition, the group treated with INH alone showed an obvious increase in the levels of ALT, AST, ALP, and total bilirubin when compared with the control group (P < 0.01). Conversely, co-administration of the XN along with INH exhibited a significant decline in the levels of these liver function parameters compared with the mice treated with INH alone (P < 0.01). These results indicated that the XN + INH against the hepatotoxic effect of INH.
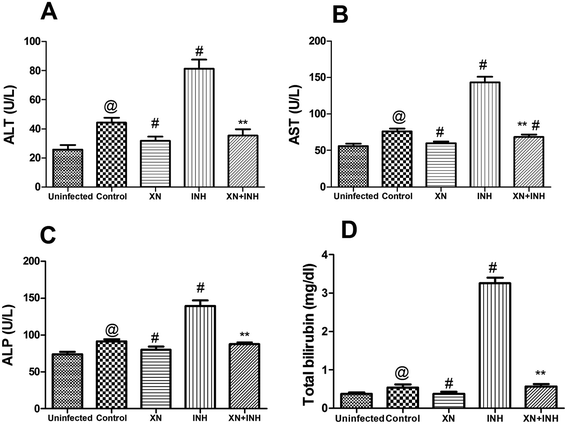 |
| Fig. 3 Effect of XN treatment on the serum levels of ALT (A), AST (B), ALP (C) and total bilirubin (D). Results are reported as the mean ± SD of ten mice per group. @P < 0.01 (vs. uninfected group), #P < 0.01 (vs. control group) and **P < 0.01 (vs. INH group). | |
XN + INH treatment reduces oxidative stress
In this study, the levels of SOD, GSH-Px, and MDA in the hepatic tissue were measured to investigate the assuage effects of XN against INH-induced oxidative stress. As displayed in Fig. 4A and B, the activities of hepatic GSH-Px and SOD were obviously decreased after administration of INH alone; however, XN + INH treatment significantly assuaged INH-induced reduction of SOD and GSH-Px when compared with the INH alone group (P < 0.01). In addition, as displayed in Fig. 4C, the content of MDA in hepatic tissue was obviously increased after the administration of INH alone. However, XN + INH treatment significantly attenuated the INH-induced elevation of MDA when compared to the INH alone group (P < 0.01).
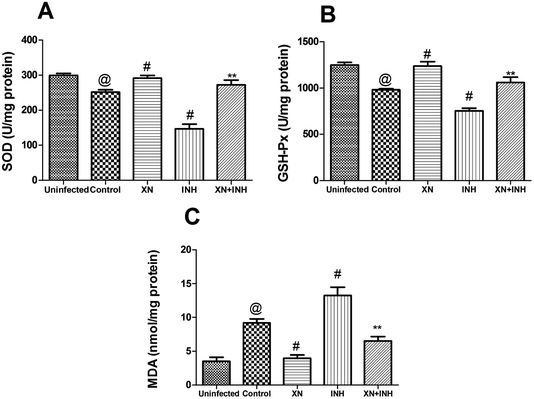 |
| Fig. 4 Effect of XN treatment on the activities of SOD (A), GSH-Px (B) and the content of MDA (C) in liver tissue. Results are reported as the mean ± SD of ten mice per group. @P < 0.01 (vs. uninfected group), #P < 0.01 (vs. control group) and **P < 0.01 (vs. INH group). | |
Histological studies
Histological studies were performed by HE stained liver and lung tissue sections. As shown in Fig. 5A, the uninfected group emerged normal architecture of hepatocytes around portal triads and central veins. However, the INH group of mice emerged altered histoarchitecture, including enlarged vacuolation, hepatocytes and inflammatory cells infiltrates. Administration of INH + XN caused the recovery of hepatocytes and minor hepatic damage.
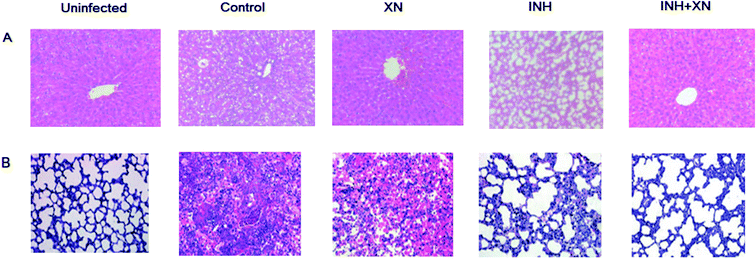 |
| Fig. 5 (A) Pathological evaluations of liver tissue stained with H&E using light microscopy (magnification: ×100). (B) Pathological evaluations of lung tissue stained with H&E using light microscopy (magnification: ×100). | |
As shown in Fig. 5B, the uninfected group emerged the normal architecture of lung tissue. The control group of mice emerged altered histoarchitecture, including granulomatous lesions, inflammation, and perivasculitis, as compared with the uninfected group. However, the combination of INH + XN treatment resulted in obviously decreased lung pathology, as compared to treatment with XN or INH alone. So, our findings revealed that XN decreases inflammatory lung pathology in Mycobacterium tuberculosis-infected mice.
Subacute toxicity study
During the subacute toxicity experimental period, mice received XN at the doses of 10, 20, 40 and 80 mg kg−1 daily for three weeks did not result in any visible symptoms of toxicity, including changes in mucous membranes, fur, eyes, and skin. In addition, these mice did not appear any behavioral changes such as diarrhea, lethargy, and coma. As shown in Fig. 6, there was no obvious difference in the biochemical parameters (ALT, AST, ALP, and total bilirubin) of mice between the control and treated groups (P > 0.05). Similarly, as shown in Fig. 7, there was no statistical difference in the liver index and bodyweight of mice between control and treated groups (P > 0.05). Therefore, the oral administration of XN to mice at doses of 10, 20, 40 and 80 mg kg−1 body weight daily for three weeks is safe.
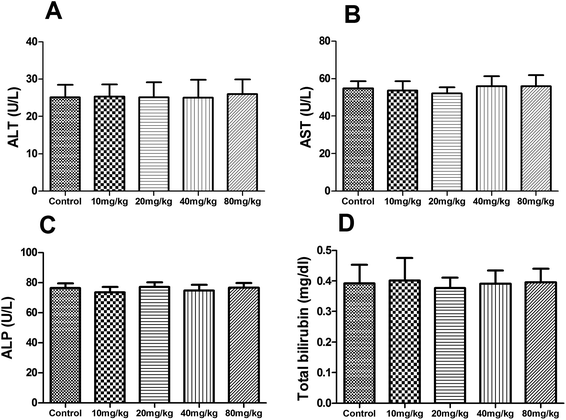 |
| Fig. 6 Effects of XN on serum biochemical parameters of mice during three weeks of subacute toxicity study. | |
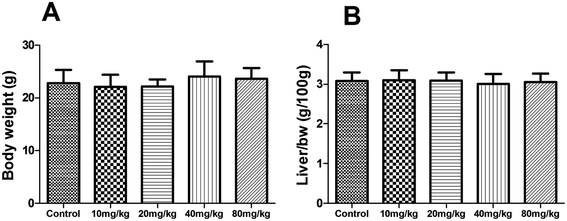 |
| Fig. 7 Effects of XN on body weight (A) and liver index (B) of mice during three weeks of subacute toxicity study. | |
Stability study of XN in vitro
As shown in Fig. 8, the incubation with intestinal fluids resulted in an XN recovery of 82.74 ± 5.38% after 1 h incubation and 46.36 ± 8.77% after 2 h incubation. However, the incubation with gastric fluids resulted in an XN recovery of 24.54 ± 10.57% after 1 h incubation.
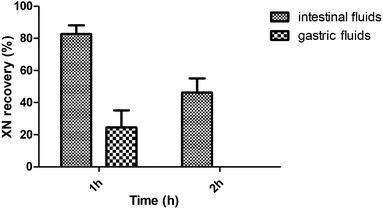 |
| Fig. 8 Stability study of XN in vitro. Results were reported as the mean ± SD. | |
Discussion
Nowadays, in spite of obvious advances in the treatment of TB, the occurrence of new multidrug-resistant TB and extensively drug-resistant TB is increasing and remains a worldwide health concern. In addition, hepatotoxicity is a common side effect during the treatment of TB. Many morphological and metabolic aberrations are commonly occurred by the administration of RIF or INH because the liver is a major detoxifying place for these anti-TB drugs.18 Hence, the development of a novelty strategy to reduce the hepatotoxicity of these anti-TB drugs without decreasing their efficacy would be desirable.
In order to investigate whether the XN can synergistically influence the therapeutic effect of INH treatment, we treated the Mtb infected mice with a combination of XN and INH. In the present research, we observed that there was an improvement in the therapeutic effect of INH when combined with XN. Our results implied that the antibacterial effect of INH was enhanced by combination with XN in Mtb infected female mice model. Although the mechanism of improving the antibacterial activity and reducing the liver damage of anti-TB drugs in combination with XN is not fully understood, it speculated that the improvement effect of XN may be related to its synergistic antibacterial activity.11 The combination of XN and INH protects against TB by suppressing bacterial burden.
As we known, aminotransferases are a vital class of enzymes that link amino acids and carbohydrate metabolism, thus clearly building the connection between the intermediates of the amino acids and the citric acid cycle. Clinically, the serum levels of AST, ALT, ALP, and bilirubin are well known diagnostic indices of hepatic disease. In cases of hepatic injury with hepatic parenchymal cell necrosis and hepatocellular lesions, these diagnostic indicators are secreted from the injured liver tissue into the bloodstream.19 In the current research, the activities of these enzymes were obviously increased in the INH group of mice that were treated anti-TB drugs, indicating that hepatocellular damage induced by anti-TB drugs and our present result is in accordance with previous study.20 However, treatment with XN and INH effectively declined these liver function biomarkers. The results implied that the combination of XN and INH could effectively inhibit liver damage in TB treatment.
As a previous report, peroxidation of membrane phospholipids not only changes the functional integrity of cell membranes but also influences the activities of the membrane-bound enzymes, such as Na+/K+, Mg2+ and Ca2+ ATPases.21 Liver damage also influences transportation through cell membranes by disrupting the membrane fluidity and inhibiting the activities of Na+/K+, Mg2+ and Ca2+ ATPases. In addition, these ATPases are also sensitive to ROS mediated oxidative stress.22 In the present research, as shown in the INH group, observation of significant decline in the activities of these ATPases, which indicating that INH treatment induced the injury of the hepatocellular membrane and changed the fluidity of the hepatocellular membrane. And these adversities may be related to the INH-induced oxidative stress. Previous researches are in agreement with our current results. This research both indicated that treated with INH could reduce the activities of ATPases in hepatic tissue.23
Earlier researches have demonstrated that INH-induced liver damage was related to the occurrence of oxidative stress. These anti-TB drugs cause a series of hepatocellular injury through the motivation of oxidative stress, an outcome of dysfunction of liver tissue antioxidant defense system.24 The formation of MDA is a classical indication of oxidative stress and induced by free radicals. GSH-Px and SOD act mutually to neutralize ROS and against the free radicals induced liver tissue injury to assuage oxidative stress.25 In the present study, we observed that the activities of liver tissue GSH-Px and SOD were obviously decreased. Conversely, the content of liver tissue MDA was markedly increased after INH administration. These results implied that oxidative stress plays a partial role in INH-induced hepatic damage. In the present study, we observed that XN + INH pretreatment significantly improved the activities of GSH-Px and SOD. Meanwhile, XN + INH pretreatment markedly decreased the content of MDA. These results of current research indicating that XN + INH might suppress lipid peroxidation and effectively recover the antioxidative defense system in TB treatment. A similar result was obtained in previous research, co-administration of kolaviron (a biflavonoid from Garcinia kola seeds) with anti-TB drugs restores antioxidant status, mitigates lipid peroxidative damage, and normalizes markers of hepatic injury in the rats.26
Indeed, XN possesses the capacity of both inhibiting the production of free radicals in vivo and suppressing peroxidation in vitro.12,27 Moreover, XN assuaged carbon tetrachloride-induced acute liver damage in rats and reduced liver injury, modulated oxidative reaction in hepatitis C virus-infected Tupaia belangeri.9,13 Hence, these results afforded interesting reference for the hepatoprotection effect of XN against INH-induced liver damage. In the current research, a preliminary investigation of the potential mechanism underlying the hepatoprotective activities of XN was carried out and manifested a free radical scavenging activity and hepatocellular membrane protective effect. Other probable mechanisms through which XN interferes with INH-induced hepatotoxicity will be demonstrated in further study. Needless to say, further validation in male mice will be necessary before this promising result can be realized.
In conclusion, the major finding was the improved anti-TB activity of INH by a combination of XN in Mtb infected female mice. In the development of anti-TB therapy, the main advantage should be the capacity to assuage the INH-induced hepatic damage by XN co-administration, demonstrating a possible hepatoprotective effect of XN against INH-induced hepatitis. The present results might provide a new perspective for the use of XN combined with INH during clinical therapy, which might assuage the hepatic damage and shorten the duration of TB treatment. In future researches, insights should be obtained into the clinical impact of the combination of XN and INH.
Conflicts of interest
The authors declare no conflict of interest.
Acknowledgements
This work is supported by the program of multi-dimensional diagnostic strategies and innovative product development for tuberculosis (2018ZX10302301).
References
- C. Lienhardt, K. Lönnroth, D. Menzies, M. Balasegaram, J. Chakaya, F. Cobelens, J. Cohn, C. Denkinger, T. Evans, G. Källenius, G. Kaplan, A. Kumar, L. Matthiessen, C. Mgone, V. Mizrahi, Y. Mukadi, V. Nguyen, A. Nordström, C. Sizemore, M. Spigelman, S. Squire, S. Swaminathan, P. Van Helden, A. Zumla, K. Weyer, D. Weil and M. Raviglione, PLoS Med., 2016, 13, e1001965 CrossRef PubMed
. - P. Ziakas and E. Mylonakis, Clin. Infect. Dis., 2009, 49, 1883–1889 CrossRef PubMed
. - M. Singh, V. Gupta, D. Amarapurkar, J. Joshi, R. Baijal, P. Ramegowda, A. Amarapurkar and P. Wangikar, Infect., Genet. Evol., 2014, 23, 42–48 CrossRef CAS PubMed
. - S. Tasduq, K. Peerzada, S. Koul, R. Bhat and R. Johri, Hepatol. Res., 2005, 31, 132–135 CrossRef CAS PubMed
. - M. Sankar, J. Rajkumar and J. Devi, Pak. J. Pharm. Sci., 2015, 28, 983–990 CAS
. - S. Khasnobis, V. Escuyer and D. Chatterjee, Expert Opin. Ther. Targets, 2002, 6, 21–40 CrossRef CAS PubMed
. - W. Willett, Science, 1994, 264, 532–537 CrossRef CAS PubMed
. - P. Zanoli and M. Zavatti, J. Ethnopharmacol., 2008, 116, 383–396 CrossRef CAS PubMed
. - C. Pinto, A. L. Duque, B. Rodríguez-Galdón, J. J. Cestero and P. Macías, Food Chem. Toxicol., 2012, 50, 3405–3412 CrossRef CAS PubMed
. - N. Zhang, Z. Liu, Q. Han, J. Chen and Y. Lv, Phytomedicine, 2010, 17, 310–316 CrossRef CAS PubMed
. - P. Natarajan, S. Katta, I. Andrei, V. Babu Rao Ambati, M. Leonida and G. J. Haas, Phytomedicine, 2008, 15, 194–201 CrossRef CAS PubMed
. - C. Pinto, J. J. Cestero, B. Rodríguez-Galdón and P. Macías, Toxicol. Rep., 2014, 1, 726–733 CrossRef CAS PubMed
. - M. Yang, N. Li, F. Li, Q. Zhu, X. Liu, Q. Han, Y. Wang, Y. Chen, X. Zeng, Y. Lv, P. Zhang, C. Yang and Z. Liu, Int. Immunopharmacol., 2013, 16, 466–474 CrossRef CAS PubMed
. - F. Iten, R. Saller, G. Abel and J. Reichling, Planta Med., 2009, 75, 1231–1236 CrossRef CAS PubMed
. - S. van Vuuren and A. Viljoen, Planta Med., 2011, 77, 1168–1182 CrossRef CAS PubMed
. - N. Xu, G. Wang, Y. Leng, X. Dong, F. Chen and Q. Xing, Exp. Ther. Med., 2018, 16, 3485–3491 Search PubMed
. - L. Hanske, G. Loh, S. Sczesny, M. Blaut and A. Braune, J. Nutr., 2009, 139, 1095–1102 CrossRef CAS PubMed
. - S. Santhosh, T. K. Sini, R. Anandan and P. T. Mathew, Eur. J. Pharmacol., 2007, 572, 69–73 CrossRef CAS PubMed
. - M. Madariaga, N. Engl. J. Med., 2006, 354, 2191–2193 CrossRef PubMed
. - I. Okwa, A. Akindele, E. Agbaje, O. Oshinuga, C. Anunobi and O. Adeyemi, EXCLI J., 2013, 12, 231–250 Search PubMed
. - K. Devi, M. Sreepriya, K. Balakrishna and T. Devaki, J. Ethnopharmacol., 2004, 93, 371–375 CrossRef PubMed
. - S. Jain and S. Shohet, Biochim. Biophys. Acta, 1981, 642, 46–54 CrossRef CAS
. - S. Tasduq, K. Singh, N. Satti, D. Gupta, K. Suri and R. Johri, Hum. Exp. Toxicol., 2006, 25, 111–118 CrossRef CAS PubMed
. - S. Bhadauria, R. Mishra, R. Kanchan, C. Tripathi, A. Srivastava, A. Tiwari and S. Sharma, Toxicol. Mech. Methods, 2010, 20, 242–251 CrossRef CAS PubMed
. - B. Halliwell, Nutr. Rev., 2012, 70, 257–265 CrossRef PubMed
. - O. A. Adaramoye, A. O. Kehinde, A. Adefisan, O. Adeyemi, I. Oyinlola and O. O. Akanni, Tokai J. Exp. Clin. Med., 2016, 41, 14–21 CAS
. - N. Yamaguchi, K. Satoh-Yamaguchi and M. Ono, Phytomedicine, 2009, 16, 369–376 CrossRef CAS PubMed
.
Footnote |
† These authors contributed equally to this work. |
|
This journal is © The Royal Society of Chemistry 2020 |